ABSTRACT
Maladaptive changes in the intestinal flora, typically referred to as bacterial dysbiosis, have been linked to intestinal aging phenotypes, including an increase in intestinal stem cell (ISC) proliferation, activation of inflammatory pathways, and increased intestinal permeability1,2. However, the causal relationships between these phenotypes are only beginning to be unravelled. We recently characterized the age-related changes that occur to septate junctions (SJ) between adjacent, absorptive enterocytes (EC) in the fly intestine. Changes could be observed in the overall level of SJ proteins, as well as the localization of a subset of SJ proteins. Such age-related changes were particularly noticeable at tricellular junctions (TCJ)3. Acute loss of the Drosophila TCJ protein Gliotactin (Gli) in ECs led to rapid activation of stress signalling in stem cells and an increase in ISC proliferation, even under axenic conditions; a gradual disruption of the intestinal barrier was also observed. The uncoupling of changes in bacteria from alterations in ISC behaviour and loss of barrier integrity has allowed us to begin to explore the interrelationship of these intestinal aging phenotypes in more detail and has shed light on the importance of the proteins that contribute to maintenance of the intestinal barrier.
Introduction
Much progress has been made in the last 30 years in understanding the cellular and molecular underpinnings of the aging process [Citation1]. Indeed, recent studies have indicated that aging of many organs is associated with impaired regenerative capacity due to altered activity of tissue-specific stem cells or the surrounding microenvironment, known as the niche [Citation2]. Consequently, the field is intent on understanding how aging impacts adult stem cell function.
Although not often a primary cause of death, gastrointestinal disorders affect 35–40% of elderly people, including chronic inflammatory diseases, colorectal cancer, metabolic imbalance, and gastrointestinal infections [Citation3–7]. Yet, the precise mechanisms leading to increased intestinal dysfunction and loss of intestinal homeostasis during aging are not well understood. Maintenance of the mammalian intestine throughout life relies on rapidly dividing intestinal stem cells (ISCs), marked by the Wnt target gene Lgr5.[Citation8] Although ISCs in the large intestine have been characterized as the cell-of-origin for colon cancer [Citation9], the role of ISCs in other intestinal diseases and the effects of increased inflammation, bacterial dysbiosis, and changes in metabolism on ISC behavior have only recently begun to be eludicated.
The Drosophila intestine shows remarkable similarity, on a cellular and molecular level, to the human intestine, including the regulation of stem cell activity via highly conserved pathways, such as the Wnt pathways [Citation10]. Furthermore, maintenance of a functional intestinal epithelium has been shown to be an important determinant of health and viability at the organismal level [Citation11–13] , making it a valuable model for investigating the impact of ISC behavior on intestinal function, longevity and healthspan. The Drosophila midgut epithelium is composed of ISCs, enteroblasts (EB), secretory enteroendocrine (EE) cells and ECs (). ISCs self‐renew to maintain the stem cell pool and give rise to EBs that will differentiate into either an EE or an EC [Citation14,Citation15]; recent data have suggested that a subset of ISCs may be primed to differentiate into EE cells directly [Citation16,Citation17]. Intestines dissected from older flies exhibit an increase in ISC proliferation, accumulation of cells that express hallmarks of both ISCs/EBs and ECs (mis-differentiated cells), an increase in bacterial load, a change in commensal populations, activation of inflammatory pathways, increases in reactive oxygen species (ROS), and altered intestinal permeability [Citation10,Citation11,Citation13,Citation18–21].
Figure 1. The Drosophila melanogaster posterior midgut epithelium. (a) Confocal immunostaining image of a posterior midgut showing the 4 cell types and their lineage relationships. ISC is pseudocolored in green, EB is pseudocolored in purple, EEs are stained in red by Prospero (nuclear), and ECs are identified based on their polyploid nuclei (stained by DAPI, in blue). (b, b’) Vertical section through the midgut epithelium. Mesh (red), a sSJ protein, is located apically in ECs (polyploid nuclei stained in blue). (c) Apical localization of the septate junction protein Scribble (green) compared to the adherens junction protein Armadillo (Arm, red). Arrowheads depict the enrichment of Scribble at the TCJs. (d) Confocal image of posterior midgut showing the localization of Gli-GFP (green) at the TCJs. Gli is mis-localized from TCJs (arrowheads) in posterior midguts from 50do flies comparing to 5do.
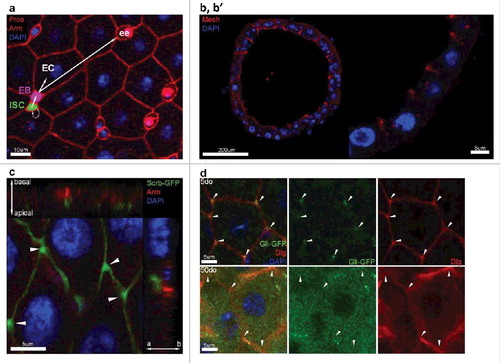
The intestinal epithelium provides an essential, selective barrier that permits passage of water, ions and nutrients, while limiting contact with harmful environmental toxins and preventing microbial contamination of interstitial tissues. Importantly, the intestinal epithelium also serves essential metabolic and innate immune functions. To undertake its barrier role, intestinal epithelial cells have specialized intercellular junctions that restrict paracellular flux. In flies, that function is carried out by septate junctions (SJ)(), which are functionally analogous to tight junctions (TJ) in chordates [Citation22].
In Drosophila, SJs are divided in two classes based on their morphological appearance: pleated SJs (pSJ) are found in ectodermally-derived tissues such as the hindgut, while smooth SJs (sSJ) are observed in endodermally-derived tissues such as the midgut [Citation22,Citation23]. There are differences between SJs and TJs, with respect to organization, protein composition, and localization; in contrast to TJs in vertebrates, most SJs are basally located with respect to adherens junctions (AJ) [Citation22,Citation24]. However, sSJs in the midgut are apically located relative to AJs () [Citation25], offering a unique model to investigate mechanisms underlying alterations in intestinal permeability that may be linked to loss of barrier function in mammalian tissues.
One of the most striking and consistent age-related changes in SJ protein localization was observed at the tricellular junction (TCJ), specialized SJs where three cells meet that control the passage of macromolecules across the intestinal epithelium [Citation26,Citation27] (, , arrowheads). In Drosophila, Gliotactin (Gli), and more recently Anakonda (Aka)/Bark beetle (Bark), are the only proteins exclusively found at the TCJ [Citation28–30]. Importantly, depletion of Gli or Aka in epithelial tissues leads to loss of the permeability barrier [Citation31,Citation28,Citation29].
Our previous study provided a direct link between modulation of SJs and changes in ISC behavior, mediated through the conserved Jun N-terminal kinase (JNK) stress signaling pathway. These changes occurred rapidly, were reversible, and were indistinguishable under axenic conditions. Importantly, these changes occurred prior to detectable loss of barrier function, indicating that modulation of cellular junctions between differentiated cells can play a proximal, non-autonomous role in regulating ISC behavior. Here we provide additional evidence that bacteria are not involved in the increases in ISC proliferation that occur downstream of modulating TCJs, in contrast to the cascade of events typically reported to trigger changes in ISC behavior as a consequence of infection, damage or aging. In addition, we speculate on potential differences between the role of TCJ and bicellular junctions in eliciting changes in stem cell behavior.
Changes to tight junctions occur normally in axenic flies
Previous work in aged, wild-type flies indicated that stereotypical alterations in the bacterial flora precede and predict the beginning of intestinal barrier dysfunction, leading to systemic immune activation and organismal death [Citation32]. In order to better comprehend the relationships between intestinal microbiota, intestinal barrier dysfunction, and SJ proteins, we generated axenic flies and assessed the levels and localization of SJ proteins. Consistent with Clark et al [Citation32], we found Gli:GFP axenic flies displayed an increase in lifespan when compared to control flies (). Interestingly, the age-related changes in TCJ proteins in axenic flies were indistinguishable from control flies at early time points (). This is in contrast to the delay in age-related changes to junctions observed in long-lived dietary restricted flies [Citation31].
Figure 2. (a) Lifespan curves for Gli:GFP (DGRC 115332) female flies (raised on under axenic conditions (red) or control flies rasied under axenic conditions and then refed homogenized flies to reconstitute the intestinal microbiome (grey). T50 and total lifespan were significantly lower in controls, when compared with axenic. Data were analysed with a non-parametric log-rank (Mantel–Cox) test; ∗∗∗∗, P < 0.0001, represents a statistically significant difference. (b) MRS plates showing bacterial presence from cultured fly homogenates in non-axenic flies and total absence of bacterial colonies from axenic flies at 10do and 60do. TCJ/Cytoplasm fluorescence ratio for Gli in axenic flies shows no statistical differences until later time point d60 compared to control flies. Asterisks represent a statistically significant difference in an unpaired Student's t-test, two tailed, indicated by the corresponding bars (* = P<0,01). 10do Ctrl n = 21 midguts; 10do Axenic n = 20; 20do Ctrl n = 20; 20do Axenic n = 21; 30do Ctrl n = 20; 30do Axenic n = 20; 40do Ctrl n = 16; 40do Axenic n = 16; 50do Ctrl n = 20; 50do Axenic n = 20; 60do Ctrl n = 20; 60do Axenic n = 20. Each data point (n = midguts) represents an average fluorescence intensity ratio from 2–7 independent measurements per midgut and the error bars are the S.E.M range of those averages. (c-f) Alterations in microbiota composition are not linked to loss of TCJ. Targeted Gli is depleted from ECs using the 5966GS GAL4 ‘driver’, when flies are fed with the progesterone analogue RU486(RU+); addition of ethanol is used as a control (RU-). Bacterial levels assayed by qPCR at different time points, of the 16S ribosomal RNA gene in dissected intestines. With universal (c), Alphaproteobacteria (d), Bacilli (e) and Gammaproteobacteria (f) primers. No significant differences were detected in bacterial expression, after 2, 9, 16, 23, 30, 37 days post Gli-RNAi expression (RU+) compared to control (RU-). Only at day 44, significant changes were scored. Boxplots display the first and third quartile, with horizontal bar at the median and whiskers showing the most extreme data point, which is no more than 1.5 times the interquartile range from the box.∗∗ = P < 0.01, represent statistically significant difference.
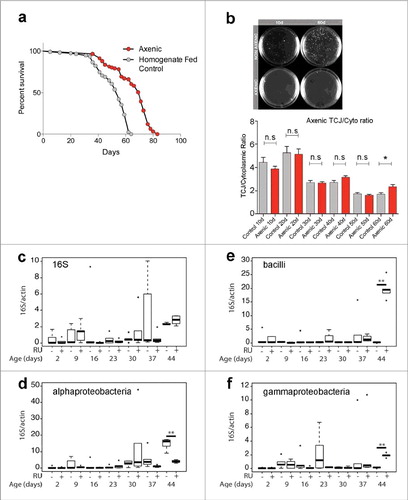
Disruption of TCJ do not trigger bacterial dysbiosis
Although flies raised under axenic conditions did not demonstrate delayed changes in TCJ proteins, we wanted to determine whether depletion of Gli would lead to an acceleration in the shift in bacterial composition that normally occurs with age and that has been correlated with loss of intestinal barrier function. Acute reduction of Gli expression, upon expression of UAS-GliRNAi in intestinal ECs using the GeneSwitch driver 5966-Gal4GS, induced a significant loss of intestinal barrier function by 12 days and ultimately decreased the median lifespan of flies [Citation31]. Employing universal primers to the bacterial 16S rRNA, qPCR was used to characterize possible variations in bacterial dynamics upon Gli depletion from ECs. Surprisingly, no consistent changes were observed after induction of GliRNAi expression during early and mid-life, the time by which loss of barrier integrity and intestinal dysplasia are clearly observed (, and [Citation31]).
Clark et al [Citation32], meticulously described the behaviour of several bacterial classes pre- and post- intestinal barrier failure. Their analysis showed that expansion of the Gammaproteobacteria begins prior to intestinal barrier failure, while Bacilli and Alphaproteobacteria classes expand after the intestinal barrier has been lost [Citation32].. In order to determine whether similar shifts in bacterial classes are observed upon Gli depletion, we performed a comprehensive analysis using primers for the 16S rRNA gene specific for three bacterial classes [Citation32]. Our results, again, indicated that there were no bacterial changes detected prior to day 44 (). After 44 days, Bacilli and Gammaproteobacteria increase significantly in flies in which Gli was depleted from ECs (RU+), when compared to controls (RU-) (); Alphaproteobacteria behaved in the opposite manner ().
Consistent with the qPCR analysis, which indicated no premature increase in bacterial dysbiosis upon disruption of TCJs, no consistent increase in expression of antimicrobial peptides (AMPs) was detected 21 days after induction of GliRNAi expression [Citation31]. In summary, only after 44 days, when >90% of the flies displayed a loss in barrier integrity [Citation31], a trend toward bacterial dysbiosis started to emerge in both control and experimental flies ().
Altogether, our data indicate that changes in bacteria do not play a major role in mediating the changes we have observed to TCJ, nor are responsible for the rapid changes in ISC behavior upon Gli depletion.
Concluding remarks
Our data suggests that altered TCJ function during the course of normal aging could contribute directly to the changes in ISC behavior [Citation31] observed in older animals. In addition, changes to the localization and levels of SJ proteins appear to occur prior to loss of barrier function using established assays [Citation31,Citation12,Citation13,Citation32]. Furthermore, changes to SJ can occur prior to detectable bacterial dysbiosis and increased expression of AMPs [Citation31].
At first blush, our findings are seemingly contradictory to previous findings that describe a distinct shift in bacterial populations [Citation13,Citation32] occurring before or concurrently with loss of the intestinal barrier. However, TCJs contribute to only a small percentage of the midgut lumen barrier, when compared to the bicellular junctions. Although some mammalian intestinal pathogens target the TCJ to facilitate cell-to-cell spreading [Citation33,Citation34], it may be that SJ in the midgut are a more potent barrier for most bacteria in flies. In addition, disruption of TCJs does not completely disrupt the bicellular SJs [Citation31]. However, depletion of Dlg from ECs, which also induces an increase in ISC proliferation, led to a complete loss of Gli from TCJs [Citation31]. These results indicate that depletion of Gli from the TCJ, rather than mis-localization of Dlg and complete disruption of SJs, is sufficient to trigger loss of barrier integrity. Due to the impossibility of uncoupling the effects of Dlg from Gli, as well as the disparity in the SJ composition along the anterior-posterior axis of the Drosophila gut [Citation31], a comprehensive study of different SJ/TCJ components should be addressed. We predict that depletion of bicellular SJ components, such as discs large or coracle, and midgut specific sSJ proteins mesh, snakeskin, big bang, or Tetraspanin2A may have a much more potent effect on bacterial dysbiosis and loss of barrier function.
Beyond their role in forming the epithelial barrier and regulating paracellular flux, it is hypothesized that mammalian TJs may also influence epithelial cell differentiation, cell proliferation or tumorigenesis [Citation35–39]. Given that intercellular junctions can function as ‘landmarks’, confining signaling molecules and polarity cues and providing docking sites for vesicles, changes in the integrity or localization of junctional complexes could have a major impact on stem cell behavior that maintain polarized epithelia [Citation40]. Therefore, we hypothesize that the Gli, specifically, and the TCJ, more generally, are playing a major role in regulating signalling throughout the midgut intestinal epithelium. Although there were no apparent age-related changes to SJ or TCJ in the hindgut epithelium with age [Citation31], both could be important for regulating the intestinal barrier and signalling in this tissue, as well. Further investigation into the interplay between TCJs and SJs, with respect to barrier function, bacterial dysbiosis, and intracellular signaling will be crucial to understanding the initiation and advancement of processes that contribute to intestinal diseases and the disruption of normal intestinal function over time.
Disclosure of potential conflicts of interest
No potential conflicts of interest were disclosed.
Acknowledgments
The authors thank the Jones and Walker laboratories for comments on the manuscript.
Additional information
Funding
References
- Lopez-Otin C, Blasco MA, Partridge L, et al. The hallmarks of aging. Cell. 2013;153:1194–1217. doi:10.1016/j.cell.2013.05.039
- Jones DL, Rando TA. Emerging models and paradigms for stem cell ageing. Nat Cell Biol. 2011;13:506–512. doi:10.1038/ncb0511-506
- Man AL, et al. Age-associated modifications of intestinal permeability and innate immunity in human small intestine. Clin Sci (Lond). 2015;129:515–527. doi:10.1042/CS20150046
- Hall KE, Proctor DD, Fisher L, et al. American gastroenterological association future trends committee report: effects of aging of the population on gastroenterology practice, education, and research. Gastroenterology. 2005;129:1305–1338. doi:10.1053/j.gastro.2005.06.013
- Patel BB, Yu Y, Du J, et al. Age-related increase in colorectal cancer stem cells in macroscopically normal mucosa of patients with adenomas: a risk factor for colon cancer. Biochem Biophys Res Commun. 2009;378:344–347. doi:10.1016/j.bbrc.2008.10.179
- Sipos F, Leiszter K, Tulassay Z. Effect of ageing on colonic mucosal regeneration. World J Gastroenterol. 2011;17:2981–2986. doi:10.3748/wjg.v17.i25.2981
- Clemente JC, Ursell LK, Parfrey LW, et al. The impact of the gut microbiota on human health: an integrative view. Cell. 2012;148:1258–1270. doi:10.1016/j.cell.2012.01.035
- Barker N, van Es JH, Kuipers J, et al. Identification of stem cells in small intestine and colon by marker gene Lgr5. Nature. 2007;449:1003–1007. doi:10.1038/nature06196
- Barker N, Ridgway RA, van Es JH, et al. Crypt stem cells as the cells-of-origin of intestinal cancer. Nature. 2009;457:608–611. doi:10.1038/nature07602
- Li H, Jasper H. Gastrointestinal stem cells in health and disease: from flies to humans. Dis Model Mech. 2016;9:487–499. doi:10.1242/dmm.024232
- Biteau B, Karpac J, Supoyo S, et al. Lifespan extension by preserving proliferative homeostasis in Drosophila. PLoS Genet. 2010;6:e1001159. doi:10.1371/journal.pgen.1001159
- Rera M, Bahadorani S, Cho J, et al. Modulation of longevity and tissue homeostasis by the Drosophila PGC-1 homolog. Cell Metab. 2011;14:623–634. doi:10.1016/j.cmet.2011.09.013
- Rera M, Clark RI, Walker DW. Intestinal barrier dysfunction links metabolic and inflammatory markers of aging to death in Drosophila. Proc Natl Acad Sci U S A. 2012;109:21528–21533. doi:10.1073/pnas.1215849110
- Micchelli CA, Perrimon N. Evidence that stem cells reside in the adult Drosophila midgut epithelium. Nature. 2006;439:475–479. doi:10.1038/nature04371
- Ohlstein B, Spradling A. The adult Drosophila posterior midgut is maintained by pluripotent stem cells. Nature. 2006;439:470–474. doi:10.1038/nature04333
- Biteau B, Jasper H. Slit/Robo signaling regulates cell fate decisions in the intestinal stem cell lineage of Drosophila. Cell Rep. 2014;7:1867–1875. doi:10.1016/j.celrep.2014.05.024
- Zeng X, Hou SX. Enteroendocrine cells are generated from stem cells through a distinct progenitor in the adult Drosophila posterior midgut. Development. 2015;142:644–653. doi:10.1242/dev.113357
- Choi YJ, Hwang MS, Park JS, et al. Age-related upregulation of Drosophila caudal gene via NF-kappaB in the adult posterior midgut. Biochim Biophys Acta. 2008;1780:1093–1100. doi:10.1016/j.bbagen.2008.06.008
- Biteau B, Hochmuth CE, Jasper H. JNK activity in somatic stem cells causes loss of tissue homeostasis in the aging Drosophila gut. Cell Stem Cell. 2008;3:442–455. doi:10.1016/j.stem.2008.07.024
- Guo L, Karpac J, Tran SL, et al. PGRP-SC2 promotes gut immune homeostasis to limit commensal dysbiosis and extend lifespan. Cell. 2014;156:109–122. doi:10.1016/j.cell.2013.12.018
- Chen H, Zheng X, Zheng Y. Age-associated loss of lamin-B leads to systemic inflammation and gut hyperplasia. Cell. 2014;159:829–843. doi:10.1016/j.cell.2014.10.028
- Izumi Y, Furuse M. Molecular organization and function of invertebrate occluding junctions. Semin Cell Dev Biol. 2014;36:186–193. doi:10.1016/j.semcdb.2014.09.009
- Tepass U, Hartenstein V. The development of cellular junctions in the Drosophila embryo. Dev Biol. 1994;161:563–596. doi:10.1006/dbio.1994.1054
- Tepass U, Tanentzapf G, Ward R, et al. Epithelial cell polarity and cell junctions in Drosophila. Annu Rev Genet. 2001;35:747–784. doi:10.1146/annurev.genet.35.102401.091415
- Baumann O. Posterior midgut epithelial cells differ in their organization of the membrane skeleton from other drosophila epithelia. Exp Cell Res. 2001;270:176–187. doi:10.1006/excr.2001.5343
- Fristrom DK. Septate junctions in imaginal disks of Drosophila: a model for the redistribution of septa during cell rearrangement. J Cell Biol. 1982;94:77–87.
- Noirot-Timothee C, Graf F, Noirot C. The specialization of septate junctions in regions of tricellular junctions. II. Pleated septate junctions. J Ultrastruct Res. 1982;78:152–165.
- Schulte J, Tepass U, Auld VJ. Gliotactin. a novel marker of tricellular junctions, is necessary for septate junction development in Drosophila. J Cell Biol. 2003;161:991–1000. doi:10.1083/jcb.200303192
- Byri S, Misra T, Syed ZA, et al. The Triple-Repeat Protein Anakonda Controls Epithelial Tricellular Junction Formation in Drosophila. Dev Cell. 2015;33:535–548. doi:10.1016/j.devcel.2015.03.023
- Hildebrandt A, Pflanz R, Behr M, et al. Bark beetle controls epithelial morphogenesis by septate junction maturation in Drosophila. Dev Biol. 2015;400:237–247. doi:10.1016/j.ydbio.2015.02.008
- Resnik-Docampo M, Koehler CL, Clark RI, et al. Tricellular junctions regulate intestinal stem cell behaviour to maintain homeostasis. Nat Cell Biol. 2017;19:52–59. doi:10.1038/ncb3454
- Clark RI, Salazar A, Yamada R, et al. Distinct Shifts in Microbiota Composition during Drosophila Aging Impair Intestinal Function and Drive Mortality. Cell Rep. 2015;12:1656–1667. doi:10.1016/j.celrep.2015.08.004
- Fukumatsu M, Ogawa M, Arakawa S, et al. Shigella targets epithelial tricellular junctions and uses a noncanonical clathrin-dependent endocytic pathway to spread between cells. Cell Host Microbe. 2012;11:325–336. doi:10.1016/j.chom.2012.03.001
- Fukumatsu M, Ogawa M, Kim M, et al. Uptake of Shigella-containing pseudopodia by neighboring epithelial cells at tricellular junctions via non-canonical clathrin-dependent trafficking pathway. Virulence. 2012;3:515–518. doi:10.4161/viru.21740
- Tsukita S, Yamazaki Y, Katsuno T, et al. Tight junction-based epithelial microenvironment and cell proliferation. Oncogene. 2008;27:6930–6938. doi:10.1038/onc.2008.344
- Ichikawa-Tomikawa N, Sugimoto K, Satohisa S, et al. Possible involvement of tight junctions, extracellular matrix and nuclear receptors in epithelial differentiation. J Biomed Biotechnol. 2011;2011:253048. doi:10.1155/2011/253048
- Mariano C, Sasaki H, Brites D, et al. A look at tricellulin and its role in tight junction formation and maintenance. Eur J Cell Biol. 2011;90:787–796. doi:10.1016/j.ejcb.2011.06.005
- Balda MS, Matter K. Tight junctions as regulators of tissue remodelling. Curr Opin Cell Biol. 2016;42:94–101. doi:10.1016/j.ceb.2016.05.006
- Landy J, Ronde E, English N, et al. Tight junctions in inflammatory bowel diseases and inflammatory bowel disease associated colorectal cancer. World J Gastroenterol. 2016;22:3117–3126. doi:10.3748/wjg.v22.i11.3117
- Martin-Belmonte F, Perez-Moreno M. Epithelial cell polarity, stem cells and cancer. Nat Rev Cancer. 2011;12:23–38. doi:10.1038/nrc3169