ABSTRACT
Lineage tracing studies have revealed that transcription factors play a cardinal role in pancreatic development, differentiation and function. Three transitions define pancreatic organogenesis, differentiation and maturation. In the primary transition, when pancreatic organogenesis is initiated, there is active proliferation of pancreatic progenitor cells. During the secondary transition, defined by differentiation, there is growth, branching, differentiation and pancreatic cell lineage allocation. The tertiary transition is characterized by differentiated pancreatic cells that undergo further remodeling, including apoptosis, replication and neogenesis thereby establishing a mature organ. Transcription factors function at multiple levels and may regulate one another and auto-regulate. The interaction between extrinsic signals from non-pancreatic tissues and intrinsic transcription factors form a complex gene regulatory network ultimately culminating in the different cell lineages and tissue types in the developing pancreas. Mutations in these transcription factors clinically manifest as subtypes of diabetes mellitus. Current treatment for diabetes is not curative and thus, developmental biologists and stem cell researchers are utilizing knowledge of normal pancreatic development to explore novel therapeutic alternatives. This review summarizes current knowledge of transcription factors involved in pancreatic development and β-cell differentiation in rodents.
Introduction
The mature pancreas is a mixed micro-organ comprising 3 major components: the exocrine, ductal and endocrine portions. The exocrine compartment is defined by acinar cells that produce digestive enzymes (e.g., lipases, proteases and nucleases) and is closely associated with ductal cells that secrete ions directed to the intestine by a branched ductal system. The endocrine pancreas is organized into functional units termed the islets of Langerhans with cells that produce the hormones glucagon (α-cells), insulin (β-cells), somatostatin (δ-cells), pancreatic polypeptide (PP-cells) and ghrelin (ϵ-cells).Citation1 The mature pancreas is mainly constituted by acinar cells (~98%) with islet cells (~2%) interspersed throughout the central regions of the organ. In mammals, each islet is a micro-organ with distinct cells (i.e., 20–30% α-cells, ˜60% β-cells, ˜10% δ-cells, <5% PP-cells and ˜1% ϵ-cells) localized in the pancreas.Citation2 The anatomical arrangement of islet cells varies between species. Diabetes mellitus is marked by persistent hyperglycemia concomitant with altered carbohydrate, protein and fat metabolism. Type 1 diabetes (T1D) is an autoimmune disease induced by a combination of genetic and environmental stimuli leading to lymphocytic infiltration of islets and β-cell loss which results in absolute insulin deficiency. Type 2 diabetes (T2D) is caused by peripheral insulin resistance, impaired insulin secretion from β-cells, a deficiency in suppression of glucagon production and excess hepatic gluconeogenesis.Citation3
In T1D, the administration of exogenous insulin via injection or pump remains the primary treatment. Therefore alternative therapeutic options such as transplantation of whole-organ pancreata or isolated isletsCitation4,5 are being explored to limit insulin dependence. Both transplantation strategies are challenged by a shortage of donors to meet clinical demand and bear the risk of an immune response and organ rejection. Hence, alternate strategies to generate β-cells are under investigation. Currently, potential sources for generating β-cells are 1) human embryonic stem cells (hESCs),Citation6,7 2) other endodermal tissue specifically the liver,Citation8 3) existing β-cells by inducing proliferation and 4) reprogramming of other pancreatic exocrine and endocrine cells.Citation9,10 Recent examples of lineage reprogramming include the conversion of acinar cells,Citation11 α-cellsCitation12 and gut cellsCitation13 to β-cells. Although considerable research has been dedicated to seeking therapeutic alternatives, generating fully functional insulin-producing β-cells, remains elusive. Thus, a greater understanding of pancreatic organogenesis, differentiation and maturation is warranted. Transcription factors are critical in pancreatic organogenesis, differentiation and maintenance. This review therefore summarizes the current knowledge of transcription factors that govern development of the pancreas into a functional organ.
Overview of pancreatic development
In the developing mouse embryo, gastrulation (a process in which a single layered blastula is reorganized into a trilaminar structure/gastrula) at embryonic day (e) 7.5 leads to the generation of 3 germ layers, specifically the ectoderm, mesoderm and endoderm.Citation14 Pancreatic development has been characterized as a series of bifurcating lineage decisions: endoderm vs. mesoderm and ectoderm; pancreas vs. duodenum; exocrine vs. endocrine; and β-cell vs. other hormone-positive cell types.Citation15 At e9.5, the pancreas originates from the foregut endoderm as 2 independent pancreatic buds, along the dorsal and ventral axis.Citation16 The dorsal bud is first detected at e9.0 while its complement, the ventral bud, develops at e9.5.Citation16-18 Interestingly, this distinction arises due to diverse signals from neighboring mesoderm-derived tissues. The dorsal bud develops adjacent to the notochord and the splanchnic mesenchyme which later constitutes the dorsal aortae.Citation19 The complex signaling networks involved in the formation of the dorsal bud include activin, fibroblast growth factor (FGF), transforming growth factor-β (TGFβ), retinoic acid, vascular endothelial growth factor (VEGF), bone morphogenetic protein (BMP) inhibitors and hedgehog-type ligands.Citation19 Initially, the ventral bud evolves as 2 independent endodermal regions that grow adjacent to the liver and the bile duct epithelium and unifies at the time of the gut tube closing.Citation19 The ventral bud connects with the cardiac mesenchyme and the septum transversumCitation20,21 and intercellular signaling through TGFβ, Notch, FGF, Wnt and Hedgehog signaling; which are critical for pancreatic development.Citation19 The dorsal bud produces more endocrine cells than the ventral bud.Citation17 and further, these buds differ in their expression of transcription factors implicated in development.Citation20 Pancreatic organogenesis, differentiation and maturation is classified into 3 stages: the primary (e8.5–12.5), secondary (e12.5–16.5) and tertiary (e16.5-postnatal) transitions.Citation16
The primary transition (e8.5–12.5)
The primary transition is a period of active proliferation of pancreatic progenitor cells which form a stratified epithelium accompanied by the formation of the multiple microlumen that later fuse.Citation16 Glucagon+, insulin+ and double-positive cells emerge from e9.0–13.5 in the dorsal budCitation16,22-25 although these early cells do not commit to mature islets.Citation16,23 Further studies have revealed that these early endocrine cells form independently of transcription factors essential for early pancreatic development.Citation26-29 Additionally, glucagon, insulin, somatostatin, pancreatic polypeptide and ghrelin are expressed at transcriptionalCitation30 and protein levels.Citation31 However, glucagon+, insulin+ and glucagon+/insulin+ cells remain the main cell subtype during the primary transition.Citation23 Gut rotation occurs which merges the 2 buds for fusion around e12–13.Citation16,32 At e12.5, the pancreatic epithelium evaginates into the neighboring mesenchyme and initiates compartmentalization into tip and trunk domains. Multipotent progenitor cells (MPCs) dominate the tip domain whereas endocrine/duct bi-potent precursors constitute the trunk domain.Citation33 This model reaffirms that the final size of the mature pancreas is determined by the number of progenitor cells assigned to the pancreas primordium between e8.5–12.5.Citation34
The secondary transition (e12.5–16.5)
During the secondary transition, the pancreatic epithelium undergoes growth, branching, differentiation and pancreatic cell lineage allocation.Citation16,38 By e13.5, MPCs located at the tip of the branching epithelium may self-renew or differentiate into acinar progenitor cells; after e13.0 these cells become restricted to the exocrine cell fate.Citation33,35 Between e13–15, fully differentiated β- and α-cells arise from the epithelial trunk.Citation36 Early in development, the acinar and duct cells share some common transcription factors; however, later these cells acquire their own unique set of transcription factors to orchestrate their differentiation and maturation.Citation37 Somatostatin-expressing δ-cells emerge within the next day and between e14–18 endocrine cells organize into small aggregates.Citation16 Thus there is an increase in endocrine and exocrine gene expression that coincides with a marked increase in cellular mass.
The tertiary transition (e16.5-Postnatal)
During the tertiary transition, differentiated pancreatic cells undergo additional remodelling and maturation.Citation16,38 From e18–18.5, differentiated PP-expressing cells emerge and persist into adulthood. The individual differentiated endocrine cells delaminate, migrate into the neighboring exocrine tissue and assemble into cell clusters to initiate the formation of mature islets.Citation15,39 In the mouse, β-cells form a central islet core with α-, δ- and PP-cells at the periphery and a small proportion of ϵ-cells scattered throughout the islet.Citation40,41
Postnatal life
At birth, about 80% of the β-cell mass originates from the neogenesis of undifferentiated precursor cells and the remaining 20% arise from β-cell proliferation.Citation42 β-cell proliferation gradually declines from the first 4 weeks postnatally to after weaning.Citation42-44 Thereafter islet cell mass is maintained through self-replication. Thus, the adult β-cell mass is not maintained through neogenesisCitation7 but is capable of limited proliferation.Citation45 In rodents aged 30–40 days, the rate of β-cell replication is steady.Citation46 Under normal conditions, the adult β-cell has a long life span but proliferation decreases with age.Citation47-49 During adulthood, islet mass is unaltered, except in response to physiological (e.g., pregnancy) and pathological (e.g., obesity and insulin resistance) states.Citation50,51 Acinar differentiation, maturation and proliferation also continue after birth and dissipate until weaning.Citation52
Transcription factors regulating pancreatic organogenesis
Pancreatic transcription factors are key factors that govern pancreatic organogenesis, differentiation and maturation. Pancreatic development is a dynamic process controlled by extrinsic signals from non-pancreatic tissues and intrinsic transcription factors.Citation53 Lineage-tracing studies and gain/loss-of-function analyses provided further insight into the molecular mechanisms involved in pancreatic development and pancreatic cell fate determination ().Citation20,32,54,55 Importantly, transcription factors are implicated at multiple levels of pancreatic development that include 1) the initiation of transcription, 2) organogenesis, 3) control mechanisms for cell differentiation and 4) maintenance of the functional cell phenotype ().Citation53
Table 1. tiTranscription factors regulating the pancreatic transitions.
Figure 1. Transcription factors regulating pancreatic organogenesis, differentiation and maturation. Key pancreatic transcription factors, in concert with extrinsic signals from non-pancreatic organs, form an intricate regulatory network orchestrating pancreatic development. Pancreatic development is classified into 3 different stages: the primary, secondary and tertiary transitions. In mice, at e7.5 prior to the primary transition (e8.5–12.5), the formation of the pancreatic endoderm is initiated and pre-differentiated cells shift to proto-differentiated cells. Several transcription factors involved in early pancreatic development are also observed in later transitions. During the secondary transition (e12.5–16.5), proto-differentiated tissue yield fully differentiated cells. A critical regulatory system, involving Sox9, Notch signaling, Hes1 and Ngn3, is required for exocrine and endocrine progenitor cell differentiation. Subsequently, endocrine precursors are further differentiated via the antagonistic relationship between Pax4 and Arx. There are several dynamic interrelationships between transcription factors that lead to cell lineage decisions. Finally, during the tertiary transition (e16.5-postnatal), differentiated endocrine cells organize into cell aggregates to undergo further maturation postnatally. These specialized islet cells are plastic during early neonatal life; throughout life they are dynamic and can compensate in response to fluctuating metabolic demand; and with aging their proliferative and compensatory abilities diminish. These specific transcription factors are thus integral for pancreatic development, cellular differentiation and maturation into a functional organ.
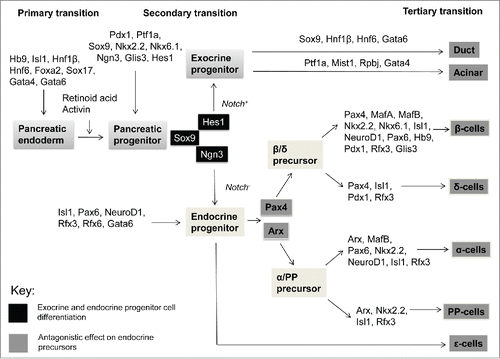
Transcription factors involved in the primary transition (e8.5–12.5)
Homeobox gene product 9 (Hb9)
Hb9/Hlxb9 (also known as the motor neuron and pancreas homeobox protein, Mnx1), is expressed around e8.0 within the notochord and in the dorsal and ventral pancreatic buds during pancreatic development.Citation56,57 At e9.5, graded expression of Hb9 was observed along the dorsal-ventral axis.Citation58 Hb9 expression decreases at e12.5 and is subsequently confined to adult β-cells.Citation56 Hb9−/− mice showed dorsal bud agenesis and the ventral bud had small islets with few β-cells ().Citation56,57 Upon closer examination, the expression of Pdx1, Nkx6.1 and Glut2 in Hb9−/− β-cells was impaired.Citation57 The over-expression of Hb9 beyond e9–10 in Pdx1 promoter-driven transgenic mice impaired pancreatic development.Citation59 Additionally, endocrine and exocrine cell differentiation was hindered and the pancreatic mesenchyme adopted a stomach/intestinal mesenchyme identity.Citation59 Further research is warranted to explain the varied expression of Hb9 in both pancreatic buds and in the different stages of pancreatic development.
Islet 1 (Isl1)
The LIM homeodomain protein, Isl1 is expressed in the dorsal bud, the mesenchyme surrounding the dorsal bud, in post-mitotic islet cells and all adult islet cells.Citation26,60 Isl1 knock-out mice embryos revealed that expression of Isl1 in the dorsal mesenchyme and endoderm is critical for the formation of the dorsal bud and dorsal exocrine cell differentiation and differentiation of all endocrine cells respectively ().Citation26 The combination of Foxa1 and Foxa2 (also known as hepatocyte nuclear factor (Hnf)) were shown to regulate Isl1 gene expression.Citation61
Hepatocyte nuclear factor (Hnf) family of transcription factors
Several Hnf members have been implicated in the formation of the foregut endoderm from which the pancreas arises including Hnf1β, Hnf3β (hereafter called Foxa2) and Hnf6 (also called Onecut-1).Citation62-65 At e9.5, Hnf1β mutant mice lacked the ventral bud but a transient dorsal bud was present with temporal expression of Pdx1 and Hb9 ().Citation66 Later by e13.5, pancreatic agenesis presented with a phenotype similar to Ptf1a deficiency.Citation66 Additionally, Hnf1β binding sites were identified on the Ptf1a promoter, suggesting a direct regulatory relationship.Citation66 Between e11.5–13.5, Hnf1β+cells in the trunk compartment were precursors of acinar, duct and endocrine cells.Citation67 By e13.5–16.5, Hnf1β+cells formed the embryonic duct epithelium and generated both ductal and endocrine cell lineages; later, Hnf1β expression was confined to ductal cells.Citation67
Hnf6 is expressed in the foregut-midgut region of the endodermCitation65,68 and pancreatic epithelium;Citation65 later in fetal life, Hnf6 is localized in ductal and acinar cells ().Citation65,68 Additionally, Hnf6 has been shown to regulate Hnf3β,Citation65,68 Pdx1 promoter regulatory regions (i.e., Areas I-III),Citation69 and is an upstream activator of Ngn3.Citation70-72 Hnf6−/− mice had islets with disrupted architecture attributed to near total loss in Ngn3 expression.Citation72 In addition, Hnf6−/− mice developed cysts in inter- and intralobular ducts.Citation73 Further, 2 binding sites for Hnf6 were located in the distal region of the Ngn3 gene.Citation72 Recently, Hnf6 was identified as a negative regulator of MafA.Citation74 Cre-mediated conditional gene inactivation confirmed that Hnf6 functions during early and late pancreatic development and is required for maintenance of Ngn3 expression and pancreatic duct morphology.Citation75 Overexpression of Hnf6 in transgenic mice leads to hyperplastic islets near the pancreatic ducts with disrupted spatial organization of endocrine cell types and a lack of Glut2 in β-cells.Citation76
The winged helix/forkhead members, Foxa1 and Foxa2, are expressed in the foregut endoderm prior to pancreatic development Citation63,64 and persist in all islet and acinar cells into adulthood.Citation77,78 The knockout of Foxa1 and Foxa2 in mice caused reduced Pdx1 expression and extreme pancreatic hypoplasia.Citation79 The mutant mice displayed hyperglycemia and impaired acinar and islet cell content, and subsequently died ().Citation79 Foxa1 and Foxa2 bind to the distal Pdx1 enhancer.Citation79 Endoderm-specific ablation of Foxa2 in mice induced extreme hypoglycemia and early death ().Citation80 Further, the differentiation of α-cells was impaired; however, the expression of the key α-cell transcription factors Arx, Pax6 and Brn4 was unaltered by Foxa2 ablation.Citation80
Sex determining region Y box 17 (Sox 17)
Sox17 is a Sry-related HMG box factor that regulates endoderm development () in concert with Foxa1 and Foxa2.Citation81 Sox17 is a common progenitor in the biliary system and ventral pancreas ().Citation82 Additionally, Sox17 regulates the segregation of the biliary system, liver and pancreas.Citation82 Down-regulation of Pdx1 expressing cells is critical for normal pancreatic development.Citation82 Sox17 and Hes1 may operate in a feedback loop to separate the biliary and pancreatic lineages.Citation82 Sox17 has been recently implicated in the regulation of insulin trafficking and secretion in adult β-cells both in normal and diabetic states.Citation83
Pancreatic duodenal homeobox gene 1 (Pdx1)
Pdx1 (also known as Ipf1) is expressed in both the dorsal and ventral buds from e8.5 and is therefore required for pancreatic development beyond initial bud formation.Citation27,84 Early hormone producing cells, which comprise insulin+ and glucagon+/insulin+ cells, form independently of Pdx1.Citation27,85 Subsequently, all cells originating from endoderm-endocrine, exocrine and ductal cells expressed Pdx1.Citation84 Importantly, Pdx1 is co-expressed with Ptf1a in this pancreatic progenitor population.Citation28 Downstream Pdx1 expression is limited to differentiated β- and δ-cells and mature β-cells.Citation86 Further, reduced expression of Pdx1 is required for acinar cell differentiation and maturation.Citation87,88 Pdx1 deficiency leads to pancreatic agenesis due to failed growth of the pancreatic primordium (),Citation27,85,89 and mutations result in maturity onset diabetes of the young (MODY) 4,Citation90 or irreversible neonatal diabetes.Citation91
The spatiotemporal expression of Pdx1 is essential for endocrine and exocrine development.Citation92 Forced expression of Pdx1 in Ngn3+ cells altered the ratio of α- and β-cells in embryos and adults.Citation93 The Pdx1 gene has 4 highly conserved regions viz., Areas I-II-III (the proximal enhancer region)Citation94 and Area IV (the distal enhancer).Citation95 Hnf1α,Citation96 Foxa2,Citation94 Hnf6,Citation69 Pax6,Citation97 and MafACitation98 have binding sites within Areas I-II-III whereas Foxa1 and Foxa2 regulate Pdx1 expression via Area IV.Citation79 Pdx1 regulates β-cell identity by repressing the α-cell program via a shift in transcription profiles.Citation95
Pancreas specific transcription factor 1a (Ptf1a)
At e9.5, a subset of cells co-express Pdx1 and the bHLH factor Ptf1a that give rise to the pancreatic anlagen.Citation29 However, by e13.5 Ptf1a is confined to acinar progenitor cells.Citation99 Ptf1a−/− mice display an extremely hypoplastic dorsal bud with a lack of total acinar cells ().Citation28,99 Hence, Ptf1a is critical for the development of the dorsal pancreatic endoderm and exocrine gene transcription.Citation99-101 The presence of a pancreatic rudiment in both Pdx1 and Ptf1a phenotypes suggest that the pancreatic genetic program can continue in transcription factor deficiency.Citation102 The transcriptional activity of Ptf1a is dependent on a third DNA binding subunit, i.e., the recombination signal binding protein kappa J (Rbpj) region,Citation103 an effector of the Notch signaling pathway.Citation104 This Ptf1a-Rbpj complex is required for pancreatic development.Citation103 A study demonstrated that mutations in one of the motifs of Ptf1a prevents the formation of the Ptf1a-Rbpj complex and resembles the Ptf1a−/− mice pancreatic phenotype.Citation105 The Ptf1a-Rbpj complex activates the expression of Rbpjl (pancreas-restricted paralog of Rbpj), a shift in the expression of Ptf1a-Rbpj to Ptf1a-Rbpjl initiates the differentiation of acinar cells.Citation106 Further, the expression of Ptf1a is auto-regulated.Citation107 Interestingly in the developing pancreas, low expression of Ptf1a triggers endocrine cell fate whereas high expression inhibits endocrine and promotes exocrine cell fate.Citation108,109
The regulation of delta-like ligand (Dll1) expression by Ptf1a is important for Notch mediated control of early pancreas development.Citation110 Ptf1a controls Dll1 to inhibit Ngn3 expression.Citation110 Further, Ptf1a is a direct target of Hb9, Pdx1, Hnf6 and Nkx6.1.Citation111,112 Recently, a study described the expression of Ptf1a+ MPCs during pancreatic organogenesis.Citation113 Limited Ptf1a+ cells were identified during the secondary transition and later Ptf1a+ cells were confined to acinar cells.Citation113 Subsequently, pancreatic duct ligation (PDL) triggered facultative reactivation of multipotent factors that comprised Sox9 and Hnf1β in Ptf1a+ acini and reprogrammed acinar cells to duct and endocrine cells.Citation113 Upon streptozotocin (Stz) administration, acinar trans-differentiation to endocrine/β-cells was enhanced.Citation113
Sex determining region Y Box 9 (Sox 9)
The HMG box transcription factor, Sox9, is co-expressed with Pdx1 in MPCs between e9.5–12.5.Citation114 Sox9 maintains pancreatic progenitors by triggering their proliferation, survival and persistence in an undifferentiated state.Citation114 During the secondary transition, Sox9 is restricted to ductal/endocrine cells of the trunk domain and later it is maintained in ductal cells.Citation114,115 Pancreas-specific ablation of Sox9 depleted the progenitor cell pool and caused pancreatic hypoplasia ().Citation114 Additionally, Sox9 maintains MPC identity via a process associated with mesenchymal FGF signaling.Citation116 Sox9 also maintains multipotent progenitors through the regulation of Hnf1β, Hnf6 and Foxa2.Citation115 Hence, Sox9 may have a central role in the regulation of MPC formation and maintenance.
Gata binding protein 4 (Gata4) and gata binding protein 6 (Gata6)
Two zinc finger transcription factor family members, Gata4 and Gata6, have been associated with pancreatic development.Citation117-119 Both proteins are co-expressed in the early foregut endoderm, later the dorsal and ventral pancreatic bud epithelia and thereafter Gata4 expression is restricted to acinar cells and Gata6 to endocrine and ductal cells.Citation117,120-123 Gata4 and Gata6 null mice undergo early embryonic demise.Citation124-126 In mice, 2 genetic studies that inactivated Gata4 or Gata6 in embryonic MPCs using Cre/LoxP technology induced minor derangements in pancreatic cell morphology that resolved postnatally whereas the loss of both Gata4 and Gata6 led to pancreatic agenesis at birth ().Citation127,128 Further, mice with pancreatic ablation of both Gata4 alleles and one Gata6 allele had less acinar cells. Pdx1 was identified as a direct target of Gata proteins.Citation128 During early endoderm formation, Gata4 is directly regulated by Foxa2.Citation129 Recently, Gata6 was reported to complete acinar differentiation via multiple transcriptional regulators including Rbpjl and Mist1.Citation130 Additionally, Gata6 was required for the maintenance of mature acinar cells.Citation130
Transcription factors involved in the secondary and tertiary transitions
Neurogenin 3 (Ngn3)
During the secondary transition, the pro-endocrine bHLH transcription factor Ngn3 initiates the genesis of all endocrine cells in the pancreatic epithelium.Citation131-134 Ngn3 expression is observed from e9.5–15.5 but is nearly undetectable at birth.Citation131 Ngn3-deficient mice lacked endocrine cells but the exocrine and ductal portions appeared intact; shortly after birth these mice succumbed to diabetes ().Citation131 Additionally, overexpression of Ngn3 under the influence of the Pdx1 promoter caused early differentiation of MPCs and expansion of endocrine cells, mostly glucagon-producing cells.Citation70,133
The Notch signaling pathway is implicated in the segregation of cells within the trunk domain via lateral inhibition.Citation70,71 Notch signaling activates Hes1 which inhibits Ngn3 and promotes the exocrine cell lineage.Citation71 Further ablation of Dll1 or Rbpj or over-expression of Ngn3 increases endocrine differentiation.Citation70 Ptf1a controls Dll1 to suppress Ngn3 expression.Citation110 The misexpression of Notch in Pdx1+ progenitor cells arrests the differentiation of endocrine and exocrine cells and enhances the maintenance of progenitor cells.Citation135
Another important regulatory system involving Sox9, Notch signaling, hairy and enhancer of split 1 (Hes1) and Ngn3 was implicated in pancreatic endocrine differentiation ().Citation136 In this system, the gradient of Notch activity regulates the proliferation and differentiation of the pancreatic endocrine and ductal progenitors.Citation136 Within the primitive epithelium, Notch signaling activates Sox9 expression. Subsequently, Sox9 triggers the expression of Ngn3. However, Ngn3 is simultaneously adversely regulated by the Notch effector, Hes1 ().Citation70,136,137 Hence, Ngn3 expression is counter regulated by the expression of Sox9 and Hes1.Citation136
When Notch signaling is elevated, Hes1 activity impairs the activation of Ngn3 by Sox9.Citation136 During intermediate Notch activity, Hes1 expression is decreased or absent, triggering the activation of Ngn3 by Sox9.Citation136 When Sox9 activity is silenced, endocrine and ductal cells fail to develop leading to polycystic ducts that lack primary cilia.Citation136 Later, cell autonomous repression of Sox9 by Ngn3 occurs to permit endocrine cell differentiation.Citation136 If Notch activity remains elevated, endocrine progenitors will conserve Sox9 and convert to ductal progenitors.Citation136 Meanwhile Sox9 regulates Hes1 and with diminished Sox9 activity there is a decline in the expression of Hes1+ cells.Citation114
The competence of Ngn3 expression is altered with time as early Ngn3+ cells develop exclusively into α-cells, but late Ngn3+ cells develop into β-, PP- (after e11.5) and δ-cells (after e14.5).Citation133 The biphasic expression of Ngn3 in relation to the primary and secondary transitions of endocrine cell differentiation was recently described.Citation39 Additionally, the threshold of Ngn3 expression is critical for determining endocrine cell fate.Citation138 High Ngn3 protein expression levels are required to direct pancreatic cell progenitors into the endocrine cell fate whereas low levels of Ngn3 prompt the formation of acinar and duct cells.Citation138 Hence, endocrine cell fate determination is dependent on the developmental stage and dosage of Ngn3.Citation138 Interestingly, a recent study supported the hypothesis that Ngn3+ cells constitute a heterogeneous population of unipotent cells each limited to a specific endocrine lineage.Citation139 Ngn3 has been shown to either positively or negatively auto-regulate its own expression.Citation140-142 Also, Ngn3 expression activates several transcription factors including NeuroD1, Pax4, Arx, Pax6, Isl1, Nkx2.2, Nkx6.1 and Rfx6 which are involved in further differentiation and subtype specification of pancreatic endocrine hormones.Citation20,140,143-146
Ngn3 triggers the epithelial-to-mesenchymal transition (EMT), later inhibiting the expression of E-cadherin which initiates delamination of endocrine cells from the pancreatic epithelium.Citation147 At e11.5, pancreatic inhibition of Ngn3 led to a reduction in insulin+ cells whereas glucagon+ cells remained unaffected.Citation148 Also, an increase in markers of undifferentiated progenitors and embryonic ductal cells was observed.Citation148 Later in development, upon Ngn3 expression, undifferentiated progenitors and embryonic ductal cells differentiated into endocrine cells; thus endocrine-committed cells preserve their ability to differentiate into endocrine cells.Citation148 Further, Ngn3-labeled progenitors were also required for controlling the fate and morphogenesis of the pancreatic duct epithelium.Citation149
A p21 protein-activated kinase 3, Pak3, is expressed in Ngn3+ progenitors, maintained in mature hormone-expressing cells and later confined to adult islets.Citation150 Pak3 regulates 1) endocrine cell differentiation via its role in cell cycle exit and 2) glucose homeostasis in mice fed a high fat diet.Citation150 Insulinoma associated 1 (Insm1) has also been identified as a regulator of a network of genes involved in endocrine differentiation including Ngn3.Citation151
Neurogenic differentiation 1 (Neurod1)
During the secondary transition, the bHLH transcription factor, NeuroD1, is expressed in all major endocrine cell types, i.e., α-, β- and δ-cells.Citation145 NeuroD1 is detected at ˜e9.5 in a subset of pancreatic epithelial cells proximal to glucagon expression.Citation152 and at e14.5 alongside the ductal epithelium but by e17.5 its expression is confined to islets.Citation145 Also, NeuroD1 is required for β-cell maturation and maintenance of glucose-responsive β-cells.Citation153 Mice lacking NeuroD1 are diabetic due to a reduction in all endocrine subtypes and succumb perinatally ().Citation145 The pancreatic epithelium ectopic expression of either Ngn3 or NeuroD1 resulted in substantial premature differentiation of endocrine cells specifically α-cells leading to a hypoplastic pancreas ().Citation70,132 In combination with other factors, NeuroD1 can drive β-cell differentiation.Citation154,155 Additionally, the combination of NeuroD1, Pdx1 and MafA in non-β-cells induced insulin production.Citation156
Regulatory factor X 3 and 6 (Rfx3 and Rfx6)
Rfx3 and Rfx6 are members of the regulatory factor X family of winged-helix transcription factors and are implicated in islet development.Citation157-159 Rfx3 is expressed in Ngn3+ progenitors, developing and mature endocrine cells.Citation159 In perinatal Rfx3−/− mice islets, a small number of cells express insulin, glucagon and ghrelin whereas PP-producing cells increased ().Citation159 Adult Rfx3−/− mice exhibited small, disorganized islets with diminished insulin production and impaired glucose tolerance.Citation159 Thus, Rfx3 is required for the differentiation and function of mature β-cells.
Rfx6 is expressed at e7.5 in the definitive endoderm, then co-expressed with Ngn3 and Nkx2.2; after e9 it becomes restricted to the pancreatic buds and is later confined and maintained in all adult endocrine cells.Citation146,160 In Arx, Pax4 and NeuroD1 deficient mice, Rfx6 expression is unaltered therefore Rfx6 acts downstream of Ngn3 and upstream of Arx, Pax4 and NeuroD1.Citation146,160 If either Ngn3 or Rfx6 genes are knocked out, mice experience loss of the hormone producing islet cells; however, Rfx6−/− mice still have PP-cells ().Citation146 The ablation of Rfx6 in adult β-cells results in loss of functionality of mature β-cells attributed to reduced expression of glucokinase, the ATP-binding cassette subfamily C member 8/sulfonylurea receptor 1 (Abcc8/SUR1) subunit of KATP channels and voltage gated Ca2+ channels.Citation161 In humans, Rfx6 regulates insulin expression and secretion via modulation of Ca2+ channel expression.Citation162
Islet 1 (Isl1)
In pancreas-specific Isl1 deficient mice, a decline in islet cell proliferation and progressive loss of islet mass was observed ().Citation163 Additionally, MafA was shown to be a direct target of Isl1.Citation163,164 Isl1 deficient embryos displayed a diminished number of Arx+ cells, confirmed by a reduction in Arx mRNA levels; further, Isl1 activator binding sites were identified within the Arx locus.Citation165 LIM-domain-binding co-regulator, Ldb1, is a transcriptional co-regulator of α-, β- and δ-cell development and produces a phenotype similar to Isl1 conditional mutants.Citation166 Also, Ldb1 co-regulates Isl1-activated genes including MafA, Arx, Insulin and Glucagon-like peptide 1 receptor (Glp1r).Citation166
Nk class of homeodomain-encoding genes 2.2 and 6.1 (Nkx 2.2 and Nkx 6.1)
Nkx2.2 has a major role in β-cell lineage differentiation. In early pancreatic development, Nkx2.2 expression is observed from e9.5 in the dorsal pancreatic epithelium and Ngn3-expressing endocrine cells; later Nkx2.2 resides in α-, β- and PP-cell subtypes.Citation132,167 In Nkx2.2−/− embryos, δ-cells remain intact but α-, β- and PP-cells are displaced by ϵ-cells ().Citation40,167 Therefore, Nkx2.2 has been implicated in the late differentiation of β-cells and the development of α- and PP-cells. Nkx2.2 interacts with other transcription factors to regulate endocrine cell differentiation. Further, Nkx2.2 activates NeuroD1 leading to β-cell generation; however, Nkx2.2 needs to repress NeuroD1 for α-cell formation.Citation40,168 Further, concomitant inactivation of Nkx2.2 and NeuroD1 enhances α- and PP-cells and reduces the ϵ-cell number without altering β-cells.Citation169 In committed β-cell precursors, Nkx2.2 and Pax 4 control Arx activity.Citation170 Nkx2.2 also interacts with Arx in defining the PP-cell lineage.Citation171 Despite Pax4 mutant mice exhibiting a similar phenotype to Nkx2.2 mutant mice, a genetic interaction has yet to be identified.Citation40 Nkx2.2 also activates the MafACitation172 and Insulin genes.Citation173
Nkx6.1 is observed at e9.5 in both pancreatic buds until e13 and is then confined to the developing β-cells.Citation174,175 Although Nkx6.1 deficient mice do not exhibit β-cells, the other cell subtypes develop normally ().Citation175 In the β-cells, Nkx6.1 inhibits glucagon promoter activity.Citation176,177 In β-cell development, Nkx6.1 is expressed in Pdx1+ progenitors prior to Ngn3 activation.Citation178
Nkx6.2 is expressed in the endoderm domain similar to Pdx1.Citation178 In Nkx6.1 single mutant embryos, β-cell numbers were impaired; however, a further reduction in β-cells was observed in Nkx6.1/Nkx6.2 double mutants ().Citation179 Additionally, in the Nkx6.1 single and Nkx6.1/Nkx6.2 double mutant embryos the mature β-cell markers MafA and Glut2 were deficient.Citation179 Also, Nkx6.1/Nkx6.2 double mutant embryos had α-cell hypoplasia, a phenotype absent in Nkx6.1 and Nkx6.2 single mutants. Hence in Nkx6.1 deficiency, Nkx6.2 completely compensates for α-cell development.Citation179 However, Nkx6.2 only partially compensates for β-cell development.Citation179 Additionally, Myt1 was identified as a downstream target of Nkx6 genes.Citation179 Genetic gain/loss-of-function studies identified a cross-repressive interaction (before e14) between Nkx6.1/Nkx6.2 (Nkx6) and Ptf1a leading to MPCs differentiating to either the endocrine or acinar cell lineages respectively.Citation112 There is a repressive relationship between Nkx6.1 and Arx which results in either β- or α-cell lineages respectively.Citation180
Aristaless paired-class homeobox gene (Arx) and paired homeodomain factor 4 (Pax4)
An important antagonistic relationship exists between Arx and Pax4 in the specification of the endocrine precursors ().Citation181,182 Arx and Pax4 reciprocate repression via direct physical interaction with the pertinent promoter.Citation181,182 Hence, Arx mutant mice upregulate Pax4 mRNA and Pax4 mutant mice demonstrate elevated expression of Arx mRNA. Arx is expressed at around e9.5 and is confined to α- and PP-cellsCitation183 operating downstream of Ngn3.Citation182 Arx-deficient mice exhibit severe hypoglycemia, weakness and dehydration concomitant with an absolute loss of α-cells and augmentation of β- and δ-cells ().Citation181,182 The overexpression of Arx in Pdx1+ progenitor cells converted β- and δ-cell precursors to α- and PP-cell precursors with unaltered total endocrine cell number concomitant with persistent Pax4 expression.Citation54
Pax4 is expressed at e9.5 in the dorsal and ventral buds but is limited to the first and second wave β-cells; however, it is down-regulated shortly after birth and undetectable in adult islets.Citation184-186 Additionally, lineage tracing revealed that Pax4+ cells represent specified endocrine progenitors that may commit to endocrine cell fate.Citation187,188 Pax4 is expressed downstream of Ngn3 and its expression is lost in Ngn3−/− mice but not vice versa.Citation131,138 Ngn3 and Hnf1α bind to the Pax4 regulatory region and may thus activate expression of Pax4 in endocrine progenitor cells.Citation143 Pancreata from Pax4−/− embryos display normal islet morphology but are devoid of β- and δ-cells and exhibit elevated levels of α- and ϵ-cells ().Citation186 Also, Pax4 is a repressor of both ghrelin expression and Pax6-mediated glucagon expression; hence there is elevated expression of these 2 cell types in Pax4 mutants.Citation185,188,189 A loss in Pax4 expression inhibits Pdx1, Hb9 and insulin mRNA in β-cell precursors.Citation190 Ectopic expression of Pax4 and Arx prompts the formation of β- and α-cell lineage respectively.Citation54,191 In early pancreatic development, inactive forms of Pax4 and Arx are co-expressed later due to either selective conformational changes or post-translational modification, with either Pax4 or Arx dominating to prompt the allocation of their respective cell lineages.Citation181,182
Paired homeodomain factor 6 (Pax6)
Pax6 is expressed at e9.5–10.5 in a subset of cells in both dorsal and ventral pancreatic buds; later its expression is confined to cells of the endocrine lineage.Citation192,193 Despite expression in glucagon+ and insulin+ cells, Pax6 is only essential for α-cells.Citation193 Pax6 mutant mice display abnormal islet organization with marked α-cell reduction relative to other cells types.Citation194 Pax6−/− mice die shortly after birth as their islets fail to form.Citation193 Conditional inactivation of Pax6 in mice resulted in reduced glucagon+ and insulin+ cells but unaffected PP- and δ-cells with these mice failing to form islets ().Citation195 Thus, Pax6 may be critical for the full expansion of islet cells.Citation196,197 Also, Pax6 mutant mice have increased ghrelin-expressing cells (independent of cell proliferation) suggesting that Pax6 directs endocrine progenitors toward the ϵ-cell fate.Citation197
Gli-Similar 3 (Glis3)
Glis3 is a Kruppel-like zinc finger transcription factor essential for the development of β-cells.Citation198,199 Glis3 knockout mice produce pups with neonatal diabetes presenting with hyperglycemia and hypoinsulinemia that die shortly after birth ().Citation200-202 These pups have diminished β-cells. Glis3 has also been shown to regulate insulin expression in mature β-cells.Citation201,203-205 Glis3 binding sites were located near the distal promoter region of Ngn3 and Glis3 also interacts with Hnf6.Citation206 The Glis3/Hnf6 protein complex may operate in conjunction with a larger transcription factor network to regulate Ngn3 expression and thus activate endocrine cell specification.Citation206
V-Maf musculoaponeuroticfibrosarcoma oncogene family protein A and B (Mafa and Mafb)
During β-cell maturation, the bZIP family members MafA and MafB, shift from a MafB+ immature state to MafA+/MafB+ and lastly to a MafA+ mature state.Citation207,208 This process may be triggered by Pdx1 upregulation.Citation208 Both MafA and MafB developmental expression patterns are remarkably delayed relative to all other islet-enriched transcription factors: Pdx1 at e8.5; Pax6 and Ngn3 at e9.0; and Isl1 and Nkx2.2 at e9.5.Citation209 MafB mutant mice have reduced α- and β-cells although the endocrine cell number is unaltered and these mice die shortly after birth ().Citation210 Further, a comparison between wild type and MafB mutant mice revealed no difference in the endocrine cell numbers.Citation210 MafA−/− mice are viable but undergo β-cell dysfunction ultimately leading to glucose intolerance and diabetes.Citation211 Additionally, the expression of key β-cell genes including Ins1, Ins2, NeuroD1 and Glut2 was impaired. Later it was identified that MafA together with Pdx1 and NeuroD1 control the level of insulin gene expression.Citation211 Postnatally, MafA is expressed exclusively in mature β-cells and serves as a marker of terminally differentiated β-cells.Citation207 Pancreatic ablation of MafA leads to impaired β-cell mass, β-cell dysfunction and disrupted islet organization in 3-week-old mice.Citation207 Earlier in pancreatic development, Maf-regulated gene expression in β-cells was altered.Citation207 MafA is important for glucose-stimulated insulin secretion (GSIS), particularly in glucose metabolism, insulin production and insulin granule docking.Citation212 Premature induction of MafA in Ngn3+ endocrine progenitors inhibited differentiation and formation of hormone+ cells.Citation213 This effect occurred after progenitors committed to a specific endocrine cell type.Citation213 However, upon removal of MafA, these cells reverted to hormone+ cells that led to an increase in immature insulin+MafB+ cells at postnatal day (p) 5.Citation213 Thus, for normal pancreatic organogenesis, MafA expression needs to follow insulin.Citation213 Also, MafB expression in mature insulin+ cells is dependent on cell-autonomous mechanisms.Citation213
Emerging pancreatic transcription factors
In the embryonic mouse endoderm, misexpression of Ptf1a (Ptf1aEDD) expanded the pancreatic gene regulatory network.Citation214 Additionally at an early stage, pancreas-proximal organ switch occurred producing all pancreatic lineages.Citation214 The endogenous endodermal Pdx1+ domain expanded and triggered other pancreatic progenitor genes.Citation214 Thus there is a developmental window during which the endoderm can be re-specified.Citation214 Nuclear receptor subfamily 5 group A member 2 (NR5A2) is a member of the nuclear hormone receptor family and has been identified as a regulator of pancreatic organogenesis.Citation215 NR5A2 is required for the expansion of the nascent pancreatic epithelium and subsequently in the genesis of MPCs. NR5A2 deficient mice display impairment in all 3 pancreatic epithelial tissue types evident by partial loss of endocrine cells, >90% deficit of acinar cells and a disrupted ductal tree.Citation215 Additionally, NR5A2, Ptf1a and Rbpjl control additional regulatory genes including Foxa2, Gata4 and myelocytomatosis oncogene (MYC).Citation215 The novel transcription factors, Ets variant 1 (Etv1), PR domain containing 16 (Prdm16), runt-related transcription factor 1 translocated to 1 (Runx1t1) and B-cell lymphoma/leukemia 11A (Bcl11a) were identified as regulators of pancreas organogenesis.Citation216 The R spondin receptor, Lgr5, is implicated with an organoid-forming epithelial progenitor population, but little is known about this organoid-initiating epithelial progenitor population. Recently, the origin of the organoid-forming epithelial cells have been identified, i.e., MIC−1C3+/CD133+/CD26− in the adult mouse pancreas and liver.Citation217 MIC−1C3+/CD133+/CD26 cells are phenotypically similar in both organs and are rich in Sox9 and forkhead box protein J1 (FoxJ1).Citation217 Later, when organoids were transplanted to Fah−/− mice, hepatocyte-like cell grafts were generated in half of the recipients.Citation217 This demonstrated a differentiation capacity similar to hepatic organoids. Later, the adenoviral delivery of Pdx1, Ngn3 and MafA induced insulin expression.Citation217 Hence the organoid-initiating cells have the capacity to differentiate.Citation217 Wingless-type MMTV integration site family member 7b (Wnt7b) is expressed in the epithelium and is required for pancreatic progenitor cell growth.Citation218 Pancreatic ablation of Wnt7b prior to and during the secondary transition inhibited proliferation of pancreatic progenitors and prompted pancreatic hypoplasia.Citation218 Surprisingly, over-expression of Wnt7b under the Pdx1 promoter did not increase progenitor mass but instead suppressed pro-endocrine and pro-acinar cells.Citation218 This was followed by arrested morphogenesis and loss of differentiated endocrine and acinar cells leading to polycystic duct-like epithelial complexes and increased pancreatic mesenchymal mass.Citation218
Perspectives
Developmental biology has enhanced our understanding of normal pancreatic development, differentiation and function through the extensive use of rodent models. The decoding of these dynamic processes serves as a pillar for novel diabetic therapies. Pancreatic transcription factors and extrinsic signals from non-pancreatic tissue form a complex gene regulatory network that orchestrates pancreatic development. Extrinsic signals are important for gut tube patterning and these intrinsic transcription factors differentiate pancreatic progenitor cells to their specific cell lineages, viz., endocrine, exocrine and ductal. The regulatory roles of transcription factors in pancreatic development, β-cell differentiation and function are complex. Despite the extensive progress, further investigation is warranted which include defining the precise functional relationship between transcription factors and extrinsic signals and the molecular mechanisms that drive pancreatic differentiation. Collective research efforts will therefore generate a comprehensive understanding of pancreatic processes which can be translated into novel therapies.
Abbreviations
Abcc8 | = | ATP-binding cassette, sub-family C, member 8 |
Arx | = | aristaless paired-class homeobox gene |
BMP | = | bone morphogenetic protein |
Dll1 | = | delta-like ligand |
Etv1 | = | Ets variant 1 |
Foxa1 | = | forkhead box protein a 1 |
Foxa2 | = | forkhead box protein a 2 |
FoxJ1 | = | forkhead box protein J 1 |
FGF | = | fibroblast growth factor |
Gata4 | = | gata binding protein 4 |
Gata6 | = | gata binding protein 6 |
Glis3 | = | Gli-similar 3 |
Glut2 | = | glucose transporter 2 |
GSIS | = | Glucose-stimulated insulin secretion |
Hb9 | = | homeobox gene product 9 |
hESCs | = | human embryonic stem cells |
Hnf | = | hepatocyte nuclear factor |
Hnf1β | = | hepatocyte nuclear factor 1 β |
Hnf3β | = | hepatocyte nuclear factor 3 β |
Hnf6 | = | hepatocyte nuclear factor 6 |
Isl1 | = | islet 1 |
Lgr5 | = | R spondin receptor |
MafA | = | V-maf musculoaponeurotic fibrosarcoma oncogene family protein A |
MafB | = | V-maf musculoaponeurotic fibrosarcoma oncogene family protein B |
MPCs | = | multipotent progenitor cells |
MYC | = | myelocytomatosis oncogene |
NeuroD1 | = | neurogenic differentiation 1 |
Ngn3 | = | neurogenin 3 |
Nkx2.2 | = | NK class of homeodomain-encoding genes 2.2 |
Nkx 6.1 | = | NK class of homeodomain-encoding genes 6.1 |
Nkx 6.2 | = | NK class of homeodomain-encoding genes 6.2 |
NR5A2 | = | Nuclear receptor subfamily 5, group A, member 2 |
Pak3 | = | p21 protein-activated kinase 3 |
Pax4 | = | paired homeodomain factor 4 |
Pax6 | = | paired homeodomain factor 6 |
Pdx1 | = | pancreatic duodenal homeobox gene 1 |
Prdm16 | = | PR domain containing 16 |
Ptf1a | = | pancreas specific transcription factor 1 a |
Ptf1aEDD | = | misexpression of Ptf1a in the embryonic mouse endoderm |
Rbpj | = | recombination signal binding protein kappa J |
Rbpjl | = | pancreas-restricted paralog of Rbpj |
Rfx3 | = | regulatory factor x 3 |
Rfx6 | = | regulatory factor x 6 |
Runx1t1 | = | runt-related transcription factor 1 translocated to 1 |
Sox 9 | = | sex determining region Y box 9 |
Sox 17 | = | sex determining region Y box 17 |
Stz | = | streptozotocin |
SUR1 | = | sulfonylurea receptor 1 |
T1D | = | type 1 diabetes |
T2D | = | type 2 diabetes |
TGFβ | = | transforming growth factor β |
VEGF | = | vascular endothelial growth factor |
Wnt | = | wingless-type MMTV integration site family |
Wnt7b | = | wingless-type MMTV integration site family member 7b. |
Disclosure of potential conflicts of interest
No potential conflicts of interest were disclosed.
References
- Slack JM. Developmental biology of the pancreas. Development 1995; 121:1569-80; PMID:7600975
- Jones PM, Persaud SJ. Textbook of diabetes. 4th ed. UK: Blackwell Publishing Ltd; c2010. Chapter 6, Islet function and insulin secretion; 87-101
- American Diabetes Association. Diagnosis and classification of diabetes mellitus. Diabetes Care 2010; 33:S62-9; PMID:20042775; http://dx.doi.org/10.2337/dc10-S062
- Shapiro AM, Lakey JR, Ryan EA, Korbutt GS, Toth E, Warnock GL, Kneteman NM, Rajotte RV. Islet transplantation in seven patients with type 1 diabetes mellitus using a glucocorticoid-free immunosuppressive regimen. New Engl J Med 2000; 343:230-8; PMID:10911004; http://dx.doi.org/10.1056/NEJM200007273430401
- Larsen JL, Colling CW, Ratanasuwan T, Burkman TW, Lynch TG, Erickson JM, Lyden ER, Lane JT, Mack-Shipman LR. Pancreas transplantation improves vascular disease in patients with type 1 diabetes. Diabetes Care 2004; 27:1706-11; PMID:15220250; http://dx.doi.org/10.2337/diacare.27.7.1706
- D'Amour KA, Bang AG, Eliazer S, Kelly OG, Agulnick AD, Smart NG, Moorman MA, Kroon E, Carpenter MK, Baetge EE. Production of pancreatic hormone-expressing endocrine cells from human embryonic stem cells. Nat Biotechnol 2006; 24:1392-401; PMID:17053790; http://dx.doi.org/10.1038/nbt1259
- Jiang J, Au M, Lu K, Eshpeter A, Korbutt G, Fisk G, Majumdar AS. Generation of insulin-producing islet-like clusters from human embryonic stem cells. Stem Cells 2007; 25:1940-53; PMID:17510217; http://dx.doi.org/10.1634/stemcells.2006-0761
- Banga A, Akinci E, Greder LV, Dutton JR, Slack JM. In vivo reprogramming of Sox9+ cells in the liver to insulin-secreting ducts. Proc Natl Acad Sci USA 2012; 109:15336-41; http://dx.doi.org/10.1073/pnas.1201701109
- Akinci E, Banga A, Greder LV, Dutton JR, Slack JM. Reprogramming of pancreatic exocrine cells towards a β cell character using Pdx1, Ngn3 and MafA. Biochem J 2012; 442:539-50; PMID:22150363; http://dx.doi.org/10.1042/BJ20111678
- Lima MJ, Docherty HM, Chen Y, Docherty K. Efficient differentiation of AR42J cells towards insulin-producing cells using pancreatic transcription factors in combination with growth factors. Mol Cell Endocrinol 2012; 358:69-80; PMID:22429991; http://dx.doi.org/10.1016/j.mce.2012.02.024
- Zhou Q, Brown J, Kanarek A, Rajagopal J, Melton DA. In vivo reprogramming of adult pancreatic exocrine cells to β-cells. Nature 2008; 455:627-32; PMID:18754011; http://dx.doi.org/10.1038/nature07314
- Thorel F, Nepote V, Avril I, Kohno K, Desgraz R, Chera S, Herrera PL. Conversion of adult pancreatic α-cells to β-cells after extreme β-cell loss. Nature 2010; 464:1149-54; PMID:20364121; http://dx.doi.org/10.1038/nature08894
- Talchai C, Xuan S, Kitamura T, DePinho RA, Accili D. Generation of functional insulin-producing cells in the gut by Foxo1 ablation. Nature Genetics 2012; 44:406-12; PMID:22406641; http://dx.doi.org/10.1038/ng.2215
- Mfopou JK, Chen B, Sui L, Sermon K, Bouwens L. Recent advances and prospects in the differentiation of pancreatic cells from human embryonic stem cells. Diabetes 2010; 59:2094-101; PMID:20805383; http://dx.doi.org/10.2337/db10-0439
- Guney MA, Gannon M. Pancreas cell fate. Birth Defects Res C Embryo Today 2009; 87:232-48; PMID:19750517; http://dx.doi.org/10.1002/bdrc.20156
- Pictet RL, Clark WR, Williams RH, Rutter WJ. An ultrastructural analysis of the developing embryonic pancreas. Dev Biol 1972; 29:436-67; PMID:4570759; http://dx.doi.org/10.1016/0012-1606(72)90083-8
- Spooner BS, Walther BT, Rutter WJ. The development of the dorsal and ventral mammalian pancreas in vivo and in vitro. J Cell Biol 1970; 47:235-46; PMID:5513553; http://dx.doi.org/10.1083/jcb.47.1.235
- Pictet RL, Rutter WJ. Handbook of physiology. Baltimore: Williams and Wilkins; c1972. Section 7, Endocrinology, endocrine pancreas; 25-66
- Lin CL, Vuguin PM. Determinants of pancreatic islet development in mice and men: a focus on the role of transcription factors. Horm Res Paediatr 2012; 77:205-13; PMID:22487552; http://dx.doi.org/10.1159/000337219
- Oliver-Krasinski JM, Stoffers DA. On the origin of the β cell. Genes Dev 2008; 22:1998-2021; PMID:18676806; http://dx.doi.org/10.1101/gad.1670808
- Kim SK, Hebrok M. Intercellular signals regulating pancreas development and function. Genes Dev 2001; 15:111-27; PMID:11157769; http://dx.doi.org/10.1101/gad.859401
- Prasadan K, Daume E, Preuett B, Spilde T, Bhatia A, Kobayashi H, Hembree M, Manna P, Gittes GK. Glucagon is required for early insulin-positive differentiation in the developing mouse pancreas. Diabetes 2002; 51:3229-36; PMID:12401714; http://dx.doi.org/10.2337/diabetes.51.11.3229
- Herrera PL. Adult insulin- and glucagon-producing cells differentiate from two independent cell lineages. Development 2000; 127:2317-22; PMID:10804174
- Kesavan G, Sand FW, Greiner TU, Johansson JK, Kobberup S, Wu X, Brakebusch C, Semb H. Cdc42-mediated tubulogenesis controls cell specification. Cell 2009; 139:791-801; PMID:19914171; http://dx.doi.org/10.1016/j.cell.2009.08.049
- Villasenor A, Chong DC, Henkemeyer M, Cleaver O. Epithelial dynamics of pancreatic branching morphogenesis. Development 2010; 137:4295-305; PMID:21098570; http://dx.doi.org/10.1242/dev.052993
- Ahlgren U, Pfaff SL, Jessell TM, Edlund T, Edlund H. Independent requirement for ISL1 in formation of pancreatic mesenchyme and islet cells. Nature 1997; 385:257-60; PMID:9000074; http://dx.doi.org/10.1038/385257a0
- Offield MF, Jetton TL, Labosky PA, Ray M, Stein RW, Magnuson MA, Hogan BL, Wright CV. PDX-1 is required for pancreatic outgrowth and differentiation of the rostral duodenum. Development 1996; 122:983-95; PMID:8631275
- Kawaguchi Y, Cooper B, Gannon M, Ray M, MacDonald RJ, Wright CV. The role of the transcriptional regulator Ptf1a in converting intestinal to pancreatic progenitors. Nat Genet 2002; 32:128-34; PMID:12185368; http://dx.doi.org/10.1038/ng959
- Burlison JS, Long Q, Fujitani Y, Wright CV, Magnuson MA. Pdx-1 and Ptf1a concurrently determine fate specification of pancreatic multipotent progenitor cells. Dev Biol 2008; 316:74-86; PMID:18294628; http://dx.doi.org/10.1016/j.ydbio.2008.01.011
- Chiang MK, Melton DA. Single-cell transcript analysis of pancreas development. Deve Cell 2003; 4:383-93; PMID:12636919; http://dx.doi.org/10.1016/S1534-5807(03)00035-2
- Jorgensen MC, Ahnfelt-Ronne J, Hald J, Madsen OD, Serup P, Hecksher-Sorensen J. An illustrated review of early pancreas development in the mouse. Endocr Rev 2007; 28:685-705; PMID:17881611; http://dx.doi.org/10.1210/er.2007-0016
- Gittes GK. Developmental biology of the pancreas: a comprehensive review. Dev Biol 2009; 326:4-35; PMID:19013144; http://dx.doi.org/10.1016/j.ydbio.2008.10.024
- Zhou Q, Law AC, Rajagopal J, Anderson WJ, Gray PA, Melton DA. A multipotent progenitor domain guides pancreatic organogenesis. Dev Cell 2007; 13:103-14; PMID:17609113; http://dx.doi.org/10.1016/j.devcel.2007.06.001
- Stanger BZ, Tanaka AJ, Melton DA. Organ size is limited by the number of embryonic progenitor cells in the pancreas but not the liver. Nature 2007; 445:886-91; PMID:17259975; http://dx.doi.org/10.1038/nature05537
- Rieck S, Bankaitis ED, Wright CV. Lineage determinants in early endocrine development. Semin Cell Dev Biol 2012; 23:673-84; PMID:22728667; http://dx.doi.org/10.1016/j.semcdb.2012.06.005
- Ben-Othman N, Courtney M, Vieira A, Pfeifer A, Druelle N, Gjernes E, Faurite B, Avolio F, Collombat P. From pancreatic islet formation to β-cell regeneration. Diabetes Res Clin Prac 2013; 101:1-9; PMID:23380136; http://dx.doi.org/10.1016/j.diabres.2013.01.013
- Cerf ME. Islet organogenesis, angiogenesis and innervation. Cell Biol Int 2011; 35:1065-78; PMID:21999312; http://dx.doi.org/10.1042/CBI20100780
- Miller K, Kim A, Kilimnik G, Jo J, Moka U, Periwal V, Hara M. Islet formation during the neonatal development in mice. PloS One 2009; 4:e7739; PMID:19893748; http://dx.doi.org/10.1371/journal.pone.0007739
- Villasenor A, Chong DC, Cleaver O. Biphasic Ngn3 expression in the developing pancreas. Dev Dynamics 2008; 237:3270-9; PMID:18924236; http://dx.doi.org/10.1002/dvdy.21740
- Prado CL, Pugh-Bernard AE, Elghazi L, Sosa-Pineda B, Sussel L. Ghrelin cells replace insulin-producing β cells in two mouse models of pancreas development. Proc Natl Acad Sci USA 2004; 101:2924-9; http://dx.doi.org/10.1073/pnas.0308604100
- Wierup N, Svensson H, Mulder H, Sundler F. The ghrelin cell: a novel developmentally regulated islet cell in the human pancreas. Regulatory Peptides 2002; 107:63-9; PMID:12137967; http://dx.doi.org/10.1016/S0167-0115(02)00067-8
- Bouwens L, Rooman I. Regulation of pancreatic β-cell mass. Physiol Rev 2005; 85:1255-70; PMID:16183912; http://dx.doi.org/10.1152/physrev.00025.2004
- Teta M, Rankin MM, Long SY, Stein GM, Kushner JA. Growth and regeneration of adult β cells does not involve specialized progenitors. Dev Cell 2007; 12:817-26; PMID:17488631; http://dx.doi.org/10.1016/j.devcel.2007.04.011
- Rankin MM, Kushner JA. Adaptive β-cell proliferation is severely restricted with advanced age. Diabetes 2009; 58:1365-72; PMID:19265026; http://dx.doi.org/10.2337/db08-1198
- Dor Y, Brown J, Martinez OI, Melton DA. Adult pancreatic β-cells are formed by self-duplication rather than stem-cell differentiation. Nature 2004; 429:41-6; PMID:15129273; http://dx.doi.org/10.1038/nature02520
- Finegood DT, Scaglia L, Bonner-Weir S. Dynamics of β-cell mass in the growing rat pancreas. Estimation with a simple mathematical model. Diabetes 1995; 44:249-56; PMID:7883109; http://dx.doi.org/10.2337/diab.44.3.249
- Teta M, Long SY, Wartschow LM, Rankin MM, Kushner JA. Very slow turnover of β-cells in aged adult mice. Diabetes 2005; 54:2557-67; PMID:16123343; http://dx.doi.org/10.2337/diabetes.54.9.2557
- Beith JL, Alejandro EU, Johnson JD. Insulin stimulates primary β-cell proliferation via Raf-1 kinase. Endocrinol 2008; 149:2251-60; PMID:18202127; http://dx.doi.org/10.1210/en.2007-1557
- Perl S, Kushner JA, Buchholz BA, Meeker AK, Stein GM, Hsieh M, Kirby M, Pechhold S, Liu EH, Harlan DM, et al. Significant human β-cell turnover is limited to the first three decades of life as determined by in vivo thymidine analog incorporation and radiocarbon dating. J Clin Endocrinol Metab 2010; 95:E234-9; PMID:20660050; http://dx.doi.org/10.1210/jc.2010-0932
- Weir GC, Laybutt DR, Kaneto H, Bonner-Weir S, Sharma A. Beta-cell adaptation and decompensation during the progression of diabetes. Diabetes 2001; 50:S154-9; PMID:11272180; http://dx.doi.org/10.2337/diabetes.50.2007.S154
- Parsons JA, Brelje TC, Sorenson RL. Adaptation of islets of Langerhans to pregnancy: increased islet cell proliferation and insulin secretion correlates with the onset of placental lactogen secretion. Endocrinol 1992; 130:1459-66; PMID:1537300
- Desai BM, Oliver-Krasinski J, De Leon DD, Farzad C, Hong N, Leach SD, Stoffers DA. Preexisting pancreatic acinar cells contribute to acinar cell, but not islet β cell, regeneration. J Clin Invest 2007; 117:971-7; PMID:17404620; http://dx.doi.org/10.1172/JCI29988
- Jiang Z, Song J, Qi F, Xiao A, An X, Liu NA, Zhu Z, Zhang B, Lin S. Exdpf is a key regulator of exocrine pancreas development controlled by retinoic acid and ptf1a in zebrafish. PLoS Biol 2008; 6:e293; PMID:19067490; http://dx.doi.org/10.1371/journal.pbio.0060293
- Collombat P, Hecksher-Sorensen J, Krull J, Berger J, Riedel D, Herrera PL, Serup P, Mansouri A. Embryonic endocrine pancreas and mature β cells acquire α and PP cell phenotypes upon Arx misexpression. J Clin Invest 2007; 117:961-70; PMID:17404619; http://dx.doi.org/10.1172/JCI29115
- Murtaugh LC. Pancreas and β-cell development: from the actual to the possible. Development 2007; 134:427-38; PMID:17185316; http://dx.doi.org/10.1242/dev.02770
- Li H, Arber S, Jessell TM, Edlund H. Selective agenesis of the dorsal pancreas in mice lacking homeobox gene Hlxb9. Nat Genet 1999; 23:67-70; PMID:10471501
- Harrison KA, Thaler J, Pfaff SL, Gu H, Kehrl JH. Pancreas dorsal lobe agenesis and abnormal islets of Langerhans in Hlxb9-deficient mice. Nat Genet 1999; 23:71-5; PMID:10471502
- Sherwood RI, Chen TY, Melton DA. Transcriptional dynamics of endodermal organ formation. Dev Dynam 2009; 238:29-42; PMID:19097184; http://dx.doi.org/10.1002/dvdy.21810
- Li H, Edlund H. Persistent expression of Hlxb9 in the pancreatic epithelium impairs pancreatic development. Dev Biol 2001; 240:247-53; PMID:11784060; http://dx.doi.org/10.1006/dbio.2001.0440
- Thor S, Ericson J, Brannstrom T, Edlund T. The homeodomain LIM protein Isl-1 is expressed in subsets of neurons and endocrine cells in the adult rat. Neuron 1991; 7:881-9; PMID:1764243; http://dx.doi.org/10.1016/0896-6273(91)90334-V
- Heddad Masson M, Poisson C, Guerardel A, Mamin A, Philippe J, Gosmain Y. Foxa1 and Foxa2 regulate α-cell differentiation, glucagon biosynthesis, and secretion. Endocrinol 2014; 155:3781-92; PMID:25057789; http://dx.doi.org/10.1210/en.2013-1843
- Maestro MA, Cardalda C, Boj SF, Luco RF, Servitja JM, Ferrer J. Distinct roles of HNF1beta, HNF1alpha, and HNF4alpha in regulating pancreas development, β-cell function and growth. Endocrine Dev 2007; 12:33-45; PMID:17923767; http://dx.doi.org/10.1159/000109603
- Monaghan AP, Kaestner KH, Grau E, Schutz G. Postimplantation expression patterns indicate a role for the mouse forkhead/HNF-3 α, β and gamma genes in determination of the definitive endoderm, chordamesoderm and neuroectoderm. Development 1993; 119:567-78; PMID:8187630
- Ang SL, Wierda A, Wong D, Stevens KA, Cascio S, Rossant J, Zaret KS. The formation and maintenance of the definitive endoderm lineage in the mouse: involvement of HNF3/forkhead proteins. Development 1993; 119:1301-15; PMID:8306889
- Landry C, Clotman F, Hioki T, Oda H, Picard JJ, Lemaigre FP, Rousseau GG. HNF-6 is expressed in endoderm derivatives and nervous system of the mouse embryo and participates to the cross-regulatory network of liver-enriched transcription factors. Dev Biol 1997; 192:247-57; PMID:9441665; http://dx.doi.org/10.1006/dbio.1997.8757
- Haumaitre C, Barbacci E, Jenny M, Ott MO, Gradwohl G, Cereghini S. Lack of TCF2/vHNF1 in mice leads to pancreas agenesis. Proc Natl Acad Sci USA 2005; 102:1490-5
- Solar M, Cardalda C, Houbracken I, Martin M, Maestro MA, De Medts N, Xu X, Grau V, Heimberg H, Bouwens L, et al. Pancreatic exocrine duct cells give rise to insulin-producing β cells during embryogenesis but not after birth. Dev Cell 2009; 17:849-60; PMID:20059954; http://dx.doi.org/10.1016/j.devcel.2009.11.003
- Rausa F, Samadani U, Ye H, Lim L, Fletcher CF, Jenkins NA, Copeland NG, Costa RH. The cut-homeodomain transcriptional activator HNF-6 is coexpressed with its target gene HNF-3 β in the developing murine liver and pancreas. Dev Biol 1997; 192:228-46; PMID:9441664; http://dx.doi.org/10.1006/dbio.1997.8744
- Jacquemin P, Lemaigre FP, Rousseau GG. The Onecut transcription factor HNF-6 (OC-1) is required for timely specification of the pancreas and acts upstream of Pdx-1 in the specification cascade. Dev Biol 2003; 258:105-16; PMID:12781686; http://dx.doi.org/10.1016/S0012-1606(03)00115-5
- Apelqvist A, Li H, Sommer L, Beatus P, Anderson DJ, Honjo T, Hrabe de Angelis M, Lendahl U, Edlund H. Notch signalling controls pancreatic cell differentiation. Nature 1999; 400:877-81; PMID:10476967; http://dx.doi.org/10.1038/23716
- Jensen J, Pedersen EE, Galante P, Hald J, Heller RS, Ishibashi M, Kageyama R, Guillemot F, Serup P, Madsen OD. Control of endodermal endocrine development by Hes-1. Nat Genet 2000; 24, 36-44
- Jacquemin P, Durviaux SM, Jensen J, Godfraind C, Gradwohl G, Guillemot F, Madsen OD, Carmeliet P, Dewerchin M, Collen D, et al. Transcription factor hepatocyte nuclear factor 6 regulates pancreatic endocrine cell differentiation and controls expression of the proendocrine gene ngn3. Mol Cell Biol 2000; 20:4445-54; PMID:10825208; http://dx.doi.org/10.1128/MCB.20.12.4445-4454.2000
- Pierreux CE, Poll AV, Kemp CR, Clotman F, Maestro MA, Cordi S, Ferrer J, Leyns L, Rousseau GG, Lemaigre FP. The transcription factor hepatocyte nuclear factor-6 controls the development of pancreatic ducts in the mouse. Gastroenterol 2006; 130:532-41; PMID:16472605; http://dx.doi.org/10.1053/j.gastro.2005.12.005
- Yamamoto K, Matsuoka TA, Kawashima S, Takebe S, Kubo F, Miyatsuka T, Kaneto H, Shimomura I. A novel function of Onecut1 protein as a negative regulator of MafA gene expression. J Biol Chem 2013; 288:21648-58; PMID:23775071; http://dx.doi.org/10.1074/jbc.M113.481424
- Zhang H, Ables ET, Pope CF, Washington MK, Hipkens S, Means AL, Path G, Seufert J, Costa RH, Leiter AB, et al. Multiple, temporal-specific roles for HNF6 in pancreatic endocrine and ductal differentiation. Mechan Dev 2009; 126:958-73; PMID:19766716; http://dx.doi.org/10.1016/j.mod.2009.09.006
- Gannon M, Ray MK, Van Zee K, Rausa F, Costa RH, Wright CV. Persistent expression of HNF6 in islet endocrine cells causes disrupted islet architecture and loss of β cell function. Development 2000; 127:2883-95; PMID:10851133
- Wu KL, Gannon M, Peshavaria M, Offield MF, Henderson E, Ray M, Marks A, Gamer LW, Wright CV, Stein R. Hepatocyte nuclear factor 3beta is involved in pancreatic β-cell-specific transcription of the pdx-1 gene. Mol Cell Biol 1997; 17:6002-13; PMID:9315659
- Cockell M, Stolarczyk D, Frutiger S, Hughes GJ, Hagenbuchle O, Wellauer PK. Binding sites for hepatocyte nuclear factor 3 β or 3 gamma and pancreas transcription factor 1 are required for efficient expression of the gene encoding pancreatic α-amylase. Mol Cell Biol 1995; 15:1933-41; PMID:7891687
- Gao N, LeLay J, Vatamaniuk MZ, Rieck S, Friedman JR, Kaestner KH. Dynamic regulation of Pdx1 enhancers by Foxa1 and Foxa2 is essential for pancreas development. Genes Dev 2008; 22:3435-48; PMID:19141476; http://dx.doi.org/10.1101/gad.1752608
- Lee CS, Sund NJ, Behr R, Herrera PL, Kaestner KH. Foxa2 is required for the differentiation of pancreatic α-cells. Dev Biol 2005; 278:484-95; PMID:15680365; http://dx.doi.org/10.1016/j.ydbio.2004.10.012
- Kanai-Azuma M, Kanai Y, Gad JM, Tajima Y, Taya C, Kurohmaru M, Sanai Y, Yonekawa H, Yazaki K, Tam PP, et al. Depletion of definitive gut endoderm in Sox17-null mutant mice. Development 2002; 129:2367-79; PMID:11973269
- Spence JR, Lange AW, Lin SC, Kaestner KH, Lowy AM, Kim I, Whitsett JA, Wells JM. Sox17 regulates organ lineage segregation of ventral foregut progenitor cells. Dev Cell 2009; 17:62-74; PMID:19619492; http://dx.doi.org/10.1016/j.devcel.2009.05.012
- Jonatan D, Spence JR, Method AM, Kofron M, Sinagoga K, Haataja L, Arvan P, Deutsch GH, Wells JM. Sox17 regulates insulin secretion in the normal and pathologic mouse β cell. PloS One 2014; 9:e104675; PMID:25144761; http://dx.doi.org/10.1371/journal.pone.0104675
- Guz Y, Montminy MR, Stein R, Leonard J, Gamer LW, Wright CV, Teitelman G. Expression of murine STF-1, a putative insulin gene transcription factor, in β cells of pancreas, duodenal epithelium and pancreatic exocrine and endocrine progenitors during ontogeny. Development 1995; 121:11-8; PMID:7867492
- Ahlgren U, Jonsson J, Jonsson L, Simu K, Edlund H. β-cell-specific inactivation of the mouse Ipf1/Pdx1 gene results in loss of the β-cell phenotype and maturity onset diabetes. Genes Dev 1998; 12:1763-8; PMID:9637677; http://dx.doi.org/10.1101/gad.12.12.1763
- Gannon M, Ables ET, Crawford L, Lowe D, Offield MF, Magnuson MA, Wright CV. Pdx-1 function is specifically required in embryonic β cells to generate appropriate numbers of endocrine cell types and maintain glucose homeostasis. Dev Biol 2008; 314:406-17; PMID:18155690; http://dx.doi.org/10.1016/j.ydbio.2007.10.038
- Holland AM, Hale MA, Kagami H, Hammer RE, MacDonald RJ. Experimental control of pancreatic development and maintenance. Proc Natl Acad Sci USA 2002; 99:12236-41; http://dx.doi.org/10.1073/pnas.192255099
- Hale MA, Kagami H, Shi L, Holland AM, Elsasser HP, Hammer RE, MacDonald RJ. The homeodomain protein PDX1 is required at mid-pancreatic development for the formation of the exocrine pancreas. Dev Biol 2005; 286:225-37; PMID:16126192; http://dx.doi.org/10.1016/j.ydbio.2005.07.026
- Jonsson J, Carlsson L, Edlund T, Edlund H. Insulin-promoter-factor 1 is required for pancreas development in mice. Nature 1994; 371:606-9; PMID:7935793; http://dx.doi.org/10.1038/371606a0
- Stoffers DA, Ferrer J, Clarke WL, Habener JF. Early-onset type-II diabetes mellitus (MODY4) linked to IPF1. Nat Genet 1997; 17:138-9; PMID:9326926; http://dx.doi.org/10.1038/ng1097-138
- Nicolino M, Claiborn KC, Senee V, Boland A, Stoffers DA, Julier C. A novel hypomorphic PDX1 mutation responsible for permanent neonatal diabetes with subclinical exocrine deficiency. Diabetes 2010; 59:733-40; PMID:20009086; http://dx.doi.org/10.2337/db09-1284
- Fujitani Y, Fujitani S, Boyer DF, Gannon M, Kawaguchi Y, Ray M, Shiota M, Stein RW, Magnuson MA, Wright CV. Targeted deletion of a cis-regulatory region reveals differential gene dosage requirements for Pdx1 in foregut organ differentiation and pancreas formation. Genes Dev 2006; 20:253-66; PMID:16418487; http://dx.doi.org/10.1101/gad.1360106
- Yang YP, Thorel F, Boyer DF, Herrera PL, Wright CV. Context-specific α- to-β-cell reprogramming by forced Pdx1 expression. Genes Dev 2011; 25:1680-5; PMID:21852533; http://dx.doi.org/10.1101/gad.16875711
- Gerrish K, Gannon M, Shih D, Henderson E, Stoffel M, Wright CV, Stein R. Pancreatic β cell-specific transcription of the pdx-1 gene. The role of conserved upstream control regions and their hepatic nuclear factor 3 β sites. J Biol Chem 2000; 275:3485-92; PMID:10652343; http://dx.doi.org/10.1074/jbc.275.5.3485
- Gerrish K, Van Velkinburgh JC, Stein R. Conserved transcriptional regulatory domains of the pdx-1 gene. Mol Endocrinol 2004; 18:533-48; PMID:14701942; http://dx.doi.org/10.1210/me.2003-0371
- Gerrish K, Cissell MA, Stein R. The role of hepatic nuclear factor 1 α and PDX-1 in transcriptional regulation of the pdx-1 gene. J Biol Chem 2001; 276:47775-84; PMID:11590182
- Samaras SE, Cissell MA, Gerrish K, Wright CV, Gannon M, Stein R. Conserved sequences in a tissue-specific regulatory region of the pdx-1 gene mediate transcription in Pancreatic β cells: role for hepatocyte nuclear factor 3 β and Pax6. Mol Cell Biol 2002; 22:4702-13; PMID:12052878; http://dx.doi.org/10.1128/MCB.22.13.4702-4713.2002
- Samaras SE, Zhao L, Means A, Henderson E, Matsuoka TA, Stein R. The islet β cell-enriched RIPE3b1/Maf transcription factor regulates pdx-1 expression. J Biol Chem 2003; 278:12263-70; PMID:12551916; http://dx.doi.org/10.1074/jbc.M210801200
- Krapp A, Knofler M, Ledermann B, Burki K, Berney C, Zoerkler N, Hagenbuchle O, Wellauer PK. The bHLH protein PTF1-p48 is essential for the formation of the exocrine and the correct spatial organization of the endocrine pancreas. Genes Dev 1998; 12:3752-63; PMID:9851981; http://dx.doi.org/10.1101/gad.12.23.3752
- Yoshitomi H, Zaret KS. Endothelial cell interactions initiate dorsal pancreas development by selectively inducing the transcription factor Ptf1a. Development 2004; 131:807-17; PMID:14736742; http://dx.doi.org/10.1242/dev.00960
- Jacquemin P, Yoshitomi H, Kashima Y, Rousseau GG, Lemaigre FP, Zaret KS. An endothelial-mesenchymal relay pathway regulates early phases of pancreas development. Dev Biol 2006; 290:189-99; PMID:16386727; http://dx.doi.org/10.1016/j.ydbio.2005.11.023
- Cano DA, Soria B, Martin F, Rojas A. Transcriptional control of mammalian pancreas organogenesis. Cell Mol Life Sci 2014; 71:2383-402; PMID:24221136; http://dx.doi.org/10.1007/s00018-013-1510-2
- Beres TM, Masui T, Swift GH, Shi L, Henke RM, MacDonald RJ. PTF1 is an organ-specific and Notch-independent basic helix-loop-helix complex containing the mammalian suppressor of hairless (RBP-J) or its paralogue, RBP-L. Mol Cell Biol 2006; 26:117-30; PMID:16354684; http://dx.doi.org/10.1128/MCB.26.1.117-130.2006
- Ehebauer M, Hayward P, Arias AM. Notch, a universal arbiter of cell fate decisions. Science 2006; 314:1414-5; PMID:17138893; http://dx.doi.org/10.1126/science.1134042
- Masui T, Long Q, Beres TM, Magnuson MA, MacDonald RJ. Early pancreatic development requires the vertebrate suppressor of hairless (RBPJ) in the PTF1 bHLH complex. Genes Dev 2007; 21:2629-43; PMID:17938243; http://dx.doi.org/10.1101/gad.1575207
- Masui T, Swift GH, Deering T, Shen C, Coats WS, Long Q, Elsasser HP, Magnuson MA, MacDonald RJ. Replacement of Rbpj with Rbpjl in the PTF1 complex controls the final maturation of pancreatic acinar cells. Gastroenterol 2010; 139:270-80; PMID:20398665; http://dx.doi.org/10.1053/j.gastro.2010.04.003
- Masui T, Swift GH, Hale MA, Meredith DM, Johnson JE, Macdonald RJ. Transcriptional autoregulation controls pancreatic Ptf1a expression during development and adulthood. Mol Cell Biol 2008; 28:5458-68; PMID:18606784; http://dx.doi.org/10.1128/MCB.00549-08
- Dong PD, Provost E, Leach SD, Stainier DY. Graded levels of Ptf1a differentially regulate endocrine and exocrine fates in the developing pancreas. Genes Dev 2008; 22:1445-50; PMID:18519637; http://dx.doi.org/10.1101/gad.1663208
- Fukuda A, Kawaguchi Y, Furuyama K, Kodama S, Horiguchi M, Kuhara T, Kawaguchi M, Terao M, Doi R, Wright CV, et al. Reduction of Ptf1a gene dosage causes pancreatic hypoplasia and diabetes in mice. Diabetes 2008; 57:2421-31; PMID:18591390; http://dx.doi.org/10.2337/db07-1558
- Ahnfelt-Ronne J, Jorgensen MC, Klinck R, Jensen JN, Fuchtbauer EM, Deering T, MacDonald RJ, Wright CV, Madsen OD, Serup P. Ptf1a-mediated control of Dll1 reveals an alternative to the lateral inhibition mechanism. Development 2012; 139:33-45; PMID:22096075; http://dx.doi.org/10.1242/dev.071761
- Thompson N, Gesina E, Scheinert P, Bucher P, Grapin-Botton A. RNA profiling and chromatin immunoprecipitation-sequencing reveal that PTF1a stabilizes pancreas progenitor identity via the control of MNX1/HLXB9 and a network of other transcription factors. Mol Cell Biol 2012; 32:1189-99; PMID:22232429; http://dx.doi.org/10.1128/MCB.06318-11
- Schaffer AE, Freude KK, Nelson SB, Sander M. Nkx6 transcription factors and Ptf1a function as antagonistic lineage determinants in multipotent pancreatic progenitors. Dev Cell 2010; 18:1022-9; PMID:20627083; http://dx.doi.org/10.1016/j.devcel.2010.05.015
- Pan FC, Bankaitis ED, Boyer D, Xu X, Van de Casteele M, Magnuson MA, Heimberg H, Wright CV. Spatiotemporal patterns of multipotentiality in Ptf1a-expressing cells during pancreas organogenesis and injury-induced facultative restoration. Development 2013; 140:751-64; PMID:23325761; http://dx.doi.org/10.1242/dev.090159
- Seymour PA, Freude KK, Tran MN, Mayes EE, Jensen J, Kist R, Scherer G, Sander M. SOX9 is required for maintenance of the pancreatic progenitor cell pool. Proc Natl Acad Sci USA 2007; 104:1865-70; http://dx.doi.org/10.1073/pnas.0609217104
- Lynn FC, Smith SB, Wilson ME, Yang KY, Nekrep N, German MS. Sox9 coordinates a transcriptional network in pancreatic progenitor cells. Proc Natl Acad Sci USA 2007; 104:10500-5; http://dx.doi.org/10.1073/pnas.0704054104
- Seymour PA, Shih HP, Patel NA, Freude KK, Xie R, Lim CJ, Sander M. A Sox9/Fgf feed-forward loop maintains pancreatic organ identity. Development 2012; 139:3363-72; PMID:22874919; http://dx.doi.org/10.1242/dev.078733
- Decker K, Goldman DC, Grasch CL, Sussel L. Gata6 is an important regulator of mouse pancreas development. Dev Biol 2006; 298:415-29; PMID:16887115; http://dx.doi.org/10.1016/j.ydbio.2006.06.046
- Watt AJ, Zhao R, Li J, Duncan SA. Development of the mammalian liver and ventral pancreas is dependent on GATA4. BMC Dev Biol 2007; 7:37; PMID:17451603; http://dx.doi.org/10.1186/1471-213X-7-37
- Ritz-Laser B, Mamin A, Brun T, Avril I, Schwitzgebel VM, Philippe J. The zinc finger-containing transcription factor Gata-4 is expressed in the developing endocrine pancreas and activates glucagon gene expression. Mol Endocrinol 2005; 19:759-70; PMID:15539431; http://dx.doi.org/10.1210/me.2004-0051
- Bossard P, Zaret KS. GATA transcription factors as potentiators of gut endoderm differentiation. Development 1998; 125:4909-17; PMID:9811575
- Cirillo LA, Lin FR, Cuesta I, Friedman D, Jarnik M, Zaret KS. Opening of compacted chromatin by early developmental transcription factors HNF3 (FoxA) and GATA-4. Mol Cell 2002; 9:279-89; PMID:11864602; http://dx.doi.org/10.1016/S1097-2765(02)00459-8
- Zaret KS, Watts J, Xu J, Wandzioch E, Smale ST, Sekiya T. Pioneer factors, genetic competence, and inductive signaling: programming liver and pancreas progenitors from the endoderm. Cold Spring Harb Symp Quant Biol 2008; 73:119-26; PMID:19028990; http://dx.doi.org/10.1101/sqb.2008.73.040
- Ketola I, Otonkoski T, Pulkkinen MA, Niemi H, Palgi J, Jacobsen CM, Wilson DB, Heikinheimo M. Transcription factor GATA-6 is expressed in the endocrine and GATA-4 in the exocrine pancreas. Mol Cell Endocrinol 2004; 226:51-7; PMID:15489005; http://dx.doi.org/10.1016/j.mce.2004.06.007
- Kuo CT, Morrisey EE, Anandappa R, Sigrist K, Lu MM, Parmacek MS, Soudais C, Leiden JM. GATA4 transcription factor is required for ventral morphogenesis and heart tube formation. Genes Dev 1997; 11:1048-60; PMID:9136932; http://dx.doi.org/10.1101/gad.11.8.1048
- Molkentin JD, Lin Q, Duncan SA, Olson EN. Requirement of the transcription factor GATA4 for heart tube formation and ventral morphogenesis. Genes Dev 1997; 11:1061-72; PMID:9136933; http://dx.doi.org/10.1101/gad.11.8.1061
- Koutsourakis M, Langeveld A, Patient R, Beddington R, Grosveld F. The transcription factor GATA6 is essential for early extraembryonic development. Development 1999; 126:723-32
- Xuan S, Borok MJ, Decker KJ, Battle MA, Duncan SA, Hale MA, Macdonald RJ, Sussel L. Pancreas-specific deletion of mouse Gata4 and Gata6 causes pancreatic agenesis. J Clin Invest 2012; 122:3516-28; PMID:23006325; http://dx.doi.org/10.1172/JCI63352
- Carrasco M, Delgado I, Soria B, Martin F, Rojas A. GATA4 and GATA6 control mouse pancreas organogenesis. J Clin Invest 2012; 122:3504-15; PMID:23006330; http://dx.doi.org/10.1172/JCI63240
- Rojas A, Schachterle W, Xu SM, Martin F, Black BL. Direct transcriptional regulation of Gata4 during early endoderm specification is controlled by FoxA2 binding to an intronic enhancer. Dev Biol 2010; 346:346-55; PMID:20692247; http://dx.doi.org/10.1016/j.ydbio.2010.07.032
- Martinelli P, Canamero M, del Pozo N, Madriles F, Zapata A, Real FX. Gata6 is required for complete acinar differentiation and maintenance of the exocrine pancreas in adult mice. Gut 2013; 62:1481-8; PMID:23002247; http://dx.doi.org/10.1136/gutjnl-2012-303328
- Gradwohl G, Dierich A, LeMeur M, Guillemot F. neurogenin3 is required for the development of the four endocrine cell lineages of the pancreas. Proc Natl Acad Sci USA 2000; 97:1607-11; http://dx.doi.org/10.1073/pnas.97.4.1607
- Schwitzgebel VM, Scheel DW, Conners JR, Kalamaras J, Lee JE, Anderson DJ, Sussel L, Johnson JD, German MS. Expression of neurogenin3 reveals an islet cell precursor population in the pancreas. Development 2000; 127:3533-42; PMID:10903178
- Johansson KA, Dursun U, Jordan N, Gu G, Beermann F, Gradwohl G, Grapin-Botton A. Temporal control of neurogenin3 activity in pancreas progenitors reveals competence windows for the generation of different endocrine cell types. Dev Cell 2007; 12:457-65; PMID:17336910; http://dx.doi.org/10.1016/j.devcel.2007.02.010
- Rukstalis JM, Habener JF. Neurogenin3: a master regulator of pancreatic islet differentiation and regeneration. Islets 2009; 1:177-84; PMID:21099270; http://dx.doi.org/10.4161/isl.1.3.9877
- Murtaugh LC, Stanger BZ, Kwan KM, Melton DA. Notch signaling controls multiple steps of pancreatic differentiation. Proc Natl Acad Sci USA 2003; 100:14920-5; http://dx.doi.org/10.1073/pnas.2436557100
- Shih HP, Kopp JL, Sandhu M, Dubois CL, Seymour PA, Grapin-Botton A, Sander M. A Notch-dependent molecular circuitry initiates pancreatic endocrine and ductal cell differentiation. Development 2012; 139:2488-99; PMID:22675211; http://dx.doi.org/10.1242/dev.078634
- Lee JC, Smith SB, Watada H, Lin J, Scheel D, Wang J, Mirmira RG, German MS. Regulation of the pancreatic pro-endocrine gene neurogenin3. Diabetes 2001; 50:928-36; PMID:11334435; http://dx.doi.org/10.2337/diabetes.50.5.928
- Wang S, Yan J, Anderson DA, Xu Y, Kanal MC, Cao Z, Wright CV, Gu G. Neurog3 gene dosage regulates allocation of endocrine and exocrine cell fates in the developing mouse pancreas. Dev Biol 2010; 339:26-37; PMID:20025861; http://dx.doi.org/10.1016/j.ydbio.2009.12.009
- Desgraz R, Herrera PL. Pancreatic neurogenin 3-expressing cells are unipotent islet precursors. Development 2009; 136:3567-74; PMID:19793886; http://dx.doi.org/10.1242/dev.039214
- Smith SB, Watada H, German MS. Neurogenin3 activates the islet differentiation program while repressing its own expression. Mol Endocrinol 2004; 18:142-9; PMID:14576336; http://dx.doi.org/10.1210/me.2003-0037
- Wang S, Hecksher-Sorensen J, Xu Y, Zhao A, Dor Y, Rosenberg L, Serup P, Gu G. Myt1 and Ngn3 form a feed-forward expression loop to promote endocrine islet cell differentiation. Dev Biol 2008; 317:531-40; PMID:18394599; http://dx.doi.org/10.1016/j.ydbio.2008.02.052
- Ejarque M, Cervantes S, Pujadas G, Tutusaus A, Sanchez L, Gasa R. Neurogenin3 cooperates with Foxa2 to autoactivate its own expression. JBiol Chem 2013; 288:11705-17; PMID:23471965; http://dx.doi.org/10.1074/jbc.M112.388173
- Smith SB, Gasa R, Watada H, Wang J, Griffen SC, German MS. Neurogenin3 and hepatic nuclear factor 1 cooperate in activating pancreatic expression of Pax4. J Biol Chem 2003; 278:38254-9; PMID:12837760; http://dx.doi.org/10.1074/jbc.M302229200
- Huang HP, Liu M, El-Hodiri HM, Chu K, Jamrich M, Tsai MJ. Regulation of the pancreatic islet-specific gene BETA2 (NeuroD) by neurogenin 3. Mol Cell Biol 2000; 20:3292-307; PMID:10757813; http://dx.doi.org/10.1128/MCB.20.9.3292-3307.2000
- Naya FJ, Huang HP, Qiu Y, Mutoh H, DeMayo FJ, Leiter AB, Tsai MJ. Diabetes, defective pancreatic morphogenesis, and abnormal enteroendocrine differentiation in BETA2/neuroD-deficient mice. Genes Dev 1997; 11:2323-34; PMID:9308961; http://dx.doi.org/10.1101/gad.11.18.2323
- Smith SB, Qu HQ, Taleb N, Kishimoto NY, Scheel DW, Lu Y, Patch AM, Grabs R, Wang J, Lynn FC, et al. Rfx6 directs islet formation and insulin production in mice and humans. Nature 2010; 463:775-80; PMID:20148032; http://dx.doi.org/10.1038/nature08748
- Gouzi M, Kim YH, Katsumoto K, Johansson K, Grapin-Botton A. Neurogenin3 initiates stepwise delamination of differentiating endocrine cells during pancreas development. Dev Dynam 2011; 240:589-604; PMID:21287656; http://dx.doi.org/10.1002/dvdy.22544
- Prasadan K, Tulachan S, Guo P, Shiota C, Shah S, Gittes G. Endocrine-committed progenitor cells retain their differentiation potential in the absence of neurogenin-3 expression. Biochem Biophys Res Commun 2010; 396:1036-41; PMID:20471370; http://dx.doi.org/10.1016/j.bbrc.2010.05.058
- Magenheim J, Klein AM, Stanger BZ, Ashery-Padan R, Sosa-Pineda B, Gu G, Dor Y. Ngn3(+) endocrine progenitor cells control the fate and morphogenesis of pancreatic ductal epithelium. Dev Biol 2011; 359:26-36; PMID:21888903; http://dx.doi.org/10.1016/j.ydbio.2011.08.006
- Piccand J, Meunier A, Merle C, Jia Z, Barnier JV, Gradwohl G. Pak3 promotes cell cycle exit and differentiation of β-cells in the embryonic pancreas and is necessary to maintain glucose homeostasis in adult mice. Diabetes 2014; 63:203-15; PMID:24163148; http://dx.doi.org/10.2337/db13-0384
- Osipovich AB, Long Q, Manduchi E, Gangula R, Hipkens SB, Schneider J, Okubo T, Stoeckert CJ, Jr., Takada S, Magnuson MA. Insm1 promotes endocrine cell differentiation by modulating the expression of a network of genes that includes Neurog3 and Ripply3. Development 2014; 141:2939-49; PMID:25053427; http://dx.doi.org/10.1242/dev.104810
- Mutoh H, Fung BP, Naya FJ, Tsai MJ, Nishitani J, Leiter AB. The basic helix-loop-helix transcription factor BETA2/NeuroD is expressed in mammalian enteroendocrine cells and activates secretin gene expression. Proc Natl Acad Sci USA 1997; 94:3560-4; http://dx.doi.org/10.1073/pnas.94.8.3560
- Gu C, Stein GH, Pan N, Goebbels S, Hornberg H, Nave KA, Herrera P, White P, Kaestner KH, Sussel L, et al. Pancreatic β cells require NeuroD to achieve and maintain functional maturity. Cell Metab 2010; 11:298-310; PMID:20374962; http://dx.doi.org/10.1016/j.cmet.2010.03.006
- Kojima H, Fujimiya M, Matsumura K, Younan P, Imaeda H, Maeda M, Chan L. NeuroD-betacellulin gene therapy induces islet neogenesis in the liver and reverses diabetes in mice. Nat Med 2003; 9:596-603; PMID:12704384; http://dx.doi.org/10.1038/nm867
- Noguchi H, Xu G, Matsumoto S, Kaneto H, Kobayashi N, Bonner-Weir S, Hayashi S. Induction of pancreatic stem/progenitor cells into insulin-producing cells by adenoviral-mediated gene transfer technology. Cell Transplantation 2006; 15:929-38; PMID:17299998; http://dx.doi.org/10.3727/000000006783981431
- Kaneto H, Matsuoka TA, Katakami N, Matsuhisa M. Combination of MafA, PDX-1 and NeuroD is a useful tool to efficiently induce insulin-producing surrogate β-cells. Curr Med Chem 2009; 16:3144-51; PMID:19689288; http://dx.doi.org/10.2174/092986709788802980
- Aftab S, Semenec L, Chu JS, Chen N. Identification and characterization of novel human tissue-specific RFX transcription factors. BMC Evol Biol 2008; 8:226; PMID:18673564; http://dx.doi.org/10.1186/1471-2148-8-226
- Feng C, Xu W, Zuo Z. Knockout of the regulatory factor X1 gene leads to early embryonic lethality. Biochem Biophys Res Commun 2009; 386:715-7; PMID:19559676; http://dx.doi.org/10.1016/j.bbrc.2009.06.111
- Ait-Lounis A, Baas D, Barras E, Benadiba C, Charollais A, Nlend Nlend R, Liegeois D, Meda P, Durand B, Reith W. Novel function of the ciliogenic transcription factor RFX3 in development of the endocrine pancreas. Diabetes 2007; 56:950-9; PMID:17229940; http://dx.doi.org/10.2337/db06-1187
- Soyer J, Flasse L, Raffelsberger W, Beucher A, Orvain C, Peers B, Ravassard P, Vermot J, Voz ML, Mellitzer G, et al. Rfx6 is an Ngn3-dependent winged helix transcription factor required for pancreatic islet cell development. Development 2010; 137:203-12; PMID:20040487; http://dx.doi.org/10.1242/dev.041673
- Piccand J, Strasser P, Hodson DJ, Meunier A, Ye T, Keime C, Birling MC, Rutter GA, Gradwohl G. Rfx6 maintains the functional identity of adult pancreatic β cells. Cell Rep 2014; 9:2219-32; PMID:25497096; http://dx.doi.org/10.1016/j.celrep.2014.11.033
- Chandra V, Albagli-Curiel O, Hastoy B, Piccand J, Randriamampita C, Vaillant E, Cave H, Busiah K, Froguel P, Vaxillaire M, et al. RFX6 regulates insulin secretion by modulating Ca(2+) homeostasis in human β cells. Cell Rep 2014; 9:2206-18; PMID:25497100; http://dx.doi.org/10.1016/j.celrep.2014.11.010
- Du A, Hunter CS, Murray J, Noble D, Cai CL, Evans SM, Stein R, May CL. Islet-1 is required for the maturation, proliferation, and survival of the endocrine pancreas. Diabetes 2009; 58:2059-69; PMID:19502415; http://dx.doi.org/10.2337/db08-0987
- May CL. The role of Islet-1 in the endocrine pancreas: lessons from pancreas specific Islet-1 deficient mice. Islets 2010; 2:121-3; PMID:21099304; http://dx.doi.org/10.4161/isl.2.2.10908
- Liu J, Hunter CS, Du A, Ediger B, Walp E, Murray J, Stein R, May CL. Islet-1 regulates Arx transcription during pancreatic islet α-cell development. J Biol Chem 2011; 286:15352-60; PMID:21388963; http://dx.doi.org/10.1074/jbc.M111.231670
- Hunter CS, Dixit S, Cohen T, Ediger B, Wilcox C, Ferreira M, Westphal H, Stein R, May CL. Islet α-, β-, and δ-cell development is controlled by the Ldb1 coregulator, acting primarily with the islet-1 transcription factor. Diabetes 2013; 62:875-86; PMID:23193182; http://dx.doi.org/10.2337/db12-0952
- Sussel L, Kalamaras J, Hartigan-O'Connor DJ, Meneses JJ, Pedersen RA, Rubenstein JL, German MS. Mice lacking the homeodomain transcription factor Nkx2.2 have diabetes due to arrested differentiation of pancreatic β cells. Development 1998; 125:2213-21; PMID:9584121
- Mastracci TL, Anderson KR, Papizan JB, Sussel L. Regulation of NeuroD1 contributes to the lineage potential of Neurogenin3+ endocrine precursor cells in the pancreas. PLoS Genet 2013; 9:e1003278; PMID:23408910; http://dx.doi.org/10.1371/journal.pgen.1003278
- Chao CS, Loomis ZL, Lee JE, Sussel L. Genetic identification of a novel NeuroD1 function in the early differentiation of islet α, PP and epsilon cells. Dev Biol 2007; 312:523-32; PMID:17988662; http://dx.doi.org/10.1016/j.ydbio.2007.09.057
- Kordowich S, Collombat P, Mansouri A, Serup P. Arx and Nkx2.2 compound deficiency redirects pancreatic α- and β-cell differentiation to a somatostatin/ghrelin co-expressing cell lineage. BMC Dev Biol 2011; 11:52; PMID:21880149; http://dx.doi.org/10.1186/1471-213X-11-52
- Mastracci TL, Wilcox CL, Arnes L, Panea C, Golden JA, May CL, Sussel L. Nkx2.2 and Arx genetically interact to regulate pancreatic endocrine cell development and endocrine hormone expression. Dev Biol 2011; 359:1-11; PMID:21856296; http://dx.doi.org/10.1016/j.ydbio.2011.08.001
- Raum JC, Gerrish K, Artner I, Henderson E, Guo M, Sussel L, Schisler JC, Newgard CB, Stein R. FoxA2, Nkx2.2, and PDX-1 regulate islet β-cell-specific mafA expression through conserved sequences located between base pairs -8118 and -7750 upstream from the transcription start site. Mol Cell Biol 2006; 26:5735-43; PMID:16847327; http://dx.doi.org/10.1128/MCB.00249-06
- Cissell MA, Zhao L, Sussel L, Henderson E, Stein R. Transcription factor occupancy of the insulin gene in vivo. Evidence for direct regulation by Nkx2.2. J Biol Chem 2003; 278:751-6; PMID:12426319; http://dx.doi.org/10.1074/jbc.M205905200
- Oster A, Jensen J, Edlund H, Larsson LI. Homeobox gene product Nkx 6.1 immunoreactivity in nuclei of endocrine cells of rat and mouse stomach. J Histochem Cytochem 1998; 46:717-21; PMID:9603782; http://dx.doi.org/10.1177/002215549804600603
- Sander M, Sussel L, Conners J, Scheel D, Kalamaras J, Dela Cruz F, Schwitzgebel V, Hayes-Jordan A, German M. Homeobox gene Nkx6.1 lies downstream of Nkx2.2 in the major pathway of β-cell formation in the pancreas. Development 2000; 127:5533-40; PMID:11076772
- Gauthier BR, Gosmain Y, Mamin A, Philippe J. The β-cell specific transcription factor Nkx6.1 inhibits glucagon gene transcription by interfering with Pax6. Biochem J 2007; 403:593-601; PMID:17263687; http://dx.doi.org/10.1042/BJ20070053
- Schisler JC, Jensen PB, Taylor DG, Becker TC, Knop FK, Takekawa S, German M, Weir GC, Lu D, Mirmira RG, et al. The Nkx6.1 homeodomain transcription factor suppresses glucagon expression and regulates glucose-stimulated insulin secretion in islet β cells. Proc Natl Acad Sci USA 2005; 102:7297-302; http://dx.doi.org/10.1073/pnas.0502168102
- Nelson SB, Schaffer AE, Sander M. The transcription factors Nkx6.1 and Nkx6.2 possess equivalent activities in promoting β-cell fate specification in Pdx1+ pancreatic progenitor cells. Development 2007; 134:2491-500; PMID:17537793; http://dx.doi.org/10.1242/dev.002691
- Henseleit KD, Nelson SB, Kuhlbrodt K, Hennings JC, Ericson J, Sander M. NKX6 transcription factor activity is required for α- and β-cell development in the pancreas. Development 2005; 132:3139-49; PMID:15944193; http://dx.doi.org/10.1242/dev.01875
- Schaffer AE, Taylor BL, Benthuysen JR, Liu J, Thorel F, Yuan W, Jiao Y, Kaestner KH, Herrera PL, Magnuson MA, et al. Nkx6.1 controls a gene regulatory network required for establishing and maintaining pancreatic β cell identity. PLoS Genet 2013; 9:e1003274; PMID:23382704; http://dx.doi.org/10.1371/journal.pgen.1003274
- Collombat P, Hecksher-Sorensen J, Broccoli V, Krull J, Ponte I, Mundiger T, Smith J, Gruss P, Serup P, Mansouri A. The simultaneous loss of Arx and Pax4 genes promotes a somatostatin-producing cell fate specification at the expense of the α- and β-cell lineages in the mouse endocrine pancreas. Development 2005; 132:2969-80; PMID:15930104; http://dx.doi.org/10.1242/dev.01870
- Collombat P, Mansouri A, Hecksher-Sorensen J, Serup P, Krull J, Gradwohl G, Gruss P. Opposing actions of Arx and Pax4 in endocrine pancreas development. Genes Dev 2003; 17:2591-603; PMID:14561778; http://dx.doi.org/10.1101/gad.269003
- Kordowich S, Mansouri A, Collombat P. Reprogramming into pancreatic endocrine cells based on developmental cues. Mol Cell Endocrinol 2010; 315:11-8; PMID:19897012; http://dx.doi.org/10.1016/j.mce.2009.10.015
- Dohrmann C, Gruss P, Lemaire L. Pax genes and the differentiation of hormone-producing endocrine cells in the pancreas. Mech Dev 2000; 92:47-54; PMID:10704887; http://dx.doi.org/10.1016/S0925-4773(99)00324-X
- Smith SB, Ee HC, Conners JR, German MS. Paired-homeodomain transcription factor PAX4 acts as a transcriptional repressor in early pancreatic development. Mol Cell Biol 1999; 19:8272-80; PMID:10567552
- Sosa-Pineda B, Chowdhury K, Torres M, Oliver G, Gruss P. The Pax4 gene is essential for differentiation of insulin-producing β cells in the mammalian pancreas. Nature 1997; 386:399-402; PMID:9121556; http://dx.doi.org/10.1038/386399a0
- Greenwood AL, Li S, Jones K, Melton DA. Notch signaling reveals developmental plasticity of Pax4(+) pancreatic endocrine progenitors and shunts them to a duct fate. Mech Dev 2007; 124:97-107; PMID:17196797; http://dx.doi.org/10.1016/j.mod.2006.11.002
- Wang Q, Elghazi L, Martin S, Martins I, Srinivasan RS, Geng X, Sleeman M, Collombat P, Houghton J, Sosa-Pineda B. Ghrelin is a novel target of Pax4 in endocrine progenitors of the pancreas and duodenum. Dev Dyn 2008; 237:51-61; PMID:18058910; http://dx.doi.org/10.1002/dvdy.21379
- Ritz-Laser B, Estreicher A, Gauthier BR, Mamin A, Edlund H, Philippe J. The pancreatic β-cell-specific transcription factor Pax-4 inhibits glucagon gene expression through Pax-6. Diabetologia 2002; 45:97-107; PMID:11845228; http://dx.doi.org/10.1007/s125-002-8249-9
- Wang J, Elghazi L, Parker SE, Kizilocak H, Asaano M, Sussel L, Sosa-Pineda B. The concerted activities of Pax4 and Nkx2.2 are essential to initiate pancreatic β-cell differentiation. Dev Biol 2004; 266:178-89; PMID:14729487; http://dx.doi.org/10.1016/j.ydbio.2003.10.018
- Collombat P, Xu X, Ravassard P, Sosa-Pineda B, Dussaud S, Billestrup N, Madsen OD, Serup P, Heimberg H, Mansouri A. The ectopic expression of Pax4 in the mouse pancreas converts progenitor cells into α and subsequently β cells. Cell 2009; 138:449-62; PMID:19665969; http://dx.doi.org/10.1016/j.cell.2009.05.035
- Turque N, Plaza S, Radvanyi F, Carriere C, Saule S. Pax-QNR/Pax-6, a paired box- and homeobox-containing gene expressed in neurons, is also expressed in pancreatic endocrine cells. Mol Endocrinol 1994; 8:929-38; PMID:7984154
- St-Onge L, Sosa-Pineda B, Chowdhury K, Mansouri A, Gruss P. Pax6 is required for differentiation of glucagon-producing α-cells in mouse pancreas. Nature 1997; 387:406-9; PMID:9163426; http://dx.doi.org/10.1038/387406a0
- Sander M, Neubuser A, Kalamaras J, Ee HC, Martin GR, German MS. Genetic analysis reveals that PAX6 is required for normal transcription of pancreatic hormone genes and islet development. Genes Dev 1997; 11:1662-73; PMID:9224716; http://dx.doi.org/10.1101/gad.11.13.1662
- Ashery-Padan R, Zhou X, Marquardt T, Herrera P, Toube L, Berry A, Gruss P. Conditional inactivation of Pax6 in the pancreas causes early onset of diabetes. Dev Biol 2004; 269:479-88; PMID:15110714; http://dx.doi.org/10.1016/j.ydbio.2004.01.040
- Heller RS, Stoffers DA, Liu A, Schedl A, Crenshaw EB, 3rd, Madsen OD, Serup P. The role of Brn4/Pou3f4 and Pax6 in forming the pancreatic glucagon cell identity. Dev Biol 2004; 268:123-34; PMID:15031110; http://dx.doi.org/10.1016/j.ydbio.2003.12.008
- Heller RS, Jenny M, Collombat P, Mansouri A, Tomasetto C, Madsen OD, Mellitzer G, Gradwohl G, Serup P. Genetic determinants of pancreatic epsilon-cell development. Dev Biol 2005; 286:217-24; PMID:16122727; http://dx.doi.org/10.1016/j.ydbio.2005.06.041
- Kang HS, ZeRuth G, Lichti-Kaiser K, Vasanth S, Yin Z, Kim YS, Jetten AM. Gli-similar (Glis) Kruppel-like zinc finger proteins: insights into their physiological functions and critical roles in neonatal diabetes and cystic renal disease. Histol Histopathol 2010; 25:1481-96; PMID:20865670
- Lichti-Kaiser K, ZeRuth G, Kang HS, Vasanth S, Jetten AM. Gli-similar proteins: their mechanisms of action, physiological functions, and roles in disease. Vitam Horm 2012; 88:141-71; PMID:22391303; http://dx.doi.org/10.1016/B978-0-12-394622-5.00007-9
- Watanabe N, Hiramatsu K, Miyamoto R, Yasuda K, Suzuki N, Oshima N, Kiyonari H, Shiba D, Nishio S, Mochizuki T, et al. A murine model of neonatal diabetes mellitus in Glis3-deficient mice. FEBS Letters 2009; 583:2108-13; PMID:19481545; http://dx.doi.org/10.1016/j.febslet.2009.05.039
- Kang HS, Kim YS, ZeRuth G, Beak JY, Gerrish K, Kilic G, Sosa-Pineda B, Jensen J, Pierreux CE, Lemaigre FP, et al. Transcription factor Glis3, a novel critical player in the regulation of pancreatic β-cell development and insulin gene expression. Mol Cell Biol 2009; 29:6366-79; PMID:19805515; http://dx.doi.org/10.1128/MCB.01259-09
- Yang Y, Chang BH, Yechoor V, Chen W, Li L, Tsai MJ, Chan L. The Kruppel-like zinc finger protein GLIS3 transactivates neurogenin 3 for proper fetal pancreatic islet differentiation in mice. Diabetologia 2011; 54:2595-605; PMID:21786021; http://dx.doi.org/10.1007/s00125-011-2255-9
- Yang Y, Chang BH, Samson SL, Li MV, Chan L. The Kruppel-like zinc finger protein Glis3 directly and indirectly activates insulin gene transcription. Nucleic Acids Res 2009; 37:2529-38; PMID:19264802; http://dx.doi.org/10.1093/nar/gkp122
- Yang Y, Chang BH, Chan L. Sustained expression of the transcription factor GLIS3 is required for normal β cell function in adults. EMBO Mol Med 2013; 5:92-104; PMID:23197416; http://dx.doi.org/10.1002/emmm.201201398
- ZeRuth GT, Takeda Y, Jetten AM. The Kruppel-like protein Gli-similar 3 (Glis3) functions as a key regulator of insulin transcription. Mol Endocrinol 2013; 27:1692-705; PMID:23927931; http://dx.doi.org/10.1210/me.2013-1117
- Kim YS, Kang HS, Takeda Y, Hom L, Song HY, Jensen J, Jetten AM. Glis3 regulates neurogenin 3 expression in pancreatic β-cells and interacts with its activator, Hnf6. Mol Cells 2012; 34:193-200; PMID:22820919; http://dx.doi.org/10.1007/s10059-012-0109-z
- Artner I, Hang Y, Mazur M, Yamamoto T, Guo M, Lindner J, Magnuson MA, Stein R. MafA and MafB regulate genes critical to β-cells in a unique temporal manner. Diabetes 2010; 59:2530-9; PMID:20627934; http://dx.doi.org/10.2337/db10-0190
- Nishimura W, Kondo T, Salameh T, El Khattabi I, Dodge R, Bonner-Weir S, Sharma A. A switch from MafB to MafA expression accompanies differentiation to pancreatic β-cells. Dev Biol 2006; 293:526-39; PMID:16580660; http://dx.doi.org/10.1016/j.ydbio.2006.02.028
- Hang Y, Stein R. MafA and MafB activity in pancreatic β cells. Trends Endocrinol Metab 2011; 22:364-73; PMID:21719305; http://dx.doi.org/10.1016/j.tem.2011.05.003
- Artner I, Blanchi B, Raum JC, Guo M, Kaneko T, Cordes S, Sieweke M, Stein R. MafB is required for islet β cell maturation. Proc Natl Acad Sci USA 2007; 104:3853-8; http://dx.doi.org/10.1073/pnas.0700013104
- Zhang C, Moriguchi T, Kajihara M, Esaki R, Harada A, Shimohata H, Oishi H, Hamada M, Morito N, Hasegawa K, et al. MafA is a key regulator of glucose-stimulated insulin secretion. Mol Cell Biol 2005; 25:4969-76; PMID:15923615; http://dx.doi.org/10.1128/MCB.25.12.4969-4976.2005
- Hang Y, Yamamoto T, Benninger RK, Brissova M, Guo M, Bush W, Piston DW, Powers AC, Magnuson M, Thurmond DC, et al. The MafA transcription factor becomes essential to islet β-cells soon after birth. Diabetes 2014; 63:1994-2005; PMID:24520122; http://dx.doi.org/10.2337/db13-1001
- Hu He K, Juhl K, Karadimos M, El Khattabi I, Fitzpatrick C, Bonner-Weir S, Sharma A. Differentiation of pancreatic endocrine progenitors reversibly blocked by premature induction of MafA. Dev Biol 2014; 385:2-12.; PMID:24183936; http://dx.doi.org/10.1016/j.ydbio.2013.10.024
- Willet SG, Hale MA, Grapin-Botton A, Magnuson MA, MacDonald RJ, Wright CV. Dominant and context-specific control of endodermal organ allocation by Ptf1a. Development 2014; 141:4385-94; PMID:25371369; http://dx.doi.org/10.1242/dev.114165
- Hale MA, Swift GH, Hoang CQ, Deering TG, Masui T, Lee YK, Xue J, MacDonald RJ. The nuclear hormone receptor family member NR5A2 controls aspects of multipotent progenitor cell formation and acinar differentiation during pancreatic organogenesis. Development 2014; 141:3123-33; PMID:25063451; http://dx.doi.org/10.1242/dev.109405
- Benitez CM, Qu K, Sugiyama T, Pauerstein PT, Liu Y, Tsai J, Gu X, Ghodasara A, Arda HE, Zhang J, et al. An integrated cell purification and genomics strategy reveals multiple regulators of pancreas development. PLoS Genet 2014; 10:e1004645; PMID:25330008; http://dx.doi.org/10.1371/journal.pgen.1004645
- Dorrell C, Tarlow B, Wang Y, Canaday PS, Haft A, Schug J, Streeter PR, Finegold MJ, Shenje LT, Kaestner KH, et al. The organoid-initiating cells in mouse pancreas and liver are phenotypically and functionally similar. Stem Cell Res 2014; 13:275-83; PMID:25151611; http://dx.doi.org/10.1016/j.scr.2014.07.006
- Afelik S, Pool B, Schmerr M, Penton C, Jensen J. Wnt7b is required for epithelial progenitor growth and operates during epithelial-to-mesenchymal signaling in pancreatic development. Dev Biol 2015; 399:204-17; PMID:25576928; http://dx.doi.org/10.1016/j.ydbio.2014.12.031
- Kroon E, Martinson LA, Kadoya K, Bang AG, Kelly OG, Eliazer S, Young H, Richardson M, Smart N, Cunningham J, Agulnick AD, D'Amour KA, Carpenter MK, Baetge, EE. Pancreatic endoderm derived from human embryonic stem cells generates glucose-responsive insulin-secreting cells in vivo. Nat Biotechnol 2008; 26:443-52; PMID:18288110; http://dx.doi.org/10.1038/nbt1393
- Ohneda K, EE H, German M. Regulation of insulin gene transcription. Seminars Cell Dev Biol 2000; 11:227-33; http://dx.doi.org/10.1006/scdb.2000.0171
- Abdellatif AM, Ogata K, Kudo T, Xiafukaiti G, Chang YH, Katoh MC, El-Morsy SE, Oishi H, Takahashi S. Role of large MAF transcription factors in the mouse endocrine pancreas. Exp Anim 2015; 64(3):305-12; PMID:25912440