Abstract
The fetal environment plays a decisive role in modifying the risk for developing diabetes later in life. Developing novel methodology for noninvasive imaging of β-cell development in vivo under the controlled physiological conditions of the host can serve to understand how this environment affects β-cell growth and differentiation. A number of culture models have been designed for pancreatic rudiment but none match the complexity of the in utero or even normal physiological environment. Speier et al. recently developed a platform of noninvasive in vivo imaging of pancreatic islets using the anterior chamber of the eye where islets get vascularized, grow and respond to physiological changes. The same methodology was adapted for the study of pancreatic development. E13.0, still undifferentiated rudiments with fluorescent lineage tracing were implanted in the AC of the eye, allowing the longitudinal study of their growth and differentiation. Within 48 h the anlages get vascularized and grow but their mesenchyme displays a selective growth advantage. The resulting imbalance leads to alteration in the differentiation pattern of the progenitors. Reducing the mesenchyme to its bare minimum before implantation allows the restoration of a proper balance and a development that mimics the normal pancreatic development. These groundbreaking observations demonstrate that the anterior chamber of the eye provides a good system for noninvasive in vivo fluorescence imaging of the developing pancreas under the physiology of the host and can have important implications for designing strategies to prevent or reverse the deleterious effects of hyperglycemia on altering β-cell function later in life.
Introduction
The capacity of β-cells to resist injury is critical in the prevention of the development of type 1 and type 2 diabetes. In type 2 diabetes, β-cells adapt to insulin resistance mainly by augmenting their proliferation, although cell size and modulation of apoptosis also play a role.Citation1,2 At birth, β-cell mass is traditionally believed to be the combination of genetic and “environmental” factors. Genetic studies have indicated that known variants can only account for approximately 10% of the genetic predisposition to type 2 diabetes. This suggests that additional unidentified genetic or environmental factors also significantly contribute to the susceptibility to this disease.Citation3,4 Animals born with a reduced β-cell mass have been described to be at a higher risk of developing type 2 diabetes in life.Citation1,5-19 A better understanding of how β-cells develop during embryogenesis will therefore have major impact for both type 1 and 2 diabetes.
The loss of pancreatic progenitors during early stages of development leads to a smaller pancreas overall and a decrease in β-cell mass.Citation20 Progenitors are extremely sensitive to stressors in particular during the primary and secondary transitions. Global caloric or protein restriction lead to decreased β-cell mass at birth and reduced insulin secretion later in lifeCitation12,21 but the effects during the development itself are still mostly unexplored.
The precise microenvironment required for normal pancreatic development remains vastly unknown. While different culture models have been designed to study pancreatic organogenesis, none of the culture conditions matches the complexity of the in vivo physiological environment or even the normal mouse physiology and the lack of interaction provided through vascularization and innervation is a major limitation.Citation22-26 Studies of the impact of the developmental programming of the β-cells remain static in nature and are typically limited to the analysis of the phenotype after birth or at selected developmental stages. Developing novel methodology for non-invasive imaging of β-cell development in vivo would serve to understand how the physiological environment impact on β-cell development and would provide clues about the impact of the diabetic milieu on differentiation, proliferation and survival of β-cells during development.
Recently, Speier et al. developed a platform of in vivo fluorescence imaging of pancreatic islets in the Anterior Chamber (AC) of the eye.Citation27 Using their approach, islets can be grafted in the AC of the mouse eye where the immune response is limited. The Iris, at the base of the AC, is highly vascularized and innervated, allowing the graft itself to be revascularized within 24 hours of implantation.Citation28,29 There, islets grow, get rapidly vascularized and innervated, and can respond to physiological changes in the host or to local interventions by topical application or intraocular injection. The implantation site is highly accessible and allows easy macroscopic observations. Fluorescent imaging techniques additionally allow repetitive in vivo imaging of the vascularization of the islets, their function and even cell death at cellular resolution.Citation27,30
In this manuscript, the same platform was adapted to study pancreas development. Pancreatic anlages grafted in the AC of the eye (see diagram in ) allow the longitudinal, in real time study of the effects of the physiological environment of the host on the development of the early endocrine progenitors. Combined with fluorescent lineage-tracing, the growth and maturation of the early pancreatic progenitors can be monitored in vivo over time. After implantation, the pancreatic anlages are rapidly vascularized and circulation is established, leading to a rapid physiological growth of the implanted pancreatic anlage in the AC of the eye. We further illustrate that the pancreatic mesenchyme has a selective growth-advantage over the epithelium and this imbalance impairs the proper differentiation of the early pancreatic progenitors. Reducing the mesenchyme to its minimum before the implantation helps restore a proper balance and a more harmonious differentiation of the early progenitors in a pattern that mimics normal pancreatic development.
Methods
Animal generation
The Pdx1-CreEarly Citation31 mouse line was obtained through a generous gift from P. Herrera. The CAG-EYFP (abbreviated as YFP) reporter miceCitation32 were purchased from The Jackson laboratory (stock number 011107, Bar Harbor, ME). Mice were maintained on a C57Bl6 background and interbred to obtain the necessary combined genotypes. Genotyping of all mice was performed by PCR. The University of Michigan Animal Studies Committees approved all the procedures.
Pancreatic rudiment dissection
Mouse pancreatic rudiments were isolated at E13.0 (E0.5 being defined as the morning of the discovery of the vaginal plug) as described previously.Citation33 Removal of the pancreatic mesenchyme was performed in cold HBSS under a Leica MZ-6 microscope (Leica Microsystems, Wetziar, Germany) with a stage providing illumination coming from underneath in order to differentiate epithelium from mesenchyme. The mesenchyme was then scraped off the epithelium using a 23G needle.
Injections
The rudiments were aspirated into the glass capillaries in a minimal volume of HBSS (10–20 μL) to facilitate injection into the AC of the eye.
CD1 albino mice were used for ease of visualization and were anesthetized by intraperitoneal injection of a ketamine/xylazine mix. The anesthetized mouse was placed under a stereomicroscope with the eye selected for transplantation facing upward. After applying a local anesthetic (proparacain), the cornea was punctured using a 32-gauge needle and a small incision was made using micro-scissors. A borosilicate glass capillary pulled with a micropipette puller (Sutter instrument) was mounted onto a Nanoject II micro-injector (Drumond Scientific, Broomall, PA, USA). The rudiments were aspirated into the glass capillaries in a minimal volume of HBSS (10–20 μL) and slowly injected into the AC of the eye.
While only one rudiment was injected at the time in the AC of the eye, for each set of experiment, 4 to 8 rudiments were injected and imaged to account for variations and reproducibility.
Image acquisition
In order to perform live imaging on the engrafted rudiments in the AC of the eye, the animals were anesthetized and either placed onto the micron III platform or on the stabilization device developed in-house and described in .
Live brightfield and fluorescent images were captured using a Micron III ophthalmology imaging microscope (Phoenix Research Laboratories, Pleasanton, CA, USA).
Brightfield and fluorescent images of the entire eyes after dissections were acquired using a Nikon Eclipse TS100 equipped with QIClick-F-M-12 camera (Q-IMaging Scientific, Surrey, BC, Canada) for fluorescence or brightfield or a Leica D-Lux camera with an eyepiece adapter mounted on a Leica MZ-6 microscope (Leica Microsystems, Wetziar, Germany) for brightfield.
When the depth of field didn't allow capturing the entire rudiment in focus on all planes, brightfield or fluorescence images were captured on multiple focal planes and Extended Depth of Field (EDF) was used to generate in focus images from the multiple captures.Citation34 The EDF image generation was performed through the Complex wavelet-based method using the EDF plug-in (available at bigwww.epfl.ch/demo/edf/) for ImageJ 1.50c.Citation35
Fluorescent immunostaining images were acquired using a Leica microscope DM5500B or a DM6000B with a motorized stage using a Leica DFC360FX camera (Leica Microsystems, Wetziar, Germany), interfaced with the OASIS-blue PCI controller and controlled by the Surveyor software (version 7.1, Objective Imaging Ltd, Cambridge, UK) for fluorescence or a DFC450C for brightfield imaging (Leica Microsystems, Wetziar, Germany).
Immunostaining
After dissection and extraction from the anterior chamber of the eye, tissues were fixed in 3.7% formalin in PBS for 20 minutes. Fixed rudiments were then embedded in Histogel (Thermo Scientific, Kalamazoo, MI) before paraffin processing and embedding. Formalin-fixed pancreatic tissues were embedded in paraffin using standard techniques. 5 µm sections were collected and analyzed for immunostaining. Slides were subjected to antigen retrieval using citrate buffer and permeabilized with 0.1% triton.
The primary antibodies were used overnight at the given dilution (Supplemental Methods Table 1). Sections were incubated with the appropriate secondary antibodies conjugated to FITC, AMCA or Cy3 (Jackson Immunoresearch, West Grove, PA). When necessary, nuclei were counterstained with DAPI (Vector Laboratories, Burlingame CA).
Results
Stabilization of the mice for improved fluorescence image acquisition
Fluorescence image acquisition requires significantly longer acquisition time than brightfield. While anesthetized, the breathing of the mice leads to rapid motion patterns of the head and affects the stable acquisition of the images, leading to motion blur. In order to limit the motion and improve the quality of the images, especially when images needed to be acquired on multiple planes to allow for the generation of EDF images, a stabilization device () was designed to stabilize the mouse head and limit the movements associated with respiratory excursions. The upper front teeth of the mouse are lodged in the front of the apparatus while the head is maintained by ear bars. Two adjustable clamps pinch the skin of the animal along the spine, while a third one holds the tail. The animal is effectively suspended, allowing the thorax to freely move bellow as the animal breathes, without inducing noticeable motions on the head. With this contraption, the animal can be properly stabilized for imaging ().
Embryonic rudiments implanted in the AC are quickly vascularized and grow
Based on our previous experience with mouse embryonic pancreatic rudiments culture,Citation33 we implanted pancreatic rudiments from E13.0 mouse embryos in the AC of the eye. We chose E13.0 because: 1) At this stage, the pancreatic anlage is mostly undifferentiated; 2) Earlier stages are difficult to maintain in 3D collagen culture gels and presented no advantages over E13.0 rudiments; and 3) at earlier stages, the rudiment might not be properly committed for the pancreatic fate, which could impair their capacity to form the different pancreatic cell types.
Rudiments were implanted by microinjection with a capillary needle in the AC of the eye immediately after dissection (one rudiment per AC). After four days, a number of vessels vascularize the graft from all sides () and penetrate the developing anlage, suggesting that vascularization of the implant stars shortly after implantation (, at 7 days, and Supplemental Video showing the blood flow penetrating in the grafted rudiment). The proper nutrients environment allows for a rapid growth of the tissue within the AC of the eye and at 7 days, the rudiments occupy most of the space available in the AC.
Visualization of fluorescent reporters during the development of the rudiments in the AC
Lineage-tracing was then used to follow the evolution of the rudiments through the cornea using mice with fluorescent labeling of all pancreatic epithelial cells (Pdx1-Cre; YFPCitation31). Mice expressing the YFP reporter under the control of the Pdx1-Cre recombinase express the reporter in all cells derived from the Pdx1 progenitors.Citation31 The development of the tissue was then followed over time by light- and fluorescent-microscopy throughout the duration of the experiment.
Fluorescence of embryonic pancreas can be observed before implantation (). After implantation, fluorescence is easily detectable through the cornea as the tissue develops in vivo (). As mentioned previously, significant growth and vascularization can be observed at 4 d post implantation () and persist throughout the duration of the experiment (). At 7 days, the implanted rudiment grew so large that EDF had to be used to reconstitute an in focus image of the tissue (). At this stage, the blood vessels form darker streaks that can be visualized on the fluorescence images (arrows in ).
Figure 3. Brightfield and fluorescent images of a Pdx1-Cre; YFP pancreatic rudiment at E13 right before implantation (A and A’ respectively); 4 d (B and B’ respectively), and 7 d after implantation (EDF images in C and C’ respectively). (D) Immunofluorescence for Insulin (blue), Glucagon (red) and CP-A (green) on sections from rudiments implanted for 7 d. (E) Immunofluorescence for E-Cadherin (red), the YFP reporter with a DAPI counterstain in a cross-section of the eye with a rudiment from a Pdx-Cre; YFP mouse. (F) Hematoxylin and Eosin stain, (G) Eosin stain and (H) immunofluorescence for Vimentin (red), YFP (green) with a DAPI counterstain on sections from Pdx-Cre; YFP rudiments implanted for 7 d Scale bars=50 μm.
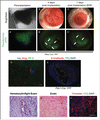
Immunostaining for endocrine (Insulin and Glucagon — which are the earliest endocrine markers expressed in developing mouse pancreas) and acinar cells (using CP-A) indicate that even though a few islet-like structures can be detected, very few acinar cells can be detected (). A wider-field image on a frontal cross section of the AC in a Pdx-Cre; YFP rudiment illustrates that the Pdx1 cells in the graft form large epithelial evaginations that extend throughout a mass of mesenchymal cells (, the cells derived from the Pdx1+ progenitors are traced with the green fluorescent YFP reporter). And are further outlined by the E-Cadherin staining. The DAPI counterstain helps illustrate how the mesenchyme dramatically outgrow the epithelium itself.
While previous publications have indicated a risk of autoimmune attacks for islets implanted in the AC of the eye,Citation36 a Hematoxylin with a light Eosin stain demonstrates the absence of proinflammatory neutrophil or eosinophil (plurilobed), or lymphocytes (dark nucleus, low nuclear/cytoplasmic ratio and blue cytoplasm) infiltration ((). A darker Eosin stain illustrates the organization of the mesenchyme cells that compose most of the rudiment at this stage () while staining for Vimentin, a mesenchymal marker, and YFP confirms that the cells are indeed mesenchyme with no expression of the YFP reporter as expected (). These studies suggest that the cells surrounding the pancreatic epithelium correspond indeed to mesenchyme and not immune infiltration.
Reduction in mesenchyme improves the differentiation of the rudiments implanted in the AC of the eye
Epithelium-mesenchyme interactions are known to control the proper growth and differentiation of the early pancreatic buds.Citation22,23,37-47 Based on this information, we hypothesized that disproportional growth of the mesenchymal cells in the implanted rudiments could affect the proper balance between the 2 compartments and alter normal pancreatic differentiation. To counteract the negative effect of the mesenchyme on the differentiation program of the pancreatic epithelium, rudiments with an approximate 80% reduction of mesenchyme were then implanted in the AC (see diagram in ) with the assumption that the remaining mesenchyme would be enough to induce proper pancreatic epithelial growth and differentiation. and B′ shows the pancreatic anlages after removal of the mesenchyme. As with the previous experiments, the implanted rudiments quickly grew and got vascularized (). Seven days after implantation, the tissue had not taken up all the space in the AC, unlike what had been seen with complete rudiments (). The experiment was prolonged to 18 days, when the rudiments had taken most of the AC, giving it more time to properly differentiate (). At 12 days, the epithelium branches and forms expansions within the surrounding mesenchyme, mirroring what would be expected during normal pancreatic development. This phenomenon was even further enhanced after 18 d (), at which point the tissue was isolated and processed. Immunostaining for CP-A revealed that the reduction in mesenchyme allowed for the maturation of acinar cells, though the cells were scattered among the epithelium (). Clusters of endocrine (Insulin or Glucagon) cells were also found throughout the tissue (). Sox9 expression was detected in a number of clusters of epithelial cells, as well as in duct-like structures (). Examination of the implant at different levels showed that the proportion of non-epithelial cells was still important, illustrating that 80% reduction in mesenchyme at the time of implantation might not have been sufficient to re-balance the amount of mesenchyme that developed throughout the experiment.
Figure 4. (A) Diagram representing the partial reduction in mesenchyme before implantation. Brightfield and fluorescent images of a Pdx1-Cre; YFP pancreatic rudiment at E13 with reduced mesenchyme right before implantation (B and B’ respectively); 2 d (C and C’ respectively), 12 d after implantation (D and D’ respectively), 18 d after implantation (E and E’ respectively) and as the eye has been removed at the end of the experiment at 18 d after implantation (F and F’ respectively). (G) Immunofluorescence for CP-A (red), and YFP (green) on sections from the rudiments implanted for 18 d H. Immunofluorescence for Insulin (blue), Glucagon (red) and YFP (green) on sections from the rudiments implanted for 18 d (I) Immunofluorescence for Sox9 (red) and YFP (green) with a DAPI nuclear counterstain (blue) on sections from the rudiments implanted for 18 d Scale bars = 50 μm.
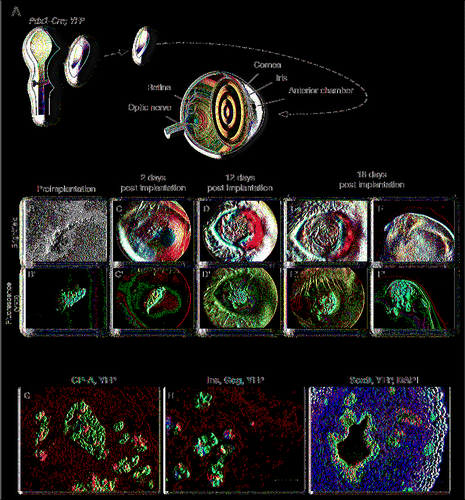
Mesenchyme depletion restores proper differentiation of the rudiments implanted in the AC
In order to further reduce the amount of mesenchyme developing post-implantation, approximately 95% of mesenchyme was removed by simply scraping it off the pancreatic epithelium instead of using the previously described “collagenase method”Citation22,23,48 (). This method was preferred because it preserves some of the mesenchyme, while preventing the stickiness of the tissue inherent to the protocol, and having any trace of the enzyme — which could be deleterious for the Cornea or any tissue surrounding the implant.
Figure 5. (A) Diagram representing the near-complete reduction in mesenchyme before implantation. Brightfield and fluorescent images of a Pdx1-Cre; YFP pancreatic rudiment at E13 with almost no mesenchyme right before implantation (B and B’ respectively); 5 d (C and C’ respectively) and 7 d after implantation (D and D’ respectively) and as the eye has been removed at the end of the experiment at 7 d after implantation (E and E’ respectively). (F) Immunofluorescence on sections from the rudiments implanted for 7 d for E-Cadherin (red), Insulin (blue) and YFP (green) (F); Pdx1 (red) and YFP (green) with a DAPI nuclear counterstain (blue; G); Sox9 (red) and YFP (green) with a DAPI nuclear counterstain (blue; H); Insulin (blue), Glucagon (red) and YFP (green; I); Insulin (blue), CP-A (red) and YFP (green; J); Amylase (red) and YFP (green) with a DAPI nuclear counterstain (blue; K); Insulin (blue), Glucagon (red) and YFP (green; I); DBA (red) and YFP (green; L); Hematoxylin staining (M with a magnification below) and vWF (red) and YFP (green; N). Scale bars = 50 μm. Scale bars = 50 μm.
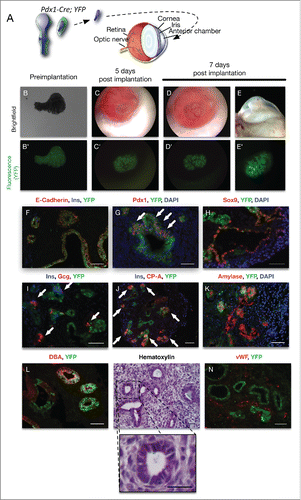
The tissue quickly grew and got vascularized () while the fluorescent reporter illustrated how the pancreatic epithelium started progressively budding within its environment (). Interestingly, while long epithelial projections could frequently be seen when the implanted rudiments had larger amounts of mesenchyme (see ), such evaginations were never observed with 95% reduction in mesenchyme ().
After 7 d in the AC, immunostaining for E-Cadherin indicated that even though most epithelial cells express the fluorescent reporter, as expected with this Pdx1-Cre line, some mosaicism could be observed within the Pdx1-Cre line and some of the epithelial cells did not express the YFP reporter (). Immunostaining for Pdx1 indicated the presence of Pdx1+ clusters, either from undifferentiated cells or from forming islets (, see white arrows). Sox9 expression was found to be high either in duct-like structures or in clusters of cells (). Small groups of endocrine cells expressing either Insulin or Glucagon were also scattered throughout the tissue, similar to what would be expected during normal pancreatic development (, see white arrows in I). Acinar cells expressing CP-A were far more frequent than in the previous experimental designs and formed acini budding from small epithelial ducts, similar to what is frequently observed in vivo during pancreatic organogenesis (). While Carboxypeptidase-A is commonly seen in both differentiating and mature acinar cells, it can be expressed in still pluripotent progenitors.Citation49 Immunostaining for Amylase confirmed that structures located at the tip of the branching within the rudiments were indeed mature acinar cells (). Ductal structures expressing DBA were also frequently found throughout the rudiments (). Concentrated Hematoxylin at normal pH binds to Mucins and proteoglycans and is routinely used in pathology (as described inCitation50). The cytoplasmic hematoxylin staining in the ducts cells in (with a magnification over a duct below) confirms the presence of Mucins in the ducts of the implanted rudiments and therefore provides additional evidence of the maturity of these cells. A staining for the von Willebrand factor illustrated that the vascularization described for reaches deep within the developing rudiments, in the vicinity of the developing epithelium ().
Discussion
This study illustrates a novel approach to study pancreas development. We show that undifferentiated pancreatic rudiments can be implanted in the AC of the eye. The grafts quickly get vascularized and grow in the rich physiological environment of the host ().
The current results demonstrate that implanting E13.0 complete pancreatic rudiments in the AC results in growth imbalance between epithelium and mesenchyme, leading to alterations in the differentiation pattern of the early pancreatic progenitors (). These results are similar to those observed when the epithelium is exposed to high levels of growth factors.Citation48 Reducing the amount of mesenchyme to its bare minimum restores a proper growth balance between mesenchyme and pancreas epithelium and allows for all the pancreatic compartments to properly differentiate (). This effect is not due to the sheer reduction in tissue size and the space available in the AC of the eye as smaller rudiments with normal epithelium/mesenchyme ratio implanted in the AC of the eye still present an unbalanced growth of the mesenchyme and altered differentiation (data not shown). The pace of the vascularization of the rudiment could also play an important role. With a reduced mesenchyme, the epithelial cells are likely to be vascularized faster. These studies confirm previous data showing the inhibitory effects of the mesenchyme on the differentiation program of the pancreatic epithelium.Citation22,23,48 During pancreatic organogenesis, the interplay between epithelium and mesenchyme essentially controls the growth and differentiation of the pancreatic progenitors.Citation22-24,40 During the early stages of pancreatic development, the mesenchyme secretes different growth factors that will signal to the subjacent epithelium. These factors stimulate the proliferation of the early progenitors, but conversely inhibit their differentiation.Citation24,48,51,52 The precise timing of these events defines the balance between growth and differentiation throughout pancreatic organogenesis. The selective growth advantage of the mesenchyme in the AC could be due to the fact that the AC itself provides growth factors that differentially affect the 2 compartments. It could also be that the mesenchyme is directly exposed to the milieu of the AC itself and gets vascularized first. The resulting imbalance is likely to explain the poor differentiation observed when all the mesenchyme was intact. Future studies can assess the role of vascularization on the differential growth of the mesenchyme by performing a time course of the vascularization process of the implant.
In a previous study, Adeghate et al. implanted pancreatic fragments from mature, adult pancreas. In their study, the acinar cells degenerated and only islets and ducts survived.Citation29 This is likely due to the stress of the isolation and the fact that the mature cells were not immediately vascularized. Acinar cells that are not in a proper environment quickly degenerate after dissection and release their digestive enzymes, leading to a cascade of acinar necrosis. In our experiments, the embryonic rudiments were implanted before they were differentiated, and were allowed to grow within the physiological environment of the host. Our data show that after achieving a proper balance of epithelium/mesenchyme interaction, the epithelium branches and acinar cells can properly differentiate and grow, budding from the early ducts ().
The growth of the rudiments in the AC is also a significant improvement over the different culture models that have been previously described. The ducts branch within the mesenchyme, forming acinar cells at the tip of the branches and endocrine cells gather in islet-like structures, which contrasts with filter-based methods, 2D on glass slide, 3D in collagen or matrigel or free-floating cultures where ductal structures typically do not form and endocrine cells are scattered throughout the acinar tissue, but never form proper islets.Citation22,24-26,53-55 Together, a qualitative assessment of these results indicate that the rudiments recapitulate pancreatic development and that the environment provides the necessary nutrients to allow the maturation of the major cell types that characterize a mature pancreas. Seven days after implantation, while the rudiments have developed in a dynamic that coincides with what would be expected in vivo,Citation56 there appears to be a slight developmental delay compared to a similar timeline in vivo. This could be due to the stress of the dissection and implantation as well as the timing for engraftment and vascular infiltration. It could also result from the fact that the AC of the eye only offers limited space to grow.
While the site of implantation doesn't allow us to perfectly re-create the in utero physiological conditions, the rudiments are nonetheless exposed to the physiology of the host during normal conditions and develop in an environment far closer to the in utero conditions than they would be in any culture media. The differences between the normal physiology of the host and the in utero conditions could also account for the apparent developmental delay that can still be observed in this model. Future experiments could further improve the model by implanting the anlages in the AC of the eye of time-matched pregnant females.
The fetal nutrient environment plays a critical role in modifying the risk for developing diabetes later in life.Citation5-19,57 The AC of the eye has already been successfully used for the study of the adaptation of pancreatic islets to various diabetogenic conditions.Citation52,58 Using pancreatic anlages, the same platform can be further used for the study of β-cell programing in a number of models of diabetes, nutrient restriction or the analysis of the effects of pharmacologic agents on the development of the pancreas, while providing a unique way to trace the effects in vivo, in real time, longitudinally, through the cornea. This may help mitigate the onset of T2D in fetuses in high-risk intrauterine exposures and can help conceive strategies to curb a lifetime-increased risk of T2D set at birth.
Abbreviations
AC | = | anterior Chamber |
AMCA | = | Aminomethylcoumarin |
CP-A | = | carboxypeptidase A |
DBA | = | lectin Dolichos biflorus agglutinin |
EDF | = | Extended Depth of Field |
FITC | = | Fluorescein isothiocyanate |
Gcg | = | Glucagon |
GFP | = | Green fluorescent protein |
Pdx1 | = | Pancreatic and duodenal homeobox 1 |
Sox9 | = | SRY (sex determining region Y)-box 9 |
Vwf | = | von Willebrand Factor |
YFP | = | Yellow fluorescent protein |
Disclosure of potential conflicts of interest
No potential conflicts of interest were disclosed.
Author contributions
C. C.-M.: Study concept and design; acquisition of data; analysis and interpretation of data; drafting of the manuscript; L.E., P.F.: Study concept and design; acquisition of data; analysis and interpretation of data, revisions of the manuscript; E. B.-M.: Study concept and design, analysis of the results and revisions of the manuscript.
KISL_1148236_Supplemental_Files.zip
Download Zip (16 MB)Acknowledgments
The Pdx1-Cre mouse line was obtained through a generous gift from P. Herrera. Tissue processing and embedding was performed through the University of Michigan Microscopy & Image Analysis Laboratory.
Funding
This work was supported by National Institutes of Health Grant RO1 DK084236 and DK073716 (to E.B-M.). This study was also supported by a Diabetes Investigator Award from the Greater Good Foundation to C. C.-M. This work also utilized the histology and imaging as 535 well as the instrument shop modules of the Core Center for Vision Research funded by P30 EY007003 from the National Eye Institute and the Michigan Morphology & Image Analysis core for the Diabetes Research Center funded by NIH (5P60 DK20572).
References
- Sandovici I, Hammerle CM, Ozanne SE, Constancia M. Developmental and environmental epigenetic programming of the endocrine pancreas: consequences for type 2 diabetes. Cell Mol Life Sci 2013; 70:1575-95; PMID:23463236; http://dx.doi.org/10.1007/s00018-013-1297-1
- Dor Y, Brown J, Martinez OI, Melton DA. Adult pancreatic beta-cells are formed by self-duplication rather than stem-cell differentiation. Nature 2004; 429:41-6; PMID:15129273; http://dx.doi.org/10.1038/natu-re02520
- Lango H, Consortium UKTDG, Palmer CN, Morris AD, Zeggini E, Hattersley AT, McCarthy MI, Frayling TM, Weedon MN. Assessing the combined impact of 18 common genetic variants of modest effect sizes on type 2 diabetes risk. Diabetes 2008; 57:3129-35; PMID:18591388; http://dx.doi.org/10.2337/db08-0504
- Morris AP, Voight BF, Teslovich TM, Ferreira T, Segre AV, Steinthorsdottir V, Strawbridge RJ, Khan H, Grallert H, Mahajan A, et al. Large-scale association analysis provides insights into the genetic architecture and pathophysiology of type 2 diabetes. Nat Gen 2012; 44:981-90; PMID:22885922; http://dx.doi.org/10.1038/ng.2383
- Hellerström C, Swenne I. Functional maturation and proliferation of fetal pancreatic beta-cells. Diabetes 1991; 40(Suppl 2):89-93; PMID:1748274; http://dx.doi.org/10.2337/diab.40.2.S89
- Berney DM, Desai M, Palmer DJ, Greenwald S, Brown A, Hales CN, Berry CL. The effects of maternal protein deprivation on the fetal rat pancreas: major structural changes and their recuperation. J Pathol 1997; 183:109-15; PMID:9370956; http://dx.doi.org/10.1002/(SICI)1096-9896(199709)183:1%3c109::AID-PATH1091%3e3.0.CO;2-B
- Dahri S, Snoeck A, Reusens-Billen B, Remacle C, Hoet JJ. Islet function in offspring of mothers on low-protein diet during gestation. Diabetes 1991; 40(Suppl 2):115-20; PMID:1748239; http://dx.doi.org/10.2337/diab.40.2.S115
- Dahri S, Reusens B, Remacle C, Hoet JJ. Nutritional influences on pancreatic development and potential links with non-insulin-dependent diabetes. Proc Nutr Soc 1995; 54:345-56; PMID:8524881; http://dx.doi.org/10.1079/PNS19950003
- Aldoretta PW, Carver TD, Hay WW. Maturation of glucose-stimulated insulin secretion in fetal sheep. Biol Neonate 1998; 73:375-86; PMID:9618055; http://dx.doi.org/10.1159/000014000
- Carver TD, Anderson SM, Aldoretta PW, Hay WW. Effect of low-level basal plus marked “pulsatile” hyperglycemia on insulin secretion in fetal sheep. Am J Physiol 1996; 271:E865-71; PMID:8944673
- Ozanne SE, Hales CN. The long-term consequences of intra-uterine protein malnutrition for glucose metabolism. Proc Nutr Soc 1999; 58:615-9; PMID:10604194; http://dx.doi.org/10.1017/S0029665199000804
- Petrik J, Reusens B, Arany E, Remacle C, Coelho C, Hoet JJ, Hill DJ. A low protein diet alters the balance of islet cell replication and apoptosis in the fetal and neonatal rat and is associated with a reduced pancreatic expression of insulin-like growth factor-II. Endocrinology 1999; 140:4861-73; PMID:10499546
- Snoeck A, Remacle C, Reusens B, Hoet JJ. Effect of a low protein diet during pregnancy on the fetal rat endocrine pancreas. Biol Neonate 1990; 57:107-18; PMID:2178691; http://dx.doi.org/10.1159/000243170
- Carver TD, Anderson SM, Aldoretta PA, Esler AL, Hay WW. Glucose suppression of insulin secretion in chronically hyperglycemic fetal sheep. Pediatr Res 1995; 38:754-62; PMID:8552445; http://dx.doi.org/10.1203/00006450-199511000-00020
- Van Assche FA, De Prins F, Aerts L, Verjans M. The endocrine pancreas in small-for-dates infants. Br J Obst Gynaecol 1977; 84:751-3; PMID:336076; http://dx.doi.org/10.1111/j.1471-0528.1977.tb12486.x
- Dumortier O, Blondeau B, Duvillié B, Reusens B, Bréant B, Remacle C. Different mechanisms operating during different critical time-windows reduce rat fetal beta cell mass due to a maternal low-protein or low-energy diet. Diabetologia 2007; 50:2495-503; PMID:17882398; http://dx.doi.org/10.1007/s00125-007-0811-0
- Garofano A, Czernichow P, Breant B. In utero undernutrition impairs rat beta-cell development. Diabetologia 1997; 40:1231-4; PMID:9349607; http://dx.doi.org/10.1007/s001250050812
- Winick M. Cellular growth in intrauterine malnutrition. Pediatr Clin North Am 1970; 17:69-78; PMID:5419110
- Garofano A, Czernichow P, Bréant B. Effect of ageing on beta-cell mass and function in rats malnourished during the perinatal period. Diabetologia 1999; 42:711-8; PMID:10382591; http://dx.doi.org/10.1007/s00125005-1219
- Stanger BZ, Tanaka AJ, Melton DA. Organ size is limited by the number of embryonic progenitor cells in the pancreas but not the liver. Nature 2007; 445:886-91; PMID:17259975; http://dx.doi.org/10.1038/nature05537
- Alejandro EU, Gregg B, Wallen T, Kumusoglu D, Meister D, Chen A, Merrins MJ, Satin LS, Liu M, Arvan P, et al. Maternal diet-induced microRNAs and mTOR underlie beta cell dysfunction in offspring. J Clin Invest 2014; 124:4395-410; PMID:25180600; http://dx.doi.org/10.1172/JCI74237
- Miralles F, Serup P, Cluzeaud F, Vandewalle A, Czernichow P, Scharfmann R. Characterization of beta cells developed in vitro from rat embryonic pancreatic epithelium. Dev Dyn 1999; 214:116-26; PMID:10030591; http://dx.doi.org/10.1002/(SICI)1097-0177(199902)214:2%3c116::AID-AJA2%3e3.0.CO;2-M
- Miralles F, Czernichow P, Scharfmann R. Follistatin regulates the relative proportions of endocrine versus exocrine tissue during pancreatic development. Development 1998; 125:1017-24; PMID:9463348
- Bhushan A, Itoh N, Kato S, Thiery J, Czernichow P, Bellusci S, Scharfmann R. Fgf10 is essential for maintaining the proliferative capacity of epithelial progenitor cells during early pancreatic organogenesis. Development 2001; 128:5109-17; PMID:11748146
- Huotari M, Miettinen P, Palgi J, Koivisto T, Ustinov J, Harari D, Yarden Y, Otonkoski T. ErbB signaling regulates lineage determination of developing pancreatic islet cells in embryonic organ culture. Endocrinology 2002; 143:4437-46; PMID:12399441; http://dx.doi.org/10.1210/en.2002-220382
- Duvillié B, Attali M, Aiello V, Quemeneur E, Scharfmann R. Label-retaining cells in the rat pancreas: location and differentiation potential in vitro. Diabetes 2003; 52:2035-42; PMID:12882920; http://dx.doi.org/10.2337/diabetes.52.8.2035
- Speier S, Nyqvist D, Köhler M, Caicedo A, Leibiger IB, Berggren P-O. Noninvasive high-resolution in vivo imaging of cell biology in the anterior chamber of the mouse eye. Nature protocols 2008; 3:1278-86; PMID:18714296; http://dx.doi.org/10.1038/nprot.2008.118
- Adeghate E. Host-graft circulation and vascular morphology in pancreatic tissue transplants in rats. Anat Rec 1998; 251:448-59; PMID:9713983; http://dx.doi.org/10.1002/(SICI)1097-0185(199808)251:4%3c448::AID-AR4%3e3.0.CO;2-O
- Adeghate E, Ponery AS, Ahmed I, Donáth T. Comparative morphology and biochemistry of pancreatic tissue fragments transplanted into the anterior eye chamber and subcutaneous regions of the rat. Eur J Morphol 2001; 39:257-68; PMID:12221507; http://dx.doi.org/10.1076/ejom.39.5.0257
- Speier S, Nyqvist D, Cabrera O, Yu J, Molano RD, Pileggi A, Moede T, Köhler M, Wilbertz J, Leibiger B, et al. Noninvasive in vivo imaging of pancreatic islet cell biology. Nat Med 2008; 14:574-8; PMID:18327249; http://dx.doi.org/10.1038/nm1701
- Herrera P-L. Adult insulin- and glucagon-producing cells differentiate from two independent cell lineages. Development 2000; 127:2317-22; PMID:10804174
- Madisen L, Zwingman TA, Sunkin SM, Oh SW, Zariwala HA, Gu H, Ng LL, Palmiter RD, Hawrylycz MJ, Jones AR, et al. A robust and high-throughput Cre reporting and characterization system for the whole mouse brain. Nat Neurosci 2010; 13:133-40; PMID:20023653; http://dx.doi.org/10.1038/nn.2467
- Cras-Méneur C, Li L, Kopan R, Permutt MA. Presenilins, Notch dose control the fate of pancreatic endocrine progenitors during a narrow developmental window. Genes Dev 2009; 23:2088-101; PMID:19723764; http://dx.doi.org/10.1101/gad.1800209
- Forster B, Van De Ville D, Berent J, Sage D, Unser M. Complex wavelets for extended depth-of-field: a new method for the fusion of multichannel microscopy images. Microsc Res Tech 2004; 65:33-42; PMID:15570586; http://dx.doi.org/10.1002/jemt.20092
- Schneider CA, Rasband WS, Eliceiri KW. NIH Image to ImageJ: 25 years of image analysis. Nat Methods 2012; 9:671-5; PMID:22930834; http://dx.doi.org/10.1038/nmeth.2089
- Mojibian M, Harder B, Hurlburt A, Bruin JE, Asadi A, Kieffer TJ. Implanted islets in the anterior chamber of the eye are prone to autoimmune attack in a mouse model of diabetes. Diabetologia 2013; 56:2213-21. PMID:23933952
- Ahlgren U, Jonsson J, Edlund H. The morphogenesis of the pancreatic mesenchyme is uncoupled from that of the pancreatic epithelium in IPF1/PDX1-deficient mice. Development 1996; 122:1409-16; PMID:8625829
- Ahlgren U, Pfaff SL, Jessell TM, Edlund T, Edlund H. Independent requirement for ISL1 in formation of pancreatic mesenchyme and islet cells. Nature 1997; 385:257-60; PMID:9000074; http://dx.doi.org/10.1038/385257a0
- Dudley AT, Godin RE, Robertson EJ. Interaction between FGF and BMP signaling pathways regulates development of metanephric mesenchyme. Gen Dev 1999; 13:1601-13; PMID:10385628; http://dx.doi.org/10.1101/gad.13.12.1601
- Scharfmann R. Control of early development of the pancreas in rodents and humans: implications of signals from the mesenchyme. Diabetologia 2000; 43:1083-92; PMID:11043853; http://dx.doi.org/10.1007/s00125005-1498
- Guo T, Landsman L, Li N, Hebrok M. Factors expressed by murine embryonic pancreatic mesenchyme enhance generation of insulin-producing cells from hESCs. Diabetes 2013; 62(5):1581-92; PMID:23305648
- Hörnblad A, Eriksson AU, Sock E, Hill RE, Ahlgren U. Impaired spleen formation perturbs morphogenesis of the gastric lobe of the pancreas. PloS One 2011; 6:e21753; PMID:21738788; http://dx.doi.org/10.1371/journal.po-ne.0021753
- Duvillié B, Attali M, Bounacer A, Ravassard P, Basmaciogullari A, Scharfmann R. The mesenchyme controls the timing of pancreatic {beta}-cell differentiation. Diabetes 2006; 55:582-9; PMID:16505219; http://dx.doi.org/10.2337/diabetes.55.03.06.db05-0839
- Landsman L, Nijagal A, Whitchurch TJ, VanderLaan RL, Zimmer WE, MacKenzie TC, Hebrok M. Pancreatic mesenchyme regulates epithelial organogenesis throughout development. PLoS biology 2011; 9:e1001143; PMID:21909240; http://dx.doi.org/10.1371/journal.pbio.1001143
- Sneddon JB, Borowiak M, Melton DA. Self-renewal of embryonic-stem-cell-derived progenitors by organ-matched mesenchyme. Nature 2012; 491:765-8. PMID:23041930
- Hecksher-Sorensen J, Watson RP, Lettice LA, Serup P, Eley L, De Angelis C, Ahlgren U, Hill RE. The splanchnic mesodermal plate directs spleen and pancreatic laterality, and is regulated by Bapx1/Nkx3.2. Development 2004; 131:4665-75; PMID:15329346; http://dx.doi.org/10.1242/dev.01364
- Asayesh A, Sharpe J, Watson RP, Hecksher-Sorensen J, Hastie ND, Hill RE, Ahlgren U. Spleen versus pancreas: strict control of organ interrelationship revealed by analyses of Bapx1−/− mice. Gen Dev 2006; 20:2208-13; PMID:16912273; http://dx.doi.org/10.1101/gad.381906
- Cras-Méneur C, Elghazi L, Czernichow P, Scharfmann R. Epidermal growth factor increases undifferentiated pancreatic embryonic cells in vitro: a balance between proliferation and differentiation. Diabetes 2001; 50:1571-9; PMID:11423478; http://dx.doi.org/10.2337/diabetes.50.7.1571
- Zhou Q, Law AC, Rajagopal J, Anderson WJ, Gray PA, Melton DA. A multipotent progenitor domain guides pancreatic organogenesis. Dev Cell 2007; 13:103-14; PMID:17609113; http://dx.doi.org/10.1016/j.devcel.2007.06.001
- Chen Y, Chen J, Chen H, Hong Z, Zhu X, Zhuo S, Chen Y, Chen J. Multiphoton microscopy as a diagnostic imaging modality for pancreatic neoplasms without hematoxylin and eosin stains. J Biomed Opt 2014; 19:96008; PMID:25216027; http://dx.doi.org/10.1117/1.JBO.19.9.096008
- Elghazi L, Cras-Méneur C, Czernichow P, Scharfmann R. Role for FGFR2IIIb-mediated signals in controlling pancreatic endocrine progenitor cell proliferation. Proc Natl Acad Sci U S A 2002; 99:3884-9; PMID:11891329; http://dx.doi.org/10.1073/pnas.062321799
- Ilegems E, Dicker A, Speier S, Sharma A, Bahow A, Edlund PK, Leibiger IB, Berggren PO. Reporter islets in the eye reveal the plasticity of the endocrine pancreas. Proceedings of the National Academy of Sciences of the United States of America 2013; 110:20581-6. PMID:24248353
- Gittes GK, Galante PE, Hanahan D, Rutter WJ, Debase HT. Lineage-specific morphogenesis in the developing pancreas: role of mesenchymal factors. JPEN J Parenter Enteral Nutr 1996; 122:439-47; PMID:8625795
- Puri S, Hebrok M. Dynamics of embryonic pancreas development using real-time imaging. Dev Biol 2007; 306:82-93; PMID:17448459; http://dx.doi.org/10.1016/j.ydbio.2007.03.003
- Guillemain G, Filhoulaud G, Da Silva Xavier G, Rutter GA, Scharfmann R. Glucose is necessary for embryonic pancreatic endocrine cell differentiation. J Biol Chem 2007; 282:15228-37; PMID:17376780; http://dx.doi.org/10.1074/jbc.M610986200
- Murtaugh LC. Pancreas and beta-cell development: from the actual to the possible. JPEN J Parenter Enteral Nutr 2007; 134:427-38; PMID:17185316
- Abdulreda MH, Faleo G, Molano RD, Lopez-Cabezas M, Molina J, Tan Y, Echeverria OA, Zahr-Akrawi E, Rodriguez-Diaz R, Edlund PK, et al. High-resolution, noninvasive longitudinal live imaging of immune responses. Proc Natl Acad Sci U S A 2011; 108:12863-8; PMID:21768391; http://dx.doi.org/10.1073/pnas.1105002108