ABSTRACT
Clinical islet transplantation has routinely been demonstrated to be an efficacious means of restoring glycemic control in select patients with autoimmune diabetes. Notwithstanding marked progress and improvements, the broad-spectrum application of this treatment option is restricted by the complications associated with intrahepatic portal cellular infusion and the scarcity of human donor pancreata. Recent progress in stem cell biology has demonstrated that the potential to expand new β cells for clinical transplantation is now a reality. As such, research focus is being directed toward optimizing safe extrahepatic transplant sites to house future alternative β cell sources for clinical use. The present study expands on our previous development of a prevascularized subcutaneous device-less (DL) technique for cellular transplantation, by demonstrating long-term (>365 d) durable syngeneic murine islet graft function. Furthermore, histological analysis of tissue specimens collected immediately post-DL site creation and acutely post-human islet transplantation demonstrates that this technique results in close apposition of the neovascularized collagen to the transplanted cells without dead space, thereby avoiding hypoxic luminal dead-space. Murine islets transplanted into the DL site created by a larger luminal diameter (6-Fr.) (n = 11), reversed diabetes to the similar capacity as our standard DL method (5-Fr.)(n = 9). Furthermore, glucose tolerance testing did not differ between these 2 transplant groups (p > 0 .05). Taken together, this further refinement of the DL transplant approach facilitates a simplistic means of islet infusion, increases the transplant volume capacity and may provide an effective microenvironment to house future alternative β cell sources.
Introduction
In a recent phase-3 clinical trial, islet transplantation has been demonstrated to restore glycemic control in patients with type-1 diabetes tormented by life-threatening hypoglycemia.Citation1 Since the initial success of the Edmonton Protocol in 2000,Citation2 islet transplantation success rates have steadily improved, whereby insulin-independence rates in at least 50% of recipients has been achieved beyond 5 y post-transplant in 6 International Centers.Citation3,4 Nevertheless, the achievement of single-donor engraftment success has been challenging, as the majority of recipients require at least 2 donors to achieve insulin-independence.Citation5,6
Various factors contribute to acute islet loss, particularly in the intraportal hepatic site, resulting in an estimated 70% loss of transplanted β-cell mass, namely due to the instant blood-mediated inflammatory reaction (IBMIR).Citation7 There is an ongoing need to identify a clinically relevant, non-blood exposed extrahepatic site to further optimize islet engraftment. Additionally, as insulin-producing stem cell-derived products and xenogeneic islet sources become available for clinical use, an engraftment site that facilitates graft monitoring and innocuous retrieval may be compulsory, as hepatectomy if required, would carry unwarranted clinical risk. The subcutaneous tissue has been identified as an alluring and clinically feasible option, as it possesses the characteristics of uncomplicated accessibility, potential for graft imaging and biopsy, as well as retrievability should graft excision be indicated.Citation8-10
In our laboratory, we have demonstrated successful restoration of euglycemia using mouse and human islets in pre-clinical animal models, by using the subcutaneous device-less (DL) transplant approach, which modifies the tissue under the skin into a highly vascularized engraftment site by temporally exploiting the natural foreign body reaction.Citation11 Furthermore, this site was also efficacious in reversing diabetes post-transplant in mice at a marginal islet dose.Citation12 However, development and refinement of this technique will likely be required to further optimize engraftment and establish this method as a clinically feasible approach for β cell replacement therapy. Herein, we sought to determine the long-term durability of syngeneic islet grafts within the DL site and further improve the technical properties of this subcutaneous islet transplant methodology.
Results
Long-term (>365 days) function of subcutaneous DL syngeneic islet grafts
The ability of the subcutaneous DL site to provide long-term and durable glycemic control post-transplant was evaluated in a syngeneic mouse model (n = 5). One month prior to transplant, the DL site was created via the implantation of a 5-Fr. nylon catheter. All recipients of islet-DL transplants (n = 5) became euglycemic, 5 of 5 (100%), within 4 weeks post-transplant. Glucose homeostasis was maintained until graft retrieval, 427 d post-transplant; at which time recipients rapidly became hyperglycemic confirming graft-dependent euglycemia ().
Figure 1. Long-term (>365 d) glycemic control and function of subcutaneous DL syngeneic islet grafts. (A) Non-fasting blood glucose measurements of euglycemic islet recipients (n = 5). Glucose homeostasis was maintained until graft excising 427 d post-transplant (arrow). (B) Blood glucose profiles post-glucose bolus during intraperitoneal glucose tolerance tests conducted on DL recipients 365 d post-transplant (5-Fr DL recipients: blue, n = 5) and on naïve non-diabetic control mice (naïve controls: black, n = 3). (C) Blood glucose area under the curve (AUC: mM*120 mins) analysis did not differ between control and DL recipients (p > 0 .05, 2-way unpaired t-test). Mice were administered 3 mg/kg 25% dextrose i.p. Blood glucose measurements were monitored at 0, 15, 30, 60, 90 and 120 min.
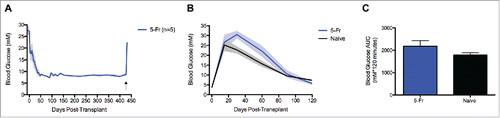
Intraperitoneal glucose tolerance testing (IPGTT) was performed on euglycemic recipients 365 d post-transplant. Mice in both DL and naïve non-diabetic control group (n = 3) demonstrated a prompt physiological glucose clearance response (). Moreover, there was no difference in mean area under the curve (AUC) ± s.e.m between the long-term DL islet recipients and naïve mice (AUC DL: 2175 ± 253 mM*120 min vs. Naive: 1783 ± 108 mM*120 min, p > 0 .05, unpaired 2-tail t-test, ).
Characterization of the DL microenvironment
The original rationale when creating the subcutaneous DL site was to utilize a catheter with a minimal outer diameter (OD), in an effort to decrease the luminal size created subsequent to catheter withdrawal. This approach, in theory, would reduce the amount of inherent “dead-space” surrounding the subsequent transplanted tissue. As such, our DL islet transplants conducted to date have implemented a 5-Fr. catheter with a 1.67mm OD and 0.89mm inner diameter (ID). However, empirical observations during the transplant procedure revealed an immediate collapse of the neovascularized tissue matrix induced by the implanted catheter. To confirm these observations, we implanted 5-Fr. nylon catheter segments for 4 weeks, at which time the catheter was withdrawn and the peri-implant tissue was immediately excised and fixed for histological evaluation. Sections from these tissue samples revealed a complete collapse of the vascularized lumen at what would be the time of transplantation (). To further confirm the prompt shrouding of the islets transplanted within the vascularized DL tissue, we transplanted research human islets in the DL site and subsequently retrieved and fixed the human islet graft 24 h post-transplant for histological assessment. Hematoxylin and eosin sections from these tissue samples demonstrated an acute cloaking of the human islets within the catheter-induced neovascularized tissue (). The lack of dead-space post-transplant and swift enveloping of the human islet in vascularized collagen tissue was confirmed with Mason's trichrome staining (). Cells within the DL lumen post-transplant stained positive for insulin and glucagon confirming the presence of β- and α-cells, respectively ().
Figure 2. Histological assessment of the prevascularized subcutaneous device-less site. (A) Hematoxylin and eosin staining illustrating the cross-section of collapse vascularized collagen lumen subsequent to catheter withdrawal (100x), (B) insert (200x). * Indicates lumen of DL transplant site.
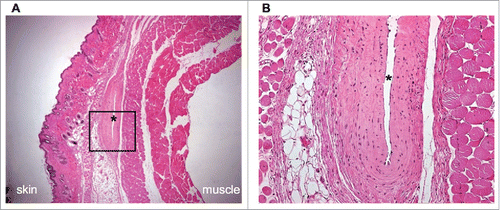
Figure 3. Histological analysis of human islet grafts 24 h post-transplant into the DL site. (A) Hematoxylin and eosin staining of a human islet graft cross-section, transplanted into the subcutaneous DL site; completely cloaked in vascularized tissue scaffold (100x), (B) insert (200x). (C) Mason's trichrome staining of human islet graft within the DL site surrounded by vascularized collagen (blue). (D) Fluorescent staining of the same DL cross-section staining for insulin (red), glucagon (green) and nuclei (blue) (200x). *Indicates human islets within the DL lumen. Scale bar represents 100μm.
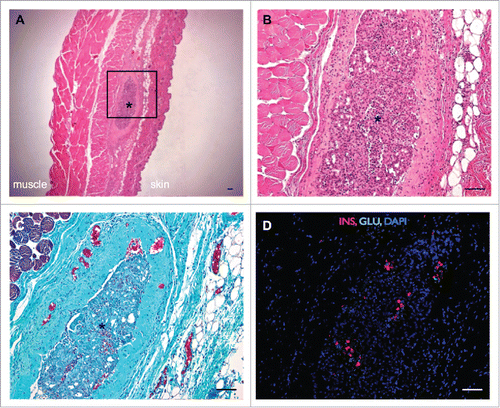
Optimizing the formation of the subcutaneous DL site
Based on the histological confirmation of a minimal dead-space evoked post-catheter withdrawal, we assessed the efficacy of syngeneic mouse islets transplanted into the DL site, created by a larger 6-Fr. nylon angiocatheter. The 6-Fr. catheter has an OD of 2.0mm and an ID of 0.97mm, which when compared to the 5-Fr. catheter of the same length increases the luminal volume (V = πr2h) available to house an islet preparation by 43%. From a practical standpoint, increasing the catheter size from 5-Fr. to 6Fr. for creating the subcutaneous DL site facilitates a simplistic means of transplanting (), as the increase in ID allows for PE-50 tubing (OD: 0.97mm) containing the islet preparation to penetrate the lumen of the indwelling catheter (). Subsequently, the catheter can be withdrawn along the PE-50, serving as a ‘guide-wire', thereby ensuring successful islet infusion, contained within the DL-vascularized lumen ().
Figure 4. Photographic overview of the subcutaneous, DL transplant procedure implementing 6-Fr. nylon catheter. (A) Angiocatheter implanted under skin 4 weeks prior to transplant. (B) Small incision made cranial to catheter. (C) Small section of implanted catheter is cut to expose vascularized tissue scaffold. (D) Catheter is exposed through superficial incision. (E) PE-50 tubing containing islet preparation inserted into the lumen of 6-Fr. (F) Implanted catheter is withdrawn long PE-50 tubing now within DL lumen. Islets are subsequently infused into resulting lumen.
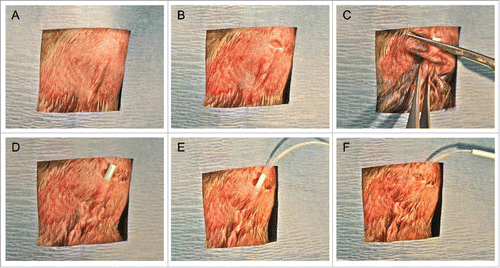
Increasing the DL lumen diameter does not hinder islet engraftment and function
To determine the impact of increasing the lumen volume on islet engraftment, and aid in confirming our hypothesis that the deleterious dead-space is minimized in our subcutaneous DL site transplant approach, we conducted DL syngeneic mouse islet transplants, using 5-Fr. (n = 9) or 6-Fr. (n = 11) nylon angiocatheters. All recipients of islet-DL transplants, regardless of catheter size, became euglycemic within one month post-transplant. Non-fasting blood glucose profiles were similar between both 5-Fr. and 6-Fr. recipients, while glucose homeostasis was maintained until graft retrieval (). Post-graft excision, all recipients swiftly became hyperglycemic confirming graft-dependent euglycemia ().
Figure 5. Glycemic control and function of subcutaneous DL syngeneic islet grafts created by 5-Fr. and 6-Fr. catheters. (A) Non-fasting blood glucose measurements of euglycemic DL islet recipients generated by 5-Fr. (n = 9, green) and 6-Fr. (n = 11, purple). Despite the larger luminal diameter 6-Fr. recipient demonstrated similar glucose homeostasis as 5-Fr. recipients. Glycemic control in both transplants groups was maintained until graft excising 60 d post-transplant (arrow). (B) Blood glucose profiles post-glucose bolus during intraperitoneal glucose tolerance tests conducted on DL recipients 60 d post-transplant (5-Fr DL recipients: green and 6-Fr. DL recipients, purple). (C) Blood glucose area under the curve (AUC: mM*120 mins) analysis did not differ between transplant groups (p > 0.05, 2-way unpaired t-test). Mice were administered 3 mg/kg 25% dextrose i.p. Blood glucose measurements were monitored at 0, 15, 30, 60, 90 and 120 min.
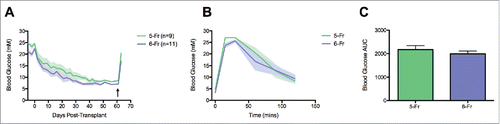
Glucose tolerance tests were performed on euglycemic recipients 60 d post-transplant. Mice in both DL transplant groups exhibited rapid glucose clearance (). Furthermore, no difference in mean area under the curve (AUC) ± s.e.m was observed between the 5-Fr. and 6Fr. DL islets recipients (5Fr. DL: 2169 ± 172 mM*120 min vs. Six-Fr. DL: 1988 ± 126 mM*120 min, p>0 .05, unpaired 2-tail t-test, ).
Discussion
While the intrahepatic infusion of islets remains the only transplant approach to routinely restore glycemic control to type 1 diabetic patients, this procedure is restricted due to its associated inflammatory reactions and procedural risks. As insulin-producing stem cell-derived therapies become more of a reality, and with the development of pathogen-free xenogeneic β cell sources, the inability to effectively monitor and retrieve intrahepatic cellular grafts limit the liver's capacity to safely house such therapeutic cell products. Therefore, significant research continues to be directed toward the evaluation of clinically feasible extrahepatic transplant approaches.Citation13
The subcutaneous space has appealing potential as a surrogate to intraportal infusion for cellular transplantation, due to its superficial characteristics that facilitate graft imaging, biopsy and retrieval. However, among others we have distinctively demonstrated that islets transplanted into an unmodified subcutaneous pocket universally fail to restore glycemic control post-transplant.Citation8,12,14-16
It has been suggested that the lack of graft viability post-subcutaneous transplantation is due to the tissue's relatively low superficial tissue oxygen tension compared to other vascularized organs.Citation16 To thwart endogenous hypoxia and improve islet engraftment, subcutaneous engraftment strategies have employed growth factors,Citation17 scaffolds,Citation18-20 encapsulation polymers,Citation15,21 immunoisolating and non-immunoisolating devices,Citation11,22-26 and oxygen delivery technologies.Citation17,24 While attractive due to the potential of avoiding systemic immunosuppression, a drawback of immunoisolating devices is the physical separation between host nutrient supply and the therapeutic cells within the device. As such, this approach limits metabolic exchange to passive diffusion and a reliance on a adequate surface area to volume ratio.Citation27 A further limitation of subcutaneous technologies that rely on permanently implanted constituents, is the lifetime chronic foreign body reaction evoked at the biomaterial - graft interface that may hamper nutrient exchange and cellular engraftment.Citation27,28
Our laboratory has developed a subcutaneous transplant approach designed to exploit the innate foreign-body response, thereby promoting local neovascularization. As a result, we have demonstrated efficacy in reversing diabetes in mice utilizing a multitude of human and murine islet transplant models.Citation11,12 The subcutaneous tissue is transformed into vascularized microenvironment for cellular engraftment through the temporary implantation of a nylon catheter. The foreign body reaction to the implanted catheter concludes upon catheter withdrawal yielding a vascularized collagen matrix into which islets are infused. In the present study, we have further demonstrated that euglycemia achieved by transplanting syngeneic murine islets into the DL site, is durable and negates graft attrition as indicated by recipient insulin independence for greater than 1 y post-transplant.
Previously, we hypothesized that, compared to permanently implant devices, our DL approach does not resist the intrinsic subcutaneous interstitial pressure, enabling the immediate collapse of the neovascularized tissue thereby reducing the presences of hypoxic ‘dead-space’ surrounding the transplanted cells.Citation12 Herein, we confirmed the immediate collapse of the vascularized tissue matrix that once shrouded the implanted catheter, via histological samples collected promptly post-catheter withdrawal. Furthermore, we verified the lack of dead-space within the transplant lumen and the rapid shrouding of transplanted islets within the DL site with vascularized collagen through the histological analysis of human islets grafts collected 24 h post-DL transplant. Based on these observations, we postulated and validated that a larger diameter nylon catheter could be utilized in the creation of the DL site. This approach indeed facilitates a simplistic means of cellular delivery into the vascularized lumen while increasing the transplantable volume by 43% compared to previous methods of creating the DL site. Importantly, beyond the procedural advantages achieved by increasing the DL luminal diameter, we validated that this increase did not hinder islet engraftment and function; whereas in previous studies conducted with rubber-based catheters of a larger diameter (8 French), we failed to reverse diabetes in mice even when 3 times the islet dose was transplanted.Citation11 Syngeneic mouse islets transplanted into the DL created by a 6Fr. catheter restored glycemic control to the same capacity achieved by a smaller 5Fr. catheter. Furthermore, no difference in glucose tolerance testing between recipients of both transplants groups was observed, indicating that a larger lumen diameter can facilitate islet engraftment, thus allowing for rapid glucose sensing and subsequent clearance.
The present study complements and expands our previous observationsCitation11,12 by establishing that the subcutaneous DL transplant approach supports long-term durable islet graft function and is not limited by luminal dead-space constraints. Continued optimization of this transplant technique may indeed translate to improved outcomes of clinical insulin independence rates in addition to potentially being an applicable and safe strategy to transplant stem cell-derived cellular therapies to patients. The utility of this transplant technique will be evaluated in a first-in-human trial currently being planned at the University of Alberta in the capacity of sentinel islet graft concurrent to a standard intraportal islet infusion.
Materials and methods
Human islets
Human islets were prepared by the Clinical Islet Laboratory at Alberta Health Services. Deceased donor pancreata were processed for islet isolation with appropriate ethical approval and consent obtained from next-of-kin of the donor. Islets were isolated from 1 donor pancreata, implementing a modified Ricordi technique.Citation29,30 Permission for these studies was granted by the Health Research Ethics Board of the University of Alberta, Edmonton, Alberta, Canada. Upon receiving the human islet preparation, islet aliquots (± 10%) were counted and placed into standard culture media for 1 h at 37°C and 5% CO2 prior to transplantation. Standard culture media (CMRL-1066, Mediatech, Manassas, VA, USA) contains fetal bovine serum (10%), L-glutamine (100 mg/l), penicillin (112 kU/l), streptomycin (112 mg/l) and HEPES (25 mM) at pH 7.4.
Mouse islet isolation and culture
Pancreatic islets were isolated from 8 to 12 week old male C57BL/6 mice (Jackson Laboratories, Canada). Animals were housed under conventional conditions having access to food and water ad libitum. Mouse care was in accordance with the guidelines approved by the Canadian Council on Animal Care. Prior to pancreatectomy, the common bile duct was cannulated with a 30G needle and the pancreas was distended with 0.125 mg/ml cold Liberase TL Research Grade enzyme (Roche Diagnostics, Laval, QC, CA) in Hank's Balanced Salt Solution (Sigma-Aldrich Canada Co., Oakville, ON, CA). Islets were isolated by digesting the pancreases in a 50 ml tube placed in a 37°C water bath for 14 min with light agitation. Following the pancreatic digestion phase, islets were purified using histopaque-density gradient centrifugation (1.108, 1.083 and 1.069 g/ml, Sigma-Aldrich Canada Co., Oakville, ON, Canada). Isolated mouse islet aliquots (± 10%) were then counted placed in standard culture media 1 h at 37°C and 5% CO2 prior to transplantation. Standard culture media (CMRL-1066, Mediatech, Manassas, VA, USA) contains fetal bovine serum (10%), L-glutamine (100 mg/l), penicillin (112 kU/l), streptomycin (112 mg/l) and HEPES (25 mM) at pH 7.4.
Diabetes induction and islet transplantation
Diabetes was chemically induced 1 week prior to transplantation in male 20-25g recipient mice, either C57BL/6 (syngeneic studies) or B6-RAG−/−(B6.129S7-Rag1tm1Mom/J) (human islet study) by an intraperitoneal Streptozotocin (180 mg/kg) (Sigma-Aldrich Canada Co., Oakville, ON, Canada), in acetate phosphate buffer, pH 4.5. Diabetes was confirmed when blood glucose levels exceeded 15 mM for 2 consecutive daily readings.
For acute human islet transplants, islets were counted and transplanted into the prevascularized subcutaneous device-less (DL) site,Citation11,12 at a marginal islet dose of 500 ± 10% per human islet equivalents per diabetic recipient. The DL site was created approximately 1 month prior to transplant by implanting a 2cm segment of either 5-French (Fr.) or 6-French (Fr.) nylon angiocatheter (Torcon NB® Advantage Beacon®tip Cook Medical, Indiana, USA) subcutaneously into the lower left quadrant of recipient mice. Syngeneic, mouse islets transplanted into 2 groups: 1) 5-Fr. DL or 2) 6-Fr. DL, both groups receiving a standard islet dose of 500 ± 10% mouse islets with purity of 90 ± 5%. Both human and mouse islets were aspirated into polyethylene (PE-50) tubing using a micro-syringe, and centrifuged into a pellet suitable for transplantation. Prior to recovery, all recipients received a 0.1 mg/kg subcutaneous bolus of buprenorphine.
Evaluation of islet graft function
Human islet recipients were not evaluated for graft function as their DL-islet grafts were procured 24 h post-transplant for histological analysis. Syngeneic mouse islet DL transplant efficacy was assessed twice per week in recipients through non-fasting blood glucose measurements, using a portable glucometer (OneTouch Ultra 2, LifeScan, Canada) in both groups tested. Graft function and reversal of diabetes was defined as 2 consecutive readings <11 .1 mM and maintained until study completion. In addition, intraperitoneal glucose tolerance tests (IPGTTs) were conducted post-transplant to assess metabolic function and glucose clearance. Recipient mice were fasted overnight prior to receiving an intraperitoneal dextrose bolus at a dose of 3 g/kg. Blood glucose levels were evaluated at baseline (Time 0), 15, 30, 60, 90 and 120 min post-injection. Blood glucose area under the curve (AUC-blood glucose: mM*120 min) was calculated and analyzed between transplant groups.
DL islet transplants were retrieved by subcutaneous graft excision as a means to confirm graft dependent euglycemia, as previously describedCitation12. Non-fasting blood glucose measurements were monitored for 7 d following graft removal to confirm hyperglycemia.
Histological evaluation
Immunofluorescence with anti-insulin and anti-glucagon antibodies was used to identify the presence of pancreatic β-cells and α-cells respectively, within acutely transplanted human islet DL grafts. Briefly, following deparaffinization and antigen heat retrieval, the graft sections were washed with phosphate buffered saline supplemented (PBS) with 1% goat serum, followed by blocking with 20% goat serum in PBS for 30 min. Sections were then treated with a primary antibody of guinea pig anti-pig insulin (Dako A0564) diluted 1:100 (PBS with 1% goat serum) and rabbit anti-glucagon (Abcam, ab43837) diluted 1:200 (PBS with 1% goat serum) for 2 h at 4°C. Samples were rinsed with PBS with 1% goat serum followed by secondary antibody treatment consisting of goat anti-guinea pig (Alexa 568) diluted 1:500 (PBS with 1% goat serum), and goat anti-rabbit (Vector Fl-1000) diluted 1:500 (PBS with 1% goat serum) for 1 h at room temperature. Samples were rinsed with PBS and counter stained with DAPI in anti-fade mounting medium (ProLong®, LifeTechnologies). Using a fluorescent microscope, the resulting microphotographs were taken using the appropriate filter with AxioVision imaging software. Representative sections were also stained with Masson's trichrome to identify collagen and hematoxylin and eosin for cytoarchitecture.
Statistical analysis
Non-fasting blood glucose and AUC-blood glucose data are represented as the mean ± standard error of mean (s.e.m.). AUC-blood glucose analysis for glucose tolerance test data was conducted by unpaired 2-tailed t-test. P < 0 .05 was considered significant.
Abbreviations
AUC | = | Area under curve |
DL | = | Device-less |
Fr | = | French |
IBMIR | = | Instant blood-mediated inflammatory reaction |
I.P. | = | Intraperitoneal |
IPGTT | = | Intraperitoneal glucose tolerance test |
PBS | = | Phosphate buffered saline |
PE | = | Polyethylene |
SEM | = | Standard error of mean |
Disclosure of potential conflicts of interest
No potential conflicts of interest were disclosed.
Notes on contributors
A.R.P participated in the research design, performance of the research, data analysis and writing of the manuscript. A.B, R.P, B.G.L, J.W and Y.R participated in the performance of the research and writing of the manuscript. T.K. provided human islets and review of the manuscript. A.M.J.S participated in research design and writing of the manuscript.
Funding
This work was supported in part by the Diabetes Research Institute Foundation of Canada (DRIFCan), Collaborative Research & Innovation Opportunities (CRIO) – Alberta Innovates- Health Solutions (AIHS)(No. 201201154) and Canadian Institutes of Health Research (CIHR) – Proof of Principle (POP)(No. 144255). Dr. A.M James Shapiro is supported through a Senior Clinical Scholarship from Alberta Innovates - Health Solutions (AIHS), and holds a Canada Research Chair in Transplantation Surgery and Regenerative Medicine funded through the Government of Canada. A.M.J.S is also supported by AIHS CRIO Team Award #201201154. Dr. Andrew R. Pepper is supported primarily through an AIHS Post-Doctoral Fellowship, and further supplemented through an AIHS CRIO Team Award. Antonio Bruni is supported through scholarships from the University of Alberta, the Alberta Diabetes Institute and the Alberta Diabetes Foundation. Dr. Boris Gala-Lopez is supported through a Clinician Fellowship from AIHS.
ORCID
Antonio Bruni http://orcid.org/0000-0002-7984-7030
Yasmin Rafiei http://orcid.org/0000-0002-2737-3742
John Wink http://orcid.org/0000-0003-4716-7281
Tatsuya Kin http://orcid.org/0000-0002-5179-6067
A. M. James Shapiro http://orcid.org/0000-0002-6215-0990
References
- Ricordi C, Goldstein JS, Balamurugan AN, Szot GL, Kin T, Liu C, Czarniecki CW, Barbaro B, Bridges ND, Cano J, et al. NIH-sponsored clinical islet transplantation consortium phase 3 trial: manufacture of a complex cellular product at eight processing facilities. Diabetes 2016 Nov; 65(11): 3418-3428.
- Shapiro AM, Lakey JR, Ryan EA, Korbutt GS, Toth E, Warnock GL, Kneteman NM, Rajotte RV. Islet transplantation in seven patients with type 1 diabetes mellitus using a glucocorticoid-free immunosuppressive regimen. N Engl J Med 2000; 343:230-8; PMID:10911004; http://dx.doi.org/10.1056/NEJM200007273430401
- Bruni A, Gala-Lopez B, Pepper AR, Abualhassan NS, Shapiro AJ. Islet cell transplantation for the treatment of type 1 diabetes: recent advances and future challenges. Diabetes Metab Syndr Obes 2014; 7:211-23; PMID:25018643
- Shapiro AMJ, Ricordi C. Islet cell transplantation procedure and surgical technique. In: AD Kirk SJK, CP Larsen, JC Madsen, TC Pearson and SA Webber, ed. Textbook of Organ Transplantation Oxford, UK: John Wiley & Sons, 2014
- Hering BJ, Kandaswamy R, Ansite JD, Eckman PM, Nakano M, Sawada T, Matsumoto I, Ihm SH, Zhang HJ, Parkey J, et al. Single-donor, marginal-dose islet transplantation in patients with type 1 diabetes. JAMA 2005; 293:830-5; PMID:15713772; http://dx.doi.org/10.1001/jama.293.7.830
- Hering BJ, Kandaswamy R, Harmon JV, Ansite JD, Clemmings SM, Sakai T, Paraskevas S, Eckman PM, Sageshima J, Nakano M, et al. Transplantation of cultured islets from two-layer preserved pancreases in type 1 diabetes with anti-CD3 antibody. Am J Transplant 2004; 4:390-401; PMID:14961992; http://dx.doi.org/10.1046/j.1600-6143.2003.00351.x
- Eich T, Eriksson O, Lundgren T. Nordic Network for Clinical Islet T. Visualization of early engraftment in clinical islet transplantation by positron-emission tomography. N Engl J Med 2007; 356:2754-5; PMID:17596618; http://dx.doi.org/10.1056/NEJMc070201
- Pepper AR, Gala-Lopez B, Ziff O, Shapiro AM. Revascularization of transplanted pancreatic islets and role of the transplantation site. Clin Dev Immunol 2013; 2013:352315; PMID:24106517; http://dx.doi.org/10.1155/2013/352315
- Rajab A. Islet transplantation: alternative sites. Curr Diab Rep 2010; 10:332-7; PMID:20665132; http://dx.doi.org/10.1007/s11892-010-0130-6
- Veriter S, Gianello P, Dufrane D. Bioengineered sites for islet cell transplantation. Curr Diab Rep 2013; 13:745-55; PMID:23959794; http://dx.doi.org/10.1007/s11892-013-0412-x
- Pepper AR, Gala-Lopez B, Pawlick R, Merani S, Kin T, Shapiro AM. A prevascularized subcutaneous device-less site for islet and cellular transplantation. Nat Biotechnol 2015; 33:518-23; PMID:25893782; http://dx.doi.org/10.1038/nbt.3211
- Pepper AR, Pawlick R, Bruni A, Gala-Lopez B, Wink J, Rafiei Y, Bral M, Abualhassan N, Shapiro AM. Harnessing the foreign body reaction in marginal mass device-less subcutaneous islet transplantation in mice. Transplantation 2016; 100:1474-9; PMID:27136258; http://dx.doi.org/10.1097/TP.0000000000001162
- Tomei AA, Villa C, Ricordi C. Development of an encapsulated stem cell-based therapy for diabetes. Expert Opin Biol Ther 2015; 15:1321-36; PMID:26156291; http://dx.doi.org/10.1517/14712598.2015.1055242
- Kemp CB, Knight MJ, Scharp DW, Ballinger WF, Lacy PE. Effect of transplantation site on the results of pancreatic islet isografts in diabetic rats. Diabetologia 1973; 9:486-91; PMID:4204180; http://dx.doi.org/10.1007/BF00461694
- Lacy PE, Hegre OD, Gerasimidi-Vazeou A, Gentile FT, Dionne KE. Maintenance of normoglycemia in diabetic mice by subcutaneous xenografts of encapsulated islets. Science 1991; 254:1782-4; PMID:1763328; http://dx.doi.org/10.1126/science.1763328
- Sakata N, Aoki T, Yoshimatsu G, Tsuchiya H, Hata T, Katayose Y, Egawa S, Unno M. Strategy for clinical setting in intramuscular and subcutaneous islet transplantation. Diabetes Metab Res Rev 2014; 30:1-10
- Ludwig B, Rotem A, Schmid J, Weir GC, Colton CK, Brendel MD, Neufeld T, Block NL, Yavriyants K, Steffen A, et al. Improvement of islet function in a bioartificial pancreas by enhanced oxygen supply and growth hormone releasing hormone agonist. Proc Natl Acad Sci U S A 2012; 109:5022-7; PMID:22393012; http://dx.doi.org/10.1073/pnas.1201868109
- Abualhassan N, Sapozhnikov L, Pawlick RL, Kahana M, Pepper AR, Bruni A, Gala-Lopez B, Kin T, Mitrani E, Shapiro AM. Lung-derived microscaffolds facilitate diabetes reversal after mouse and human intraperitoneal islet transplantation. PLoS One 2016; 11:e0156053; PMID:27227978; http://dx.doi.org/10.1371/journal.pone.0156053
- Kim JS, Lim JH, Nam HY, Lim HJ, Shin JS, Shin JY, Ryu JH, Kim K, Kwon IC, Jin SM, et al. In situ application of hydrogel-type fibrin-islet composite optimized for rapid glycemic control by subcutaneous xenogeneic porcine islet transplantation. J Control Release 2012; 162:382-90; PMID:22820449; http://dx.doi.org/10.1016/j.jconrel.2012.07.018
- Perez-Basterrechea M, Briones RM, Alvarez-Viejo M, Garcia-Perez E, Esteban MM, Garcia V, Obaya AJ, Barneo L, Meana A, Otero J. Plasma-fibroblast gel as scaffold for islet transplantation. Tissue Eng Part A 2009; 15:569-77; PMID:18694292; http://dx.doi.org/10.1089/ten.tea.2008.0088
- Juang JH, Bonner-Weir S, Ogawa Y, Vacanti JP, Weir GC. Outcome of subcutaneous islet transplantation improved by polymer device. Transplantation 1996; 61:1557-61; PMID:8669096; http://dx.doi.org/10.1097/00007890-199606150-00001
- Dufrane D, Goebbels RM, Gianello P. Alginate macroencapsulation of pig islets allows correction of streptozotocin-induced diabetes in primates up to 6 months without immunosuppression. Transplantation 2010; 90:1054-62; PMID:20975626; http://dx.doi.org/10.1097/TP.0b013e3181f6e267
- Kumagai-Braesch M, Jacobson S, Mori H, Jia X, Takahashi T, Wernerson A, Flodström-Tullberg M, Tibell A. The TheraCyte device protects against islet allograft rejection in immunized hosts. Cell Transplant 2013; 22:1137-46; PMID:23043940; http://dx.doi.org/10.3727/096368912X657486
- Ludwig B, Reichel A, Steffen A, Zimerman B, Schally AV, Block NL, Colton CK, Ludwig S, Kersting S, Bonifacio E, et al. Transplantation of human islets without immunosuppression. Proc Natl Acad Sci U S A 2013; 110:19054-8; PMID:24167261; http://dx.doi.org/10.1073/pnas.1317561110
- Sorenby AK, Kumagai-Braesch M, Sharma A, Hultenby KR, Wernerson AM, Tibell AB. Preimplantation of an immunoprotective device can lower the curative dose of islets to that of free islet transplantation: studies in a rodent model. Transplantation 2008; 86:364-6; PMID:18645504; http://dx.doi.org/10.1097/TP.0b013e31817efc78
- Pepper AR, Pawlick R, Gala-Lopez B, MacGillivary A, Mazzuca DM, White DJ, Toleikis PM, Shapiro AM. Diabetes is reversed in a murine model by marginal mass syngeneic islet transplantation using a subcutaneous cell pouch device. Transplantation 2015; 99:2294-300; PMID:26308506
- Wilson JT, Chaikof EL. Challenges and emerging technologies in the immunoisolation of cells and tissues. Adv Drug Deliv Rev 2008; 60:124-45; PMID:18022728; http://dx.doi.org/10.1016/j.addr.2007.08.034
- Anderson JM, Rodriguez A, Chang DT. Foreign body reaction to biomaterials. Semin Immunol 2008; 20:86-100; PMID:18162407; http://dx.doi.org/10.1016/j.smim.2007.11.004
- Kin T. Islet isolation for clinical transplantation. Adv Exp Med Biol 2010; 654:683-710; PMID:20217520; http://dx.doi.org/10.1007/978-90-481-3271-3_30
- Ricordi C, Lacy PE, Finke EH, Olack BJ, Scharp DW. Automated method for isolation of human pancreatic islets. Diabetes 1988; 37:413-20; PMID:3288530; http://dx.doi.org/10.2337/diab.37.4.413