ABSTRACT
Proton-gated channels of the ASIC family are widely distributed in the mammalian brain, and, according to the recent data, participate in synaptic transmission. However, ASIC-mediated currents are small, and special efforts are required to detect them. This prompts the search for endogenous ASIC ligands, which can activate or potentiate these channels. A recent finding of the potentiating action of histamine on recombinant homomeric ASIC1a has directed attention to amine-containing compounds. In the present study, we have analyzed the action of histamine, tyramine, and tryptamine on native and recombinant ASICs. None of the compounds caused potentiation of native ASICs in hippocampal interneurons. Furthermore, when applied simultaneously with channel activation, they produced voltage-dependent inhibition. Experiments on recombinant ASIC1a and ASIC2a allowed for an interpretation of these findings. Histamine and tyramine were found to be inactive on the ASIC2a, while tryptamine demonstrated weak inhibition. However, they induce both voltage-dependent inhibition of open channels and voltage-independent potentiation of closed/desensitized channels on the ASIC1a. We suggest that the presence of an ASIC2a subunit in heteromeric native ASICs prevents potentiation but not inhibition. As a result, the inhibitory action of histamine, which is masked by a strong potentiating effect on the ASIC1a homomers, becomes pronounced in experiments with native ASICs.
Introduction
Trimeric proton-gated ion channels of the ASIC family participate in various brain functions and contribute to different pathologies.Citation1–3 According to an increasing amount of evidence, they are involved in the postsynaptic response.Citation4–7 Since the content of synaptic vesicles is acidic, the release of a neurotransmitter results in synaptic cleft acidification. Protons released together with a neurotransmitter can reach postsynaptic ASICs and activate them, thus producing sodium influx, which additionally depolarizes the postsynaptic membrane. However, available electrophysiological data show very small ASIC-mediated currents, which can hardly play a significant role. Relatively large currents were registered in the Calyx of Held.Citation7 This result clearly demonstrates the synaptic localization and function of the ASICs, but the contribution of these channels to the function of classical CNS synapses remains unclear.
A possible solution to the apparent disagreement between the well-established role of ASICs in the CNS and the subtle currents recorded in synaptic preparations is that some presently unknown endogenous ligand(s) potentiate ASICs responses. Many endogenous modulators of the ASICs are known.Citation8 Extracellular Ca2+ ions inhibit ASICs through two distinct mechanisms. They can bind to the acidic pocket and thus reduce pH sensitivityCitation9; another binding site exists at the entrance to the pore.Citation10 Other divalent cations also inhibit ASICs.Citation11,Citation12 Endogenous polyamines agmatine and arcaine cause activation of ASIC3-containing channels.Citation13 Spermine potentiates ASICs of different subunit compositions, including native ASICs in hippocampal neurons.Citation14,Citation15 The proposed mechanism of spermine action is a shift of pH-dependence of inactivation. Another class of ASIC potentiators includes neuropeptides like FMRF-amide and dynorphin.Citation16–19 Arachidonic acid and anandamide potentiate ASICs by shifting pH-dependence of activation.Citation20–22 Nitric oxide also should be mentioned as an ASIC potentiator.Citation23 Thus, the list of endogenous ASIC modulators is rather long, possible mechanisms are diverse, and it remains unclear how they affect ASIC activity in physiological and pathological conditions. Drug action depends strongly on subunit composition, which greatly complicates the development of ASIC pharmacology. ASIC subunits readily form various functional heteromers and, as a result, native ASICs can show very complex pharmacological profile.Citation24,Citation25
We have found recently that various amine-containing compounds potentiate or inhibit ASICs depending on the structural peculiarities and subunit composition of the target.Citation26,Citation27 In particular, the phenylcyclohexyl derivative IEM-2044 in which the hydrophobic moiety is connected to the amino group by two methylene spacers was found to potentiate ASIC1a homomers.Citation28 A similar structural organization occurs naturally in histamine. Subsequent experiments revealed significant potentiation of ASIC1a by histamine.Citation29 It demonstrates the most prominent potentiation at weak acidification conditions, which cause a minor response, and is virtually ineffective at saturating acidifications. Histamine produces a parallel shift of the concentration dependence of ASIC1a activation. This effect may be due to an allosteric increase of proton sensitivity. Alternatively, the amino group of histamine may directly interact with one of the proton binding sites, thus providing the co-agonistic effect. Therefore, histamine can play the role of a positive ASIC1a modulator in the CNS.
A number of synthetic amines were found to modulate ASICs.Citation28 Consequently, endogenous amines besides histamine should be considered as putative ASIC ligands. In the present work, we studied the action of naturally occurring amines tyramine and tryptamine on native and recombinant ASICs and compared them with histamine. The aim was to extend the list of endogenous ASIC modulators and to understand their mechanisms of action.
Different rat brain neurons respond to external acidification by ASIC-mediated currents with varying characteristics.Citation30 The ASICs in the hippocampal CA1 interneurons from the oriens lacunosum-moleculare layer are likely to be composed of ASIC1a and ASIC2 subunits, and they produce rather large currents in response to acidification.Citation31 In contrast, the pyramidal neurons from the same area produce only small currents in response to acidification.Citation30,Citation31 In our previous study, synthetic monoamines were shown to significantly affect native ASICs in hippocampal interneurons: IEM-1921 and IEM-2117 potentiate them, whereas memantine and 9-aminoacridine cause inhibition.Citation26 These characteristics do not match the data obtained on homomeric recombinant receptors, suggesting a heteromeric organization of ASICs in hippocampal interneurons. Potentiation caused by IEM-1921 and IEM-2117 is likely to be due to the presence of an ASIC2a in the heteromeric receptors; memantine and 9-aminoacridine work as inhibitors for an ASIC1a or an ASIC1b. Thus, our data agree with earlier results demonstrating ASIC1a and ASIC2 subunits contribution to native ASICs in the hippocampal interneurons. In the present work, we used this type of neurons to study the action of endogenous amines on native ASICs. Taking into account putative subunit composition of ASICs in hippocampal interneurons, we compared the data on these native ASICs with recombinant ASIC1a and ASIC2a.
Results
Action of endogenous amines on native ASICS in hippocampal interneurons
Channels were activated by 7sec pH drops from 7.4 to 6.5 with 30 sec intervals that provide complete recovery from desensitization. Experiments were performed at -80mV holding voltage, unless otherwise stated. Structures of the amines studied are presented in .
Figure 1. Action of histamine, tyramine and tryptamine on native ASICs. A, chemical structures of the compounds. B, representative recording demonstrates dependence of tryptamine action on the application protocol. After control recording (response I) tryptamine was applied in both neutral- and low-pH solution and caused inhibition (response II). Analogous effect was observed if tryptamine was applied simultaneously with activation (response III). In case of application of tryptamine between activations, no significant effect was detected (response IV). C, summary of effects of tryptamine, tyramine and histamine (1 mM) in three application protocols. D, concentration-dependence of inhibition evoked by tryptamine application simultaneously with activation.
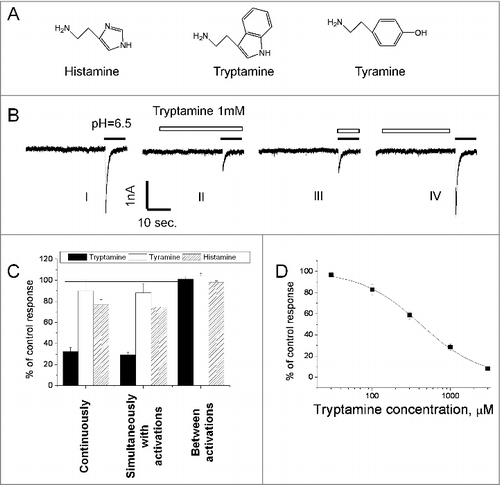
Drug action on ion channels, in particular on ASICs, can be mediated by different molecular mechanisms. To fully understand their effects in vivo and to design new potent compounds, we need to uncover the mechanisms of drug action. To that end, after recording the control response (response I in ), we have used three protocols of drug application. The continuous presence of a compound (see response II in ) may produce an environment which is similar to the physiological conditions of action. However, this type of drug application does not allow us to determine if a drug interacts with an open channel, a closed channel, or its binding is state-independent. To reveal an interaction with an open channel, the drugs were applied simultaneously with the activating acidification (see response III in ). When the compounds were applied between activations (see response IV in ), they interacted mainly with the closed channels. After each type of drug application, we recorded test responses in the absence of a drug, in order to take into account response rundown during the experiment and to ensure reversibility of the effects (not shown).
1mM tryptamine demonstrated drastically different action in different application protocols (). Application between activations did not cause any effect, but continuous application and application simultaneous with activation evoked 72 ± 15% (n = 6) and 68 ± 18% (n = 6) inhibition ( and C). According to paired t-test, these effects were not statistically different (P > 0.1, n = 6). Thus, tryptamine does not affect ASICs in the closed state and inhibits them in the open state. Analysis of the concentration-dependence of tryptamine action was performed for the simultaneous drug application. 3 mM evoked almost complete inhibition, the IC50 value was estimated as 420 ± 80 µM and Hill coefficient was 1.1 ± 0.2 ().
Our previous study has demonstrated that histamine selectively potentiates recombinant ASIC1a.Citation29 In the present work, we did not observe the potentiating effect of histamine on native ASICs in the hippocampal interneurons (), thus confirming our previous suggestion that the response evoked in hippocampal interneurons by pH drops is not mediated by a homomeric ASIC1a.
Moreover, we found a subtle, but significant (paired t-test, n = 6, P < 0.05) difference of the effect in different application protocols. If 1mM histamine was applied continuously or during activations it caused 25 ± 6% (n = 6) inhibition. Such effect was absent if histamine was applied between ASIC activations. Similar minor effect (11 ± 8%, n = 6) was observed for 1mM tyramine applied continuously and during activations (). This dependence of action on the application protocol suggested an effect of the drugs on the open but not on the closed channels.
The open-channel inhibition effect often occurs if the drug molecule enters the open pore and sterically blocks ion permeation. In such cases, the charged blocking molecule binds in the area of the membrane electric field and the binding depends on the membrane voltage. Analysis of the tryptamine block revealed significant voltage dependence. 1mM produced only about 20% inhibition at 0 mV, but the effect reached 80% at – 120mV (). Quantitative analysis of voltage dependence was performed using Woodhull model.Citation32 According to this model, the fraction of the membrane field that the tryptamine molecule crosses from external surface to the binding site (δ value) is 0.63 ± 0.17, n = 7 (). The finding of the voltage dependence of tryptamine action allowed us to readdress the nature of weak effects of histamine and tyramine. In this series of experiments, the concentration of tyramine and histamine was increased to 3 mM. They were applied simultaneously with activation since this protocol favors the inhibitory effect. For both drugs significant voltage dependence was detected; δ values for tyramine and histamine were 0.58 ± 0.14, n = 5 and 0.48 ± 0.16, n = 6, respectively. Thus, all three amines cause a voltage-dependent open-channel block of native ASICs in the hippocampal interneurons. Despite certain differences between the δ values obtained for tyramine, tryptamine, and histamine, the data suggest a common binding region in the ion pore of ASICs.
Figure 2. Action of endogenous amines on native ASICs is voltage-dependent. A, control responses (black) are superimposed with responses in the presence of 1 mM tryptamine applied simultaneously with activation (gray). Tryptamine caused strong inhibition at −120 mV but was virtually inactive at 0 mV. B, voltage dependence of action of tyramine, tryptamine and histamine. Although tryptamine is more potent than other amines, all three compounds demonstrated similar voltage dependence of inhibition.
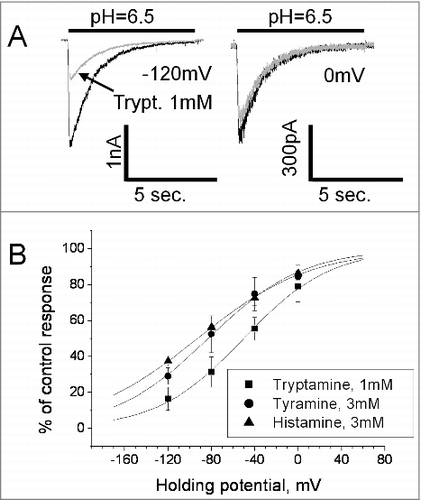
Action of endogenous amines on homomeric ASIC1a
Heteromeric nature of ASIC response in hippocampal interneurons requires analysis of drug action on homomeric recombinant ASICs to reveal subunit-specificity. ASIC1a homomers were activated by 10sec pH drops from 7.4 to 6.5 at −80 mV holding voltage. The data are presented in
Figure 3. Action of endogenous amines on ASIC1a. A, summary of effects of tryptamine, tyramine and histamine (1 mM) in three application protocols at two conditioning pH values. Histamine strongly potentiates the response in all conditions. Tryptamine caused inhibition being applied simultaneously with activation. At conditioning pH = 7.1 application of tryptamine between activations potentiates the response. Action of tyramine is weak. Only at conditioning pH = 7.1 continuous application and application between activations caused significant potentiation. B, representative recordings demonstrate opposite effects of tryptamine applied simultaneously with activation (left) and between activations (right). Control responses (black) are superimposed with responses affected by 1 mM tryptamine (gray). C, representative recordings demonstrate that at conditioning pH = 7.1 tyramine causes potentiation (left), whereas application simultaneously with activation does not produce any effect (right). D, at conditioning pH = 7.1 histamine causes strong potentiation being applied between activation (left); application simultaneously with activation produces significantly smaller potentiation.
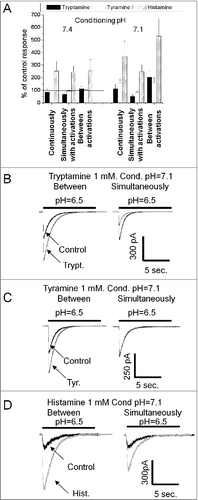
Histamine caused strong potentiation in all three protocols (). In contrast, 1mM tyramine was virtually inactive (n = 7, paired t-test drug vs control, P > 0.1). When 1 mM tryptamine was applied simultaneously with activations, it caused 31 ± 18% (n = 7 paired t-test drug vs control, P < 0.05) inhibition. Surprisingly, application of 1mM tryptamine between channel activations induced some potentiation of the response (13 ± 14%, n = 7). Although the effect was not statistically significant (paired t-test drug vs control, P > 0.1) the result suggested that tryptamine may cause different effects on ASIC1a depending on the application protocol.
If a drug applied between activations significantly interacts with the channels in the resting state, its action can depend on the initial pH value, and in particular on the steady-state desensitization. To test this possibility we performed experiments in which initial pH was reduced from 7.4 to 7.1 (). Such modest acidification causes 50–70% desensitization of the ASIC1a (data not shown). Analysis of tryptamine action on responses evoked by pH drops from 7.1 to 6.5 () revealed a strong (100 ± 40%, n = 5) potentiating effect of the drug applied between activations. shows that we were also able to reveal potentiation by tyramine applied between activations (62 ± 19%, n = 5) at conditions of partially inactivated channels. We also found a significant difference of histamine action at different application protocols in these experiments. Application simultaneous with activation caused much smaller potentiation than application between activations (). The continuous presence of the drug resulted in an intermediate effect on the ASIC1a in all cases.
It has been shown in our previous workCitation29 that the potentiating action of histamine strongly depends on the activating pH, and at saturating acidification potentiation becomes negligible. Therefore, we tested the dependence of tryptamine-induced potentiation on the activating pH. Because significant potentiation was observed only for the application between activations at conditioning pH = 7.1, we used this protocol to compare the effect tryptamine had on ASIC1a responses when activated by a pH drop to 6.5 and to 5.0. Direct comparison of the effects in paired experiments did not reveal statistical differences (n = 6, paired t-test, P > 0.1).
Next, we analyzed voltage- and concentration-dependencies of the potentiating and inhibitory action of tryptamine at conditioning pH = 7.1. Inhibition was studied for the drug application simultaneous with activation. The IC50 value for inhibitory action was estimated as 1.3 ± 0.2 mM (n = 6). Application of 3mM tryptamine (highest concentration used) between activations resulted in 410 ± 110% (n = 5) potentiation but did not demonstrate saturation, and it was impossible to estimate the parameters of the curve. Qualitatively similar data were obtained for tyramine, but the effects were much weaker in all cases (data not shown). Comparison of the effects at -40, -80 and -120 mV revealed a drastic difference between inhibition and potentiation of the ASIC1a by tryptamine. Inhibition, measured in the protocol of drug application simultaneous with activation, demonstrated strong voltage dependence ( and B), whereas potentiation caused by application between activations did not demonstrate significant dependence on the holding voltage ( and D).
Figure 4. Voltage dependence of action on ASIC1a. A, C and E representative recordings of ASIC1a currents in control (black) superimposed with responses in the presence of endogenous amines (gray) at conditioning pH = 7.1. B, D and F summary data. A and B, inhibitory action of tryptamine applied simultaneously with activation increases with hyperpolarization. C and D, potentiating action of tryptamine applied between activations is voltage-independent. E and F, Potentiating action of histamine applied simultaneously with activation decreases with hyperpolarization suggesting that total effect includes voltage-dependent inhibitory component.
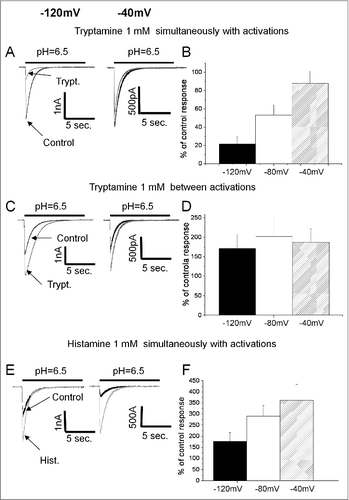
Although histamine demonstrated potentiating effect in all protocols, it remains possible that a modest inhibition is masked by the strong potentiation. To test for this possibility we studied the voltage dependence of its action at conditioning pH = 7.1, and found that the potentiation by histamine applied simultaneously with activation is significantly more pronounced at 0 mV than at -140 mV ( and F). The finding of the voltage dependence of histamine effect agrees with the data demonstrating a difference between application protocols. Taken together, these results suggest that in addition to a strong potentiation effect described by us previously,Citation29 histamine can block open ASIC1a channels.
Action of endogenous amines on homomeric ASIC2a
Taking into account different sensitivity of ASIC1a and ASIC2a to protons, as well as different activation and desensitization kinetics, ASIC2a homomers were activated by 40sec pH drops from 7.4 to 5.0. Holding voltage was -80mV. To test the possible effect of the steady-state desensitization we also used conditioning pH = 6.5 (). Tyramine and histamine in a 1 mM concentration were inactive (paired t-test, drug vs control, P > 0.1, n = 5 for all series) in all six series of experiments. Tryptamine was ineffective in the protocol of application between activations independently of the value of conditioning pH (data not shown). However, statistically significant inhibition () was found in the protocol of application simultaneous with activation (25 ± 12%, n = 12, paired t-test drug vs control, P < 0.05). Continuous application also caused statistically significant inhibition (22 ± 18%, n = 10, paired t-test drug vs control, P < 0.05). The values of inhibition did not depend on conditioning pH (n = 5, paired t-tests, P > 0.1). Application of 3 mM of tryptamine caused a 64 ± 22%, n = 5 inhibition in the protocol of application simultaneous with activation. The IC50 value calculated from the concentration dependence of tryptamine action was 1.5 ± 0.4 mM, n = 6. Continuous application produced the same value of inhibition (data not shown).
Figure 5. Action of endogenous amines on ASIC2a. A, summary of effects of tryptamine, tyramine and histamine (1 mM) in three application protocols at conditioning pH = 6.5. B, at conditioning pH = 6.5 1 mM tryptamine applied between activations does not produce an effect (left), whereas application simultaneously with activation causes inhibition (right).
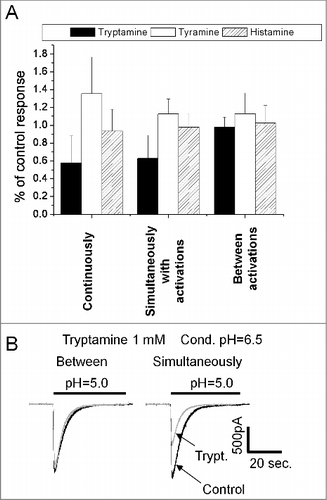
Thus, there was a drastic difference between the action of amines tested on ASIC1a and ASIC2a. No ASIC2a potentiation was detected even at the most favorable conditions, i.e. in the case of an application between activations at conditioning pH causing partial steady-state inactivation. Significant inhibition was found only for tryptamine, whereas histamine and tyramine were completely ineffective.
Discussion
In the present work, we demonstrated that ASICs are modulated not only by histamine but also by tyramine and tryptamine. The action of these monoamines is relatively weak and it is hardly possible that they significantly contribute to the physiological ASIC modulation. However, the fact that different endogenous amines are able to interact with ASICs should prompt further search for more potent native modulators. Another finding is that histamine, which was previously described as a selective potentiator of the ASIC1a homomers,Citation29 does not potentiate heterogenic ASIC response in the hippocampal interneurons. Moreover, in the absence of potentiation, we can reveal the inhibitory action of histamine.
Various factors could determine the differences of drug action in native and recombinant systems. In the case of neurons, we cannot rule out the possibility of involvement of the histamine receptors (HR1-HR4). However, the obvious similarity of action of all three endogenous amines is evidence against such possibility. Other cell-specific modulation mechanisms, which can exist in neurons but not in CHO cells, may contribute to the observed differences. However, the most plausible explanation is heteromeric subunit composition of native ASICs in the hippocampal interneurons. Different ASIC subunits readily form functional heteromeric channels and characteristics of heteromeric and homomeric channels are markedly different.Citation33 Single-cell RT-PCR analysis reveals coexpression of the ASIC1a and the ASIC2 subunit transcripts in hippocampal CA1 interneurons from the oriens lacunosum-moleculare layer.Citation31 Notably, the same study demonstrated that tarantula venom psalmotoxin1, a specific blocker for ASIC1a homomers, did not inhibit ASIC currents in these neurons. Therefore, we suggest that the lack of potentiating effect of histamine on hippocampal interneurons is due to the presence of an ASIC2 subunit, which, according to our data, forms histamine-insensitive channels. Inhibitory effects are seen in both the ASIC1a and native ASICs. Thus, the presence of ASIC2a, which is almost insensitive to the amines tested, seems to destroy potentiation but not inhibition. As a result, the inhibitory action of histamine, masked by a strong potentiating effect on the ASIC1a homomers, becomes pronounced in experiments with native ASICs. Systematic testing of this suggestion would require a comparison of action of a representative series of compounds on both heteromeric recombinant and native ASICs from different brain structures.
Comparison of different application protocols allowed us to reveal important determinants of the mechanism of action of endogenous amines on native and recombinant ASICs. The total effect, which is observed in the continuous presence of a drug in solution, is a sum of two independent types of action. If the drugs are applied simultaneously with activation they interact mainly with the open channels. In this case, we observe voltage-dependent channel block. If the drugs are applied only between activations and interact with resting or desensitized channels, they can cause voltage-independent potentiation. The existence of two binding sites and two independent mechanisms of ASIC modulation by amine-containing compounds was proposed by us previously to explain complex structure-activity relationships.Citation28 In the present work, we separated these mechanisms experimentally.
Dualistic ligand action on ASICs is also known for amiloride, which is usually considered to be an ASIC inhibitor but can induce a potentiating effect.Citation34,Citation35 Although the sites of action are still not exactly known, the X-ray dataCitation36 shows that amiloride can bind in the channel pore and cause blocking effect. Another putative site of amiloride is near the “acidic pocket”. Binding to this site can be responsible for the potentiating effectCitation36 since a positively charged group of the amiloride molecule can directly interact with the negatively charged groups in the acidic pocket. Thus, the charged group of an organic ligand can substitute one of the free protons required for activation. Another X-ray structure demonstrates that psalmotoxin also binds in the vicinity of the acidic pocket and that the positively charged groups of this peptide do interact with the negatively charged groups in this site.Citation37 Thus, it appears that the specific pattern of drug interactions with components of the acidic pocket can determine the mode of drug action on ASIC activation and desensitization properties. We have previously shown that synthetic monoamines IEM-1921 and IEM-2117 can potentiate currents both through the native hippocampal ASICs and the homomeric ASIC2a channels.Citation26 The most plausible structural explanation of the difference in the action of IEM-1921 and IEM-2117 and the compounds studied in this work lies in the different distance between the hydrophobic moiety and the amino group. In contrast to IEM-1921 and IEM-2117, in tryptamine, tyramine, and histamine the amino group is connected to the hydrophobic moiety with two methylene spacers. This difference can determine a different pattern of interactions with the receptor. For instance, the amino groups of short (IEM-1921 and IEM-2117) and long (histamine and IEM-2044) amines can interact with different acidic residues in the acidic pocket. It should be noted, however, that there are structuralCitation36 and mutationalCitation38 data suggesting an involvement of the other sites in ASIC activation and the binding of modulating drugs to these sites. A mutational approach is required to uncover the sites and the structural determinants of action of synthetic and endogenous amines.
Despite the fact that solid conclusion about the binding site requires mutagenesis studies, some suggestions can be made based on the available data. Sequences of rat ASICs differ in the region of acidic pocket. Particularly, ASIC1a contains His residue in position 173, whereas ASIC1b, ASIC2a and ASIC3 contain Ser, Lys and Arg in this position, correspondingly. It seems likely that ASIC1a-specific ligands interact with the ASIC1a-specific residues. Modeling calculations were performed for the rat ASIC1a using the ZMM program package.Citation39 We found that aromatic moiety of histamine and related amines can bind to histidine in position 173 via the stacking mechanism. Homologous phenylalanine (Phe174 in chicken ASIC1a) strongly interacts with psalmotoxin and amiloride in available X-ray structures ( and B). From this position the amino group connected to the aromatic moiety by two spacers can reach several acidic residues ( and D) including ASIC1a-specific Asp349 (ASIC2a contains Gly residue in this position). Thus, available data on amino acid sequences and spatial structure support the hypothesis that amine-containing ASIC ligands can bind at the acidic pocket region.
Figure 6. Possible binding of amines at the acidic pocket of ASIC1a. A and B, X-ray structures show interactions of Phe174 with psalmotoxin (A, PDB code 4fz0) and amiloride (B, PDB code 4ntx). C and D, putative binding modes of histamine. Aromatic moiety binds to ASIC1a-specific His173, whereas aminogroup can reach Asp349 (C) or Glu242 (D).
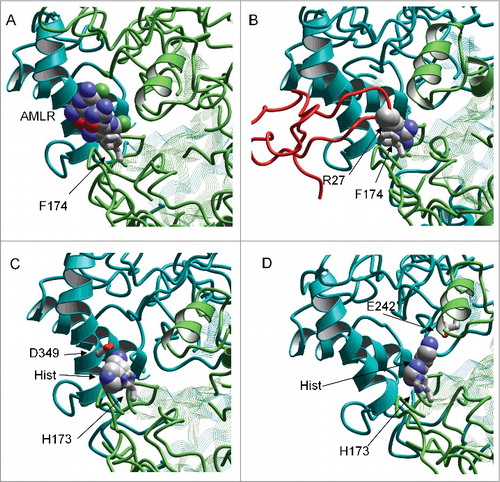
We have demonstrated in our previous studies that synthetic amines are a new class of ASIC ligands, which produce various effects depending on the chemical structure and the subunit composition of the target. Here we found that different endogenous amines also target these channels. Assuming a large diversity of endogenous amines we can suspect that some members of this class can control ASIC activity in physiologically relevant concentrations. It is also important that a number of medically used drugs belong to the same class of amine-containing compounds. Many antidepressants and antihistamine drugs, in particular, should be considered as putative ASIC ligands.
Materials and methods
Native receptors
Experiments were conducted in agreement with the Rules of Animal Care and Use Committee of the Sechenov Institute of Evolutionary Physiology and Biochemistry (IEPHB) of the Russian Academy of Sciences, which is fully compatible with European Community Council directives 86/609/EEC. Outbred male Wistar rats 12–17 days old and 25–35g were obtained from a local (IEPHB) animal facility. Rats were anesthetized with urethane and then decapitated. Maximum efforts were made to minimize the number of animals used and to minimize discomfort.
Brains were removed quickly and cooled to 2–4 °C in an ice bath. Transverse hippocampal slices (250 μm thick) were prepared using a vibratome (Campden Instruments Ltd., Loughborough, UK) and stored in a solution containing (in mM): NaCl 124, KCl 5, CaCl2 1.3, MgCl2 2.0, NaHCO3 26, NaH2PO4 1.24 and D-glucose 10, aerated with carbogen (95% O2, 5%CO2). Typically, 6–7 slices were obtained from 1 rat brain.
The neurons were isolated from slices with vibrodissociation.Citation40 The method allows a cell to be isolated from a local part of the slice under visual control using an inverted microscope. The experiments were carried out on hippocampal interneurons isolated from the lacunosum moleculare and radiatum layers of the CA1 region. Interneurons were identified by morphological and electrical characteristics. The neurons were discriminated from glial cells by the appearance of action potentials in response to depolarizing pulses under the current clamp. Pyramidal neurons usually have pyramidal-like somata and preserved apical and basal (in some neurons) dendrites, the interneurons varied in size and shape; however, most were round to oval. Extracellular acidification at -80mV holding voltage caused larger (up to 2–3 nA) response in interneurons, whereas response of pyramidal neurons was about 0.2 nA only.
The whole-cell patch clamp technique was used to record the membrane currents generated in response to fast acidification. Series resistance of about 20 MΩ was compensated by 70–80% and monitored during the experiments. Currents were recorded using an EPC-8 amplifier (HEKA Electronics, Lambrecht, Germany), filtered at 5 kHz, sampled and stored on a personal computer. Drugs were applied using the RSC-200 perfusion system (BioLogic Science Instruments, Claix, France) under computer control. The extracellular solution contained the following (in mM): NaCl 143, KCl 5, MgCl2 2.0, CaCl2 2.5, D-glucose 18, HEPES 10 and MES 10 (pH was adjusted to 7.35 with HCl). The pipette solution contained the following (in mM): CsF 100, CsCl 40, NaCl 5, CaCl2 0.5, EGTA 5 and HEPES 10 (pH was adjusted to 7.35 with CsOH). All experiments were performed at room temperature (22–24 °C).
Recombinant receptors
CHO cells were cultured at 37°C in a humidified atmosphere of 5% CO2. Cells were maintained with standard culture conditions (Dulbecco's modified Eagle's medium DMEM + 10% fetal bovine serum + 5% streptomycin/penicillin). Plasmids encoding ASIC subunits were transfected using Lipofectamine 2000 (Invitrogen, USA) following the manufacturer's protocol. Expression vectors encoding rat ASIC1a and ASIC2a were a kind gift from Dr. A. Staruschenko. For the expression of the homomeric channels, cells were transfected with 0.5 μg rASIC1a or rASIC2a cDNA per 35 mm2 dish together with 0.5 μg eGFP. Electrophysiological experiments were performed 48–72 h after transfection. Transfected cells were identified with green fluorescence using the Leica DMIL microscope.
Whole-cell currents were recorded in the voltage-clamp mode. The pipette solution contained the following (in mM): CsF 100, CsCl 40, NaCl 5, CaCl2 0.5, EGTA 5 and HEPES 10 (pH was adjusted to 7.35 with CsOH). Cells were continuously superfused with an extracellular solution containing the following (in mM): NaCl 150, CaCl2 1, MgCl2 2, HEPES 10 and MES 10, adjusted to pH 7.35. Current recordings were acquired with an EPC10-USB (HEKA Elektronik, Lambrecht, Germany) patch clamp amplifier connected to a computer running Patchmaster software (HEKA Elektronik, Lambrecht, Germany). Only recordings where access resistance and capacitance changed <10% over the course of the experiment were used.
Data analysis and statistics
All values are presented as mean ± standard deviation (SD) from at least five experiments. Significance of the effects was tested with paired t-test with p = 0.05 (drug versus control).
Disclosure of potential conflicts of interest
The authors declare that they have no conflict of interest.
Funding
This work was supported by the Russian Science Foundation grant 16-14-00122.
References
- Zhou RP., Wu XS., Wang ZS., Xie YY., Ge JF., Chen FH. Novel insights into acid-sensing ion channels: implications for degenerative diseases. Aging Dis. 2016;7:491–501. doi:10.14336/AD.2015.1213. PMID:27493834.
- Dussor G. ASICs as therapeutic targets for migraine. Neuropharmacology. 2015;94:64–71. doi:10.1016/j.neuropharm.2014.12.015. PMID:25582295.
- Chu XP., Xiong ZG. Acid-Sensing Ion Channels in Pathological Conditions. Adv Exp Med Biol 2013; 961:419–31. doi:10.1007/978-1-4614-4756-6_36. PMID:23224900.
- Wemmie JA., Chen JG., Askwith CC., Hruska-Hageman AM., Price MP., Nolan BC., Yoder PG., Lamani E., Hoshi T., Freeman JH.Jr, et al. The acid-activated ion channel ASIC contributes to synaptic plasticity, learning, and memory. Neuron. 2002;34:463–77. doi:10.1016/S0896-6273(02)00661-X. PMID:11988176.
- Du JY., Reznikov LR., Price MP., Zha XM., Lu Y., Moninger TO., Wemmie JA., Welsh MJ. Protons are a neurotransmitter that regulates synaptic plasticity in the lateral amygdala. P Natl Acad Sci USA. 2014;111:8961–6. doi:10.1073/pnas.1407018111.
- Kreple CJ., Lu Y., Taughert RJ., Schwager-Gutman AL., Du JY., Stump M, Wang Y., Ghobbeh A., Fan R., Cosme CV, et al. Acid-sensing ion channels contribute to synaptic transmission and inhibit cocaine-evoked plasticity. Nat Neurosci. 2014;17:1083–91. doi:10.1038/nn.3750. PMID:24952644.
- Gonzalez-Inchauspe C., Urbano FJ., Di Guilmi MN., Uchitel OD. Acid-sensing ion channels activated by evoked released protons modulate synaptic transmission at the mouse calyx of held synapse. J Neurosci. 2017;37:2589–99. doi:10.1523/JNEUROSCI.2566-16.2017. PMID:28159907.
- Baron A., Lingueglia E. Pharmacology of acid-sensing ion channels – Physiological and therapeutical perspectives. Neuropharmacology. 2015;94:19–35. doi:10.1016/j.neuropharm.2015.01.005. PMID:25613302.
- Paukert M., Chen XM., Polleichtner G., Schindelin H., Grunder S. Candidate amino acids involved in H+ gating of acid-sensing ion channel 1a. J Biol Chem. 2008;283:572–81. doi:10.1074/jbc.M706811200. PMID:17981796.
- Paukert M., Babini E., Pusch M., Grunder S. Identification of the Ca2+ blocking site of acid-sensing ion channel (ASIC) 1: Implications for channel gating. J Gen Physiol. 2004;124:383–94. doi:10.1085/jgp.200308973. PMID:15452199.
- Wang W., Duan B., Xu H., Xu L., Xu TL. Calcium-permeable acid-sensing ion channel is a molecular target of the neurotoxic metal ion lead. J Biol Chem. 2006;281:2497–505. doi:10.1074/jbc.M507123200. PMID:16319075.
- Wang W., Yu Y., Xu TL. Modulation of acid-sensing ion channels by Cu2+ in cultured hypothalamic neurons of the rat. Neuroscience. 2007;145:631–41. doi:10.1016/j.neuroscience.2006.12.009. PMID:17224241.
- Li WG., Yu Y., Zhang ZD., Cao H., Xu TL. ASIC3 Channels integrate agmatine and multiple inflammatory signals through the nonproton ligand sensing domain. Mol Pain. 2010;6:88. doi:10.1186/1744-8069-6-88.
- Duan B., Wang YZ., Yang T., Chu XP., Yu Y., Huang Y, Cao H., Hansen J., Simon RP., Zhu MX, et al. Extracellular spermine exacerbates ischemic neuronal injury through sensitization of ASIC1a channels to extracellular acidosis. J Neurosci. 2011;31:2101–12. doi:10.1523/JNEUROSCI.4351-10.2011. PMID:21307247.
- Babini E., Paukert M., Geisler HS., Grunder S. Alternative splicing and interaction with di- and polyvalent cations control the dynamic range of acid-sensing ion channel 1 (ASIC1). J Biol Chem. 2002;277:41597–603. doi:10.1074/jbc.M205877200. PMID:12198124.
- Askwith CC., Cheng C., Ikuma M., Benson C., Price MP., Welsh MJ. Neuropeptide FF and FMRFamide potentiate acid-evoked currents from sensory neurons and proton-gated DEG/ENaC channels. Neuron. 2000;26:133–41. doi:10.1016/S0896-6273(00)81144-7. PMID:10798398.
- Catarsi S., Babinski K., Seguela P. Selective modulation of heteromeric ASIC proton-gated channels by neuropeptide FF. Neuropharmacology. 2001;41:592–600. doi:10.1016/S0028-3908(01)00107-1. PMID:11587714.
- Deval E., Baron A., Lingueglia E., Mazarguil H., Zajac JM., Lazdunski M. Effects of neuropeptide SF and related peptides on acid sensing ion channel 3 and sensory neuron excitability. Neuropharmacology. 2003;44:662–71. doi:10.1016/S0028-3908(03)00047-9. PMID:12668052.
- Sherwood TW., Askwith CC. Endogenous arginine-phenylalanine-amide-related peptides alter steady-state desensitization of ASIC1a. J Biol Chem. 2008;283:1818–30. doi:10.1074/jbc.M705118200. PMID:17984098.
- Allen NJ., Attwell D. Modulation of ASIC channels in rat cerebellar Purkinje neurons by ischaemia-related signals. J Physiol-London. 2002;543:521–9. doi:10.1113/jphysiol.2002.020297. PMID:12205186.
- Deval E., Noel J., Lay N., Alloui A., Diochot S., Friend V, Jodar M., Lazdunski M., Lingueglia E. ASIC3, a sensor of acidic and primary inflammatory pain. Embo J. 2008;27:3047–55. doi:10.1038/emboj.2008.213. PMID:18923424.
- Smith ES., Cadiou H., McNaughton PA. Arachidonic acid potentiates acid-sensing ion channels in rat sensory neurons by a direct action. Neuroscience. 2007;145:686–98. doi:10.1016/j.neuroscience.2006.12.024. PMID:17258862.
- Cadiou H., Studer M., Jones NG., Smith ESJ., Ballard A., McMahon SB, McNaughton PA. Modulation of acid-sensing ion channel activity by nitric oxide. J Neurosci. 2007;27:13251–60. doi:10.1523/JNEUROSCI.2135-07.2007. PMID:18045919.
- Jiang Q., Li MH., Papasian CJ., Branigan D., Xiong ZG., Wang JQ, Chu XP. Characterization of Acid-Sensing Ion Channels in Medium Spiny Neurons of Mouse Striatum. Neuroscience. 2009;162:55–66. doi:10.1016/j.neuroscience.2009.04.029. PMID:19376200.
- Kusama N., Gautam M., Harding AMS., Snyder PM., Benson CJ. Acid-sensing ion channels (ASICs) are differentially modulated by anions dependent on their subunit composition. Am J Physiol-Cell Ph. 2013;304:C89–C101. doi:10.1152/ajpcell.00216.2012.
- Tikhonova TB., Nagaeva EI., Barygin OI., Potapieva NN., Bolshakov KV., Tikhonov DB. Monoamine NMDA receptor channel blockers inhibit and potentiate native and recombinant proton-gated ion channels. Neuropharmacology. 2015;89:1–10. doi:10.1016/j.neuropharm.2014.08.018. PMID:25196733.
- Nagaeva EI., Potapieva NN., Tikhonov DB. The effect of hydrophobic monoamines on acid-sensing ion channels ASIC1B. Acta Naturae. 2015;7:95–101. PMID:26085950.
- Nagaeva EI., Potapieva NN., Nikolaev MV., Gmiro VE., Magazanik LG., Tikhonov DB. Determinants of action of hydrophobic amines on ASIC1a and ASIC2a. Eur J Pharmacol. 2016;788:75–83. doi:10.1016/j.ejphar.2016.06.013. PMID:27288880.
- Nagaeva EI., Tikhonova TB., Magazanik LG., Tikhonov DB. Histamine selectively potentiates acid-sensing ion channel 1a. Neurosci Lett. 2016;632:136–40. doi:10.1016/j.neulet.2016.08.047. PMID:27574729.
- Bolshakov KV., Essin KV., Buldakova SL., Dorofeeva NA., Skatchkov SN., Eaton MJ, Tikhonov DB., Magazanik LG. Characterization of acid-sensitive ion channels in freshly isolated rat brain neurons. Neuroscience 2002; 110:723–30. doi:10.1016/S0306-4522(01)00582-6. PMID:11934479.
- Weng JY., Lin YC., Lien CC. Cell type-specific expression of acid-sensing ion channels in hippocampal interneurons. J Neurosci. 2010;30:6548–58. doi:10.1523/JNEUROSCI.0582-10.2010. PMID:20463218.
- Woodhull AM. Ionic blockage of sodium channels in nerve. J Gen Physiol. 1973;61:687–708. doi:10.1085/jgp.61.6.687. PMID:4541078.
- Hesselager M., Timmermann DB., Ahring PK. pH dependency and desensitization kinetics of heterologously expressed combinations of acid-sensing ion channel subunits. J Biol Chem. 2004;279:11006–11015. doi:10.1074/jbc.M313507200. PMID:14701823.
- Adams CM., Snyder PM., Welsh MJ. Paradoxical stimulation of a DEG ENaC channel by amiloride. J Biol Chem. 1999;274:15500–4. doi:10.1074/jbc.274.22.15500. PMID:10336442.
- Yagi J., Wenk HN., Naves LA., McCleskey EW. Sustained currents through ASIC3 ion channels at the modest pH changes that occur during myocardial ischemia. Circ Res. 2006;99:501–9. doi:10.1161/01.RES.0000238388.79295.4c. PMID:16873722.
- Baconguis I., Bohlen CJ., Goehring A., Julius D., Gouaux E. X-Ray Structure of Acid-Sensing Ion Channel 1-Snake Toxin Complex Reveals Open State of a Na+-Selective Channel. Cell. 2014;156:717–29. doi:10.1016/j.cell.2014.01.011. PMID:24507937.
- Baconguis I., Gouaux E. Structural plasticity and dynamic selectivity of acid-sensing ion channel-spider toxin complexes. Nature. 2012;489:400–5. doi:10.1038/nature11375. PMID:22842900.
- Yu Y., Chen Z., Li WG., Cao H., Feng EG., Yu F, Liu H., Jiang H., Xu TL. A nonproton ligand sensor in the acid-sensing ion channel. Neuron. 2010;68:61–72. doi:10.1016/j.neuron.2010.09.001. PMID:20920791.
- Garden DP., Zhorov BS. Docking flexible ligands in proteins with a solvent exposure- and distance-dependent dielectric function. J Comput Aid Mol Des. 2010;24:91–105. doi:10.1007/s10822-009-9317-9.
- Vorobjev VS. Vibrodissociation of Sliced Mammalian Nervous-Tissue. J Neurosci Meth. 1991;38:145–50. doi:10.1016/0165-0270(91)90164-U.