ABSTRACT
Phosphorylation and SUMOylation of the kainate receptor (KAR) subunit GluK2 have been shown to regulate KAR surface expression, trafficking and synaptic plasticity. In addition, our previous study has shown that a phosphorylation-dependent interaction of 14–3–3τ and GluK2a-containing receptors contributes to the slow decay kinetics of native KAR-EPSCs. However, it is unknown whether SUMOylation participates in the regulation of the interaction between 14–3–3τ and GluK2a-containing receptors. Here we report that SUMOylation of PKC, but not GluK2, represses the binding of 14–3–3τ to GluK2a via decreasing the phosphorylation level of GluK2a. These results suggest that PKC SUMOylation is an important regulator of the 14–3–3 and GluK2a protein complex and may contribute to regulate the decay kinetics of KAR-EPSCs.
Introduction
Kainate receptors (KARs) are ionotropic glutamate receptors consisting of tetrameric arrangements of 5 subunits (GluK1–5).Citation1,2 At the presynaptic-terminal KARs can modulate neurotransmitter release, and at the postsynaptic membrane they contribute to fast excitatory synaptic transmission.Citation3-6 Slow decay kinetics are one of the important gating features of KARs.Citation7-9 Our previous study showed that protein kinase C (PKC) regulates the properties of GluK2a receptors by controlling phosphorylation-dependent binding of 14–3–3 to GluK2a, and such modulation may contribute to the slow decay kinetics of KAR-excitatory postsynaptic currents (EPSCs).Citation10 Additionally, phosphorylation of KARs by PKC can regulate KAR-mediated synaptic transmission and long-term depression.Citation11-13
SUMOylation is a form of posttranslational modification (PTM) in which the small ubiquitin-related modifier (SUMO) can be covalently attached to lysine residues on target proteins, and regulates target proteins function. Previous studies show that GluK2 can be modified by SUMOylation at lysine residue (K886).Citation14-16 In neurons, SUMOylation participates in regulating ion channel function, membrane protein endocytosis, neuronal differentiation, synaptic transmission and cell survival.Citation14-20 In particular, dynamic control of synaptic SUMOylation regulates KAR synaptic transmission and plasticity.Citation14-16 Previously, both phosphorylation and SUMOylation of KARs have been shown to individually regulate KAR surface expression,Citation13,14 but it is unclear whether the binding of 14–3–3 to GluK2a is modulated by SUMOylation and consequently contributes to the slow kinetics of KAR-EPSCs.
Here, we show that SUMOylation inhibits the binding of 14–3–3 to GluK2a but does not change the level of mRNA and protein expression of GluK2a and 14–3–3τ. We also report that SUMOylation of PKC, but not GluK2, suppresses 14–3–3τ binding to GluK2a. In addition, PKC SUMOylation reduces GluK2 phosphorylation. In view of our previous data showing that PKC can be SUMOylated and its SUMOylation inhibits its activity,Citation20 these results suggest that PKC SUMOylation represses the binding of 14–3–3 to GluK2a by reducing the phosphorylation level of GluK2a.
Result
SUMOylation inhibits the binding of 14–3–3τ to homomeric GluK2a receptors
Our previous study revealed that decay kinetics of GluK2a-containing receptors are modulated by closely associated 14–3–3τ proteins in a phosphorylation-dependent manner.Citation15 To assess whether SUMOylation modification affects the binding of 14–3–3τ to GluK2a, we expressed Flag-tagged 14–3–3τ and GluK2a, either alone or together with GFP-SUMO1 in HEK293T cells. As assessed by coimmunoprecipitation and Western blot analysis, the interaction between 14–3–3τ and GluK2a was significantly attenuated in SUMO1-cotransfected cells compared with a control group that was not cotransfected with SUMO1 ( and ), indicating that SUMOylation inhibited the binding of 14–3–3τ to GluK2a. To provide further support for the inhibitory effect of SUMOylation, we examined the effect of overexpressed wild-type sentrin/SUMO-specific protease 1 (SENP1) and a catalytically inactive mutant SENP1 (SENP1m) in HEK293T cells. As shown in and , the binding of 14–3–3τ to GluK2a was enhanced by overexpression SENP1, but not SENP1m ( and ). To exclude the possibility of a transcriptional mechanism involved in the inhibitory effect of SUMOylation, we examined the mRNA and protein expression of GluK2a and 14–3–3τ in HEK293T transfected with plasmids as indicated. Western blot analysis and quantitative RT-PCR showed that overexpression of either SUMO1 or SENP1 did not change the mRNA ( and ) or protein levels of 14–3–3τ and GluK2a ( and ), suggesting that transcriptional mechanism did not involve in regulating the interaction between 14–3–3τ and GluK2a.
Figure 1. SUMOylation inhibits the binding of 14–3–3τ to homomeric GluK2a receptors. (A, C) Western blot analyses of immunoprecipitates and cell lysates from HEK293T cells transfected with GluK2, Flag-tagged 14–3–3τ and other proteins as indicated. Whole-cell lysates were immunoprecipitated with an anti-Flag antibody and blotted with anti-Flag or anti-GluK2 antibodies. (B, D) Quantification of Western blots in (A) and (C), respectively. These values were determined by measuring the relative intensity of immunoprecipitated bands and their corresponding lysate (L) bands on Western blots and then normalized to and compared with first lane (control). The blot is representative of 4 independent experiments. Data are means ± SEM, **P < 0.01.
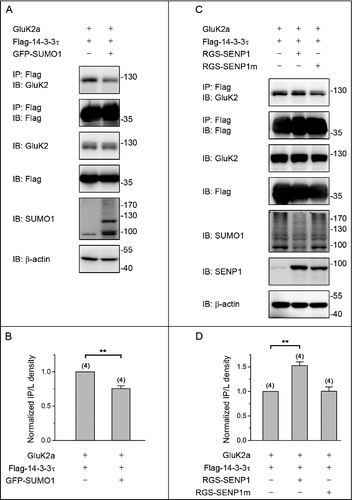
Figure 2. SUMO/deSUMOylation do not alter the level of mRNA and protein expression of GluK2a and 14–3–3τ. (A, C) Western blot analyses of cell lysates from HEK293T cells transfected with GluK2a, Flag-tagged 14–3–3τ and other proteins as indicated. The blot is representative of 3 independent experiments. (B, D) The relative 14–3–3τ and GluK2a mRNA levels in HEK239T cells which were transfected with GluK2a, Flag-tagged 14–3–3τ and other proteins as indicated by quantitative real-time PCR. Data are shown as means ± SEM from 3 independent experiments.
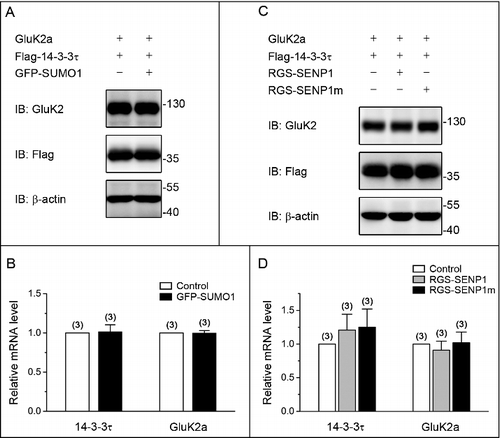
GluK2a SUMOylation does not alter the binding of GluK2a to 14–3–3τ
Previous studies have shown that SUMOylation of GluK2 at lysine 886 regulates endocytosis of KAR and modifies synaptic transmission.Citation11-13 To explore the potential underlying mechanisms for SUMOylation mediated suppression of the binding of GluK2a to 14–3–3τ, we transfected either wild type or the SUMOylation-deficient mutant GluK2a (GluK2a K886R) with Flag-tagged 14–3–3τ and GFP-SUMO1 in HEK293T cells. This revealed that both GluK2a and GluK2a K886R were present with no significant difference in 14–3–3τ immunoprecipitates from the 2 groups ( and ), demonstrating that SUMOylation of GluK2a had no major effect on modulating the interaction of 14–3–3τ and GluK2a.
Figure 3. GluK2a SUMOylation does not change the binding of GluK2a to 14–3–3τ. (A) Western blot analyses of immunoprecipitates and cell lysates from HEK293T cells cotransfected with either GluK2 or SUMOylation mutant site of GluK2 (GluK2 k886R) with Flag-tagged 14–3–3τ. Cell lysates were prepared 24 h post-transfection and immunoprecipitated with anti-Flag antibody followed by Western blot with an anti-GluK2 or anti-Flag antibodies. (B) Quantification of Western blots in (A). The blot is representative of 3 independent experiments. Data are means ± SEM
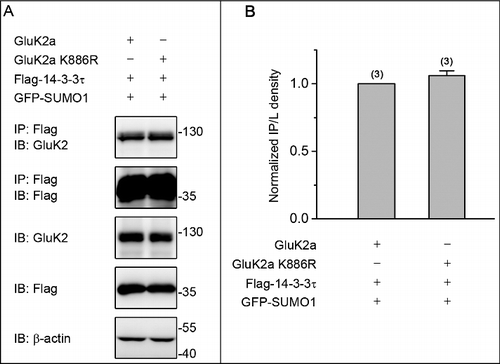
PKC SUMOylation inhibits the binding of 14–3–3τ to GluK2a by decreasing GluK2a phosphorylation
We previously showed that Ser-846 and Ser-868 residues were major 14–3–3-binding sites in the GluK2a subunit, and 14–3–3 proteins modulated the kinetics of GluK2a containing kainate receptors in a PKC phosphorylation-dependent manner.Citation10 Moreover, PKC SUMOylation suppressed its enzymatic activity both in vivo and in vitro.Citation20 Based upon our previous study and current findings that SUMOylation inhibits the binding of GluK2 to 14–3–3τ, we therefore hypothesized that SUMOylation of PKC may repress the binding of GluK2 to 14–3–3τ by altering the status of GluK2 phosphorylation. To test this hypothesis, we transiently transfected HEK293T cells with GluK2a, Flag-tagged 14–3–3τ, HA-tagged PKCα or HA-tagged PKCα K465R (the SUMOylation-deficient K465R PKCα) with or without GFP-SUMO1. Cell lysates were immunoprecipitated using an anti-Flag antibody, followed by immunoblotting using an anti-GluK2 antibody. As expected, PKCα K465R promoted the binding of 14–3–3τ to GluK2a either with or without coexpression of SUMO1 ( and ). Similar results were also obtained using a reverse coimmunoprecipitation strategy ( and ). Together, these data indicate that SUMOylation of PKC represses the binding of GluK2 to 14–3–3τ.
Figure 4. PKC SUMOylation repressed the binding of 14–3–3τ to GluK2. (A) Western blot analyses of immunoprecipitates and cell lysates from HEK293T cells cotransfected with Flag-tagged 14–3–3τ, GluK2, PKCα or the SUMOylation-deficient K465R PKCα, with or without GFP-SUMO1. Whole-cell lysates were immunoprecipitated with anti-Flag antibody and blotted with anti-Flag or anti-GluK2 antibodies. The blot is representative of 4 independent experiments. (B) Quantification of Western blots in (A). (C) HEK293T cells were transfected with plasmids as indicated. Whole-cell lysates were immunoprecipitated with an anti-GluK2 antibody and blotted with anti-Flag or anti-GluK2 antibodies. (D) Quantification of Western blots in (C). The blot is representative of 3 independent experiments. Data are means ± SEM, *P < 0.05, **P < 0.01.
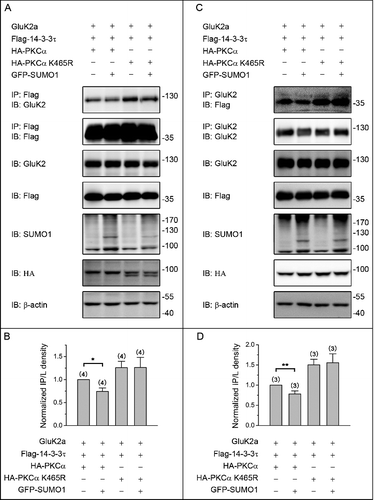
Finally, we compared the phosphorylation status of GluK2a in cotransfected HEK293T cells. As shown in and , the level of GluK2a phosphorylation was significantly reduced by expression of GFP-SUMO1 in the group of cells cotransfected with wild type PKC ( and ). However, GFP-SUMO1 did not alter GluK2a phosphorylation in the groups of cells cotransfected with SUMOylation-deficient K465R PKC ( and ). Taken together, these results suggest that PKC SUMOylation suppresses the binding of 14–3–3τ to GluK2a via decreasing GluK2a phosphorylation.
Figure 5. PKC SUMOylation decreases the level of GluK2a phosphorylation. (A) Western blot analyses of immunoprecipitates and cell lysates from HEK293T cells cotransfected with Flag-tagged 14–3–3τ, GluK2, PKCα or the SUMOylation-deficient K465R PKCα, with or without GFP-SUMO1. Whole-cell lysates were immunoprecipitated with an anti-GluK2 antibody and blotted with anti-phospho-(Ser), anti-Flag or anti-GluK2 antibodies. The blot is representative of 3 independent experiments. (B) Quantification of Western blots in (A). Data are means ± SEM, *P < 0.05.
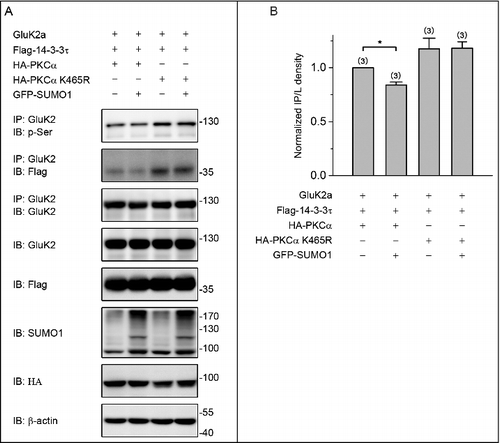
Discussion
Here, we show that inhibition of the binding between GluK2a and 14–3–3τ by SUMOylation is not due to the changes of mRNA or protein expression of GluK2a and 14–3–3τ. Additionally, the binding of GluK2 to 14–3–3τ is modulated by SUMOylation of PKC but not GluK2. Taken together, our results suggest that PKC SUMOylation inhibits the binding of 14–3–3τ to GluK2a by reducing the phosphorylation level of GluK2a.
Our previous study has shown that Ser-846 and Ser-868 residues, the major phosphorylation sites of PKC within the GluK2a C-terminal tail,Citation21 are the binding sites of 14–3–3τ on GluK2.Citation10 More importantly, PKC modulates desensitization kinetics of GluK2a receptors by regulating phosphorylation-dependent binding of 14–3–3 to GluK2a. In addition, SUMOylation of GluK2 regulates endocytosis of KARs and modifies synaptic transmission.Citation14-16 However, our study found that PKC SUMOylation repressed the binding of 14–3–3τ to GluK2, but GluK2 SUMOylation did not alter the binding of GluK2a to 14–3–3τ ( and ). Based on our previous reports that SUMOylation of PKC inhibits its activity,Citation20 those results suggest that the level of GluK2 phosphorylation is decreased via PKC SUMOylation and consequently results in decreased the binding of GluK2 to 14–3–3τ.
In summary, we report that SUMOylation of PKC, but not GluK2, represses the binding of 14–3–3τ to GluK2a via reducing the phosphorylation of GluK2a. Because a protein can be modified by more than one type of PTM, recent studies have provided evidence for functional cross-talk and complex interplay among SUMOylation, phosphorylation, and ubiquitination for several proteins.Citation22-27 Phosphorylation of GluK2 by PKC, PKA and CamKII regulates KAR expression, trafficking, and excitatory synaptic transmission.Citation21,28,29 GluK2 can also be modified by Ubiquitin and SUMO, and consequently regulates its degradation and membrane expression, trafficking.Citation14-16,30 Our results show that SUMOylation of PKC, but not GluK2, inhibits the binding of 14–3–3τ to GluK2a through reducing the phosphorylation of GluK2a ( and ). Previous studies have shown that 14–3–3 proteins are an important regulator of GluK2a-containing KARs and contribute to the slow decay kinetics of native KAR-EPSCs.Citation10 Taken together, these results suggest that PKC SUMOylation is an important regulator of 14–3–3 and GluK2a protein complex and may contribute to regulate the decay kinetics of KAR-EPSCs. Therefore, it is important for future studies to further determine the function of PKC SUMOylation on decay kinetics of KAR-EPSCs.
Materials and methods
Plasmids and antibodies
pcDNA3.1-GluK2a, Flag-14–3–3τ, GFP-SUMO1, RGS-SENP1 and RGS-SENP1m were described previously.Citation10,19,27 GluK2a mutation (GluK2a K886R) was generated using the QuikChange site-directed mutagenesis kits and confirmed by DNA sequencing. Antibodies used were as follows: rabbit anti-GluK2/3 poly-clonal antibody (53518) was from AnaSpec; rabbit anti-GluK5 polyclonal antibody (06–315) was from Millipore; mouse anti-Flag (F1804) was from Sigma-Aldrich; rabbit anti-SUMO1 (4940) was from Cell Signaling Technology.
Quantitative real-time PCR
Total RNA was extracted from HEK293T cells using TRIzol reagents and reverse transcribed to obtain single-strand cDNA using a Reverse Transcription System as described previously.Citation31 Reactions were performed in a 20 μl final volume with Power SYBR Green PCR Master Mix and 0.2–0.5 mM primers using the Applied Biosystems 7500 fast Real-Time PCR System according to the manufacturer's instructions. Results are the average of at least 3 independent biologic replicates. Primers used are: GluK2a: Forward: 5’-AGCGTCGGCTCAAACATAAG-3’ and Reverse: 5’-TTTCTTTACCTGGCAACCTTCT-3’; 14–3–3τ: Forward: 5’-GGACTATCGGGAGAAAGTGG-3’ and Reverse: 5’-TCCTGCACTGTCTGATGTCC-3’; GAPDH: Forward: 5’-CATGGCCTTCCGTGTTCC-3’ and Reverse: 5’-GCCTGCTTCACCACCTTCTT-3’.
Cell culture, transfection, and protein preparation
HEK293T cells were cultured and maintained at 37°C in a 5% CO2 humidified incubator supplemented with Dulbecco's modified Eagle's medium containing 10% fetal bovine serum (Fisher Scientific). Cells were grown to 70–90% confluency and transfected using Lipofectamine 2000 in accordance with manufacturer's instructions.
Immunoprecipitation and western blot
Immunoblotting was performed as described previously with modifications.Citation20 After 24 h of transfection, HEK293T cells were lysed in lysis buffer containing 50 mM Tris-HCl (pH 7.4), 150 mM NaCl, 1% Nonidet P-40, 20 mM NEM (Sigma-Aldrich) and protease inhibitor cocktail (Sigma-Aldrich), and phosphatase inhibitor cocktail (Roche) were added to cell lysates for the detection of phosphorylated GluK2a. The lysates were immunoprecipitation was performed using standard approaches. Briefly, lysates containing 1 mg of proteins were incubated with 1 mg of antibodies overnight at 4 °C, followed by the incubation with 15 μl of protein A/G-agarose at 4 °C for 3 h. The beads were subsequently washed 3 times with lysis buffer and then boiled for 10 min in sample buffer and further analyzed by immunoblotting. Protein densities on Western blots were analyzed by ImageJ software. Relative band densities were determined by normalizing the immunoprecipitated band density to that of the lysate band. All experiments were repeated at least 3 times.
Statistical analysis
Data are expressed as mean ± SEM with statistical significance assessed by Student's t test for 2 group comparisons or one-way analysis of variance tests for multiple comparisons. The value of *, P < 0.05, was considered statistically significant difference.
Disclosure of potential conflicts of interest
No potential conflicts of interest were disclosed.
Funding
This study was supported by grants from the National Basic Research Program of China 2014CB910303 (YL), the National Natural Science Foundation of China 31671053 (YL), 31371064 (YL), and 81171230 (YL), the China Postdoctoral Science Foundation 2016M601608 (YW) and the Shanghai Committee of Science and Technology (11DZ2260200).
References
- Roche KW, Mellor J, Isaac JTR, Hurtado D. Kainate receptor trafficking: physiological roles and molecular mechanisms. Pharmacol Ther. 2004;104(3):163-72. doi:10.1016/j.pharmthera.2004.08.006. PMID:15556673
- Huettner JE. Kainate receptors and synaptic transmission. Prog Neurobiol. 2003;70(5):387-407. doi:10.1016/S0301-0082(03)00122-9. PMID:14511698
- Schmitz D, Mellor J, Nicoll RA. Corrections and Clarifications: Presynaptic Kainate Receptor Mediation of Frequency Facilitation at Hippocampal Mossy Fiber Synapses. Science. 2001;292(5522):1656–. doi:10.1126/science.1057105
- Kamiya H, Ozawa S. Kainate receptor-mediated presynaptic inhibition at the mouse hippocampal mossy fibre synapse. J Physiol. 2000;523 Pt 3(3):653. doi:10.1111/j.1469-7793.2000.t01-1-00653.x. PMID:10718745
- Rodríguez-Moreno A, Herreras O, Lerma J. Kainate receptors presynaptically downregulate GABAergic inhibition in the rat hippocampus. Neuron. 1997;19(4):893-901. doi:10.1016/S0896-6273(00)80970-8. PMID:9354335
- Lerma, J. Roles and rules of kainate receptors in synaptic transmission. Nat Rev Neurosci. 2003;4(6):481-495. doi: 10.1038/nrn1118
- Lerma J, Paternain AV, Rodríguez-Moreno A, López-García JC. Molecular physiology of kainate receptors. Physiol Rev. 2001;81(3):971. PMID:11427689
- Castillo PE, Malenka RC, Nicoll RA. Kainate receptors mediate a slow postsynaptic current in hippocampal CA3 neurons. Nature. 1997;388(6638):182-6. doi:10.1038/40645. PMID:9217159
- Vignes M, Collingridge GL. The synaptic activation of kainate receptors. Nature. 1997; 388(6638):179. doi:10.1038/40639. PMID:9217158
- Sun C, Qiao H, Zhou Q, Wang Y, Wu Y, Zhou Y, Li Y. Modulation of GluK2a subunit-containing kainate receptors by 14-3-3 proteins. J Biol Chem. 2013;288(34):24676-90. doi:10.1074/jbc.M113.462069. PMID:23861400
- Hirbec H, Francis JC, Lauri SE, Braithwaite SP, Coussen F, Mulle C, Dev KK, Coutinho V, Meyer G, Isaac JT. Rapid and Differential Regulation of AMPA and Kainate Receptors at Hippocampal Mossy Fibre Synapses by PICK1 and GRIP. Neuron. 2003;37(4):625-38. doi:10.1016/S0896-6273(02)01191-1. PMID:12597860
- Park Y, Jo J, Isaac JT, Cho K. Long-term depression of kainate receptor-mediated synaptic transmission. Neuron. 2006; 49(1):95-106. doi:10.1016/j.neuron.2005.11.035. PMID:16387642
- Rivera R, Rozas JL, Lerma J. PKC‐dependent autoregulation of membrane kainate receptors. EMBO J. 2007;26(20):4359-67. doi:10.1038/sj.emboj.7601865. PMID:17898803
- Martin S, Nishimune A, Mellor JR, Henley JM. SUMOylation regulates kainate-receptor-mediated synaptic transmission. Nature. 2007;447(7142):321-5. doi:10.1038/nature05736. PMID:17486098
- Konopacki FA, Jaafari N, Rocca DL, Wilkinson KA, Chamberlain S, Rubin P, Kantamneni S, Mellor JR, Henley JM. Agonist-induced PKC phosphorylation regulates GluK2 SUMOylation and kainate receptor endocytosis. Proc Nati Acad Sci U S A. 2011;108(49):19772-7. doi:10.1073/pnas.1111575108. PMID:22089239
- Chamberlain SE, Gonzalez-Gonzalez IM, Wilkinson KA, Konopacki FA, Kantamneni S, Henley JM, Mellor JR. SUMOylation and phosphorylation of GluK2 regulate kainate receptor trafficking and synaptic plasticity. Nat Neurosci. 2012;15(6):845-52. doi:10.1038/nn.3089. PMID:22522402
- Wilkinson KA, Nakamura Y, Henley JM. Targets and consequences of protein SUMOylation in neurons. Brain Res Rev. 2010; 64(1):195-212. doi:10.1016/j.brainresrev.2010.04.002. PMID:20382182
- Rajan S, Plant LD, Rabin ML, Butler MH, Goldstein SA. Sumoylation silences the plasma membrane leak K+ channel K2P1. Cell. 2010; 121(1):37-47. doi:10.1016/j.cell.2005.01.019
- Qi Y, Wang J, Bomben VC, Li DP, Chen SR, Sun H, Xi Y, Reed JG, Cheng J, Pan HL, et al. Hyper-SUMOylation of the Kv7 potassium channel diminishes the M-current leading to seizures and sudden death. Neuron. 2014;83(5):1159-71. doi:10.1016/j.neuron.2014.07.042. PMID:25189211
- Sun H, Lu L, Zuo Y, Wang Y, Jiao Y, Zeng WZ, Huang C, Zhu MX, Zamponi GW, Zhou T, et al. Kainate receptor activation induces glycine receptor endocytosis through PKC deSUMOylation. Nat Commun. 2014;5:4980. doi:10.1038/ncomms5980. PMID:25236484
- Nasu-Nishimura Y, Jaffe H, Isaac JTR, Roche KW. Differential regulation of kainate receptor trafficking by phosphorylation of distinct sites on GluR6. J Biol Chem. 2010;285(4):2847-56. PMID:19920140
- Desterro JM, Rodriguez MS, Hay RT. SUMO-1 modification of IkappaBalpha inhibits NF-kappaB activation. Mol Cell. 1998;2:233-9. doi:10.1016/S1097-2765(00)80133-1. PMID:9734360
- Glotzer JB, Saltik M, Chiocca S, Michou A-I, Moseley P, Cotten M. Activation of heat-shock response by an adenovirus is essential for virus replication. Nature. 2000;407(6801):207-11. doi:10.1038/35025102. PMID:11001061
- Carter S, Bischof O, Dejean A, Vousden KH. C-terminal modifications regulate MDM2 dissociation and nuclear export of p53. Nat Cell Biol. 2007;9(4):428-35. doi:10.1038/ncb1562. PMID:17369817
- Guo Z, Kanjanapangka J, Liu N, Liu S, Liu C, Wu Z, Wang Y, Loh T, Kowolik C, Jamsen J, et al. Sequential posttranslational modifications program FEN1 degradation during cell-cycle progression. Mol Cell. 2012; 47(3):444-56. doi:10.1016/j.molcel.2012.05.042. PMID:22749529
- Luo HB, Xia YY, Shu XJ, Liu ZC, Feng Y, Liu XH, Yu G, Yin G, Xiong YS, Zeng K, et al. SUMOylation at K340 inhibits tau degradation through deregulating its phosphorylation and ubiquitination. Proc Nati Acad Sci U S A. 2014;111(46):16586-91. doi:10.1073/pnas.1417548111. PMID:25378699
- Wang Y, Wang Y, Zhang H, Gao Y, Chao H, Zhou A, Yi Z, Yong L. Sequential posttranslational modifications regulate PKC degradation. Molecular biology of the cell. 2015;27(2):410-20. doi:10.1091/mbc.E15-09-0624. PMID:26564794
- Cho K, Francis JC, Hirbec H, Dev K, Brown MW, Henley JM, Bashir ZI. Regulation of kainate receptors by protein kinase C and metabotropic glutamate receptors. J Physiol. 2003;548(3):723-30. doi:10.1113/jphysiol.2003.040188. PMID:12640005
- Carta M, Opazo P, Veran J, Athané A, Choquet D, Coussen F, Mulle C. CaMKII-dependent phosphorylation of GluK5 mediates plasticity of kainate receptors. EMBO J. 2013;32(4):496-510. doi:10.1038/emboj.2012.334. PMID:23288040
- Salinas GD, Blair LA, Needleman LA, Gonzales JD, Chen Y, Li M, Singer JD, Marshall J. Actinfilin is a Cul3 substrate adaptor, linking GluR6 kainate receptor subunits to the ubiquitin-proteasome pathway. J Biol Chem. 2006;281(52):40164-73. doi:10.1074/jbc.M608194200. PMID:17062563
- Zhang H, Wang Y, Zhu A, Huang D, Deng S, Cheng J, Zhu MX, Li Y. SUMO-specific protease 1 protects neurons from apoptotic death during transient brain ischemia/reperfusion. Cell Death Dis. 2016;7(11):e2484. doi:10.1038/cddis.2016.290