ABSTRACT
The Drosophila tracheal system consists of a widespread tubular network that provides respiratory functions for the animal. Its development, from ten pairs of placodes in the embryo to the final stereotypical branched structure in the adult, has been extensively studied by many labs as a model system for understanding tubular epithelial morphogenesis. Throughout these studies, a breathless (btl)-GAL4 driver has provided an invaluable tool to either mark tracheal cells during development or to manipulate gene expression in this tissue. A distinct shortcoming of this approach, however, is that btl-GAL4 cannot be used to specifically visualize tracheal cells in the presence of other GAL4 drivers or other UAS constructs, restricting its utility. Here we describe a direct-drive btl-nGFP reporter that can be used as a specific marker of tracheal cells throughout development in combination with any GAL4 driver and/or UAS construct. This reporter line should facilitate the use of Drosophila as a model system for studies of tracheal development and tubular morphogenesis.
Introduction
The insect tracheal system consists of a complex branching network of tubes that extends throughout the body, providing the oxygen required for cellular function. Many studies have focused on tracheal development in Drosophila as a model for organogenesis and tubular morphogenesis, with implications for the development of more complex organs such as the mammalian lung and kidneys [Citation1–4]. The tracheal system originates as ten pairs of placodes that invaginate into the embryonic body and connect to form a stereotypical branched structure. This structure refines during embryogenesis into two long dorsal tracheal trunks that run from the anterior to posterior ends of the developing larva. Regularly-spaced tubular branches extend laterally off these dorsal trunks and elaborate into a fine network of tracheal terminal cells that extend throughout the body. This tracheal system acts as the respiratory organ for the growing larva and is remodelled during metamorphosis to provide a similar function for the adult fly [Citation1].
The predominant genetic tool used to characterize tracheal development and function is a GAL4 driver from the Hayashi lab that carries regulatory sequences from the breathless (btl) locus in Drosophila [Citation5]. Btl encodes a Fibroblast Growth Factor (FGF) receptor that is expressed in the trachea and required for early tracheal development as well as the outgrowth of tracheal terminal cells in response to anoxia [Citation6–8]. The btl-GAL4 driver thus provides an ideal means of specifically targeting genetic rescue constructs or RNAi to tracheal cells. In addition, combining this driver with UAS-regulated reporter constructs provides the most commonly used method to specifically mark the tracheal system of the animal [Citation5]. This approach has been widely used in many studies of tracheal development and morphology over the past 25 years, usually using btl-GAL4 to drive a GFP reporter (btl>GFP) [e.g. Citation9–13]. A disadvantage of using this system, however, is that it is not possible to combine btl>GFP with UAS-RNAi transgenes that disrupt gene expression in other tissues since the RNAi construct will also be active in the tracheal system. A similar problem is encountered when the GAL4/UAS system is used to overexpress genes in different tissues. Accordingly, several labs have developed direct-drive fluorescent markers to follow tracheal development independently of the GAL4 system. These include btl-GFP-moe and btl-RFP-moe that carry fusions of fluorescent marker proteins to the actin binding domain of moesin [Citation14,Citation15]. This allows specific labelling of the actin cytoskeleton in developing tracheal cells and thus provides a useful marker of cellular morphology. In addition, a direct-drive SRF-GFP reporter has been constructed to specifically follow the morphology of tracheal terminal cells [Citation16]. Here we describe a fusion of the btl promoter to a nuclear GFP reporter gene (nGFP). The restricted localization of nuclear GFP often results in a stronger fluorescent signal than cytoplasmic expression, thus facilitating cellular detection. Here we describe this btl-nGFP reporter and demonstrate that it provides a strong nuclear signal in developing tracheal cells. This construct should provide a useful tool for researchers interested in studying Drosophila tracheal development and function.
Materials and methods
Construction of the btl-nGFP reporter transgene
An attB attachment site for the PhiC31 integrase system was inserted into the pH-Stinger vector described by Barolo et al. [Citation17]. This was accomplished by using the following primers to PCR amplify the attB sequence from a pUAST-attB template: ACGTCCTAGGGTCGACGATGTAGGTCACGG and GACTCCTAGGTCGACATGCCCGCCGTGACCG. This resulted in a ~ 300 bp fragment containing an attB site with AvrII restriction sites at the ends. This fragment was inserted into the single AvrII site in pH-Stinger to generate the pH-Stinger-attB vector [Citation17].
Two primers were used to PCR amplify the second intron of the btl locus from the pCas4-btl-GAL4 plasmid (a generous gift from S. Hayashi) [Citation5]: GAATTCTGCGACCTTGGCTTAAAGAC and CTCGAGTCAAATCGAGCGTTCTCCAG. This resulted in a 3.33 kb fragment with EcoRI and XhoI sites at the ends. This was inserted between the EcoRI and XhoI sites in the multiple cloning site of the pH-Stinger-attB vector to generate the btl-nGFP reporter construct. This was introduced into the Drosophila genome using the PhiC31 integrase system, targeting the construct into the attP-3B insertion site on the X chromosome (Bloomington stock number 9753; VK00038) [Citation18]. Proper integration was confirmed by PCR amplification and DNA sequencing. The stock carrying this btl-nGFP reporter can be obtained from the Bloomington stock centre (stock # 93131): y [Citation1] w[1118] PBac[y[+mDint2] w[+mC] = btl-H-Stinger]VK00038.
Microscopy and imaging
Flies carrying the btl-nGFP reporter construct were transferred to bottles with standard grape juice agar caps supplemented with yeast paste. Embryos collected from these plates were harvested, washed with water, dechorionated for 1 minute using 50% bleach in PBS, washed again with water, and mounted in glycerol on slides for imaging with a Leica SP8 laser confocal microscope.
Individual living second and third instar larvae carrying the btl-nGFP construct were serially transferred to glycerol to remove food and then transferred to a slide containing a 3% agar base. The larvae were pressed with a cover slip and tape to minimize their movement during imaging, essentially as described [Citation19]. Larvae were imaged using a Leica SP8 laser confocal microscope () or a Zeiss Axioskop2 Plus microscope (), Movie S1).
Results & discussion
Construction of a transgenic Drosophila line that carries the btl-nGFP reporter
Our original goal was to fuse the same btl regulatory sequences contained in the btl-GAL4 driver described by Shiga et al. [Citation5] with a GFP reporter gene. In their description of this construct, the btl sequences were reported as an ‘approximately 3 kb EcoRI-NdeI fragment carrying the btl promoter’ [Citation5]. The Drosophila reference genome sequence for 10 kb surrounding the btl locus, however, does not have a restriction fragment of this size. This is likely due to polymorphisms in the genomic DNA sequence of the stock used by Shiga et al. relative to the FlyBase reference genome. In addition, the btl gene is closely flanked on both sides by other protein-coding genes – the Pex1 gene, which overlaps with the 5’ end of the btl gene, and CG8100, which lies ~350 bp downstream from the 3’ end of btl (). We thus focused on the only large non-coding sequence within the btl locus, which corresponds to the second intron of the btl-A mRNA isoform (). We used PCR to amplify sequences from the middle of the second exon of btl-A to the beginning of the third exon, encompassing the entire 3.3 kb region encoding the second intron of this transcript (). Importantly, this fragment includes the ~400 bp region identified by Ohshiro and Saigo that contains important cis-regulatory elements that control btl expression in the tracheal system of Drosophila [Citation20].
Figure 1. A direct-drive btl-nGFP reporter construct. A 3.3 kb region corresponding to the second intron of the btlA mRNA isoform was inserted into the pH-Stinger-attB vector for fly transformation. This region corresponds to the only large non-coding sequences within the btl locus. The pH-Stinger-attB construct is shown below in a linearized format with the attB sequences at the ends, representing its structure upon insertion into the Drosophila genome. The two gypsy transposon insulator elements (yellow), multiple cloning site (red), minimal promoter (black arrow), GFP reporter gene (green, GFP), nuclear localization signal (blue), mini-white marker gene (white), P element ends (black), and bacterial pUC8 plasmid are shown.
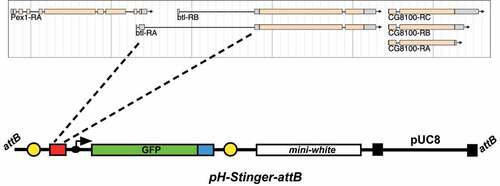
The pH-Stinger construct was described by Barolo et al. [Citation17] as a tool to facilitate studies of gene regulation in Drosophila. It consists of a bacterial plasmid with Drosophila P-element ends for fly transformation and a mini-white reporter to identify transformants (). In addition, the plasmid carries a multiple cloning site upstream from a minimal Drosophila promoter fused to a nuclear GFP reporter gene. Thus, an enhancer sequence inserted into the multiple cloning site will direct nGFP expression in a specific pattern that reflects its activity. The vector was further modified by inserting insulator elements from the gypsy transposon at either side of the GFP expression cassette. This provides a means of effectively suppressing the influence of neighbouring sequences on the expression of the GFP reporter. We modified the pH-Stinger vector by inserting a single copy of the attB targeting sequence for the PhiC31 integrase system [Citation21]. This facilitates the use of the library of attP-carrying Drosophila strains for targeting plasmid constructs to specific regions of the fly genome [Citation18]. Finally, we inserted the 3.3 kb fragment corresponding to the second intron of btl into the multiple cloning site of the pH-Stinger-attB plasmid to generate the btl-nGFP reporter. The pattern of nuclear GFP expressed by this construct should reflect the expression pattern dictated by the btl regulatory sequences. This final construct was integrated into the Drosophila genome on the X chromosome and used to characterize the pattern of nuclear GFP expression.
Characterization of the btl-nGFP expression pattern in Drosophila
The tracheal system develops from pairs of ectodermal placodes arranged in a segmented pattern along the lateral sides of the stage 10 embryo. These cells invaginate to form ten tracheal pits that expand internally to form the precursors of the branched tracheal system. During stage 13, the adjacent dorsal trunk branches in each segment fuse to form a continuous network of tracheal precursor cells that display a characteristic branching pattern along the sides of the embryo. This epithelial network continues to undergo tubular morphogenesis during the subsequent stages of embryogenesis, eventually forming the elaborate tracheal system of the first instar larva. As expected, the btl-nGFP reporter is expressed throughout embryonic tracheal development, revealing the ten clusters of tracheal precursor cells in the stage 11 germband extended embryo ()). Dorsal trunk fusion and tracheal branch outgrowth is also evident in stage 13 embryos ()). A higher magnification image reveals that the majority of the GFP from the btl-nGFP reporter resides in the nucleus, as expected ()).
Figure 2. The btl-nGFP construct is expressed in the developing embryonic tracheal system. (a) A lateral view of a stage 11 embryo is depicted with the anterior end on the left. The btl-nGFP construct is expressed in the ten clusters of tracheal precursor cells that are distributed in a segmented pattern along the length of the germband extended embryo. The central amnioserosa is illuminated by background fluorescence. Scale bar = 50 µm. (b) A lateral view of a stage 13 embryo is depicted with the anterior end on the left. The btl-nGFP construct is expressed in the developing tracheal branches. Scale bar = 50 µm. (c) A higher magnification image is depicted of a stage 13 embryo viewed from the lateral perspective with the anterior end on the left. The nuclear localization of the btl-nGFP reporter can be clearly seen in the developing tracheal system and newly-fused dorsal trunk branches. Scale bar = 30 µm.
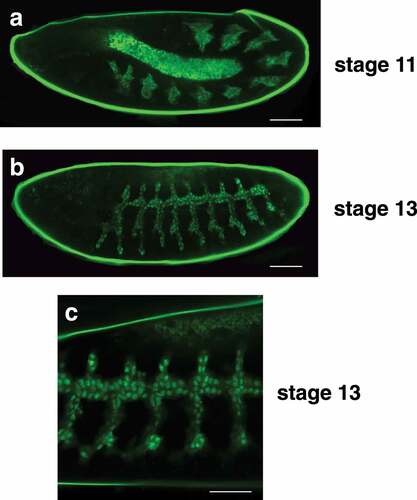
The elaborate structure of the tracheal system is revealed in the second instar larva by btl-nGFP expression ()). The pair of tracheal trunks can be seen along the dorsal surface of the animal with regularly-spaced tracheal branches that extend laterally down the sides. It is more difficult to visualize the tracheal system in third instar larvae because of their large size, but the btl-nGFP reporter is expressed in nuclei throughout the tracheal branches of these animals (, Movie S1). The spiracular branch tracheoblasts can also be seen in these images (, arrows). These small clusters of imaginal cells are located adjacent to the dorsal trunk in third instar larvae and will develop into the adult tracheal tubes and spiracles during metamorphosis [Citation22,Citation23]. Finally, the btl-nGFP reporter can be used to visualize the tracheal system in living animals, providing a dynamic approach for studies of tracheal development and function (Movie S1).
Figure 3. The btl-nGFP reporter is expressed throughout the tracheal system of second and third instar larvae. (a) A dorsal view of a second instar larva is depicted with the anterior end at the top and the posterior spiracles marked with GFP in the lower left. The btl-nGFP reporter can be seen throughout the branched tracheal system. Some background fluorescence is seen in the larval fat body. Scale bar = 100 µm. (b) A dorsal view of a third instar larva is depicted with the anterior end at the top. This panel was constructing by aligning four images captured on the microscope from a single animal. The dashed white lines represent the boundaries of each image. btl-nGFP expression can be seen throughout the branched tracheal system. The spiracular branch tracheoblasts, which are known to express btl, are marked by arrows. Scale bar = 100 µm. (c) A lateral view of a third instar larva is depicted with the anterior end at the top. btl-nGFP expression can be seen throughout the branched tracheal system. The spiracular branch tracheoblasts are marked by arrows. Scale bar = 300 µm.
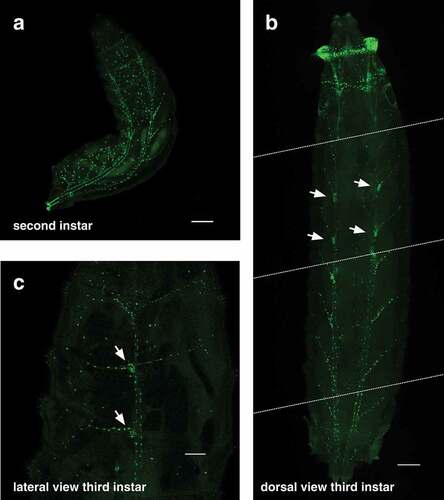
In conclusion, we have generated a direct-drive btl-nGFP reporter that directs high levels of nuclear GFP expression throughout the tracheal system of Drosophila. This construct provides a valuable tool for researchers studying tubular morphogenesis as well as tracheal development and function. The btl-nGFP reporter is available from the Bloomington stock centre (stock # 93131)
Supplemental Material
Download MP4 Video (127.3 MB)Acknowledgments
We thank S. Hayashi for providing pCas4-btl-GAL4 DNA and for discussions regarding btl regulatory sequences, M. Metzstein and C. Merrill for technical advice about imaging living larvae, the University of Utah Cell Imaging Core for access to the confocal microscope, and FlyBase for informatic support. Stocks obtained from the Bloomington Drosophila Stock Center (NIH P40OD018537), and constructs from the Drosophila Genomics Resource Center (NIH 2P40OD010949), were used in this study. This research was supported by the NIH (DK075607, DK108941).
Disclosure statement
No potential conflict of interest was reported by the author(s).
Supplementary material
Supplemental data for this article can be accessed here.
Additional information
Funding
References
- Hayashi S, Kondo T. Development and function of the Drosophila tracheal system. Genetics. 2018;209(2):367–380.
- Kotini MP, Mae MA, Belting HG, et al. Sprouting and anastomosis in the Drosophila trachea and the vertebrate vasculature: similarities and differences in cell behaviour. Vascul Pharmacol. 2019;112:8–16.
- Schottenfeld J, Song Y, Ghabrial AS. Tube continued: morphogenesis of the Drosophila tracheal system. Curr Opin Cell Biol. 2010;22(5):633–639.
- Metzger RJ, Krasnow MA. Genetic control of branching morphogenesis. Science. 1999;284(5420):1635–1639.
- Shiga Y, Tanaka-Matakatsu M, Hayashi S. A nuclear GFP/ß-galactosidase fusion protein as a marker for morphogenesis in living Drosophila. Dev Growth Differ. 1996;38:99–106.
- Glazer L, Shilo BZ. The Drosophila FGF-R homolog is expressed in the embryonic tracheal system and appears to be required for directed tracheal cell extension. Genes Dev. 1991;5(4):697–705.
- Klambt C, Glazer L, Shilo BZ. breathless, a Drosophila FGF receptor homolog, is essential for migration of tracheal and specific midline glial cells. Genes Dev. 1992;6(9):1668–1678.
- Ghabrial A, Luschnig S, Metzstein MM, et al. Branching morphogenesis of the Drosophila tracheal system. Annu Rev Cell Dev Biol. 2003;19:623–647.
- Jazwinska A, Ribeiro C, Affolter M. Epithelial tube morphogenesis during Drosophila tracheal development requires Piopio, a luminal ZP protein. Nat Cell Biol. 2003;5(10):895–901.
- Jiang L, Crews ST. Dysfusion transcriptional control of Drosophila tracheal migration, adhesion, and fusion. Mol Cell Biol. 2006;26(17):6547–6556.
- Metzstein MM, Krasnow MA. Functions of the nonsense-mediated mRNA decay pathway in Drosophila development. PLoS Genet. 2006;2(12):e180.
- Roy S, Hsiung F, Kornberg TB. Specificity of Drosophila cytonemes for distinct signaling pathways. Science. 2011;332(6027):354–358.
- Massarwa R, Schejter ED, Shilo BZ. Apical secretion in epithelial tubes of the Drosophila embryo is directed by the Formin-family protein Diaphanous. Dev Cell. 2009;16(6):877–888.
- Kato K, Chihara T, Hayashi S. Hedgehog and Decapentaplegic instruct polarized growth of cell extensions in the Drosophila trachea. Development. 2004;131(21):5253–5261.
- Ribeiro C, Neumann M, Affolter M. Genetic control of cell intercalation during tracheal morphogenesis in Drosophila. Curr Biol. 2004;14(24):2197–2207.
- Nikolova LS, Metzstein MM. Intracellular lumen formation in Drosophila proceeds via a novel subcellular compartment. Development. 2015;142(22):3964–3973.
- Barolo S, Carver LA, Posakony JW. GFP and beta-galactosidase transformation vectors for promoter/enhancer analysis in Drosophila. Biotechniques. 2000;29(4):727–732.
- Venken KJ, Bellen HJ. Genome-wide manipulations of Drosophila melanogaster with transposons, Flp recombinase, and PhiC31 integrase. Methods Mol Biol. 2012;859:203–228.
- Weiner AT, Seebold DY, Torres-Gutierrez P, et al. Endosomal Wnt signaling proteins control microtubule nucleation in dendrites. PLoS Biol. 2020;18(3):e3000647.
- Ohshiro T, Saigo K. Transcriptional regulation of breathless FGF receptor gene by binding of TRACHEALESS/dARNT heterodimers to three central midline elements in Drosophila developing trachea. Development. 1997;124(20):3975–3986.
- Fish MP, Groth AC, Calos MP, et al. Creating transgenic Drosophila by microinjecting the site-specific phiC31 integrase mRNA and a transgene-containing donor plasmid. Nat Protoc. 2007;2(10):2325–2331.
- Pitsouli C, Perrimon N. Embryonic multipotent progenitors remodel the Drosophila airways during metamorphosis. Development. 2010;137(21):3615–3624.
- Weaver M, Krasnow MA. Dual origin of tissue-specific progenitor cells in Drosophila tracheal remodeling. Science. 2008;321(5895):1496–1499.