ABSTRACT
Sperm quality, an important male fitness trait, is commonly compared between studies. However, few studies consider how genetic and environmental variation affects sperm quality, even in the genetic model Drosophila melanogaster. Here we show that sperm viability, the proportion of live sperm, differed across the genotypes Oregon-R, Dahomey, and Canton-S by more than 15%, and across buffers (phosphate-buffered saline (PBS), Grace’s Medium and Drosophila Ringer solution) by more than 20%. In terms of genotype-buffer pair comparisons, nearly half of the comparisons would produce significant differences in sperm viability (15 in 36), or its temporal decrease in a stress medium (19 in 36). Grace’s medium produced the longest-lived sperm in vitro and the smallest differences between genotypes, Drosophila Ringer Solution produced the shortest lifespan and the largest differences. Our results suggest that fly and other sperm researchers would benefit from a standardized protocol of measuring sperm viability.
Introduction
Sperm function, essential to male fertility, is influenced by both genetic and environmental factors and can be phenotypically plastic [Citation1,Citation2]. Sperm function is therefore expected to differ across laboratory settings and genotypes. For humans, globally standardized protocols [Citation3] exist to reduce the methodological component but for animals used in research, such standardization seems less common. This can be problematic if the methodological variability is in the same order of magnitude as the biological variability, such as the genetic or environmental variation of male fertility in animals. For example, biological models that predict how sperm competition produces evolutionary change [Citation4–6] rest on genotypic differences between males in sperm quality. Such between-male genotypic differences in sperm quality exist but often are relatively small [e.g., Citation1, Citation7–9]. Detecting such subtle genetic differences in sperm quality would require highly standardized methodology. Environmental effects (or lifestyle effects in the medical literature), such as temperature, microbes, osmolarity and oxidants were found in hundreds of studies to influence sperm function, most often by phenotypic plasticity [reviewed Citation2]. Moreover, a number of studies report genotype-by-environment interaction effects on sperm quality [Citation10, Citation11, reviewed in Citation2], and without methodological agreement between studies, the interpretation of such complex interaction effects on sperm quality seems difficult.
A commonly used aspect of sperm function is sperm viability, the proportion of live sperm in a sample, especially since the commercial fluorescent live/dead kit has become available. One concern [Citation12] with that kit was that the proportion of live sperm at sample collection may not necessarily reflect the performance of those sperm that have survived and so may not a priori be seen as a predictor of sperm function. Instead, the resistance of sperm cells against osmotic and other stress (sperm stress resistance), measured as the change of sperm viability over time, is expected to provide a measure of the elasticity of the cell membrane [Citation12].
Among the practical problems of sperm viability measurement is that different labs and different research fields prefer different types of sperm diluents, and so even for the same species, different values exist and prevent direct comparison [see e.g., Citation12, Citation13]. For example, at least three different buffers, Drosophila Ringer’s solution, HEPES-buffered saline and Grace’s Medium, have been used for sperm viability measurement in Drosophila melanogaster [Citation12,Citation14,Citation15], and for honeybees Apis mellifera even more [Citation16–19]. Because buffers vary in their chemical composition, they can be expected to have different impacts on sperm. Quantitative comparisons of sperm quality across studies will, therefore, be limited, even for the same genotype. Moreover, given that plasticity and genotype-by-environment interaction effects on sperm function are likely, genotypes may differ in sperm viability across buffers. In these cases, even qualitative comparisons between studies would be limited because some genotypes might behave differently in different buffers. To the best of our knowledge, no comparison seems to exist for any genetic model systems how different genotypes respond to different sperm buffers.
Here, we test the impact of using different buffers on sperm viability in the three most commonly used wildtype D. melanogaster genotypes, Oregon-R, Dahomey and Canton-S. We examine sperm viability and sperm stress resistance in three buffers that are commonly used in Drosophila reproductive, evolutionary and developmental biology: phosphate-buffered saline (PBS), Grace’s Medium and Drosophila Ringer solution. Our results allow us to characterize the comparability of studies and to provide some recommendations for future research.
Material and methods
Fly genotypes and husbandry
Throughout we used sperm of virgin males. We used three Drosophila wildtype genotypes. Dahomey was originally collected from Benin [e.g., Citation20] and since cultured in the lab, Oregon R and Canton S are long established lab populations widely shared between Drosophila laboratories. These genotypes have been cultured in our laboratory for three years on standard corn-yeast-sugar medium (corn 90 g/l, yeast 40 g/l, sugar 7164 100 g/l, agar 12 g/l, Nipagin 20 ml/l, propionic acid 3 ml/l). For this experiment, cultures were maintained in such a way that, at dissection, males were 10 or 11 d old. Densities were not fully controlled but exactly 20 females were placed into vials with fresh medium to lay eggs. The females were removed after 10 hours and the vials incubated in an incubator at 25°C and 65% RH on a 12:12 h L:D cycle. Two weeks later, virgins were collected every 6 hours and placed in new vials in a group of 20 individuals. For sorting and transfer, CO2 anaesthesia (fly pads) was used. The virgins were maintained in the incubator before and during the experiment.
Dissection, sperm viability and sperm stress resistance
We used three types of buffers: Grace’s Medium, phosphate-buffered saline (PBS) and Drosophila Ringer solution. Their detailed composition and origin can be found in Table S1. Males of every genotype and every buffer were tested in random order. This was done by drawing individuals from numbered vials and the numbers were obtained from a random-numbers generator. Sample sizes ranged from 12 to 16 for each genotype-buffer combination (Table S2). In total, 118 individuals were individually tested.
Males were anaesthetised with CO2, and a seminal vesicle was dissected into 10 µl of specific buffer. The seminal vesicle was then transferred to another drop of 10 µl of the same buffer on the same slide. The sperm were extracted by puncturing the seminal vesicle with an insect pin and then pulled out carefully. They were immediately stained with 0.5 µl of 2 µM SYBR 14 (1:50 in DMSO) and 1 µl of 2.4 mM propidium iodide (live/dead sperm viability kit, ThermoFisher Scientific). A 22 × 22 mm coverslip was placed on the sample before taking images on an epifluorescence microscope (Leica DMi8, Germany). One area of the sample was haphazardly chosen, containing 45.51 ± SD 26.13 visible sperm (range 9–131). Four images of the same area were taken immediately (t0), and 5 (t5), 15 (t15) and 30 (t30) minutes after staining [Citation12]. The data for t0 correspond to sperm viability, the decline over time corresponds to sperm stress resistance. The excitation light was switched off between each time point. The number of live and dead sperm was manually counted with ImageJ V. 1.34 software. Sperm with green heads were counted as live, those with red heads or partially red heads as dead. All sperm measurements were done by one person (ALH) whereby images were labelled by vial number only. The counting person was, therefore, blind to treatment.
Statistical analysis
All data analysis was performed in R, version 3.3.3 [Citation21]. Sperm viability and sperm stress resistance data were fitted into a GLMM (generalized linear mixed model) with binomial error distribution using the lme4 package [Citation22]. Buffer, Drosophila genotype and its interaction were treated as fixed factors and male ID as random factor. Image ID was added as another random factor in order to achieve correction for overdispersion [Citation23]. For sperm viability, t0 data were used, for sperm stress resistance, all data (t0, t5, t15, t30). Here, time and its interactions between buffer and genotype were treated as fixed factors and male ID as random factor. The goodness of fit of all models was examined visually using normal Q-Q plot and the histogram of model residuals. Step-wise model reduction was performed backwards to get the best-fitting model. Significance of terms in the models was examined with type III ANOVA implemented in the car package [Citation24]. The fitted estimates and the standard errors predicted from the model were obtained with the effects package [Citation25].
If three buffers and three genotypes are compared the results might show no statistically significant buffer x genotype effects because the intermediate values may overlap with either extremes. When two laboratories compare studies, it seems more likely that only two buffer types or two genotypes are used. We mimicked such an effect by comparing how many such genotype-buffer comparisons would have turned out statistically significant. We employed the emmeans package for means comparisons [Citation39]. We did not correct for multiple comparisons because comparisons between two studies would be individual rather than table-wide.
Results
Sperm viability differed significantly across Drosophila genotypes. Oregon-R had the highest sperm viability (mean 88.5% across the three buffers), Canton-S the lowest (pooled mean of 72.2% across buffers) (, ). Buffer had a significant effect on sperm viability as well. Sperm viability was the highest in Grace’s Medium, regardless of genotype, followed by PBS (, ). Across all three buffer-genotype combinations, we found no genotype x buffer interaction effect on sperm viability (). However, among 36 possible pairwise buffer-genotype comparisons, 15 would have to be considered statistically significantly different (41.7%; ). Among those, genotypes did not differ when examined in Grace’s Medium, whereas the Grace’s Medium-Drosophila Ringer solution comparison would have been significant for every genotype ().
Table 1. Effects of male genotype, sperm diluent buffer and its interaction on sperm viability. Significant effects are indicated in bold. d.f., degrees of freedom
Table 2. Probabilities of statistical significance (t-tests) if sperm viability is compared across different genotype x buffer combinations (mimicking differences between studies). Values above the diagonal are for sperm viability, below the diagonal for sperm stress resistance. Comparisons between two studies would be individual rather than table-wide, and so p-values are not adjusted for multiple comparisons
Figure 1. The effect of male genotype and sperm diluent buffer on the sperm viability (proportion of live sperm) of Drosophila melanogaster wildtype. Predicted means are shown ± SE. Wildtypes are CantonS, Dahomey and OregonR, buffers are Drosophila Ringer Solution (DRS), Grace’s Medium and phosphate-buffered saline (PBS)
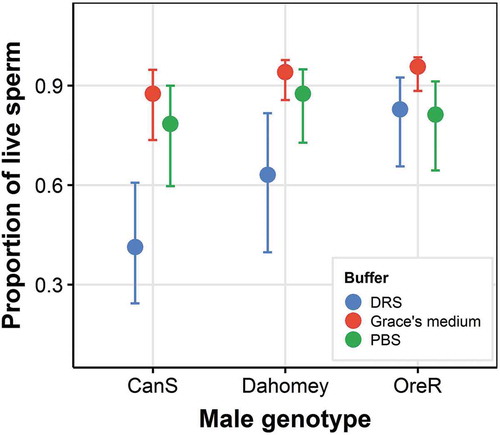
Sperm viability declined significantly over 30 minutes across all genotypes and incubation buffers (time, p < 0.001). The decline, i.e., sperm stress resistance showed no significant effect (no interaction effects with time) and no three-way interaction (buffer x genotype x time, p = 0.376) (, ). Here, 19 out of 36 pairwise buffer-genotype comparisons (averaged across levels of time) would have produced a statistically significant difference between labs (52.8%; ), among them the Grace’s Medium-DRS comparison in all three genotypes. At face value, the differences can be substantial, especially at minutes 5 and 15 where samples could easily differ by containing 50% more, or less, live sperm ().
Table 3. Effects of Drosophila genotype, sperm diluent buffer, the duration of exposure (Time; sperm stress resistance) and their interaction effects on sperm viability. Significant effects are indicated in bold. d.f., degrees of freedom
Figure 2. Buffer and time interaction effects on the change in sperm viability over time (sperm stress resistance) for three different wildtype genotypes of D. melanogaster (Canton-S, Dahomey and Oregon-R). Predicted means are shown ± SE. Buffers are Drosophila Ringer Solution, Grace’s Medium and phosphate-buffered saline (PBS)
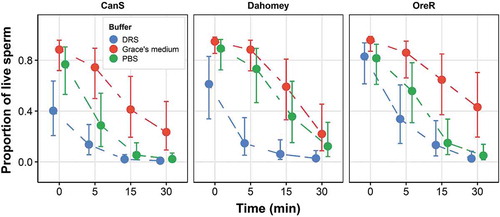
Discussion
That differences in sperm viability and sperm stress resistance exist across buffers and Drosophila wildtype genotypes is not surprising. However, the fact that such differences have not been quantified before, is, and that some of them were substantial. Among the buffers used, sperm viability was highest in Grace’s Medium, in which also the lowest variation between genotypes was observed. Hence, we can recommend Grace’s Medium for protocols that require D. melanogaster sperm to be alive as long as possible. Oregon-R males had the highest, and Canton-S males the lowest values, even in the same buffer (Drosophila Ringer solution). Thus, the simple truth that not all Drosophila wildtypes are equal extends to their sperm performance, i.e. their germline.
Neither for sperm viability nor stress resistance of sperm did we find an overall interaction effect, i.e., in principle, there was no reason to assume that genotype A behaved differently in buffer X than buffer Y. However, our analysis showed that the overall interaction effect was masked by overlapping intermediate values. Thus, we showed that a study comparing two buffers and two genotypes, rather than three, would have reported significant differences between genotype A and B in buffers X and Y in nearly half the cases. Clearly, an unadjusted application of the absolute sperm viability results from one study to the other would not have been justified. The differences were particularly large at 5 to 15 min of exposure. In addition to these methodological cautionary suggestions, our results also provide clues in terms of sperm metabolism that are briefly discussed below.
Buffer chemistry and sperm function
Grace’s Medium, the buffer that produced the highest sperm viability, contains free amino acids and carbohydrates, whereas Drosophila Ringer solution, which generated the lowest sperm viability is lacking both. Na+, K+, PO43- or Cl− ions are abundantly found in all three buffers (Table S1), although Drosophila Ringer solution and PBS, which produced a difference in sperm viability within Dahomey wildtype, did differ substantially in Na+ and K +. The main difference between Grace’s Medium and Drosophila Ringer solution (and PBS) is, therefore, related to the presence of certain sugars or certain amino acids. External carbohydrate supplementation is routinely used in extenders for sperm cryopreservation and storage in a variety of vertebrate species. It can improve sperm membrane integrity, viability, motility and metabolism [e.g., Citation26–29] even though for mostly unknown reasons. The effects of carbohydrates and amino acids on sperm viability might be caused by their potential roles in sperm metabolism. Both sugars (glycolysis) and amino acids (Arginine) have previously been invoked in contributing to Drosophila sperm metabolism [Citation30–32]. Future experiments to disentangle their effects will be useful, as will be studies that examine to what extent Grace’s Medium might mimic seminal fluid and so improve sperm quality. As the largest variation in viability was found 5 to 15 minutes after exposure of sperm to the diluent (), the clearest differences between metabolic treatments of sperm should be expected at this time point.
Genotype and sperm function
Heritable phenotypic variation (even of small amount) in sperm function is at the heart of sperm competition theory. Several genes have previously been found to affect sperm function in D. melanogaster as well as other organisms [e.g., Citation1, Citation7–9]. However, two aspects warrant consideration. First, sperm quality likely is a quantitative trait [Citation8,Citation33] and despite effects by individual genes, sperm competitiveness often has very low to no heritability [reviewed Citation34]. Second, in one case sperm were sampled from the female 4 d after mating [Citation35] and so sperm viability may additionally be affected by the female genotype. For example, in crickets, Gryllus bimaculatus, sperm metabolism measured in the male did not predict sperm metabolism in the female [Citation36].
D. melanogaster sperm has only recently been shown to produce reactive oxygen species [Citation37]. Therefore, mitochondria and oxidative phosphorylation must be involved in D. melanogaster sperm metabolism. However, it is less likely that mitochondrial variation in oxidative phosphorylation contributes to the differences observed between genotypes in this study. This is because Oregon-R flies were more similar in sperm viability and sperm stress resistance to Dahomey flies but their mitochondrial genomes are phylogenetically more closely related to the Canton-S genotype [Citation38–39].
In conclusion, comparing the performance of three buffers in sustaining the temporal change of sperm viability across three common Drosophila wildtypes allowed us to provide methodological suggestions for future analysis and may be regarded as a first step to standardize protocols of sperm metabolism across research labs. Our results allowed glimpses into aspects of sperm metabolism and these may be usefully applied to comparing sperm extracted from different female organs or from males differing in age or nutrition. More generally, our results lend support to the notion that sperm performance is environment-dependent and so may evolve under such environments [Citation2] and point out difficulties in examining genotype-by-environment interaction effects if three, not two, levels of a variable are used in a study design.
Author contributions
RG and KR conceived research
RG and ALH conducted experiments
RG and KR analysed data and conducted statistical analyses
RG, ALH and KR wrote the manuscript
All authors read and approved the manuscript
Disclosure of potential conflict of interest
No potential conflict of interest was reported by the authors.
Declaration of interests
The authors have no competing interests.
Supplemental Material
Download MS Word (23.9 KB)Acknowledgments
We thank Christin Froschauer for providing a constant supply of same-aged flies. Marko Brankatschk (BIOTEC, TU Dresden) and Bernard Moussian (University of Nizza) provided the Oregon R and Canton S flies. RG was supported by a fellowship from the Chinese Scholarship Council (CSC).
Data Availability Statement
Data are available at the permanent, open repository, http://dx.doi.10.25532/OPARA-84
Supplementary material
Supplemental data for this article can be accessed here.
Additional information
Funding
References
- Evans JP, Wilson AJ, Pilastro A, et al. Ejaculate-mediated paternal effects: evidence, mechanisms and evolutionary implications. Reproduction. 2019;157:R109–R126.
- Reinhardt K, Dobler R, Abbott J. An ecology of sperm: sperm diversification by natural selection. Annu Rev Ecol Evol Syst. 2015;46(1):435–459.
- World Health Organization. WHO laboratory manual for the examination and processing of human sperm. 5th ed. Geneva: WHO; 2010.
- Birkhead TR, Møller AP. Sperm competition. New York: Academic; 1998.
- Parker GA. Sperm competition and its evolutionary consequences in the insects. Biol Rev. 1970;45(4):525–567.
- Simmons LW. Sperm competition and its evolutionary consequences in the insects. Princeton: University Press; 2001.
- Birkhead TR, Hosken DJ, Pitnick S, eds. Sperm Biology: an Evolutionary Perspective. New York: Academic; 2009.
- Snook RR, Bacigalupe LD, Moore AJ. The quantitative genetics and coevolution of male and female reproductive traits. Evolution. 2010;64:1926–1934.
- Tourmente M, Hirose M, Ibrahim S, et al. mtDNA polymorphism and metabolic inhibition affect sperm performance in conplastic mice. Reproduction. 2017;154:341–354.
- Axelsson J, Bonde JP, Giwercman YL, et al. Gene-environment interaction and male reproductive function. Asian J Androl. 2010;12(3):298–307.
- Otti O, Johnston PR, Horsburgh GJ, et al. Female transcriptomic response to male genetic and nongenetic ejaculate variation. Behav Ecol. 2015;26(3):681–688.
- Eckel BA, Guo R, Reinhardt K. More pitfalls with sperm viability staining and a viability-based stress test to characterize sperm quality. Front Ecol Evol. 2017;5:599.
- Holman L. Sperm viability staining in ecology and evolution: potential pitfalls. Behav Ecol Sociobiol. 2009;63:1679–1688.
- Decanini DP, Wong BB, Dowling DK. Context-dependent expression of sperm quality in the fruitfly. Biol Lett. 2013;9:20130736.
- Radhakrishnan P, Fedorka KM. Influence of female age, sperm senescence and multiple mating on sperm viability in female Drosophila melanogaster. J Insect Physiol. 2011;57(6):778–783.
- den Boer SPA, Stürup M, Boomsma JJ, et al. The ejaculatory biology of leafcutter ants. J Insect Physiol. 2015;74:56–62.
- Gençer HV, Kahya Y, Woyke J. Why the viability of spermatozoa diminishes in the honeybee (Apis mellifera) within short time during natural mating and preparation for instrumental insemination. Apidologie. 2014;45(6):757–770.
- Paynter E, Baer-Imhoof B, Linden M, et al. Flow cytometry as a rapid and reliable method to quantify sperm viability in the honeybee. Apis mellifera. Cytometry. 2014;85:463–472.
- Rzymski P, Langowska A, Fliszkiewicz M, et al. Flow cytometry as an estimation tool for honey bee sperm viability. Theriogenology. 2012;77(8):1642–1647.
- Clancy DJ. Variation in mitochondrial genotype has substantial lifespan effects which may be modulated by nuclear background. Aging Cell. 2008;7(6):795–804.
- R Development Core Team. R: A language and environment for statistical computing. Vienna: Foundation for Statistical Computing; 2016.
- Bates D, Mächler M, Bolker B, et al. Fitting linear mixed-effects models using lme4. J. Stat Software. 2015; 67:1.
- Browne WJ, Subramanian SV, Jones K, et al. Variance partitioning in multilevel logistic models that exhibit overdispersion. J Royal Stat Soc Series A. 2005;168(3):599–613.
- Fox J, Weisberg S. An R companion to applied regression. 2nd ed. CA,USA: Sage: Thousand Oaks; 2011.
- Fox J. Effect displays in R for generalised linear models. J Stat Softw. 2003;8(15):1–27.
- Aboagla EME, Terada T. Trehalose-enhanced fluidity of the goat sperm membrane and its protection during freezing. Biol Reprod. 2003;69:1245–1250.
- Bucak MN, Tekin N. Protective effect of taurine, glutathione and trehalose on the liquid storage of ram semen. Small Ruminant Res. 2007;73(1–3):103–108.
- Gómez-Fernández J, Gómez-Izquierdo E, Tomás C, et al. Effect of different monosaccharides and disaccharides on boar sperm quality after cryopreservation. Anim Reprod Sci. 2012;133:109–116.
- Hu JH, Zan LS, Zhao XL, et al. Effects of trehalose supplementation on semen quality and oxidative stress variables in frozen-thawed bovine semen. J Anim Sci. 2010;88:1657–1662.
- Geer BW, Kelley KR, Pohlmann TH, et al. A comparison of rat and Drosophila spermatozoan metabolisms. Comparative Biochem Physiol Part B: Biochem Mol Biol. 1975;50: 41–50.
- Osanai M, Chen PS. A comparative study on the arginine degradation cascade for sperm maturation of Bombyx mori and Drosophila melanogaster. Amino Acids. 1993;5:341–350.
- Wetzker C, Reinhardt K. Distinct metabolic profiles in Drosophila sperm and somatic tissues revealed by two-photon NAD(P)H and FAD autofluorescence lifetime imaging. Sci Rep. 2019;9(1):19534.
- Hughes KA, Leips J. Quantitative trait locus analysis of male mating success and sperm competition in Drosophila melanogaster. Evolution. 2006;60(7):1427–1434.
- Dobler R, Reinhardt K. Heritability, evolvability, phenotypic plasticity and temporal variation in sperm-competition success of Drosophila melanogaster. J Evol Biol. 2016;29(5):929–941.
- Civetta A, Rosing KR, Fisher JH. Differences in sperm competition and sperm competition avoidance in Drosophila melanogaster. Animal Behav. 2008;75: 1739–1746.
- Ribou AC, Reinhardt K. Reduced metabolic rate and oxygen radicals production in stored insect sperm. Proc Royal Soc B Biol Series. 2012;279:2196–2203.
- Turnell BR, Reinhardt. Metabolic rate and oxygen radical levels increase but radical generation rate decreases with male age in Drosophila melanogaster sperm. J Gerontol A Biol Med Sci. 2020. DOI:https://doi.org/10.1093/gerona/glaa078
- Ilinsky Y. Coevolution of Drosophila melanogaster mtDNA and Wolbachia genotypes. PLoS ONE. 2013;8:e54373.
- Lenth R, Buerkner P, Herve M, et al. emmeans: estimated marginal means, aka least-squares means. Implemented in R. 1.5.1. 2019.