Similar to GABAA receptor-channels the calcium-mediated gating of the small-conductance KCa2 and the intermediate-conductance KCa3.1 channels can be positively or negatively modulated by small molecule drugs, which, in analogy to the GABA field, have been termed positive (PAM) or negative allosteric modulators. While positive gating modulators like EBIO, NS309, SKA-31 and SKA-121 shift the calcium-response curve of these voltage-independent, calmodulin-gated channels to the left and apparently increase their sensitivity to calcium, negative gating modulators decrease calcium sensitivity.Citation1 However, in contrast to GABAA receptors, where the binding site for the endogenous ligand GABA is located on the extracellular side and where allosteric modulation by benzodiazepines, neurosteroids and barbiturates has been studied in exquisite detail, only a small number of studies have been performed for KCa channels. One reason is of course the lower level of pharmacological interest. While GABAA receptors are firmly established as clinically used drug targets, no KCa2 or KCa3.1 channel modulators have yet reached the clinic despite their undeniable therapeutic potential for neurological, cardiovascular and inflammatory diseases.Citation1 Another reason is the technical challenge involved in studying KCa channel gating. The gating apparatus is located at the intracellular C-terminus, where calmodulin, which functions as a calcium-sensing β-subunit, is constitutively associated with the calmodulin binding domain of the channels,Citation2 necessitating the performance of inside-out patch-clamp recordings when aiming to work at defined intracellular calcium concentrations. Nevertheless, a few studies, including some exquisite X-ray crystallography,Citation3,4 have been performed and it is currently hypothesized that KCa channel PAMs bind at the interface between the calmodulin N-lobe and the calmodulin-binding domain of the channels and thus “facilitate” mechanical opening ( = increased open channel probability) at a given Ca2+ concentration.
Both benzimidazole-type activators like EBIO and NS309 and naphthothiazole/oxazole-type activators like SKA-31 and SKA-121 () have been shown to bind in this interface pocket either through co-crystallization of calmodulin in complex with the calmodulin-binding domain of KCa2.2,Citation3,4 or, more recently, by our own group using a combination of electrophysiology and site-directed mutagenesis.Citation5 The latter study was guided by homology modeling of the KCa2.3 and KCa3.1 interface pocket and docking studies using the RosettaLigand computational modeling software. While the crystallography studiesCitation3,4 afforded the first insight into the atomistic mechanism of action of KCa activators, our molecular modeling study provides a plausible explanation for why KCa channel activators in general are 5–10-fold more potent in activating KCa3.1 than KCa2 channels.Citation5 The presence of R362 creates an extensive “background” hydrogen-bond network in the KCa3.1 interface pocket that stabilizes the main contacts NH2-substituted KCa activators make with M51 and E54 in calmodulin (). The three KCa2 channels have shorter N or S residues in the corresponding position and therefore cannot form this hydrogen-bond network. The Rosetta models further suggested an explanation for why the 5-position methyl substituted SKA-121 is more potent on KCa3.1 and less potent on KCa2.3 than its parent compound SKA-31 by identifying an increased number of hydrophobic interactions in the “back” of the interface pocket for SKA-121 in the most frequently sampled lowest energy binding poses in KCa3.1.
Figure 1. Top, Chemical structures of the KCa channel activators and Rosetta model of SKA-121 (orange) docked into the interface between the KCa3.1 calmodulin-binding domain (blue) and calmodulin (yellow). See Brown et al.Citation5 for details. Bottom, Cartoon of the effect of EBIO or NS309 on the calcium-response curve of KCa2.2 and of SKA-121 on the calcium-response curve of KCa3.1.
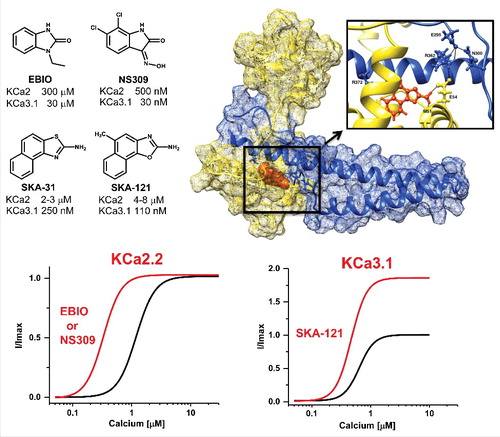
While these homology models are certainly helpful for explaining selectivity or for attempting structure based drug design, they fail to explain the experimentally observed ability of SKA-121 to further potentiate KCa currents at saturating Ca2+ concentrations. All previously published calcium-response curves for EBIO or NS309 on KCa2.2 show a “clean” left-ward shift without any increase in maximal effect (). SKA-121, in contrast, doubles KCa3.1 currents even in the presence of 10 μM of free intracellular calcium. This potentiation above the effect of the endogenous ligand, which is reminiscent of the superagonism observed on extrasynaptic GABAA receptors, could potentially be explained by the assumed relatively low Ca2+-dependent Po(max) of KCa3.1. However, it becomes harder to explain for KCa2.3, where SKA-121 is also still able to further potentiate currents in the presence of even 30 μM free calciumCitation5 despite the fact that KCa2 channels are supposedly already fully open.
Future studies of KCa channel gating and the mechanism of action of KCa activators therefore will have to address several questions. First, how does the calmodulin mediated gating of the channels actually work? The dimer-of-dimers model suggested by the C-terminal crystal structures,Citation3,4,6 which all show two anti-parallel KCa2.2 fragments and two anti-parallel calmodulins forming a dimeric complex, has been questioned in favor of a model with four-fold rotational symmetry.Citation7 This debate is unlikely to be resolved before a full-length structure of a KCa2 or KCa3.1 channel becomes available. Second, how do small molecules affect the gating and do they have the same effects on KCa3.1 and KCa2 channels? Up to now our laboratory is the only group that published KCa3.1 calcium-response curves in the presence of a KCa activator raising the question whether there are intrinsic differences between KCa3.1 and KCa2 channels, for which phosphatidylinositol 4,5-bisphosphate (PIP2) has recently been shown to regulate channel activity by binding to the KCa2.2 calmodulin-binding domain/calmodulin complex.Citation8 3) Are there superagonists and partial agonists for KCa channels? And lastly, how different are KCa agonists that bind in the C-terminal interface pocket from KCa agonistsCitation1 that bind in the pore domain?
Disclosure of potential conflicts of interest
No potential conflicts of interest were disclosed.
Funding
This work was supported by the CounterACT Program, National Institutes of Health Office of the Director [U54NS079202], and the National Institute of Neurological Disorders and Stroke [R21NS101876].
References
- Christophersen P, Wulff H. Pharmacological gating modulation of small- and intermediate-conductance Ca2+-activated K+ channels (KCa2.x and KCa3.1). Channels (Austin). 2015;9:336-343. doi:10.1080/19336950.2015.1071748. PMID:26217968
- Kaczmarek LK, Aldrich RW, Chandy KG, Grissmer S, Wei AD, Wulff H. International Union of Basic and Clinical Pharmacology. C. Nomenclature and properties of calcium-activated and sodium-activated potassium channels. Pharmacol Rev. 2017; 69:1-11. doi:10.1124/pr.116.012864. PMID:28267675
- Zhang M, Pascal JM, Schumann M, Armen RS, Zhang JF. Identification of the functional binding pocket for compounds targeting small-conductance Ca2+-activated potassium channels. Nat Commun. 2012;3:1021. doi:10.1038/ncomms2017. PMID:22929778
- Zhang M, Pascal JM, Zhang JF. Unstructured to structured transition of an intrinsically disordered protein peptide in coupling Ca2+-sensing and SK channel activation. Proc Natl Acad Sci USA. 2013;110:4828-4833. doi:10.1073/pnas.1220253110. PMID:23487779
- Brown BM, Shim H, Zhang M, Yarov-Yarovoy V, Wulff H. Structural determinants for the selectivity of the positive KCa3.1 gating modulator 5-methylnaphtho[2,1-d]oxazol-2-amine (SKA-121). Mol Pharmacol. 2017; 92:469–480; doi:10.1124/mol.117.109421. PMID:28760780
- Halling DB, Kenrick SA, Riggs AF, Aldrich RW. Calcium-dependent stoichiometries of the KCa2.2 (SK) intracellular domain/calmodulin complex in solution. J Gen Physiol. 2014;143:231-252. doi:10.1085/jgp.201311007. PMID:24420768
- Schumacher MA, Rivard AF, Bächinger HP, Adelman JP. Structure of the gating domain of a Ca2+-activated K+ channel complexed with Ca2+/calmodulin. Nature. 2001;410:1120-1124. doi:10.1038/35074145. PMID:11323678
- Zhang M, Meng XY, Zhang JF, Cui M, Logothetis DE. Molecular overlap in the regulation of SK channels by small molecules and phosphoinositides. Sci Adv. 2015;1:e1500008. doi:10.1126/sciadv.1500008. PMID:26366439