ABSTRACT
Somatic recombination is essential to protect genomes of somatic cells from DNA damage but it also has important clinical implications, as it is a driving force of tumorigenesis leading to inactivation of tumor suppressor genes. Despite this importance, our knowledge about somatic recombination in adult tissues remains very limited. Our recent work, using the Drosophila adult midgut has demonstrated that spontaneous events of mitotic recombination accumulate in aging adult intestinal stem cells and result in frequent loss of heterozygosity (LOH). In this Extra View article, we provide further data supporting long-track chromosome LOH and discuss potential mechanisms involved in the process. In addition, we further discuss relevant questions surrounding somatic recombination and how the mechanisms and factors influencing somatic recombination in adult tissues can be explored using the Drosophila midgut model.
Introduction
The genomes of somatic cells remain under a constant threat of DNA damage from both endogenous and external insults. Although complex mechanisms have evolved to cope with such damage, in some cases imprecise repair results in the occurrence of somatic mutations. In short, DNA repair may proceed by mechanisms involving direct ligation of broken DNA strands in the process of non-homologous end joining (NHEJ) or DNA lesions can be repaired using homologous sequences in a process called homologous recombination (HR). In contrast to NHEJ, which is frequently imprecise, HR is often described as an “error-free” DNA repair mechanism. However, growing evidence suggests that HR-related mechanisms can be highly mutagenic leading to base pair substitutions, insertions or deletions (indels) and chromosome rearrangements.Citation1-5 In diploid cells, if a DNA lesion is repaired using the unbroken homologous chromosome as a template, it may lead to mitotic recombination. If the homologous chromosomes are heterozygous for given loci, mitotic recombination may result in loss of heterozygosity (LOH; ).
Figure 1. Mechanisms and genetic outcomes of mitotic recombination. Black and red lines indicate 2 homologous chromosomes of a diploid cell and circles indicate centromeres. Upon DNA replication, chromosomes consist of 2 sister chromatids. (A) During a reciprocal crossover event, chromatid arms are exchanged between the homologs after a strand-specific cleavage of a double Holliday junction intermediate. If in the following division, recombinant molecules segregate to different cells, LOH occurs in both daughter cells. (B) A nonreciprocal transfer of genomic sequence between the homologues leads to a gene conversion event and LOH in one of the daughter cells. (C) Break-induced replication leads to a nonreciprocal transfer of genomic sequence extending until the end of a chromosome arm. LOH occurs in one of the 2 daughter cells.
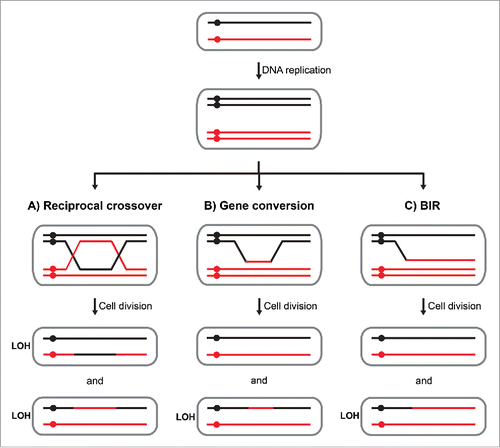
Mitotic recombination in somatic cells, also referred to as somatic recombination, was first reported in Drosophila.Citation6 Since then, a number of studies have used fruit flies to study rare events of somatic recombination occurring during development.Citation7-10 Furthermore, investigations in yeast have provided important insights into the biology of mitotic recombination.Citation11-14 Somatic recombination may arise through a reciprocal crossover event leading to the exchange of homologous chromosome arms (). Alternatively, DNA sequences from the unbroken homolog may be copied, without a reciprocal exchange, in the process of gene conversion (). In some cases, nonreciprocal transfer of genomic sequences from an unbroken chromosome extends until the end of a chromosome arm in a replicative mechanism called break-induced replication (BIR; ).Citation15-17
It is well known that in mammalian somatic cells, mitotic recombination occurs and can be modulated by a genetic background.Citation18-21 However, the process of somatic recombination still remains poorly understood and difficult to study in higher model systems.
Why is it important?
LOH plays an important role in cancer and additional human pathologies. A number of familial cancers have been shown to arise as a consequence of germline mutations in one allele and subsequent somatic inactivation of the second functional allele. For example, somatic LOH of the adenomateous polyposis (Apc) gene is well recognized as a cause of colorectal cancer and studies of heterozygous mutant Apc−/+ mouse models have proven that LOH is frequently caused by somatic recombination.Citation22-28 Somatic recombination also underlies clinical cases of spontaneous revertants in skin and blood diseases.Citation29-31 Finally, in addition to these well-documented examples, in theory, LOH has the potential to modify many other disease phenotypes. Despite the biological and clinical importance of somatic recombination, many questions regarding this process remain unanswered. In particular, relatively little is known about molecular mechanisms of recombination as well as factors initiating and regulating recombination rates in somatic tissues.
Somatic recombination in the Drosophila adult midgut
In our recent work, we uncovered high rates of spontaneous mutation in aging Drosophila adult intestinal stem cells (ISCs).Citation32 At least 2 distinct mechanisms contribute to such mutation. First, we identified that spontaneous deletions and complex rearrangements occur on the X chromosome in male flies, leading to inactivation of a tumor suppressor gene Notch and formation of intestinal neoplasia. Such neoplasia do not arise spontaneously in wild-type female flies, which have 2 X chromosomes. However, in females heterozygous mutant for Notch, frequent events of LOH occur in aging midguts resulting from the inactivation of the wild type copy of Notch. In addition, our data using other marker genes demonstrated that LOH occurs throughout the genome and suggested that it proceeds by a mitotic recombination-based mechanism. The evidence in support of LOH via mitotic recombination is as follows: LOH frequency in aged ISCs is higher in presence of a homologous chromosome and depends on the position of the studied marker, with genes located distally lost more frequently than those more proximal to the centromere. Furthermore, the LOH frequency is reduced in the presence of a balancer chromosome, which suppresses recombination between homologs. By further exploring this in vivo model system it will now be possible to better understand how somatic recombination occurs in adult tissue-specific stem cells.
What mechanisms underlie somatic recombination in adult tissues?
In order to gain more insight into the nature of mitotic recombination events occurring spontaneously in aging Drosophila ISCs, we further explored our LOH assay where flies heterozygous for mutant Notch and a GAL80 repressor are used. In this system, LOH of Notch in a stem cell leads to a clonal Notch loss of function phenotype, whereas LOH of the GAL80 repressor results in a GAL4-driven GFP expression, both of which can be visualized by immunolabelling (). We previously demonstrated that when GAL80 is inserted around 18 Mb away from the centromere and close the wild-type Notch locus, the vast majority (93.9%) of phenotypically Notch mutant LOH clones are also GFP positive indicating simultaneous inactivation of GAL80 ().Citation32 This result can be explained by recombination events initiated at positions on the X chromosome anywhere between the centromere and the GAL80 insertion site. In order to further investigate the location of mitotic recombination events, we have now changed the position of the GAL80 and placed it close to the centromere (2.5 Mb) (). Interestingly, again a large fraction of LOH events (84.2%, n = 57 clones analyzed) showed inactivation of both Notch and GAL80.
Figure 2. Centromere-proximal bias of spontaneous LOH events in aging Drosophila ISCs. (A) Example of an aged female midgut heterozygous for an X-linked Notch mutation and a GAL80 transgene insertion proximal to the centromere. Dashed yellow lines indicate spontaneous LOH clones. Clonal loss of Notch is evident by accumulation of cells positive for Dl (cytosolic red in A, or cytosolic gray in A') or Prospero (nuclear red in A, or nuclear gray in A') markers. Loss of GAL80 activity is reflected by GFP expression (green in A, gray in A″). Shown are 3 clonal events: 2 with concomitant inactivation of GAL80 therefore expressing GFP, and 1 with only Notch LOH. Scale bar=50μm. (B-C) Schematic representations of somatic recombination events that could lead to identified clonal phenotypes in midguts with 2 different GAL80 insertion sites: distal (B) and proximal (C) to the centromere on the X chromosome. Yellow boxes represent regions of potential recombination initiation sites. For simplicity only the daughter cell in which LOH occurred is shown. Crossover-type events are represented, however BIR could also be involved. Fly crosses, aging and immunofluorescence were performed as previously described.Citation32
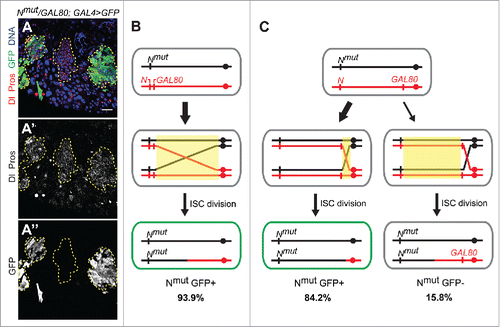
This finding has several important implications. First, it suggests that majority of the spontaneous recombination events occurring on the X chromosome have initiated within the 2.5 Mb region between the centromere and the GAL80 insertion at the cytological position 19E. Secondly, these data imply that the recombination events occur quite close to the centromere and lead to LOH of almost entire length of the chromosome arm covering as much as 20 Mb. Thirdly, these data argue against gene conversion as a primary mechanism, as gene conversion usually results in the copying of relatively small DNA tracts (up to about 100 kb in yeast)Citation33-35 and suggests the use of either crossover or break-induced replication (BIR) mechanisms. To further distinguish between relative contributions of these 2 recombination-driven mechanisms it will be necessary to make use of the Drosophila genetic tools to modify our assay in such a way that both ISC daughter cells can be followed after a recombination event. The remaining 15.8% of LOH events, which showed Notch loss of function phenotype but were not GFP positive, likely resulted from recombination events initiated between the GAL80 insertion and the Notch locus ().
Importantly, the centromere proximal “hot-spot” on the X-chromosome may not be the same on other chromosomes. In support of that notion, we have previously reported that LOH of a marker proximal to the centromere of the third chromosome is much less frequent than for the X chromosome.Citation32 Thus, this observation suggests that LOH frequencies are not only dependent on the distance from the centromere, but also implies that some genomic regions are more prone to mitotic recombination than others. Similarly, a nonrandom distribution of mitotic crossover events has been reported in the Drosophila male and female germlines, in yeast as well as mammalian models.Citation12,36-39
What defines chromosomal locations of mitotic recombination?
If somatic recombination sites are not equally distributed along chromosome arms, what determines regions of the genome with higher or lower recombination frequencies? The location of the genomic initiation site of recombination events is likely directly linked to the type of event that promotes somatic recombination in the first place. Homologous recombination DNA repair pathways can be triggered in response to double-strand breaks, single-stranded gaps or stalled replication forks. However, the exact nature of the spontaneous lesions initiating somatic recombination in adult tissues is unknown and should be further investigated.
Recent studies have demonstrated that instability of chromosome fragile sites can lead to frequent mitotic recombination and LOH.Citation34,37,40 In mammalian cells and yeast, such chromosome fragile sites are structurally prone to undergo DNA breaks under conditions of replication stress due to DNA sequence features or delayed replication timing.Citation41-44 Regions sensitive to such instability can involve repetitive DNA sequences (such as rDNA repeats), centromeres, tRNA genes, DNA secondary structure features or large and highly transcribed genes.Citation45-49 It remains to be addressed if these sites also promote mitotic recombination in somatic cells of higher organisms.
What factors modify somatic recombination?
An assay for a spontaneous LOH in human lymphocytes performed on a population of adults, revealed large inter-individual variation in mitotic recombination frequencies.Citation50 The factors underlying such variation are unknown, but both genetic and environmental influences are likely to be involved.
A large number of mutant yeast strains have been isolated with a so-called mitotic hyper-recombination (or hyper-rec) phenotype (reviewed in ref.Citation51). Among these are mutants of genes involved in DNA repair, DNA replication, transcription or cell cycle control, whose normal function is to suppress somatic recombination. However, in higher eukaryotes, genetic factors modulating the rates of somatic recombination remain largely unknown. Increased recombination rates have been reported in flies, mice and human cells mutant for the Bloom syndrome protein (Blm), a DNA helicase suppressing inappropriate homologous replication.Citation20,21,52-54 Furthermore, a number of reports have identified genomic loci functioning as modifiers of LOH of Apc in mouse models of colorectal carcinoma further indicating that genetic background can have important implications for somatic recombination frequencies.Citation55-60
Interestingly, elegant work on Apc−/+ mice with chromosomal translocations revealed that genetic background could impact LOH through modifying nuclear architecture and chromosome proximity in somatic nuclei.Citation28 In Drosophila (and other Diptera insects) homologous chromosome pairing is maintained not only in meiotic but also somatic cells, potentially facilitating the use of the homologous chromosome during DNA repair.Citation61,62 However, chromosome pairing also exists in mammalian cells, though appears not to affect the entire chromosome, instead being loci- and cell-type dependent.Citation63-67 Furthermore, somatic recombination rates in Apc−/+ mice are modified in animals mutant for cohesion, further suggesting an important role of chromosome architecture and pairing in somatic recombination.Citation68,69
Apart from genetic influences, environmental factors could also impact somatic recombination rates in adult tissues. A somatic recombination test developed to measure recombination events occurring during wing formation in Drosophila has been widely used to test genotoxicity of different compounds.Citation70 It will now be interesting to use the assays and tools that we have developed to address how recombination rates in adult tissues can be modified, not only by exposure to genotoxic agents, but also by differential diet or presence of commensal or pathogenic bacteria.
Last but not least, the most important risk factor in accumulation of somatic mutation and cancer formation is age.Citation71 LOH frequencies increase during replicative aging in yeast as well as in aged human lymphocytes,Citation72-74 however the exact impact of aging on mitotic recombination in adult somatic tissues remains unclear. It will be interesting to address differences between young and aged adult tissue stem cells in respect to the rates of spontaneous LOH but also chromosomal locations of spontaneous recombination events.
Conclusions
In contrast to meiotic recombination, which creates genome diversity driving evolution, mechanisms of mitotic recombination are much less understood. Mitotic recombination has important consequences on adult tissues, creating genetic mosaicism, promoting tumor formation and having the potential to modify disease phenotypes. A great majority of what we know about the process comes from studies in unicellular organisms. The use of higher model organisms, and in particular the fly, will be crucial in addressing what drives and regulates somatic recombination in complex tissues in vivo.
Disclosure of potential conflicts of interest
No potential conflicts of interest were disclosed.
Acknowledgments
We acknowledge the Cell and Tissue Imaging Platform of the Genetics and Developmental Biology Department (UMR3215/U934) of Institut Curie, member of France-Bio imaging (ANR-10-INSB-04), for help with confocal microscopy.
Funding
The Bardin lab is supported by grants from Worldwide Cancer Research, Fondation pour la Recherche Medicale, La Ligue Contre le Cancer, and the Fondation ARC, as well as funding from the program “Investissements d'Avenir” launched by the French Government and implemented by ANR, references Labex DEEP (ANR-11-LBX-0044) and IDEX PSL (ANR-10-IDEX-0001-02 PSL).
References
- Hicks WM, Kim M, Haber JE. Increased mutagenesis and unique mutation signature associated with mitotic gene conversion. Science 2010; 329:82-5; PMID:20595613; http://dx.doi.org/10.1126/science.1191125
- Deem A, Keszthelyi A, Blackgrove T, Vayl A, Coffey B, Mathur R, Chabes A, Malkova A. Break-induced replication is highly inaccurate. PLoS Biol 2011; 9:e1000594; PMID:21347245; http://dx.doi.org/10.1371/journal.pbio.1000594
- Carvalho CM, Lupski JR. Copy number variation at the breakpoint region of isochromosome 17q. Genome Res 2008; 18:1724-32; PMID:18714090; http://dx.doi.org/10.1101/gr.080697.108
- Smith CE, Llorente B, Symington LS. Template switching during break-induced replication. Nature 2007; 447:102-5; PMID:17410126; http://dx.doi.org/10.1038/nature05723
- Rodgers K, McVey M. Error-prone repair of DNA double-strand breaks. J Cell Physiol 2016; 231:15-24; PMID:26033759; http://dx.doi.org/10.1002/jcp.25053
- Stern C. Somatic crossing over and segregation in drosophila melanogaster. Genetics 1936; 21:625-730; PMID:17246815
- Kaplan WD. The influence of minutes upon somatic crossing over in drosophila melanogaster. Genetics 1953; 38:630-51; PMID:17247462
- Brosseau GE, Jr. The environmental modification of somatic crossing over in Drosophila melanogaster with special reference to developmental phase. J Exp Zool 1957; 136:567-93; PMID:13525600; http://dx.doi.org/10.1002/jez.1401360309
- Weaver EC. Somatic crossing over and its genetic control in drosophila. Genetics 1960; 45:345-57; PMID:17247929
- Ronen A. Interchromosomal effects on somatic recombination in drosophila melanogaster. Genetics 1964; 50:649-58; PMID:14221873
- Andersen SL, Zhang A, Dominska M, Moriel-Carretero M, Herrera-Moyano E, Aguilera A, Petes TD. High-resolution mapping of homologous recombination events in rad3 hyper-recombination mutants in yeast. PLoS Genet 2016; 12:e1005938; PMID:26968037; http://dx.doi.org/10.1371/journal.pgen.1005938
- Lee PS, Greenwell PW, Dominska M, Gawel M, Hamilton M, Petes TD. A fine-structure map of spontaneous mitotic crossovers in the yeast Saccharomyces cerevisiae. PLoS Genet 2009; 5:e1000410; PMID:19282969; http://dx.doi.org/10.1371/journal.pgen.1000410
- Symington LS, Rothstein R, Lisby M. Mechanisms and regulation of mitotic recombination in Saccharomyces cerevisiae. Genetics 2014; 198:795-835; PMID:25381364; http://dx.doi.org/10.1534/genetics.114.166140
- Yim E, O'Connell KE, St. Charles J, Petes TD. High-resolution mapping of two types of spontaneous mitotic gene conversion events in Saccharomyces cerevisiae. Genetics 2014; 198:181-92; PMID:24990991; http://dx.doi.org/10.1534/genetics.114.167395
- Haber JE. DNA recombination: the replication connection. Trends Biochem Sci 1999; 24:271-5; PMID:10390616; http://dx.doi.org/10.1016/S0968-0004(99)01413-9
- Morrow DM, Connelly C, Hieter P. “Break copy” duplication: a model for chromosome fragment formation in Saccharomyces cerevisiae. Genetics 1997; 147:371-82; PMID:9335579
- Esposito MS, Ramirez RM, Bruschi CV. Nonrandomly-associated forward mutation and mitotic recombination yield yeast diploids homozygous for recessive mutations. Curr Genet 1994; 26:302-7; PMID:7882423; http://dx.doi.org/10.1007/BF00310493
- Shao C, Deng L, Henegariu O, Liang L, Raikwar N, Sahota A, Stambrook PJ, Tischfield JA. Mitotic recombination produces the majority of recessive fibroblast variants in heterozygous mice. Proc Natl Acad Sci U S A 1999; 96:9230-5; PMID:10430925; http://dx.doi.org/10.1073/pnas.96.16.9230
- Gupta PK, Sahota A, Boyadjiev SA, Bye S, Shao C, O'Neill JP, Hunter TC, Albertini RJ, Stambrook PJ, Tischfield JA. High frequency in vivo loss of heterozygosity is primarily a consequence of mitotic recombination. Cancer Research 1997; 57:1188-93; PMID:9067291
- Luo G, Santoro IM, McDaniel LD, Nishijima I, Mills M, Youssoufian H, Vogel H, Schultz RA, Bradley A. Cancer predisposition caused by elevated mitotic recombination in Bloom mice. Nat Genet 2000; 26:424-9; PMID:11101838; http://dx.doi.org/10.1038/82548
- Goss KH, Risinger MA, Kordich JJ, Sanz MM, Straughen JE, Slovek LE, Capobianco AJ, German J, Boivin GP, Groden J. Enhanced tumor formation in mice heterozygous for Blm mutation. Science 2002; 297:2051-3; PMID:12242442; http://dx.doi.org/10.1126/science.1074340
- Spirio LN, Samowitz W, Robertson J, Robertson M, Burt RW, Leppert M, White R. Alleles of APC modulate the frequency and classes of mutations that lead to colon polyps. Nat Genet 1998; 20:385-8; PMID:9843214; http://dx.doi.org/10.1038/3865
- Cottrell S, Bodmer WF, Bicknell D, Kaklamanis L. Originally published as Volume 2, Issue 8820Molecular analysis of APC mutations in familial adenomatous polyposis and sporadic colon carcinomas. The Lancet 1992; 340:626-30; http://dx.doi.org/10.1016/0140-6736(92)92169-G
- Luongo C, Moser AR, Gledhill S, Dove WF. Loss of Apc+ in intestinal adenomas from Min mice. Cancer Research 1994; 54:5947-52; PMID:7954427
- Oshima M, Oshima H, Kitagawa K, Kobayashi M, Itakura C, Taketo M. Loss of Apc heterozygosity and abnormal tissue building in nascent intestinal polyps in mice carrying a truncated Apc gene. Proc Natl Acad Sci U S A 1995; 92:4482-6; PMID:7753829; http://dx.doi.org/10.1073/pnas.92.10.4482
- Smits R, Kartheuser A, Jagmohan-Changur S, Leblanc V, Breukel C, de Vries A, van Kranen H, van Krieken JH, Williamson S, Edelmann W, et al. Loss of Apc and the entire chromosome 18 but absence of mutations at the Ras and Tp53 genes in intestinal tumors from Apc1638N, a mouse model for Apc-driven carcinogenesis. Carcinogenesis 1997; 18:321-7; PMID:9054624; http://dx.doi.org/10.1093/carcin/18.2.321
- Haigis KM, Caya JG, Reichelderfer M, Dove WF. Intestinal adenomas can develop with a stable karyotype and stable microsatellites. Proc Natl Acad Sci U S A 2002; 99:8927-31; PMID:12060718; http://dx.doi.org/10.1073/pnas.132275099
- Haigis KM, Dove WF. A Robertsonian translocation suppresses a somatic recombination pathway to loss of heterozygosity. Nature genetics 2003; 33:33-9; PMID:12447373; http://dx.doi.org/10.1038/ng1055
- Choate KA, Lu Y, Zhou J, Choi M, Elias PM, Farhi A, Nelson-Williams C, Crumrine D, Williams ML, Nopper AJ, et al. Mitotic recombination in patients with ichthyosis causes reversion of dominant mutations in KRT10. Science 2010; 330:94-7; PMID:20798280; http://dx.doi.org/10.1126/science.1192280
- Kiritsi D, He Y, Pasmooij AM, Onder M, Happle R, Jonkman MF, Bruckner-Tuderman L, Has C. Revertant mosaicism in a human skin fragility disorder results from slipped mispairing and mitotic recombination. J Clin Invest 2012; 122:1742-6; PMID:22466645; http://dx.doi.org/10.1172/JCI61976
- Jongmans MC, Verwiel ET, Heijdra Y, Vulliamy T, Kamping EJ, Hehir-Kwa JY, Bongers EM, Pfundt R, van Emst L, van Leeuwen FN, et al. Revertant somatic mosaicism by mitotic recombination in dyskeratosis congenita. Am J Hum Genet 2012; 90:426-33; PMID:22341970; http://dx.doi.org/10.1016/j.ajhg.2012.01.004
- Siudeja K, Nassari S, Gervais L, Skorski P, Lameiras S, Stolfa D, Zande M, Bernard V, Frio TR, Bardin AJ. Frequent somatic mutation in adult intestinal stem cells drives neoplasia and genetic mosaicism during aging. Cell Stem Cell 2015; 17:663-74; PMID:26607382; http://dx.doi.org/10.1016/j.stem.2015.09.016
- Judd SR, Petes TD. Physical lengths of meiotic and mitotic gene conversion tracts in Saccharomyces cerevisiae. Genetics 1988; 118:401-10; PMID:2835285
- Chumki SA, Dunn MK, Coates TF, Mishler JD, Younkin EM, Casper AM. Remarkably long-tract gene conversion induced by fragile site instability in Saccharomyces cerevisiae. Genetics 2016; 204:115-28; PMID:27343237
- Sweetser DB, Hough H, Whelden JF, Arbuckle M, Nickoloff JA. Fine-resolution mapping of spontaneous and double-strand break-induced gene conversion tracts in Saccharomyces cerevisiae reveals reversible mitotic conversion polarity. Mol Cell Biol 1994; 14:3863-75; PMID:8196629; http://dx.doi.org/10.1128/MCB.14.6.3863
- Lafave MC, Andersen SL, Stoffregen EP, Holsclaw JK, Kohl KP, Overton LJ, Sekelsky J. Sources and structures of mitotic crossovers that arise when BLM helicase is absent in Drosophila. Genetics 2014; 196:107-18; PMID:24172129; http://dx.doi.org/10.1534/genetics.113.158618
- St. Charles J, Petes TD. High-resolution mapping of spontaneous mitotic recombination hotspots on the 1.1 Mb arm of yeast chromosome IV. PLoS Genet 2013; 9:e1003434; PMID:23593029; http://dx.doi.org/10.1371/journal.pgen.1003434
- Hagstrom SA, Dryja TP. Mitotic recombination map of 13cen-13q14 derived from an investigation of loss of heterozygosity in retinoblastomas. Proc Natl Acad Sci USA 1999; 96; PMID:10077618; http://dx.doi.org/10.1073/pnas.96.6.2952
- Howarth K, Ranta S, Winter E, Teixeira A, Schaschl H, Harvey JJ, Rowan A, Jones A, Spain S, Clark S, et al. A mitotic recombination map proximal to the APC locus on chromosome 5q and assessment of influences on colorectal cancer risk. BMC Med Genet 2009; 10:1-11; PMID:19133158; http://dx.doi.org/10.1186/1471-2350-10-54
- Rosen DM, Younkin EM, Miller SD, Casper AM. Fragile site instability in Saccharomyces cerevisiae causes loss of heterozygosity by mitotic crossovers and break-induced replication. PLoS Genet 2013; 9:e1003817; PMID:24068975; http://dx.doi.org/10.1371/journal.pgen.1003817
- Le Beau MM, Rassool FV, Neilly ME, Espinosa R, Glover TW, Smith DI, McKeithan TW. Replication of a common fragile site, FRA3B, occurs late in S phase and is delayed further upon induction: Implications for the mechanism of fragile site induction. Hum Mol Genet 1998; 7:755-61; PMID:9499431; http://dx.doi.org/10.1093/hmg/7.4.755
- Arlt MF, Casper AM, Glover TW. Common fragile sites. Cytogenetic Genome Res 2003; 100:92-100; PMID:14526169; http://dx.doi.org/10.1159/000072843
- Hansen RS, Canfield TK, Lamb MM, Gartler SM, Laird CD. Association of fragile X syndrome with delayed replication of the FMR1 gene. Cell 1993; 73:1403-9; PMID:8324827; http://dx.doi.org/10.1016/0092-8674(93)90365-W
- Letessier A, Millot GA, Koundrioukoff S, Lachages AM, Vogt N, Hansen RS, Malfoy B, Brison O, Debatisse M. Cell-type-specific replication initiation programs set fragility of the FRA3B fragile site. Nature 2011; 470:120-3; PMID:21258320; http://dx.doi.org/10.1038/nature09745
- Brewer BJ, Fangman WL. A replication fork barrier at the 3' end of yeast ribosomal RNA genes. Cell 1988; 55:637-43; PMID:3052854; http://dx.doi.org/10.1016/0092-8674(88)90222-X
- Brewer BJ, Lockshon D, Fangman WL. The arrest of replication forks in the rDNA of yeast occurs independently of transcription. Cell 1992; 71:267-76; PMID:1423594; http://dx.doi.org/10.1016/0092-8674(92)90355-G
- Deshpande AM, Newlon CS. DNA Replication fork pause sites dependent on transcription. Science 1996; 272:1030-3; PMID:8638128; http://dx.doi.org/10.1126/science.272.5264.1030
- Azvolinsky A, Giresi PG, Lieb JD, Zakian VA. Highly transcribed RNA polymerase II genes are impediments to replication fork progression in Saccharomyces cerevisiae. Mol Cell 2009; 34:722-34; PMID:19560424; http://dx.doi.org/10.1016/j.molcel.2009.05.022
- Paeschke K, Capra JA, Zakian VA. DNA replication through G-quadruplex motifs is promoted by the Saccharomyces cerevisiae Pif1 DNA helicase. Cell 2011; 145:678-91; PMID:21620135; http://dx.doi.org/10.1016/j.cell.2011.04.015
- Holt D, Dreimanis M, Pfeiffer M, Firgaira F, Morley A, Turner D. Interindividual variation in mitotic recombination. Am J Hum Genet 1999; 65:1423-7; PMID:10521309; http://dx.doi.org/10.1086/302614
- Aguilera A, Chavez S, Malagon F. Mitotic recombination in yeast: elements controlling its incidence. Yeast 2000; 16:731-54; PMID:10861900; http://dx.doi.org/10.1002/1097-0061(20000615)16:8%3c731::AID-YEA586%3e3.0.CO;2-L
- McVey M, Andersen SL, Broze Y, Sekelsky J. Multiple functions of Drosophila BLM helicase in maintenance of genome stability. Genetics 2007; 176:1979-92; PMID:17507683; http://dx.doi.org/10.1534/genetics.106.070052
- Langlois RG, Bigbee WL, Jensen RH, German J. Evidence for increased in vivo mutation and somatic recombination in Bloom's syndrome. Proc Natl Acad Sci U S A 1989; 86:670-4; PMID:2911598; http://dx.doi.org/10.1073/pnas.86.2.670
- Kusunoki Y, Hayashi T, Hirai Y, Kushiro J, Tatsumi K, Kurihara T, Zghal M, Kamoun MR, Takebe H, Jeffreys A, et al. Increased rate of spontaneous mitotic recombination in T lymphocytes from a Bloom's syndrome patient using a flow-cytometric assay at HLA-A locus. Jpn J Cancer Res 1994; 85:610-8; PMID:8063614; http://dx.doi.org/10.1111/j.1349-7006.1994.tb02403.x
- Gould KA, Dietrich WF, Borenstein N, Lander ES, Dove WF. Mom1 is a semi-dominant modifier of intestinal adenoma size and multiplicity in Min/+ mice. Genetics 1996; 144:1769-76; PMID:8978062
- Dietrich WF, Lander ES, Smith JS, Moser AR, Gould KA, Luongo C, Borenstein N, Dove W. Genetic identification of Mom-1, a major modifier locus affecting Min-induced intestinal neoplasia in the mouse. Cell 1993; 75:631-9; PMID:8242739; http://dx.doi.org/10.1016/0092-8674(93)90484-8
- Perreault N, Sackett SD, Katz JP, Furth EE, Kaestner KH. Foxl1 is a mesenchymal Modifier of Min in carcinogenesis of stomach and colon. Genes Dev 2005; 19:311-5; PMID:15650110; http://dx.doi.org/10.1101/gad.1260605
- Crist RC, Roth JJ, Lisanti MP, Siracusa LD, Buchberg AM. Identification of Mom12 and Mom13, two novel modifier loci of Apc (Min) -mediated intestinal tumorigenesis. Cell Cycle 2011; 10:1092-9; PMID:21386660; http://dx.doi.org/10.4161/cc.10.7.15089
- Otterpohl KL, Gould KA. Genetic dissection of the Mom5 modifier locus and evaluation of Mom5 candidate genes. Mamm Genome 2015; 26:235-47; PMID:25976411; http://dx.doi.org/10.1007/s00335-015-9567-x
- Kwong LN, Shedlovsky A, Biehl BS, Clipson L, Pasch CA, Dove WF. Identification of Mom7, a novel modifier of Apc(Min/+) on mouse chromosome 18. Genetics 2007; 176:1237-44; PMID:17435219; http://dx.doi.org/10.1534/genetics.107.071217
- Metz CW. Chromosome studies on the Diptera. II. The paired association of chromosomes in the Diptera, and its significance. J Experimental Zool 1916; 21:213-79; http://dx.doi.org/10.1002/jez.1400210204
- Rong YS, Golic KG. The homologous chromosome is an effective template for the repair of mitotic DNA double-strand breaks in Drosophila. Genetics 2003; 165:1831-42; PMID:14704169
- Krueger C, King MR, Krueger F, Branco MR, Osborne CS, Niakan KK, Higgins MJ, Reik W. Pairing of homologous regions in the mouse genome is associated with transcription but not imprinting status. PLoS One 2012; 7:e38983
- Rao SS, Huntley MH, Durand NC, Stamenova EK, Bochkov ID, Robinson JT, Sanborn AL, Machol I, Omer AD, Lander ES, et al. A 3D map of the human genome at kilobase resolution reveals principles of chromatin looping. Cell 2014; 159:1665-80; PMID:25497547; http://dx.doi.org/10.1016/j.cell.2014.11.021
- Masui O, Bonnet I, Le Baccon P, Brito I, Pollex T, Murphy N, Hupé P, Barillot E, Belmont AS, Heard E. Live-cell chromosome dynamics and outcome of X chromosome pairing events during ES cell differentiation. Cell 2011; 145:447-58; PMID:21529716; http://dx.doi.org/10.1016/j.cell.2011.03.032
- Gandhi M, Evdokimova VN, K TC, Nikiforova MN, Kelly LM, Stringer JR, Bakkenist CJ, Nikiforov YE. Homologous chromosomes make contact at the sites of double-strand breaks in genes in somatic G0/G1-phase human cells. Proc Natl Acad Sci U S A 2012; 109:9454-9; PMID:22645362; http://dx.doi.org/10.1073/pnas.1205759109
- Hogan MS, Parfitt DE, Zepeda-Mendoza CJ, Shen MM, Spector DL. Transient pairing of homologous Oct4 alleles accompanies the onset of embryonic stem cell differentiation. Cell Stem Cell 2015; 16:275-88; PMID:25748933; http://dx.doi.org/10.1016/j.stem.2015.02.001
- Xu H, Yan Y, Deb S, Rangasamy D, Germann M, Malaterre J, Eder NC, Ward RL, Hawkins NJ, Tothill RW, et al. Cohesin Rad21 mediates loss of heterozygosity and is upregulated via Wnt promoting transcriptional dysregulation in gastrointestinal tumors. Cell Rep 2014; 9:1781-97; PMID:25464844; http://dx.doi.org/10.1016/j.celrep.2014.10.059
- Huang J, Brito IL, Villén J, Gygi SP, Amon A, Moazed D. Inhibition of homologous recombination by a cohesin-associated clamp complex recruited to the rDNA recombination enhancer. Genes Dev 2006; 20:2887-901; PMID:17043313; http://dx.doi.org/10.1101/gad.1472706
- Graf U, Wurgler FE, Katz AJ, Frei H, Juon H, Hall CB, Kale PG. Somatic mutation and recombination test in Drosophila melanogaster. Environ Mutagen 1984; 6:153-88; PMID:6423380; http://dx.doi.org/10.1002/em.2860060206
- DePinho RA. The age of cancer. Nature 2000; 408:248-54; PMID:11089982; http://dx.doi.org/10.1038/35041694
- McMurray MA, Gottschling DE. An age-induced switch to a hyper-recombinational state. Science 2003; 301:1908-11; PMID:14512629; http://dx.doi.org/10.1126/science.1087706
- Lindstrom DL, Leverich CK, Henderson KA, Gottschling DE. Replicative age induces mitotic recombination in the ribosomal RNA gene cluster of Saccharomyces cerevisiae. PLoS Genetics 2011; 7:e1002015; PMID:21436897; http://dx.doi.org/10.1371/journal.pgen.1002015
- Grist SA, McCarron M, Kutlaca A, Turner DR, Morley AA. In vivo human somatic mutation: frequency and spectrum with age. Mutat Res 1992; 266:189-96; PMID:1373828; http://dx.doi.org/10.1016/0027-5107(92)90186-6