Abstract
Exogenous melatonin can be helpful for treatment of some sleep disorders. However, immediate-release formulations are rapidly absorbed and cleared from the body making it difficult to provide coverage for an entire sleep period. Extended-release melatonin formulations can better mimic the naturally occurring melatonin profile and increase efficacy, but few studies have reported on their pharmacokinetics. To assess the pharmacokinetics of extended-release melatonin, we conducted a randomized, double-blind, crossover study of extended-release melatonin (4 mg) compared to immediate-release melatonin (4 mg) in 18 healthy adults, ages 18–65 years. Participants received immediate-release or extended-release melatonin in clinic after an 8 h fast, and blood samples were taken over a 10-h period. After a 7-day washout period, the same procedures were repeated with the melatonin form not previously received. Extended-release melatonin had a longer time to peak concentration (1.56 vs 0.6 h) and elimination half-life (1.63 vs 0.95 h) compared with immediate-release melatonin. Maximum concentration was lower for extended-release melatonin compared with immediate-release melatonin (7581 pg/mL vs 13120 pg/mL). Extended-release melatonin raised melatonin levels in as little as 15 min and sustained elevated melatonin levels (>300 pg/mL) for 6 h before falling below 50 pg/mL by 9 h. No clinically relevant adverse events were observed, and safety parameters remained within normal ranges for both formulations. The pharmacokinetic profile of this extended-release melatonin formulation suggests that it could be used for future efficacy studies of melatonin and sleep outcomes. This trial is registered at ClinicalTrials.gov, NCT04067791.
Introduction
Insufficient and suboptimal sleep is an ongoing public health crisis, where over 30% of the U.S. population does not get adequate sleep (Liu et al. Citation2016). Short sleep (less than 7 h per night) is associated with a range of negative effects on overall health and mental wellness (Chattu et al. Citation2018). These include increased risk for cardiovascular disease (Cappuccio et al. Citation2011; Cepeda et al. Citation2016; Seixas et al. Citation2018; Tobaldini et al. Citation2019), weight gain and obesity (Stamatakis and Brownson Citation2008; Grandner et al. Citation2015), and the development of metabolic disorders and diabetes (Chaput et al. Citation2007). Adequate sleep is important for immune health and mental wellness (Irwin Citation2015; Besedovsky et al. Citation2019). Various sleep troubles and disorders can contribute to difficulty falling or staying asleep. Recently, the ongoing COVID-19 pandemic has further contributed an increase in sleep complaints and the use of prescription sleep aids (Mandelkorn et al. Citation2020; Beck et al. Citation2021). Prescription sleep aids, such as hypnotics and benzodiazepines, are effective for the treatment of insomnia, but are limited by factors that include negative side effects and risk for tolerance, abuse, and dependency (Kripke Citation2016). Melatonin is a non-habit-forming alternative that is readily available as a dietary supplement that can assist individuals struggling with occasional sleeplessness.
Melatonin supplements mimic endogenous melatonin in the body to assist with sleep. Melatonin is the primary hormone produced by the pineal gland, and it plays a key role in regulating sleep and circadian rhythm. Endogenous melatonin secretion increases soon after the onset of darkness and reaches maximum serum levels of 100 pg/mL during the middle of the night between 2:00–4:00 am. During the second half of the night, levels gradually decrease and reach less than 10 pg/mL during the day (Zhdanova et al. Citation1998). The endogenous melatonin profile changes with age, and excursions away from the normal profile can lead to an inability to sleep and poor quality of sleep (Karasek Citation2004; Bubenik and Konturek Citation2011).
Exogenous melatonin preparations can be used when a person’s endogenous clock has become desynchronized from ambient day-night cycles such as with circadian-related sleep disorders, jet lag, shift work insomnia, non-restorative sleep quality, and Alzheimer’s disease. Treatment with exogenous melatonin decreases sleep onset latency, increases total sleep time, and improves overall sleep quality in individuals diagnosed with primary sleep disorders (Brzezinski et al. Citation2005; Buscemi et al. Citation2005; Ferracioli-Oda et al. Citation2013). Exogenous melatonin is also effective for preventing and reducing jet lag (Herxheimer and Petrie Citation2002). More recently, improvements in circadian regulation have been shown to translate to improvements in sleep quality, cognition, healthy aging, and decreased risk of cardiovascular disease (Wade et al. Citation2007; Cardinali et al. Citation2012; Sun et al. Citation2016). Unlike with many prescription sleep medications, patients are unlikely to become dependent on melatonin, habituate to it after repeated use, or experience rebound insomnia (Lemoine et al. Citation2011).
One of the challenges of using exogenous melatonin as a sleep aid is that melatonin is short-lived in humans. Immediate-release formulations are rapidly absorbed with time to peak concentration (Tmax) ranging from 15 min to 90 min depending on the dose (Harpsoe et al. Citation2015). Melatonin is also rapidly cleared with a short elimination half-life (t1/2) of approximately 45 min (Harpsoe et al. Citation2015). Thus, repeated administration or high doses would be needed to cover the 7 to 9-h sleep period recommended by the National Sleep Foundation (Hirshkowitz et al. Citation2015).
In order to better mimic a naturally occurring melatonin profile and improve efficacy, extended-release (i.e. prolonged-release) melatonin formulations have been developed (Lemoine et al. Citation2007; Citation2011; Lemoine and Zisapel Citation2012). Clinical trials in adults (55+ years) with primary insomnia have demonstrated that 2 mg extended-release melatonin is more effective than placebo at improving quality of sleep and the ability to function normally the following day (European Medical Agency Citation2007; Lemoine and Zisapel Citation2012). Extended-release melatonin appears to improve cognitive functioning and sleep maintenance in patients with mild to moderate Alzheimer’s disease, especially for those individuals with an insomnia comorbidity when combined with standard therapy (Wade et al. Citation2014). While extended-release melatonin appears to be efficacious for certain conditions, few studies have reported the pharmacokinetic profile. Previous work investigated the pharmacokinetic properties of a surge-sustained release melatonin formulation, which combined immediate-release and controlled-release dosing. While the Tmax and t1/2 were substantially increased compared with documented immediate-release formulations, melatonin levels remained elevated above 50 pg/mL for an average of 10 h, which could result in elevated melatonin levels beyond the typical sleep period (Gooneratne et al. Citation2012). Here we report on the pharmacokinetics of an extended-release melatonin formulation designed to quickly increase melatonin levels, sustain elevated levels for the duration of a typical sleep period, and return plasma concentrations to daytime levels upon wake time.
Materials and methods
Ethics
The study was reviewed and approved by the Natural Health Product Directorate, Health Canada and a research ethics board prior to study initiation. This study was conducted in accordance with the ethical principles that originate in the Declaration of Helsinki and its subsequent amendments. Informed consent was obtained from each participant at the screening visit prior to performing any study-related activities.
Participants
Eligible participants were 18 to 65 years of age, with a body mass index (BMI) of 18.5 to 29.9 kg/m2. Criteria for inclusion required women to not be pregnant (negative urine pregnancy test), breastfeeding, or planning to become pregnant during the study (by using medically approved contraception). Criteria for inclusion required men to either be sterile or willing to use medically-approved contraception. All participants needed to be medically healthy, with no clinically significant medical history, physical examination results, or laboratory profiles, as deemed by the investigator. All participants were required to have a regular sleep-wake cycle with a bedtime between 9:00 pm to 12:00 am and receive between 7 and 8 h of sleep for at least 3 wk prior to baseline. Exclusion criteria included previous diagnosis of a sleep disorder, use of a C-PAP machine, previous diagnosis of pineal gland abnormalities, pinealectomy, vivid nightmares or sleepwalking, shift work within the previous 3 wk, and travel across one or more time zones in the past 2 wk. Participants on any of the following prescribed medications or treatments were excluded from enrollment: sedatives, any medications taken for sleep, hormone replacement therapy, fluvoxamine, anticoagulants, antiplatelets, verapamil, antibiotics, proton pump inhibitors, ADHD medications, calcium channel blockers, non-steroidal anti-inflammatory drugs, or steroids. Additionally, participants who were consuming any of the following OTC medications, supplements, or food/drinks were not allowed to participate unless they were willing to undergo the specified washout period prior to their baseline visit and agreed not to take any of the specified OTC medications or supplements throughout the study: melatonin supplements (7 days); tryptophan supplements (2 days); 5-HTP supplements (3 days); any supplements and/or teas taken for sleep (washout was assessed by the medical director based on dose and frequency of use); St. John’s wort (30 days); Grapefruit and grapefruit juice (7 days); Caffeine (1 day); NSAIDS (7 days); Vitamin B12 supplements (14 days); Echinacea (2 days); Tart cherry and tart cherry-containing products (3 days); Medications containing sleep aids such as: Gravol (7 days); Tylenol PM (7 days); Nyquil PM (7 days).
Study design
This randomized, double-blind, comparator-controlled, crossover study was conducted at a single site in Ontario, Canada from July to August 2019. The primary objective was to compare the pharmacokinetic parameters of extended-release and immediate-release melatonin. The study consisted of two 1-day study periods separated by a 7-day washout (). If the potential participant met al.l the inclusion criteria and did not meet any of the exclusion criteria at baseline, a randomization number was assigned to the participant by a blinded investigator per the order of the randomization list generated by www.randomization.com. Eligible participants were randomized in a double-blinded manner at a ratio of 1:1 to one of the two treatment sequences: 1) extended-release melatonin → immediate-release melatonin, or 2) immediate-release melatonin → extended-release melatonin. Each study period consisted of a 10-h clinic visit during which a single dose of melatonin was orally administered in the morning after an 8 h fast. The study was performed during the daytime to minimize the confound of nighttime endogenous melatonin production. Both extended-release and immediate-release formulations consisted of a bi-layer tablet containing 4 mg of melatonin with differing release profiles. No differences in size, color, taste, texture, or packaging were detectable between the two products. Investigators, other site personnel, and participants were blinded to the product. Blood melatonin concentrations were characterized by sampling at multiple timepoints: pre-dose (baseline), followed by 0.25, 0.50, 0.75, 1, 2, 3, 4, 5, 6, 8, and 10 h post-dose. Blood plasma melatonin concentrations were quantified by Keystone Bioanalytical, Inc using LC/MS/MS. General safety and tolerability assessments included adverse events, vital signs, and clinical laboratory evaluations (hematology and clinical chemistry). At each in-clinic visit, participants’ 7-day, as well as 24 h food records were reviewed to confirm dietary and sleep compliance. Participants were provided standardized meals after their overnight fast during visits 2 and 3 to control for known impacts of food intake on melatonin pharmacokinetics (European Medical Agency Citation2007). Breakfast was provided after blood sampling at 1-h post-dose, lunch was provided after blood sampling at 5-h post-dose, and dinner was provided 9-h post-dose. Breakfast was comprised of 2 slices of whole wheat toast, 1 tbsp jam, 1 tbsp butter, 1 cup yogurt parfait (Greek yogurt, berries, granola, and honey), and ad libitum water. No coffee, tea, or juices were permitted at breakfast. Lunch was comprised of Campbell’s Tomato Basil Bisque, either turkey and Swiss or roasted vegetable and Swiss sandwich on whole wheat bread, a dessert bar, and ad libitum water. One cup of herbal decaffeinated tea or decaffeinated coffee was permitted at lunch. Dinner was comprised of chicken or vegetarian stir fry and steamed rice, a brownie/blondie, and ad libitum water. One cup of herbal decaffeinated tea or decaffeinated coffee was permitted at dinner.
Treatment products
Both immediate- and extended-release study products were identical in bi-layer tablet appearance and melatonin content, with differing melatonin dissolution rates. Melatonin content of each study product was verified using a validated, high-performance liquid chromatography (HPLC) assay for melatonin tablets, following the International Council for Harmonization of Technical Requirements for Pharmaceuticals for Human Use (ICH) guidelines for analytical method validation. The analysis showed that both treatment products contained 4.47 mg melatonin/tablet at the time of the study, which includes 0.47 mg melatonin overage to ensure that the product contains a minimum of 4 mg melatonin at the end of shelf life. To ensure rapid melatonin release from the immediate-release product, tablet dissolution was no less than 75% within 30 min. To ensure both rapid- and sustained-release characteristics from the extended-release melatonin product, tablet dissolution was no more than 50% within 60 min.
Statistical analysis
The study was powered to detect a difference in area under the curve (AUC) of 97.7 μg.h/L, a difference in Cmax of 11.6 μg/L, and a difference in t1/2 of 2.5 h between the two treatments. A sample size of 18 total participants gives 80% power to detect differences at an overall 5% significance level. The significance level was adjusted for multiple testing. This sample size takes into account a loss to follow up rate of 20%.
All statistical analyses were performed using R version 3.5.3. The intent-to-treat (ITT) population consisted of all participants who received either product and for whom any post-randomization efficacy information was available. The per protocol (PP) population consisted of all participants who consumed at least 80% of either product dose, did not have any major protocol violations, and who completed all study visits and procedures connected with measurement of the primary variable.
AUC0-10h and incremental AUC (iAUC0-10h) were calculated using the trapezoid method. AUC0-10h, iAUC0-10h, and Cmax were compared between the two groups after natural logarithmic transformation. Sequence, period, and form (extended-release and immediate-release melatonin) were included in the model as the fixed effects. For Tmax, terminal disposition rate constant (λ), terminal half-life (t½), absorption rate constant (ka) and absorption half-life (ta½), differences between the two study groups were analyzed using analysis of variance (ANOVA) models. Sequence, period, and form (extended-release and immediate-release melatonin) were included in the model as the fixed effects. Q-Q plots and histograms were generated for the evaluation of normality of the residuals of models. Where residuals were found to be non-normally distributed, ranked transformation was performed before analysis. A p-value ≤ 0.05 was considered significant.
Results
Participant demographics and baseline characteristics
Eighteen participants were randomized, and 17 completed the trial (); 1 participant discontinued due to an adverse event deemed unrelated to study product by medical staff. Of the 18 enrolled participants, 9 were male and 9 were female, and 67% were White. At baseline, participant’s age ranged from 20–60 years and BMI ranged from 22.8–29.2 kg/m2. Participant demographics and baseline characteristics are summarized in . Participants did not differ in demographics or baseline characteristics for the two different study sequences.
Table 1. Demographics and baseline characteristics.
Pharmacokinetics
Plasma concentrations of melatonin were similar between PP (n = 17) and ITT (n = 18) populations, so for the purpose of this manuscript we only report PP data, shown in . Between the extended-release and immediate-release formulations, differences in the mean plasma melatonin concentrations were noted at timepoints 0.25 h (p < 0.001), 0.5 h (p < 0.001), 0.75 h (p = 0.003), 1 (p = 0.038), 2 h (p = 0.031), 3 h (p = 0.006), 4 h (p = 0.004), 5 h (p = 0.005), and 6 h (p = 0.011). Statistically significant excursions from pre-ingestion melatonin levels occurred at all timepoints from 0.25 to 6 h. From 8 h to 10 h, melatonin levels were not significantly different from pre-ingestion levels. Previous studies have indicated that 0.3 mg melatonin is the minimum efficacious single dose of melatonin for falling asleep with a corresponding Cmax of approximately 300 pg/mL (Dollins et al. Citation1994; Zhdanova et al. Citation1996; Citation1998; Fourtillan et al. Citation2001). We considered this the minimum threshold for maintaining efficacious plasma melatonin concentrations. Immediate-release melatonin raised plasma melatonin levels above 300 pg/mL within 15 min and sustained elevated levels for approximately 4 h. Extended-release melatonin also raised plasma melatonin levels above 300 pg/mL within 15 min and sustained elevated levels for approximately 6 h, see .
Figure 2. Total plasma melatonin concentrations. Data are mean ± SD at pre-dose (0 h) and 0.25, 0.5, 0.75, 1, 2, 3, 4, 5, 6, 8, and 10 h post-dose for participants in the PP population (n = 17). PP: per protocol; SD: standard deviation.
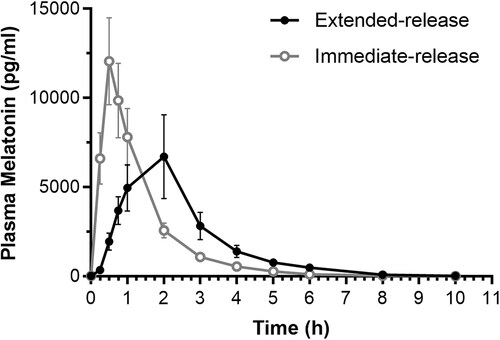
A comparison of pharmacokinetic parameters of the PP population (n = 17) is summarized in . Significant differences in Cmax and Tmax were seen between the extended-release and the immediate-release melatonin formulations. The extended-release melatonin had a lower maximum concentration (Cmax, p < 0.002) and a longer time to reach maximum concentration in the blood (Tmax = 1.56 h, p < 0.001), while the immediate-release melatonin reached maximum concentration in 0.6 h. Excursions in plasma melatonin were similar with no significant differences in AUC and iAUC(0-10h) between the extended- and the immediate-release melatonin preparations.
Table 2. Comparison of pharmacokinetic parameters of extended-release and immediate-release melatonin.
The absorption rate constant (ka) (p < 0.001) and the terminal disposition rate constant (λ) (p < 0.001) were lower for extended-release melatonin than for the immediate-release formulation indicating a slower rate of absorption (77% less) and elimination while the immediate-release was absorbed more quickly and showed faster elimination. The absorption half-life (ta1/2) (p < 0.002) was greater with the immediate-release formulation compared with the extended-release formulation. The terminal half-life (t1/2) however, was greater with the extended-release formulation compared with the immediate-release formulation (p < 0.001),.
General safety and tolerability
Both formulations were well tolerated, and there were no clinically relevant adverse events. Safety parameters remained within normal clinical range for both formulations.
Discussion
Few studies have reported on the pharmacokinetic profile of extended-release melatonin. The results presented here demonstrate that the extended-release melatonin formulation raises melatonin levels in as little as 15 min and sustains elevated melatonin levels (>300 pg/mL) for 6 h before gradually falling below 50 pg/mL. In contrast, immediate-release melatonin rapidly raised melatonin levels within 15 min to a much higher peak concentration, but it was only elevated for 4 h. This more rapid decline in melatonin levels with immediate-release melatonin was reflected by a greater elimination rate constant and shorter elimination half-life compared with extended-release melatonin.
Previous studies have indicated that 0.3 mg melatonin is the minimum efficacious single dose of melatonin for falling asleep with a corresponding Cmax of approximately 300 pg/mL (Dollins et al. Citation1994; Zhdanova et al. Citation1996, Citation1998; Fourtillan et al. Citation2001). We considered this the minimum threshold for maintaining efficacious plasma melatonin concentrations. Compared to the immediate-release formulation, the extended-release formula kept plasma melatonin concentration above this threshold for 2 h longer. Additionally, extended-release melatonin was generally safe and well-tolerated with no clinically relevant adverse events and safety parameters remained within normal ranges.
Our pharmacokinetic results were consistent with those of other extended-release melatonin formulations. Previous reports on the pharmacokinetics of extended-release formulations given at 2–5 mg observed Tmax ranging from 1.5 to 2.7 h and t1/2 ranging from 1.5 to 2.1 h (European Medical Agency Citation2007; López-Gamboa et al. Citation2010; Gooneratne et al. Citation2012). While gradual release of melatonin is desirable for coverage of the entire nighttime sleep period, it is also important for melatonin levels to fall back to lower levels by wake time so not to interfere with daily function and alertness. At doses of 4 or 5 mg, previous extended-release melatonin formulations have caused melatonin levels to remain elevated (>50 pg/mL) for up to 10–12 h, which is beyond the typical sleep period and could increase risk of daytime sleepiness (López-Gamboa et al. Citation2010; Gooneratne et al. Citation2012). In contrast, we observed that after administration of this 4 mg extended-release formulation, melatonin levels fell below this threshold 9 h after ingestion which is a more desirable time period with less risk of a next-day carryover effect. Thus, the rate of release is an important consideration when designing melatonin formulations.
Limitations
As this study was designed to assess pharmacokinetics, safety, and tolerability of a new extended-release melatonin formulation, participants were restricted to healthy adults age 18–65 years, since melatonin metabolism is known to differ in older adults, as well as in children. Previous research has shown that extended-release melatonin may provide the greatest benefit to patients over 55 years of age with primary insomnia (Lemoine et al. Citation2007; Wade et al. Citation2007; Citation2011). Endogenous melatonin production is known to decline with age, and older adults may also have different kinetic parameters due to age-related alterations in renal and hepatic clearance, as well as body composition (Sharma et al. Citation1989; Mangoni and Jackson Citation2004). Similarly, the rates of melatonin metabolism and clearance are faster in prepubertal children than in adults (Cavallo and Ritschel Citation1996), so a better understanding of extended-release melatonin pharmacokinetics in children merits further investigation. The American Academy of Sleep Medicine currently recommends that children 6 to 12 years of age should sleep 9 to 12 h/night and teenagers 13 to 18 years of age should sleep 8 to 10 h/night for improved attention, learning, memory, emotional regulation, and physical health (Paruthi et al. Citation2016). While childrens’ sleep issues are often resolved with good bedtime routines, melatonin is an effective short-term way to help children get rest, while establishing good sleep habits and consistency in bedtime routines.
In conclusion, this extended-release melatonin formulation quickly raised plasma melatonin levels and sustained levels above 300 pg/mL for 6 h before falling below 50 pg/mL by 9 h. This profile provides coverage for the recommended 7 to 9 h sleep period recommended by the American Academy of Sleep Medicine and the Sleep Research Society, while minimizing risk of daytime sleepiness the next day. Future research should assess efficacy of this extended-release melatonin formulation on behavioral sleep variables including sleep onset latency, sleep quality, and daytime function.
Author contributions
JGM, DW, DLDF, and SHM conceived of and designed the study. JGM, DW, MF, SJG performed key procedures and acquired data. JGM, RWG, SHM contributed to the analysis and interpretation of the data. All authors critically reviewed the manuscript and approved the final version.
Acknowledgements
We thank Ann G. Liu, PhD for assistance with the preparation of this report.
Disclosure statement
JGM, DW, DLDF, MF, SJG, RWG, and SHM are employees of Pharmavite, LLC.
Additional information
Funding
Notes on contributors
Jonathan G. Mun
Jonathan Mun, PhD is a Principal Nutrition Scientist at Pharmavite, a dietary supplements company dedicated to quality products that help people lead healthier lives. He holds a PhD in Nutritional Sciences from the University of Illinois at Urbana-Champaign, and his areas of expertise include plant science, nutritional biochemistry, neuroscience, and sleep. His interest is in translating preclinical and clinical research into affordable products that improve public health and quality of life.
Dan Wang
Dan Wang, PhD is Product Research and Development Scientist of Pharmavite. He has contributed to the growth of the dietary supplement industry for past 30 years. He is an inventor of two patented technology/products, and developer of over 50 dietary supplement products. His major research interests included therapeutic nutrition of cereal dietary fiber for hypercholesterolemia and the genetics of the nutrition quality of cereal grain.
Denise L. Doerflein Fulk
Denise Doerflein Fulk, BS currently holds the position of Senior Product Development Manager at Pharmavite, a dietary supplements company. She has more than 20 years of experience in the food/supplement industry, providing technical and strategic leadership in the areas of product development, research, quality assurance and production. She has developed a multitude of innovative products in both the food and supplement industries.
Mina Fakhary
Mina Fakhary, MS, she serves as Principal Scientist at Pharmavite. She obtained her master’s degree in chemistry from California State University Northridge. She possesses over 16 years of experience in pharmaceutical and nutraceutical industry. She has extensive experience on developing and validating analytical methods for identification and measurement of actives in finished products and raw materials.
Scott J. Gualco
Scott Gualco, MSc currently holds the position of Verification Programs Manager at Pharmavite. He obtained a Master of Science in Agriculture with a specialization in Dairy Products Technology from California Polytechnic State University, San Luis Obispo, and has over 10 years of experience in Quality Assurance in the food industry. His areas of expertise include new product development, GMP systems auditing, and manufacturing process control.
Ryan W. Grant
Ryan Grant, PhD is the Senior Manager of Nutrition Science at Pharmavite. He received his PhD in Nutritional Sciences from the University of Illinois at Urbana-Champaign. Ryan’s team provides technical and strategic leadership for developing the science-supported benefits of Pharmavite’s supplements. Ryan applies his broad background in nutrition and specific expertise in metabolism, immunology and inflammation to tackle scientific questions from proof-of-concept research to clinical trials supporting commercialized products.
Susan Hazels Mitmesser
Susan Hazels Mitmesser, PhD, is a creative and dynamic research scientist with nutritional biochemistry experience in various industries, academia, and clinical settings. Her multidisciplinary training in basic, applied, and human clinical sciences, with extensive experience in biomedical research, experimental design, statistical analyses, and abstract/manuscript preparation sets her apart as a visionary in strategic research planning, implementation, and execution. Dr. Mitmesser has 20 years of leadership experience and currently serves as the VP of Science and Technology at Pharmavite. At Pharmavite, she provides scientific and technical leadership on pre-clinical and clinical research, innovation, product development, medical education with a focus on the safety, effectiveness, and biological activity of compounds found in foods. Additionally, physical research is emphasized to determine the best way to deliver compounds to improve consumer compliance and satisfaction. Dr. Mitmesser serves on the Editorial Board of four peer-reviewed journals, has published in numerous peer-reviewed journals and is a contributing author for book chapters relating to nutrition in adult and pediatric populations. She is an active member of the American Society of Nutrition, the American Association for the Advancement of Science, and serves on the Senior Scientific Advisory Council for the Council for Responsible Nutrition. Dr. Mitmesser holds two adjunct professorships at the University of Connecticut and Tufts University.
References
- Beck F, Léger D, Fressard L, Peretti-Watel P, Verger P, The Coconel Group. 2021. Covid-19 health crisis and lockdown associated with high level of sleep complaints and hypnotic uptake at the population level. J Sleep Res. 30(1):e13119. doi:10.1111/jsr.13119.
- Besedovsky L, Lange T, Haack M. 2019. The sleep-immune crosstalk in health and disease. Physiol Rev. 99(3):1325–1380. doi:10.1152/physrev.00010.2018.
- Brzezinski A, Vangel MG, Wurtman RJ, Norrie G, Zhdanova IV, Ben-Shushan A, Ford I. 2005. Effects of exogenous melatonin on sleep: a meta-analysis. Sleep Med Rev. 9(1):41–50. doi:10.1016/j.smrv.2004.06.004.
- Bubenik GA, Konturek SJ. 2011. Melatonin and aging: prospects for human treatment. J Physiol Pharmacol. 62(1):13–19.
- Buscemi N, Vandermeer B, Hooton N, Pandya R, Tjosvold L, Hartling L, Baker G, Klassen TP, Vohra S. 2005. The efficacy and safety of exogenous melatonin for primary sleep disorders. A meta-analysis. J Gen Intern Med. 20(12):1151–1158. doi:10.1111/j.1525-1497.2005.0243.x.
- Cappuccio FP, Cooper D, D'Elia L, Strazzullo P, Miller MA. 2011. Sleep duration predicts cardiovascular outcomes: a systematic review and meta-analysis of prospective studies. Eur Heart J. 32(12):1484–1492. doi:10.1093/eurheartj/ehr007.
- Cardinali DP, Vigo DE, Olivar N, Vidal MF, Furio AM, Brusco LI. 2012. Therapeutic application of melatonin in mild cognitive impairment. Am J Neurodegener Dis. 1(3):280–291.
- Cavallo A, Ritschel WA. 1996. Pharmacokinetics of melatonin in human sexual maturation. J Clin Endocrinol Metab. 81(5):1882–1886. doi:10.1210/jcem.81.5.8626852.
- Cepeda MS, Stang P, Blacketer C, Kent JM, Wittenberg GM. 2016. Clinical relevance of sleep duration: Results from a cross-sectional analysis using NHANES. J Clin Sleep Med. 12(6):813–819. doi:10.5664/jcsm.5876.
- Chaput JP, Despres JP, Bouchard C, Tremblay A. 2007. Association of sleep duration with type 2 diabetes and impaired glucose tolerance. Diabetologia. 50(11):2298–2304. doi:10.1007/s00125-007-0786-x.
- Chattu VK, Manzar MD, Kumary S, Burman D, Spence DW, Pandi-Perumal SR. 2018. The global problem of insufficient sleep and its serious public health implications. Healthcare (Basel). 7(1):1. doi:10.3390/healthcare7010001.
- Dollins AB, Zhdanova IV, Wurtman RJ, Lynch HJ, Deng MH. 1994. Effect of inducing nocturnal serum melatonin concentrations in daytime on sleep, mood, body temperature, and performance. Proc Natl Acad Sci U S A. 91(5):1824–1828. doi:10.1073/pnas.91.5.1824.
- European Medical Agency. 2007. Circadin: EPAR Assessment Report. EMEA; https://www.ema.europa.eu/en/medicines/human/EPAR/circadin.
- Ferracioli-Oda E, Qawasmi A, Bloch MH. 2013. Meta-analysis: melatonin for the treatment of primary sleep disorders. PLoS One. 8(5):e63773. doi:10.1371/journal.pone.0063773.
- Fourtillan JB, Brisson AM, Fourtillan M, Ingrand I, Decourt JP, Girault J. 2001. Melatonin secretion occurs at a constant rate in both young and older men and women. Am J Physiol Endocrinol Metab. 280(1):E11–22. doi:10.1152/ajpendo.2001.280.1.E11.
- Gooneratne NS, Edwards AY, Zhou C, Cuellar N, Grandner MA, Barrett JS. 2012. Melatonin pharmacokinetics following two different oral surge-sustained release doses in older adults. J Pineal Res. 52(4):437–445. doi:10.1111/j.1600-079X.2011.00958.x.
- Grandner MA, Schopfer EA, Sands-Lincoln M, Jackson N, Malhotra A. 2015. Relationship between sleep duration and body mass index depends on age. Obesity (Silver Spring). 23(12):2491–2498. doi:10.1002/oby.21247.
- Harpsoe NG, Andersen LP, Gogenur I, Rosenberg J. 2015. Clinical pharmacokinetics of melatonin: a systematic review. Eur J Clin Pharmacol. 71(8):901–909. doi:10.1007/s00228-015-1873-4.
- Herxheimer AD, Petrie KJ. 2002. Melatonin for the prevention and treatment of jet lag (Review). The Cochrane Collab. 2.
- Hirshkowitz M, Whiton K, Albert SM, Alessi C, Bruni O, DonCarlos L, Hazen N, Herman J, Katz ES, Kheirandish-Gozal L, et al. 2015. National Sleep Foundation’s sleep time duration recommendations: methodology and results summary. Sleep Health. 1(1):40–43. doi:10.1016/j.sleh.2014.12.010.
- Irwin MR. 2015. Why sleep is important for health: a psychoneuroimmunology perspective. Annu Rev Psychol. 66:143–172. doi:10.1146/annurev-psych-010213-115205.
- Karasek M. 2004. Melatonin, human aging, and age-related diseases. Exp Gerontol. 39(11-12):1723–1729. doi:10.1016/j.exger.2004.04.012.
- Kripke DF. 2016. Hypnotic drug risks of mortality, infection, depression, and cancer: but lack of benefit. F1000Res. 5:918. doi:10.12688/f1000research.8729.1.
- Lemoine P, Garfinkel D, Laudon M, Nir T, Zisapel N. 2011. Prolonged-release melatonin for insomnia - an open-label long-term study of efficacy, safety, and withdrawal. Ther Clin Risk Manag. 7:301–311. doi:10.2147/TCRM.S23036.
- Lemoine P, Nir T, Laudon M, Zisapel N. 2007. Prolonged-release melatonin improves sleep quality and morning alertness in insomnia patients aged 55 years and older and has no withdrawal effects. J Sleep Res. 16(4):372–380. doi:10.1111/j.1365-2869.2007.00613.x.
- Lemoine P, Zisapel N. 2012. Prolonged-release formulation of melatonin (Circadin) for the treatment of insomnia. Expert Opin Pharmacother. 13(6):895–905. doi:10.1517/14656566.2012.667076.
- Liu Y, Wheaton AG, Chapman DP, Cunningham TJ, Lu H, Croft JB. 2016. Prevalence of healthy sleep duration among adults–United States, 2014. MMWR Morb Mortal Wkly Rep. 65(6):137–141. doi:10.15585/mmwr.mm6506a1.
- López-Gamboa M, Canales-Gómez JS, Sandoval TJC, Tovar EN, Mejía MA, Baltazar MAM, Palma-Aguirre J. 2010. Bioavailability of long acting capsules of melatonin in Mexican healthy volunteers. J Bioequiv Availab. 2(5):116–119.
- Mandelkorn U, Genzer S, Choshen-Hillel S, Reiter J, Meira ECM, Hochner H, Kheirandish-Gozal L, Gozal D, Gileles-Hillel A. 2020. Escalation of sleep disturbances amid the COVID-19 pandemic: a cross-sectional international study. J Clin Sleep Med.
- Mangoni AA, Jackson SH. 2004. Age-related changes in pharmacokinetics and pharmacodynamics: basic principles and practical applications. Br J Clin Pharmacol. 57(1):6–14. doi:10.1046/j.1365-2125.2003.02007.x.
- Paruthi S, Brooks LJ, D'Ambrosio C, Hall WA, Kotagal S, Lloyd RM, Malow BA, Maski K, Nichols C, Quan SF, et al. 2016. Consensus statement of the American academy of sleep medicine on the recommended amount of sleep for healthy children: methodology and discussion. J Clin Sleep Med. 12(11):1549–1561. doi:10.5664/jcsm.6288.
- Seixas AA, Vallon J, Barnes-Grant A, Butler M, Langford AT, Grandner MA, Schneeberger AR, Huthchinson J, Zizi F, Jean-Louis G. 2018. Mediating effects of body mass index, physical activity, and emotional distress on the relationship between short sleep and cardiovascular disease. Medicine (Baltimore). 97(37):e11939. doi:10.1097/MD.0000000000011939.
- Sharma M, Palacios-Bois J, Schwartz G, Iskandar H, Thakur M, Quirion R, Nair NP. 1989. Circadian rhythms of melatonin and cortisol in aging. Biol Psychiatry. 25(3):305–319. doi:10.1016/0006-3223(89)90178-9.
- Stamatakis KA, Brownson RC. 2008. Sleep duration and obesity-related risk factors in the rural Midwest. Prev Med. 46(5):439–444. doi:10.1016/j.ypmed.2007.11.008.
- Sun H, Gusdon AM, Qu S. 2016. Effects of melatonin on cardiovascular diseases: progress in the past year. Curr Opin Lipidol. 27(4):408–413. doi:10.1097/MOL.0000000000000314.
- Tobaldini E, Fiorelli EM, Solbiati M, Costantino G, Nobili L, Montano N. 2019. Short sleep duration and cardiometabolic risk: from pathophysiology to clinical evidence. Nat Rev Cardiol. 16(4):213–224. doi:10.1038/s41569-018-0109-6.
- Wade AG, Crawford G, Ford I, McConnachie A, Nir T, Laudon M, Zisapel N. 2011. Prolonged release melatonin in the treatment of primary insomnia: evaluation of the age cut-off for short- and long-term response. Curr Med Res Opin. 27(1):87–98. doi:10.1185/03007995.2010.537317.
- Wade AG, Farmer M, Harari G, Fund N, Laudon M, Nir T, Frydman-Marom A, Zisapel N. 2014. Add-on prolonged-release melatonin for cognitive function and sleep in mild to moderate Alzheimer’s disease: a 6-month, randomized, placebo-controlled, multicenter trial. Clin Interv Aging. 9:947–961. doi:10.2147/CIA.S65625.
- Wade AG, Ford I, Crawford G, McMahon AD, Nir T, Laudon M, Zisapel N. 2007. Efficacy of prolonged release melatonin in insomnia patients aged 55-80 years: quality of sleep and next-day alertness outcomes. Curr Med Res Opin. 23(10):2597–2605. doi:10.1185/030079907X233098.
- Zhdanova IV, Wurtman RJ, Balcioglu A, Kartashov AI, Lynch HJ. 1998. Endogenous melatonin levels and the fate of exogenous melatonin: age effects. J Gerontol A Biol Sci Med Sci. 53(4):B293–298. doi:10.1093/gerona/53a.4.b293.
- Zhdanova IV, Wurtman RJ, Morabito C, Piotrovska VR, Lynch HJ. 1996. Effects of low oral doses of melatonin, given 2-4 hours before habitual bedtime, on sleep in normal young humans. Sleep. 19(5):423–431. doi:10.1093/sleep/19.5.423.