ABSTRACT
Objectives: Although it has been shown that exposure to environmental endocrine disruptors (EDCs) has been implicated as a potential risk factor for metabolic disease, information on adverse effect of chronic low-dose exposure to nonylphenol (NP), on the development and progress of type 2 diabetes mellitus (T2DM) is scarce. NP, as an EDC, is a ubiquitous degradation product of nonylphenol polyethoxylate (NPE) that is primarily used in cleaning and industrial processes. Method: Eighty Sprague-Dawley rats were assigned into 8 groups (n = 10 per group): rats fed a normal-diet (ND) as the control (C-ND); rats fed a normal diet and were gavaged with NP at a dose level of 0.02 μg/kg/day (NP-L-ND), 0.2 μg/kg/day (NP-M-ND) or 2 μg/kg/day (NP-H-ND), respectively; rats fed a high-sucrose/high-fat diet (HSHFD) as the HSHFD control (C-HSHFD); rats fed a HSHFD and were gavaged with NP at a dose level of 0.02 μg/kg/day (NP-L-HSHFD), 0.2 μg/kg/day (NP-M-HSHFD) or 2 μg/kg/day (NP-H-HSHFD), respectively. Result: On day 180, the rats in the groups treated with NP-M-HSHFD and NP-H-HSHFD showed significant increases in body weight (p < 0.05) in comparison with the C-ND group. Fast blood glucose (FBG) level in the NP-M-HSHFD and NP-H-HSHFD groups was higher than that in the C-ND group (F = 96.17, p < 0.001). The fast serum insulin (FINS) level of rats was lower in both the NP-M-HSHFD and NP-H-HSHFD groups compared with the C-ND group (F = 145.56, p < 0.001). Serum leptin (LEP) level in both the NP-M-HSHFD and NP-H-HSHFD groups was lower when compared with the C-ND group (F = 34.62, p < 0.001). The effect of NP at the dose level of 0.2 μg/kg/day on FBG, serum FINS and LEP levels in rats was greatest among the treatment groups (p < 0.05). Oral glucose tolerance test showed increased area under the curve (AUC) in treatment groups at week 12 (p < 0.05). A decrease of pancreatic islet numbers and size was exhibited in the pancreatic tissue of NP-M-HSHFD and NP-H-HSHFD treated rats compared with C-ND treated rats. Co-exposure to NP and HSHFD causes inflammatory changes histologically. Conclusion: Chronic low-dose exposure to NP might induce impaired glucose tolerance, which further lead to insulin resistance, and pancreatic β cell insulin secretion deficiency, ultimately increase the risk of T2DM. Moreover, additive toxic effects of NP and HSHFD on pancreatic beta-cell function and glucose metabolism have been identified in rats as well.
Introduction
Diabetes, one of the most prevalent chronic diseases, can be simply classified into type 1 diabetes mellitus and type 2 diabetes mellitus. Globally, as of the year 2013, an estimated 382 million people are living with diabetes, and this number is expected to reach 592 million by 2035,Citation1 the vast majority of the cases is type 2 diabetes mellitus (T2DM). According to the most recent surveillance data in China, the overall prevalence of diabetes mellitus among adult population is approximately 11.6%,Citation2 which is far higher than the estimated global prevalence. T2DM, mainly appearing in adulthood, is characterized by insulin resistance and a relative deficiency of insulin secretion.Citation3 A common assumption is that environmental risk factor exposure and lifestyle changes have led to the T2DM epidemic. Giving thought to the number of patients impacted by T2DM and its significant morbidity and mortality and economic costs, there is considerable interest in understanding the contribution of non-traditional risk factors to the diabetes epidemic, especially environmental endocrine disruptors (EDCs).
Recently, certain qualitative studies have reported that exposure to EDCs as a risk factor for diabetes mellitus development. Exposure to persistent organic pollutants (POPs) mixtures can increase T2DM risk in humans.Citation4 A positive association between Bisphenol A exposure and serum insulin level and β-cell function as well as insulin resistance was discovered among U.S. adults.Citation5 Repeated low dose of dioxin (500 ng/kg bw), gavaged, reduced glucokinase gene expression, predicting a rise in blood glucose levels on C57BL/6 mice.Citation6
Nonylphenol (NP) is a well-known EDC with weak estrogenic activity, which is widely used in cleaning and industrial processes, and residential and commercial cleaning products.Citation7 Such wide application and use makes NP frequently detected in the environment. Our prior study testified that the concentration for NP measured in Xiangjiang River in Zunyi city of China ranged from 0.174 to 3.411 μg/L with a mean value of 1.73 μg/L, maintaining at a higher level compare to the developed countries and other civil citiesCitation8. Exposure to NP is nearly ubiquitous. NP was detectable in human blood serum,Citation9 urine,Citation10 adipose tissue,Citation11 liver and brain.Citation12 There is strong evidence demonstrating greater risk for NP with increasing risk of endocrine,Citation13 reproductive,Citation14 and immuneCitation15 as well as nervousCitation16 system diseases in animals and humans. However, the potential impacts of chronic low-dose exposure to NP on the glucose metabolism and pancreatic endocrine function have scarcely been reported. Therefore, in this study, we employed a developmental NP exposure paradigm in rats to test the hypothesis that chronic low-dose exposure to NP caused a predisposition to T2DM. Furthermore, any additive effect of a high-sucrose/high-fat diet on the toxic effects induced by NP was also evaluated.
Material and method
Ethics statement
All procedures were conducted at Zunyi Medical University and were performed in strict accordance with the guidelines and regulations set forth by the Zunyi Medical University ethics committee with full approval from its Animal Care and Use Committee.
Reagents
The NP was purchased from the Tokyo Chemical Co., Ltd (Tokyo, Japan). Automatic biochemical analyzer was purchased from Beckman Coulter (Villepinte, France). High-speed centrifuge was purchased from Wilcon-Xiangying (Changsha, China). Glucometer (GA-3) was purchased from Sannuo (Changsha, China). Rat insulin ELISA kit (No. E-EL-R2466c) and rat leptin ELISA kit (No. E-EL-R0582c) were purchased from Elabscience Biotechnology Co., Ltd (Wu Han, China). All other chemicals were commercially available. All chemical purities were at least 99%.
Animals and treatments
Eighty Sprague-Dawley (SD) rats from the Animal Center of the Third Military Medical University (Chongqing, China) were fed commercial rat chow for 1 weeks. The 80 rats with half males and half females were assigned into 8 groups (n = 10 per group), and each group was treated with a specific testing sample for 180 days. The 80 rats were orally exposed to NP or to groundnut oil alone (vehicle control, 2 ml/kg/day). The first group was fed a normal diet (SCXK2012-0012, the Third Affiliated Hospital of Third Military Medical University) and received gavage with groundnut oil alone, and served as the normal-diet (ND) control (C-ND); the second, third and fourth groups were fed a normal diet and were gavaged with NP, which was dissolved in groundnut oil, at a dose level of 0.02 μg/kg/day (NP-L-ND), 0.2 μg/kg/day (NP-M-ND) or 2 μg/kg/day (NP-H-ND), respectively; the fifth group was fed a high-sucrose/high-fat diet (HSHFD) (SCXK2012-0012, The Third Affiliated Hospital of Third Military Medical University), and served as the high-sucrose/high-fat-diet control (C-HSHFD); the sixth, seventh and eighth groups were fed a high-sucrose/high-fat diet and were gavaged with NP, which was dissolved in groundnut oil, at a dose level of 0.02 μg/kg/day (NP-L-HSHFD), 0.2 μg/kg/day (NP-M-HSHFD) or 2 μg/kg/day (NP-H-HSHFD), respectively. The rats fasted for 10 hrs before gavaging with 5 ml/kg testing sample in every morning. The compositions of the ND and HSHFD were shown in . The animals were maintained under controlled temperature (20 ± 1°C) and humidity (60±5%), on a 12-hr light (09:00–21:00 hr), 12-hr dark (21:00–09:00 hr) cycle. Food and water were freely available. All procedures involving the use of laboratory animals were in accordance with Animal Care and Use Guidelines in China.
Table 1. The compositions of the normal diet and high-sucrose/high-fat diet.
Detection of fast blood glucose (FBG)
The animals were kept fasting for 10 hr overnight. In all eight groups' blood for estimation of fast blood glucose were drawn on day 60, day 120, and day 180 from the tail vein of the rats.
Oral glucose tolerance test (OGTT)
The rats were selected for OGTT test after a 12-h overnight fast at week 12. The animals were fed the glucose solution with the dose of 2 mg/kg body weight (BW). The baseline glucose level was measured by glucometer. Serum glucose of blood sample from tail vein was estimated by using glucometer at 0, 30, 60, and 120 minutes. After the data were collected the area under curve of OGTT (OGTT-AUC) was calculated using the trapezoidal method.
Biochemical studies
On day 180, the rats were killed by decapitation after pentobarbital sodium was given at a dose of 20 mg/kg intraperitoneal injection to induce anesthesia. Blood (8 ml) was collected from the abdominal aorta in heparinized vials, and the plasma was isolated by centrifugation and stored at -80°C until analyzed by enzyme-linked immuno sorbent assay (ELISA) for plasma insulin using a rat insulin ELISA kit, and plasma leptin using a rat leptin ELISA kit.
Histological analysis
The animals underwent pancreatectomy on day 180. One sample of tail pancreatic tissue from each group were taken and fixed in 10% formaldehyde at room temperature. Each formalin-fixed and paraffin-embedded specimen was cut into sections 3 µm thick. Staining was performed with hematoxylin-eosin (H&E) for routine histological observations.
Statistical analysis
The statistical analyses were performed with SPSS software, version 18.0 for Windows (SPSS Inc., Chicago, IL). Values of all variables are presented as mean and standard deviation. A factorial design was used to investigate the interaction effect between nonylphenol and high sucrose-high fat diet, if there is no interaction effect, significance between groups was evaluated by one way analysis of variance (ANOVA) followed by a Bonferroni post hoc test. A P-value <0.05 was considered as the level of statistical significance.
Result
Body weight
No interactions between NP and HSHFD approached significance. On days 120 (p = 0.026) and 180 (p = 0.019), exposure to NP-only (i.e., NP-L-ND, NP-M-ND, NP-H-ND) significantly increased body weight.
On day 60 (P = 0.041, P = 0.024), day 120 (P = 0.014, P = 0.016) and day 180 (P = 0.025, P = 0.008), the rats in the groups treated with NP-M-HSHFD and NP-H-HSHFD showed significant increases in body weight in comparison with the C-ND group, respectively. On day 180, exposure to NP-H-HSHFD significantly increased body weight when compared with the NP-L-ND (P = 0.029) and NP-M-ND (P = 0.042) groups ().
Table 2. The effect of NP on body weight in rats on day 180 (g, n = 10, χ ± S).
Fast blood glucose
There was no significant interaction between NP and HSHFD on day 60. However, co-exposure to NP and HSHFD induced an increase in FBG level of rats when compared with the control groups on days 120 and 180 (p = 0.45, 0.19). Moreover, the effect of NP-only (i.e., NP-L-ND, NP-M-ND, NP-H-ND) exposure on FBG level of rats was greater than that of C-HSHFD exposure (p < 0.001).
On day 180, FBG levels in both NP-only exposure and NP plus HSHFD exposure groups increased with an increase of NP exposure dose in a dose response manner. The effect of NP at the dose of 0.2 μg/kg/day on the FBG level of rats was greatest among the treatment groups (p < 0.05). the results in multiple comparisons among the 8 groups shown that there were no significant differences between NP-L-ND and NP-H-ND groups (p = 0.052), NP-L-ND and NP-L-HSHFD groups (p = 0.125), NP-M-ND and NP-H-HSHFD groups (p = 0.861), and NP-H-ND and NP-L-HSHFD groups (p = 0.671), the results in the rest of multiple comparisons among the treatment groups shown significant differences (p < 0.05, ).
Fast serum insulin (FINS) level
The effect that co-exposure to NP and HSHFD caused the reduction in the FINS level of rats was more remarkable than that of NP-only (p = 0.002) or C-HSHFD (p < 0.001) exposure on day 180. Furthermore, the effect of NP-only exposure on FINS level of rats was more remarkable than that of C-HSHFD exposure (p < 0.001) .
There was significant difference in the FINS levels among the 8 treatment groups (F = 145.56, P < 0.001). In multiple comparison, when compared to the C-ND group, FINS levels were lower in the NP-L-ND (P = 0.022), NP-M-ND (P < 0.001), NP-H-ND (P < 0.001), NP-L-HSHFD (P < 0.001), NP-M-HSHFD (P < 0.001) and NP-H-HSHFD (P < 0.001) group. Similarly, FINS level in the C-HSHFD group was higher than that of the NP-L-ND (P = 0.036), NP-M-ND (P < 0.001), NP-H-ND (P < 0.001), C-HSHFD (P = 0.036), NP-L-HSHFD (P = 0.012), NP-M-HSHFD (P < 0.001) and NP-H-HSHFD (P < 0.001) group, respectively. The effect of NP at the dose of 0.2 μg/kg/day on the FINS level of rats was most remarkable among the treatment groups (p < 0.05). ( ).
Serum leptin (LEP) level
On day 180, the action that co-exposure to NP and HSHFD lead to a decrease in the serum LEP level of rats was more noticeable than that of NP-only or C-HSHFD exposure (p < 0.001). The action of NP-only exposure on the LEP level of rats was more noticeable than that of C-HSHFD exposure (p < 0.001).
LEP level had an inverse relationship with NP exposure dose in the NP and HSHFD co-exposure group (p < 0.05). The effect of NP at the dose of 0.2 μg/kg/day on the serum LEP level of rats was still most noticeable among the treatment groups (p < 0.05). In multiple comparison among the 8 groups, LEP levels in the C-ND group were higher compared with the NP-M-ND (p = 0.004), NP-H-ND (p = 0.018), NP-M-HSHFD (p < 0.001) and NP-H-HSHFD (p = 0.005) groups, while LEP level in the C-ND group was lower than that of the C-HSHFD group (p < 0.001).LEP level in the C-HSHFD group was higher than that of the NP-L-ND (p < 0.001), NP-M-ND (p < 0.001), NP-H-ND (p < 0.001), NP-L-HSHFD (p < 0.001), NP-M-HSHFD (p < 0.001), and NP-H-HSHFD (p < 0.001) group, respectively. LEP level in the NP-L-HSHFD group was higher than that of the NP-M-HSHFD (p < 0.001), LEP level in the NP-M-HSHFD group was lower than that of the NP-L-ND (p < 0.001), NP-M-ND (p < 0.001), NP-H-ND (p < 0.001), and NP-H-HSHFD (p < 0.001) group. ().
Oral glucose tolerance test
Exposure to NP-only had highest FBG level at 60 minutes compared to the 0 minute, 30 minutes, 90 minutes and 120 minutes in oral glucose tolerance test at week 12. Exposure to NP-only caused an increase in the FBG level of rats in both NP-L-ND and NP-H-ND groups compared to the C-ND group (P < 0.001).Exposure to NP-only led to an increase in the FBG level of rats in NP-H-ND group compared to both the NP-L-ND (P < 0.001) and NP-M-ND (P < 0.001) groups. ().
Figure 4. OGTT curve in NP-only treatment groups in rats in oral glucose tolerance test at week 12 (n = 10, χ±S).avs C-ND, P < 0.05; bvs NP-L-ND, P < 0.05; cvs NP-M-ND, P < 0.05.
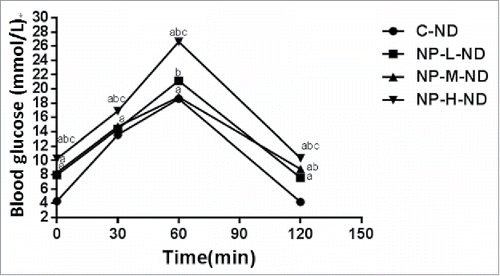
Similarly, co-exposure to NP and HSHFD had highest FBG level at 60 minutes compared to the 0 minute, 30 minutes, 90 minutes and 120 minutes in oral glucose tolerance test at week 12. Co-exposure to NP and HSHFD led to an increase in the FBG level of rats in the NP-H-HSHFD group compared to both the C-HSHFD (P = 0.032) and NP-M-HSHFD (P = 0.008) groups (P < 0.001, ).
Figure 5. OGTT curve in NP+HSHFD treatment groups in rats in oral glucose tolerance test at week 12 (n = 10, χ±S).evs C-HSHFD, P < 0.05; fvs NP-L-HSHFD, P < 0.05; gvs NP-M-HSHFD, P < 0.05.
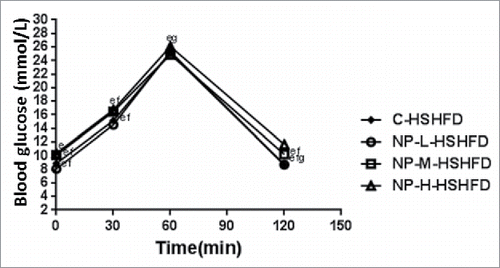
Oral glucose tolerance test showed increased area under the curve (AUC) in treatment groups at week 12 (p < 0.05). During OGTT, exposure to NP plus ND or HSHFD exhibited impaired glucose tolerance compared with the control groups. OGTT-AUC increased with an increase in NP exposure concentration in a dose-response manner. Moreover, exposure to NP-M-HSHFD and NP-H-HSHFD exhibited more obvious abnormal glucose tolerance in comparison with the control groups (p < 0.001, ).
Histological changes in pancreatic tissue
In the histological examinations using HE staining, no abnormal histological phenomena were seen in the pancreatic tissue of C-ND treated rats. In contrast, moderate interlobular edema and necrosis was accompanied by multifocal perivascular lymphocyte infiltrations of the pancreatic tissue in the NP treatment groups. NP provoked abnormal histological changes within the pancreatic tissue in a dose-dependent manner. A decrease of pancreatic islet numbers and size was exhibited in the pancreatic tissue of NP-M-HSHFD and NP-H-HSHFD treated rats compared with C-ND treated rats. Co-exposure to NP and HSHFD causes inflammatory changes histologically ().
Discussion
Here we have shown that chronic low-dose treatment with NP, as an EDC, with the capacity to impair the well-established diagnostic markers of diabetes such as body weight, fasting blood glucose, fast serum insulin level, serum leptin level, glucose tolerance, and histological alterations in high sucrose-high fat diet-treated rats using a paradigm that mimics human exposure. Although it has been shown previously that exposure to EDCs have been implicated as a potential risk factor in the development of metabolic disease, to our knowledge the present study is the first to identify the adverse effects of chronic low-dose exposure to NP, administrated orally, on T2DM.
One of the salient findings of this study is the observation that chronic low-dose exposure to NP led to and aggravate pancreatic tissue inflammation, which inferred that NP exposure might promote the development and progress of T2DM. This notion is supported by 1) an increased trend shown of body weight in rats exposed to NP. The results are compatible with results from our previous study,Citation17 which reported an increased body weight in pups in adulthood and dams exposed to NP during perinatal period. 2) Elevated fast blood glucose level and abnormal glucose tolerance, as well as decreased serum insulin level of rats were present along with an increase in NP exposure concentration. 3) Potential insulin secretion deficiency in pancreatic β cells as supported histologically by the lymphocyte infiltrations of pancreatic tissue, together with a decrease of pancreatic islet numbers and size in the groups treated with NP at the dose of 0.2 μg/kg/day or 2.0 μg/kg/day. Histological damage to β cells could be caused by insulin resistance and lipotoxicity. This possibility is supported by increased body weight after NP exposure in the treatment groups, therefore, we surmised that NP might cause insulin resistance and lipotoxocity. Previous studies by other investigators have shown that exposure to EDCs may promote the development of metabolic diseases such as insulin resistanceCitation18,19 and T2DM.Citation20,21 Glucose-stimulated insulin secretion from islets isolated from NP-fed rats had not been detected in the current study, but we speculated on insulin secretion deficiency according to fast serum insulin level and histological changes in pancreatic tissue.
In the present work, NP chronic low-dose treatment led to changes in glucose homeostasis. Environmental concentration exposure to NP affected the glycometabolism and function of pancreatic cell in rats. Previous studies focus on NP as a risk factor for metabolic syndrome, especially under acute and sub-chronic conditions, however, the effects of chronic exposure to NP on glycometabolism is scarce, especially concerning in vivo experiment. High-sucrose/high-fat diet is at a higher risk of developing diabetes, hence, two experimental control groups of normal diet and high-sucrose/high-fat diet were set up in a similar manner as was done in humans in this study, for the purpose of exploring the impaired glucose metabolism and morphological changes induced by chronic exposure NP in pancreatic tissue; besides, comparing the risk differences in the development of diabetes caused by NP via different ways of eating. On days 120 and/or 180 rats treated with NP-only showed increased body weight; co-exposure to NP and HSHFD induced an elevated FBG, and reduced serum LEP, as well as FINS level with the greatest impact of NP at a dose level of 0.2 μg/kg/day. Our results showed that chronic exposure to NP may have main effects on an increased risk of T2DM. Moreover, the exposure of NP in conjunction with HSHFD, as an improper dietary habit, appeared to cause an additive effect on the glycometabolism disorders. In addition, an exposure gradient of NP treatment concentration ranging from 0.02 to 2.0 μg/kg/day designed in the current research was used to assess the threshold effect for NP-induced glycometabolism disruption.
Leptin is an adipose-derived hormone that contributes, in part, to NASH development in obesity. Leptin decreases the expression of or exigenic neuropeptidesCitation22 and increases the expression of anorectic peptidesCitation23 in the hypothalamus to modify hunger and food intake. Leptin could improve the glucose and lipids metabolism of type 2 diabetic mice, which might related to the mechanism that leptin may reduce liver oxidative stress and endoplasmic reticulum stress, as well as restoring sensitivity to insulin.Citation24 Leptin was considered as a new therapeutic treatment for T2DM.Citation25 On day 180 serum insulin level increased with an increase in NP exposure concentration, whereas an opposite trend was observed with serum leptin level. Abnormal glucose tolerance test value and impaired glucose tolerance were also observed at 12 week in this study. The results shown that insulin resistance may be further present in rats. Base on the logical deduction, dynamic fasting insulin monitoring would also be conducted in rats, which helps make more explicit the fact that chronic exposure to NP affects glycometabolism. One research result in this study showed interesting findings: the NP-M-HSHFD group at 180 days appeared a higher body weight than almost all other treatment groups, while its serum leptin level was the lowest. The reason for this paradox may be due to the low dose effects of NP because the mechanism related to the effects of NP on obesity or diabetes has been indefinite unitl now.
Animal species differences in NP metabolism exist remarkably. The extrapolation of toxicity data obtained in animal models on NP to assess human exposure and health risk has many uncertainties, which can cause subtle risk assessment estimates. There is discrepancy in the no observable adverse effect level (NOAEL) of NP and lowest observable adverse effect level (LOAEL) in various studies.Citation26,27 NOAELs for renal toxicity and reproductive changes are in the same range of 10–15 mg/kg/day.28 NP induced toxic effect on cells in a dose-dependent manner in the micromolar range. Taking into account our prior research result that the load of serum nonylphenol in patients with T2DM (584.71 ± 30.79 ng/mL) was higher than that of the healthy (523.60 ± 52.78 ng/mL).Citation29 Moreover, the concentrations of NP in pancreas tissues in both the NP normal diet group (2045.0 ± 130.1 μg/L) and NP high-energy diet groups (2038.0 ± 104.2 μg/L) were significantly higher than those in the control normal diet (499.5 ± 27.4 μg/L) and control high-energy diet groups (494.2 ± 22.4 μg/L).Citation30 We speculated that NP might affect glycometabolism in rats.
Conclusion
In contrast to previous reports, we, for the first time, observed a joint toxic effect of NP and HSHFD on the development and progress of T2DM of rats. In our study, chronic low-dose exposure to NP might induce impaired glucose tolerance, which further lead to insulin resistance, and pancreatic β cell insulin secretion deficiency, ultimately increase the risk of T2DM. Moreover, the additive toxic effects of NP and HSHFD on pancreatic beta-cell function and glucose metabolism have been identified among rats as well.
Disclosure of potential conflicts of interest
No potential conflicts of interest were disclosed.
Additional information
Funding
References
- Guariguata L, Whiting DR, Hambleton I, Beagley J, Linnenkamp U, Shaw JE. Global estimates of diabetes prevalence for 2013 and projections for 2035. Diabetes Res Clin Pract. 2014;103(2):137–49. doi:10.1016/j.diabres.2013.11.002. PMID:24630390.
- Xu Y, Wang L, He J, Bi Y, Li M, Wang T, Wang L, Jiang Y, Dai M, Lu J, et al. Prevalence and control of diabetes in Chinese adults. JAMA, 2013;310 (9) :948–59. doi:10.1001/jama.2013.168118. PMID:24002281.
- Martin BC, Warram JH, Krolewski AS, Bergman RN, Soeldner JS, Kahn CR. Role of glucose and insulin resistance in development of type 2 diabetes mellitus: results of a 25-year follow-up study. Lancet. 1992;340(8825):925–9. doi:10.1016/0140-6736(92)92814-V. PMID:1357346.
- Lee D H, Porta M, Jr J D, Vandenberg LN. Chlorinated persistent organic pollutants, obesity, and type 2 diabetes[J]. Endocrine Reviews. 2014;35(4):557. doi:10.1210/er.2013-1084. PMID:24483949.
- Beydoun HA, Khanal S, Zonderman AB, Beydoun MA. Sex Differences in the Association of Urinary Bisphenol-A Concentration with Selected Indices of Glucose Homeostasis among U.S. adults. Ann Epidemiol. 2014;24(2):90–7. doi:10.1016/j.annepidem.2013.07.014.
- Sato S, Shirakawa H, Tomita S, Ohsaki Y, Haketa K, Tooi O, Santo N, Tohkin M, Furukawa Y, Gonzalez FJ, et al. Low-dose dioxins alter gene expression related to cholesterol biosynthesis, lipogenesis, and glucose metabolism through the aryl hydrocarbon receptor-mediated pathway in mouse liver. Toxicol Appl Pharmacol. 2008;229(1):10–9. doi: 10.1016/j.taap.2007.12.029.
- Jie X, Jianmei L, Zheng F, Lei G, Biao Z, Jie Y. Neurotoxic effects of nonylphenol: A Review[J]. Wien Klin Wochenschr. 2013;125(3-4):61–70. doi:10.1007/s00508-012-0221-2. PMID:23334477.
- Jie Y, Jie Z, Ya L, Xuesong Y, Jing Y, Yu Y, Jiaqi Y, Jie X. Pollution by Nonylphenol in river, tap water, and aquatic in an acid rain-plagued city in southwest China. Journal International Journal of Environmental Health Research. 2017;27(3):179–90. doi:10.1080/09603123.2017.1332345. PMID:28587474.
- Asimakopoulos AG. 2010. Determination of bisphenol A, 4-t-octylphenol and 4-nonylphenol in human blood serum by liquid chromatography–electrospray tandem mass spectrometry (LC–MS/MS). M.Sc. Thesis submitted to the Department of Chemistry of the National and Kapodistrian University of Athens.
- Calafat AM, Kuklenyik Z, Reidy JA, Caudill SP, Ekong J, Needham LL. Urinary Concentrations of Bisphenol A and 4-Nonylphenol in a Human Reference Population. Environ Health Perspect. 2005;113(4): 391–5. doi:10.1289/ehp.7534. PMID:15811827.
- Ferrara F, Ademollo N, Orrù MA, Silvestroni L, Funari E. Alkylphenols in adipose tissues of Italian population. Chemosphere. 2011;82(7):1044–49. doi:10.1016/j.chemosphere.2010.10.064. PMID:21075420.
- Geens T, Neels H, Covaci A. Distribution of bisphenol-A, triclosan and n-nonylphenol in human adipose tissue, liver and brain. Chemosphere. 2012;87(7):796–802. doi:10.1016/j.chemosphere.2012.01.002. PMID:22277880.
- Verner MA, Magher T, Haddad S. High concentrations of commonly used drugs can inhibit the in vitro glucuronidation of bisphenol A and nonylphenol in rats. Xenobiotica. 2010;40:83–92. doi:10.3109/00498250903383334. PMID:19916736.
- Kazemi S, Feizi F, Aghapour F, Joorsaraee GA, Moghadamnia AA. Histopathology and Histomorphometric Investigation of Bisphenol A and Nonylphenol on the Male Rat Reproductive System. N Am J Med Sci. 2016;8(5):215–21. doi:10.4103/1947-2714.183012. PMID:27298816.
- Xu J, Wang Y, Yu J, Fan QY, Liu XY, Li Y.;Huang HJ. Immune effects of nonylphenol on offspring of rats exposed during pregnancy. Hum. Ecol. Risk Assess. 2010;16:444–52. doi:10.1080/10807031003670485.
- Yu Jie, Fan QY, Binli H, Biao Z, Zheng F, Jianmei L, Jie X. Joint neurodevelopmental and behavioral effects of nonylphenol and estradiol on F(1) male rats. Int J Environ Heal R 2013;23:321–30. doi:10.1080/09603123.2012.733936.
- Yu J, Luo Y, Yang XF, Yang MX, Yang J, Yang XS, Zhou J, Gao F, He LT, Xu J. Effects of perinatal exposure to nonylphenol on delivery outcomes ofpregnant rats and inflammatory hepatic injury in newborn rats[J]. Brazilian Journal of Medical & Biological Research. 2016;49(12):e5647. doi:10.1590/1414-431x20165647.
- Batista TM, Alonso-Magdalena P, Vieira E, Amaral ME, Cederroth CR, Nef S, Quesada I, Carneiro EM, Nadal A. Short-Term Treatment with Bisphenol-A Leads to Metabolic Abnormalities in Adult Male Mice. PLoS One. 2012;7(3):e33814. doi:10.1371/journal.pone.0033814. PMID:22470480.
- Hind A Beydoun, Suraj Khanal, Alan B Zonderman, May A Beydoun. Sex Differences in the Association of Urinary Bisphenol-A Concentration with Selected Indices of Glucose Homeostasis among U.S. adults. Ann Epidemiol. 2014;24(2):90–7. doi:10.1016/j.annepidem.2013.07.014.
- Iavicoli I, Fontana L, Leso V, Bergamaschi A. The Effects of Nanomaterials as Endocrine Disruptors. Int J Mol Sci. 2013;14(8):16732–801. PMID:23949635.
- Regnier SM, Kirkley AG, Ye H, El-Hashani E, Zhang X, Neel BA, Kamau W, Thomas CC, Williams AK, Hayes ET, et al. Dietary Exposure to the Endocrine Disruptor Tolylfluanid Promotes Global Metabolic Dysfunction in Male Mice. Endocrinology. 2015;156(3):896–910. PMID:25535829.
- Bayer L, Jacquemard C, Fellmann D, and Griffond B. Survival of rat MCH (melanin-concentrating hormone) neurons inhypothalamus slice culture: histological, pharmacological and molecular studies. Cell Tissue Res. 1999;297;23–33. PMID:10398880.
- Timothy T Lu, Makoto Makishima, Joyce J Repa, Kristina Schoonjans, Thomas A Kerr, Johan Auwer, Timothy T Lu, Makoto Makishima, Joyce J Repa, Kristina Schoonjans, Thomas A Kerr, Johan Auwerx, David J Mangelsdorf. Leptin increases hypothalamic pro-opiomelanocortin mRNA expression in the rostral arcuate nucleus. Diabetes. 1997;46(12):2119–23. PMID:9392508.
- Yang Weicheng. A study on possible mechanism of leptin improves glucose and lipids metabolism in type 2 diabetic ob/ob mice[D]. Anhui Medical University. 2016.
- Unger RH, Roth MG. A new biology of diabetes revealed by leptin. Cell Metab. 2015;21(1):15–20. PMID:25456738.
- Ademollo N, Ferrara F, Delise M, Fabietti F, Funari E. Nonylphenol and octylphenol in human breast milk. Environment International. 2008;34(7):984–7. PMID:18410965.
- Hasegawa R, Koizumi M, Hirose A, Maekawa A. Estrogenic Activity and Reproductive Organ Toxicity of Alkylphenols. Japanese Journal of Food Chemistry. 2000;7:1–9.
- Noorimotlagh Z, Haghighi NJ, Ahmadimoghadam M, Rahim F. An updated systematic review on the possible effect of nonylphenol on male fertility. Environmental Science & Pollution Research. 2017;24(4):3298.
- Luo Ya, Zheng Guobo, Yang Xuefeng, Wang Deqian, Yang Xuesong, Yang Jing, Xu Jie. The load of serum nonylphenol in patients with type-αdiabetes mellitus. Journal of Practical Medicine. 2016;32(13):2227–30.
- Yang J, Yu J, Wang P, Luo Y, Yang XF, Yang XS, Lia WM, Xua J. The adverse effects of perinatal exposure to nonylphenol on carbohydrate metabolism in male offspring rats. 2017;27(5):368–76.