Abstract
Background
Urinary tract infection (UTI) prevention benefits of cranberry intake are clinically validated, especially for women and children. To ensure the benefits of cranberry dietary supplement products, the anti-adhesion activity (AAA) against uropathogenic bacteria is routinely used in in vitro bioassays to determine the activity in whole product formulations, isolated compounds, and ex vivo bioassays to assess urinary activity following intake. D-mannose is another dietary supplement taken for UTI prevention, based on the anti-adhesion mechanism.
Objective
Compare the relative AAA of cranberry and D-mannose dietary supplements against the most important bacterial types contributing to the pathogenesis of UTI, and consider how certain components potentially induce in vivo activity.
Methods
The current study used a crossover design to determine ex vivo AAA against both P- and Type 1-fimbriated uropathogenic Escherichia coli of either D-mannose or a cranberry fruit juice dry extract product containing 36 mg of soluble proanthocyanidins (PACs), using bioassays that measure urinary activity following consumption. AAA of extracted cranberry compound fractions and D-mannose were compared in vitro and potential induction mechanisms of urinary AAA explored.
Results
The cranberry dietary supplement exhibited both P-type and Type 1 in vitro and ex vivo AAA, while D-mannose only prevented Type 1 adhesion. Cranberry also demonstrated more robust and consistent ex vivo urinary AAA than D-mannose over each 1-week study period at different urine collection time points. The means by which the compounds with in vitro activity in each supplement product could potentially induce the AAA in urines was discussed relative to the data.
Conclusions
Results of the current study provide consumers and healthcare professionals with additional details on the compounds and mechanisms involved in the positive, broad-spectrum AAA of cranberry against both E. coli bacterial types most important in UTIs and uncovers limitations on AAA and effectiveness of D-mannose compared to cranberry.
Introduction
There is worldwide concern around increasing antibiotic resistance development to trimethoprim/sulfamethoxazole, fluoroquinolones, nitrofurantoin and other antibiotics used to prevent and treat urinary tract infections (UTIs) according to the Global Antimicrobial Resistance and Use Surveillance System (GLASS) report 2022 (Global antimicrobial resistance and use surveillance system (GLASS) report 2022, Citation2022). In response, many health care professionals are practicing antibiotic stewardship and seeking infection prevention strategies that do not involve repeat use of low dose antibiotics. One approach is daily use of dietary supplement products that prevent uropathogenic bacterial attachment to the uroepithelium, which interrupts the initial step in the urinary tract infection process (Beachey Citation1981). By not killing the bacteria, this anti-adhesion mechanism may reduce the selection pressure for proliferation of resistant bacterial strains thereby preventing resistance development to the product (Klinth et al. Citation2012; Ofek et al. Citation2003). Regular use of these prevention products could potentially slow the pace of antibiotic resistance development by reducing the number of UTIs and ultimate need for antibiotic-based treatments.
In 2023, a large-scale meta-analysis in The Cochrane Database of Systematic Reviews which analyzed results of 50 clinical trials found that consumption of certain cranberry (Vaccinium macrocarpon Ait.) products can prevent and reduce the risk of recurrent UTIs in women, children, and other at-risk individuals (Williams et al. Citation2023). Cranberry dietary supplement products are used worldwide for prevention of UTIs, with their mechanism of action based predominantly on uropathogenic Escherichia coli (E. coli) bacterial anti-adhesion. This bacterial anti-adhesion activity (AAA) was originally discovered in the 1980s (Sobota Citation1984) and successfully disproved the theory that the UTI benefits were due to the acidity of cranberry causing a bacteriostatic effect in urine. In the late 1990s, extensive bioassay-directed fractionation of cranberry fruit using bacterial anti-adhesion assays determined that cranberry proanthocyanidins (PACs), flavan-3-ol oligomers with one or more A-type linkages prevented in vitro AAA (Howell et al. Citation1998, Citation2005; Foo et al. Citation2000a, Citation2000b; Gupta et al. Citation2007) with this in vitro activity, correlating with ex vivo activity in the urine following cranberry intake (Howell et al. Citation2005, Citation2022; Kaspar et al. Citation2015). This activity was detected against P-type uropathogenic E. coli which express fimbriae with PapG adhesins that initiate bladder and upper UTI pyelonephritis kidney infections by binding to a specific galactosyl-galactose structure on uroepithelial cells (Johnson et al. Citation1998). Since then, AAA of cranberry has been detected against Type 1 E. coli expressing fimbriae with FimH adhesins that bind to mannose-like receptors (Bosley et al. Citation2024; Rafsanjany et al. Citation2015; Di Martino et al. Citation2006) causing the majority of lower UTI bladder infections (Rafsanjany et al. Citation2015; Liu et al. Citation2019), implicating PACs (Lavigne et al. Citation2008), flavonols (Scharf et al. Citation2020) and oligosaccharides (Hotchkiss et al. Citation2015) as potential actives. Additional research is needed to definitively determine the specifics of how components are involved in inducing the anti-adhesion effect for both bacterial types.
In 2022, the Cochrane Database of Systematic Reviews on D-mannose for preventing and treating urinary tract infections (Cooper et al. Citation2022) concluded that “there is currently little to no evidence to support or refute the use of D-mannose to prevent or treat UTIs in all populations.” The simple sugar D-mannose is a dietary supplement also marketed to prevent UTIs by the anti-adhesion mechanism, preventing adhesion to uroepithelial cells when excreted in the urine by binding to the FimH adhesin on the Type 1 E. coli fimbrial tip (Hu et al. Citation2016; Kranjčec et al. Citation2014). It has not been shown to have either in vitro or urinary P-type AAA, as E. coli expressing P fimbriae are mannose-resistant. D-mannose is a monosaccharide C-2 epimer of glucose that is important in human metabolism, especially in the glycosylation of certain proteins. D-Mannose can be produced in the human body from glucose, converted into glucose or taken as a supplement. Some fruits contain D-mannose, but cranberries contain very negligible amounts (0.04%–0.14% of DW) (Johnson-White et al. Citation2006) and bioavailability of D-mannose from dietary sources is poor (Yamabhai et al. Citation2016). The two most common sources for commercial D-mannose dietary supplements are corn and birchwood (Hu et al. Citation2016). D-mannose has gained in popularity, and there are some studies that have found benefit following D-mannose supplementation (Kyriakides et al. Citation2021), but many of the interventions, to date, have used combinations of D-mannose with other components, such as cranberry, propolis, and probiotics (Russo et al. Citation2020; Del Popolo and Nelli Citation2018; DE Leo et al. Citation2017; Vicariotto Citation2014), confounding the results and making it difficult to determine the contribution of D-mannose alone. Even though more rigorously well-designed, adequately powered double blind placebo-controlled studies are needed to confirm the in vivo efficacy and safety, D-mannose is currently recognized as having Type 1 in vitro AAA.
For the current study, to obtain robust data on cranberry for comparison to D-mannose on the basis of ex vivo AAA, it is important to test a PAC-standardized cranberry fruit juice dry extract product that has been validated in previous studies to induce significant AAA and is in conformity with the USP guideline monograph: Cranberry Fruit Juice Dry Extract Capsules (Monagas et al. Citation2010). Not all cranberry supplements provide sufficient AAA (Chughtai et al. Citation2016; Mannino et al. Citation2020) and accurate determination of the efficacy of those formulated with different parts of the cranberry can be challenging. In a recent study, two cranberry dietary supplement products were compared for P-type AAA, one a water-soluble dry extract derived from cranberry juice and the other product from mostly skin, seeds and pulp (pomace) left over after the juicing process (Howell et al. Citation2022). The product derived from cranberry juice was highly effective at preventing P-type AAA in the urines of participants that consumed it once daily, compared to the pomace-derived product. The researchers determined that the soluble PAC fraction at 36 mg PAC/serving in the cranberry juice-derived product measured with the DMAC method, as previously described, (Sintara et al. Citation2018; Birmingham et al. Citation2021) with the A2 reference standard was responsible for the in vitro AAA which correlated with the ex vivo urinary AAA (Howell et al. Citation2022). The insoluble PACs present in the pomace-based product were likely complexed with fiber in the skins resulting in lower contribution to urinary AAA. This is a common issue with the majority of cranberry supplements composed of pomace that are tested in clinical trials and do not show a benefit for UTI prevention (Jepson et al. Citation2012). Results of these trials are published with negative results and can influence the overall perception regarding effectiveness of cranberry supplement products for UTI prevention. Thus, obtaining biologically relevant data in studies can be enhanced by utilizing standardized cranberry supplement products derived from cranberry juice with sufficient PAC content.
Given the uncertainty regarding UTI-preventative benefits of D-mannose, the current study evaluates the overall AAA of D-mannose and compare it to that of a well-characterized and previously tested cranberry fruit juice dry extract product (Howell et al. Citation2022; Bosley et al. Citation2024) for Type 1 and P-type AAA in vitro and ex vivo at two collection time-points following intake over each consecutive 24-h period during the 1-week intervention periods when administered in a crossover design to human participants. Fractions of extracted cranberry compounds were tested in vitro to determine which parent compounds could be contributing directly or indirectly to the Type 1 and P-type urinary AAA for cranberry and how active they were when compared to the D-mannose monomer.
Materials and methods
Investigational product information
Two commercially available dietary supplement products sold in the US were purchased online. Product 1: D-mannose (500 mg Veg Capsules, NOW® products) plus excipients (rice flour, stearic acid, magnesium stearate and silicon dioxide) with a recommended serving size of three 500-mg hypromellose vegetable capsules for a total of 1500 mg of D-mannose per serving. Product 1 will be referred to as D-mannose (DM). Product 2: Cranberry fruit juice dry extract capsules (Ellura®, produced by Pharmatoka, distributed by Solv Wellness in the US, and available as a traditional herbal medicine worldwide) with a recommended serving size of one 265-mg hypromellose vegetable capsule containing 206 mg Gikacran® with excipients that were tested independently prior to this study and found not to possess AAA (mannitol, magnesium stearate, silicon dioxide). Gikacran® cranberry fruit juice dry extract contains 36 mg soluble PAC quantified using the DMAC assay with the A2 reference standard and is in conformity with the USP monograph: Cranberry Fruit Juice Dry Extract (Monagas, Citation2023). Product 2 will be referred to as Cranberry Juice Extract (CJE).
Isolation of crude extract fractions from CJE and PAC fraction from cranberry fruit
Fractions of extracted cranberry compounds were prepared for in vitro testing to determine which parent compounds could be contributing to the Type 1 and P-type urinary AAA for cranberry and how active they were when compared to D-mannose. CJE and whole cranberry fresh fruit (Vaccinium macrocarpon Ait. cv. Early Black) collected from fields at the Marucci Center for Blueberry Cranberry Research at Rutgers University and briefly frozen, were subjected to solid-phase chromatography to isolate crude fractions of sugars/acids, anthocyanin/flavonol glycosides and specifically target soluble PACs from the CJE and the soluble PAC fraction from the cranberry fruit as described by Howell et al. (Citation2022). These PAC fractions are not available as marketed products but are only prepared as research samples. Briefly, the CJE sample was dissolved in dH2O and cranberry fruit pulverized in a blender with distilled water (dH2O). Slurries were filtered through cheesecloth and applied to separate C18 SepPak® cartridge (Waters Corp., Milford, MA), preconditioned with methanol (MeOH) followed by dH2O. Cartridges were washed with dH2O then dH2O:MeOH (85:15) (v/v) and acidified aqueous methanol to elute the sugar/acid fraction, which was dried under reduced pressure. Total polyphenolics were then collected from the cartridges with 1% acetic acid (HOAc) in MeOH (v/v), dried under reduced pressure to remove solvent and then suspended in 50% ethanol (EtOH). Fractions were loaded separately onto Sephadex™ LH-20 (Sigma Chemical Co., St. Louis, MO) columns that were pre-equilibrated overnight in EtOH:dH2O (50:50) (v/v). The anthocyanin/flavonol glycoside fraction was collected with 50% EtOH. Elution of the PAC fraction with 80% aqueous acetone was monitored using diode array detection at 280 nm. Acetone was removed under reduced pressure, and the resulting CJE fractions and cranberry fruit PAC fraction were dried and stored in air-tight containers at 4 °C.
Purity of PAC fraction isolated from CJE
HPLC analysis to determine purity of the soluble PAC fraction isolated from CJE was performed by Complete Phytochemical Solutions, LLC, Cambridge, WI, using a Waters Alliance HPLC system with an e2695 separations module and a Waters 2998 PDA detector. PAC sample was separated using an Agilent Zorbax SB-C18 (4.6 mm × 150 mm × 5 µm; PN: 883975-902) HPLC column with an Agilent Zorbax SB-C18 (4.6 mm × 12.5 mm × 5 µm; PN: 820950-920) guard column. The column was held at 40 °C in the column heating compartment. The Waters Empower 3 software was used for data acquisition and integration. Mobile phase A was a solution of 10% formic acid in water and mobile phase B was a composition of (4:1:2.25:2.25) H2O:formic acid:ACN:MeOH. Flow rate for the analysis was 1.0 mL/min with the PDA detector set to collect the UV spectrum from 190–600 nm. The gradient profile was: 7%–25% mobile phase B, 0–35 min; 25%–65% B, 35–45 min; 65%–100% B, 45–46 min; 100% B, 46–50 min; 100%–7% B, 50–53 min. The HPLC column was then equilibrated at 7% B for 10 min after the gradient had finished. Four unique 2D channels were extracted from the collected 3D spectrum of 190-600 nm range: 280 nm (PACs), 320 nm (hydroxycinnamic acids), 370 nm (flavonols) and 520 nm (anthocyanins). These four separate channels were then overlayed on the same chromatogram to facilitate identification of peaks associated with each 2D channel and estimate levels of residual non-PAC compounds.
Authentication of PAC structures in PAC fraction isolated from CJE
Comparison of the soluble PAC structural features in CJE with those from authentic whole cranberry fresh fruit was done to ensure the CJE PACs had a similar profile with characteristic A-type linkages that were not missing or degraded by product processing. Matrix-assisted laser desorption/ionization-time of flight mass spectrometry (MALDI-TOF MS) analysis was performed by Complete Phytochemical Solutions, LLC following AOAC First Action Method: 2019.05 (Esquivel-Alvarado et al. Citation2021). Individual samples were extracted and prepared for MALDI-TOF MS analysis. Data was acquired on three technical replicates (individual wells on the MALDI target) for each PAC sample.
Uropathogenic Escherichia coli strains utilized in bacterial anti-adhesion assays (in vitro and ex vivo)
E. coli expressing P-type fimbriae
E. coli wild-type bacterial strain CPS001 (expresses PapG mannose resistant (MR) adhesins on P-fimbriae) isolated from the urine of a human pyelonephritis patient was obtained from Scripps Medical Laboratory, Scripps Clinic, La Jolla, CA. Strain was subcultured in tryptose broth at 37 °C for 16 h, streaked on colonization factor antigen (CFA) agar plates and grown overnight at 37 °C to enhance production of P fimbriae.
E. coli expressing Type 1 fimbriae
E. coli strain CFT073 (ATCC 700928) (expresses FimH mannose-sensitive (MS) adhesins on Type 1 fimbriae) was subcultured in tryptose broth in a 100 mm tissue culture plate. The plate was swirled to mix the bacteria with the broth and then incubated statically at 37 °C for 12 h. The large surface area of the plate provides oxygen exchange with the tryptose broth enhancing the expression of Type 1 fimbriae.
Both P-type and Type 1 strains were cultured on agar slants at 4 °C for short-term use (1–2 months) and kept frozen at −70 C in tryptose broth (30% glycerol) in cryogenic vials for long-term storage.
P-type and Type 1 E. coli AAA of investigational products and isolated fractions (in vitro)
CJE and DM products were tested for in vitro bacterial AAA on a per weight basis using an improved industry-established mannose-resistant hemagglutination assay (MRHA) specific for uropathogenic P-fimbriated E. coli and mannose-sensitive hemagglutination assay (MSHA) specific for Type 1 E. coli (Bosley et al. Citation2024). E. coli strains CPS001 (expressing P-type fimbriae) and CFT073 (expressing Type 1 fimbriae), both previously tested to confirm fimbrial expression and binding specificity to blood cells used in the assay (Bosley et al. Citation2024) were collected from agar slants, suspended separately in PBS at pH 7.0 and diluted to an optical density (OD) of 0.5 absorbance units (600 nm) to achieve a concentration of 5 × 108 bacteria/mL of PBS. CJE and DM capsule contents were individually suspended (60 mg/mL) in phosphate buffered saline solution (PBS), neutralized to pH 7 with 1 N NaOH and diluted in separate two-fold dilution series in PBS, one to measure P-type AAA (MRHA) and one to determine Type 1 AAA (MSHA). A 90-μL aliquot of each serial dilution was incubated in duplicate with 30 μL of bacterial suspension in a 96-well V-bottomed plate for 15 min at room temperature on a rotary shaker (1000 RPM). The MRHA assay utilized human red blood cells (HRBC), A type Rh + (Innovative Research, Inc., Novi, MI) which selectively agglutinate P-type E. coli but not Type 1. The MSHA assay used guinea pig red blood cells (GPRBC) (Innovative Research, Inc., Novi, MI) which selectively agglutinate Type 1 E. coli but not P-type. HRBC and GPRBC were each suspended (3%) in PBS and 30 μL added to each test well. Suspensions were incubated for 15 min on a rotary shaker at room temperature followed by centrifugation (1,000 × g, 1 min) to concentrate suspended bacteria and blood cells at the bottom of the wells. Wells on each plate were evaluated microscopically for hemagglutination using an Echo Resolve microscope with a 4× objective. The dilution concentration at which hemagglutination activity was suppressed by 50% was recorded as the endpoint for both MRHA and MSHA assays and was considered the minimum inhibitory concentration (MIC). The lower the MIC, the higher the AAA of the sample. Anti-adhesion assays were repeated twice on duplicate samples to verify results. Negative controls included wells containing bacteria + PBS, HRBC or GPRBC + PBS, bacteria + test material, HRBC or GPRBC + test material. Positive control well was bacteria + HRBC or GPRBC. Representative digital photomicrographs were taken of relevant dilution wells to illustrate how the MIC was determined for the MRHA and MRSA assays on CJE and DM products.
The isolated PACs from whole cranberry fresh fruit and the isolated PAC fraction from CJE were suspended separately in PBS and tested for P-fimbriated and Type 1-fimbriated E. coli AAA in a 2-fold dilution series, as described above, with a starting concentration of 5 mg/mL. The MICs for the cranberry fruit PACs and CJE PAC fraction were determined microscopically in the MRHA and MSHA assays. Digital photomicrographs were taken of the dilution series wells of both PAC fractions to allow comparison of results with unfractionated CJE and DM. The other fractions isolated from CJE (anthocyanin/flavonol, and sugars/acids) were also tested using the above procedure to determine the MIC values for AAA.
Urinary P-type and Type 1 E. coli AAA following consumption of CJE and DM (ex vivo)
The Institutional Review Board at Rutgers, The State University of New Jersey approved the ex vivo study protocol 09-243Mx. Prior to enrollment, informed consent was obtained from volunteers in the study. Participants included twenty healthy females and males between the ages of 25 and 60 with no history of current or recurrent UTIs, urinary disorders, diabetes, or antibiotic use within the last six months. Prior to the study, participants were instructed to refrain from consuming D-mannose-containing products, cranberry, lingonberry, blueberry, pomegranate, grape, chocolate, red wine and other high-polyphenolic foods for a 5-day wash out period prior to consuming CJE and DM and throughout the testing period. Fluid consumption was standardized to 240 mL every 3 h to avoid dilution of urine samples and allow for detection of AAA, if present.
Investigational product #1 consisted of three 500-mg DM capsules (taken together for a total intake of 1500 mg D-mannose per serving) and investigational product #2 was one 265-mg CJE (Ellura®) capsule providing 36 mg soluble PAC. Participants were randomized to receive the products which were administered in a repeated measures (crossover) design, with a wash-out period of 5 days between each intervention period of 7 days. All 20 participants self-collected clean-catch baseline urine samples at 7:45 am on day 1, prior to consuming CJE or DM. The first product dose was taken on day 1 at 8 am (time 0) with 240 mL of water, and then subsequent doses every 24 h for 7 days. Fluid consumption was standardized to 240 mL every 3 h during the day. Each day over the 1-week period, participants self-collected clean-catch urine (approximately 25 mL) at the following time intervals: 0 h (just prior to treatment product administration), 8 h and 24 h after product intake (the 24-h time point being equivalent to the 0-h time point for the following day, just prior to the next intake of the product). A total of 30 urine samples were collected from each participant over both 168-h intervention periods after completing both CJE and DM intake regimens. Urines were immediately frozen by participants following each collection and brought to Rutgers Research Center at the conclusion of the intervention where they were kept frozen at −20 C until analyzed.
Urinary AAA was measured according to Howell et al. (Citation2022) using both the MRHA for P-type activity and MSHA for Type 1 activity. Urines were thawed, centrifuged, filtered (0.45 μm filter) and tested full-strength. MRHA activity was measured by pipetting a 30-μL drop of each urine onto 24-well polystyrene plates and incubating with 10 μL of P-type E. coli bacterial suspension at 5 × 108 bacteria/mL of PBS for 10 min at room temperature on a rotary shaker. Freshly drawn A + HRBCs were suspended (3%) in PBS and 10-μL drops were added to test suspensions, which were then incubated for 20 min on a rotary shaker at room temperature and evaluated microscopically for the ability to prevent agglutination. For MSHA activity, the procedure was repeated but with Type 1 E. coli bacterial suspension and GPRBC. AAA of each urine sample was scored visually based on a semi-quantitative estimation of percent agglutination of each sample according to the following scale: 0 = no AAA, 1 = 50% AAA (moderate activity), 2 = 100% AAA (high activity). Anti-adhesion assays were repeated four times per sample to ensure consistent results. Controls included wells containing bacteria + PBS, HRBC or GPRBC + PBS, bacteria + test material, HRBC or GPRBC + test material, and bacteria + HRBC or GPRBC.
Data were analyzed to determine the effects of CJE and DM intake on Type 1 and P-type bacterial AAA in urine samples collected from participants over each 1-week period in the crossover study. Average percent urinary AAA/time period was calculated by adding the AAA levels (0 = no AAA, 1 = 50% AAA, 2 = 100% AAA) for each participant at each time period and dividing this number by the highest score possible (in this case if all 20 participant urines had a score of 2, the highest possible total score for that time period would be 40), then converting this to a percentage (100%). Participants with positive background urines were excluded from the average percent urinary AAA calculations. Maximum AAA level (0, 1 or 2) reached in urines after intake of CJE or DM over the 1-week intervention period was determined and analyzed statistically using the bootstrap method with a randomization 1-sample randomization t-test and the nonparametric Wilcoxon test. Area Under the Curve (AUC) was calculated for both types of bacteria, on each day and for each participant. The AUC of AAA was calculated as follows: AAA16H = (AAA8H+AAA24H)/2. AUC for a given day was calculated as: AUC = (AAA0H+AAA8H)/2 + (AAA8H+AAA16H)/2 + (AAA16H+AAA24H)/2 (AAA24H corresponded to AAA0H of the following day). The maximal score was 6 and the minimal score was 0. Since there was one AUC for CJE and one for DM each day, the difference between the two values was calculated using analysis of variance with one within-case factor.
Measurement of urine pH during ex vivo urine collections
All urines collected by participants over the 7-day intervention period were tested for pH values to determine if urine pH was affected by intake of the treatment products. The pH levels in urines of each participant taken at hour 0 as background collections were compared to the average pH levels of each participant’s urines collected during the CJE and DM intake periods from hour 8 to hour 168 over each 1-week intervention period.
Results
Purity and authentication of PAC structures in PAC fraction isolated from CJE
HPLC purity analysis of the PAC fraction isolated from CJE indicated that PACs were the predominant compound in the extract (Supplementary Figure 1). Overlaid chromatograms show the presence of very high levels of PAC (280 nm) with low level detection of hydroxycinnamic acids (320 nm), and very minimal detection of flavonols (370 nm) and anthocyanins (520 nm).
Results of the MALDI-TOF MS positive reflectron mode analysis of the soluble PAC fraction isolated from CJE () indicate that the structural features of these PACs are similar to those isolated from fresh cranberry fruit () and have the typical A-type linkages. Deconvolution of the MALDI-TOF spectra shows both cranberry fruit PACs and CJE PACs have predominantly one or more A-type interflavan bonds at each degree of polymerization ().
Figure 1. (A) MALDI-TOF MS positive reflectron mode analysis of the PAC fraction from Ellura® cranberry juice extract (CJE) (A) and the PAC fraction from whole cranberry fresh fruit (B), preceded by Sephadex LH-20 clean up. The predominate oligomeric distribution (Δ 288 amu) (m/z = 887, 1175, 1463, 1751, 2039) is representative of PACs that contain at least one A-type interflavan bond at each degree of polymerization from trimer to heptamer. All PACs are detected as sodium ion adducts [M + Na]+.
![Figure 1. (A) MALDI-TOF MS positive reflectron mode analysis of the PAC fraction from Ellura® cranberry juice extract (CJE) (A) and the PAC fraction from whole cranberry fresh fruit (B), preceded by Sephadex LH-20 clean up. The predominate oligomeric distribution (Δ 288 amu) (m/z = 887, 1175, 1463, 1751, 2039) is representative of PACs that contain at least one A-type interflavan bond at each degree of polymerization from trimer to heptamer. All PACs are detected as sodium ion adducts [M + Na]+.](/cms/asset/3432858e-5c36-4345-9975-911303976e67/ijds_a_2356592_f0001_b.jpg)
Table 1. Deconvolution of positive reflectron mode MALDI-TOF MS showing cranberry fruit PAC and CJE PAC samples containing PAC oligomeric distribution from trimers through hexamers, with predominantly one or more A-type bonds at each degree of polymerization.
P-type and Type 1 E. coli AAA of investigational products and isolated fractions (in vitro)
Results of in vitro AAA testing against P-type and Type 1 E. coli of DM, CJE, CJE fractions (sugar/acid, anthocyanin/flavonol glycosides, soluble PACs), and cranberry fruit soluble PAC fraction are expressed as the MIC (mg/mL) required to inhibit bacterial adhesion by 50% (). DM inhibited adhesion of Type 1 E. coli with a MIC of 0.23 mg/mL, however it did not inhibit mannose-resistant P-type E. coli at any concentration, as expected. CJE inhibited both P-type and Type 1 E. coli with MICs of 0.23 mg/mL and 0.47 mg/mL, respectively. The CJE sugar/acid and anthocyanin/flavonol glycoside fractions expressed low AAA (high MICs) against Type 1 E. coli but did not elicit AAA with P-type E. coli. Both PAC fractions from CJE and cranberry fruit significantly inhibited AAA with very low MICs for P-type E. coli (0.06 mg/mL) and Type 1 (0.12 mg/mL). Controls all performed as expected. Photomicrographs of the MIC results for DM, CJE and PACs isolated from CJE following hemagglutination of HRBCs (measuring P-type AAA) and GPRBCs (measuring Type 1 AAA) are presented to illustrate how the AAA of the isolated PACs compares to the AAA of whole investigational products against each bacterial type (). The AAA scale, as previously described (Bosley et al. Citation2024) may be useful for estimating potential in vivo efficacy of the products and isolated fractions (). The scale (low/medium/high) references the correlation of in vitro AAA results with ex vivo urinary post-consumption AAA data in previous studies (Howell et al. Citation2005, Citation2010, Citation2022; Kaspar et al. Citation2015).
Figure 2. Representative photomicrographic images of P-type and Type 1 E. coli in vitro anti-adhesion activity (AAA) results expressed as minimum inhibitory concentration (MIC) of D-mannose (DM), Ellura® cranberry juice extract (CJE) and PAC fraction isolated from CJE using hemagglutination-based assays. The dilution series from 60 mg/mL–0.03 mg/mL is presented along the top row and the MIC results for each test sample are displayed in each column. Yellow star indicates resulting MIC dilution required to suppress hemagglutination of bacteria by 50% in each test run. *Scale of relative AAA represents potential in vivo efficacy of samples. (See Bosley et al. Citation2024, for further explanation of the scale).

Table 2. P-type and Type 1 E. coli in vitro bacterial anti-adhesion activity (AAA) of investigational products and crude extract fractions from cranberry juice extract (CJE) product and whole cranberry fresh fruit.
Urinary P-type and Type 1 E. coli AAA following consumption of CJE and DM (ex vivo)
Participant response to each treatment
Urinary P-type and Type 1 E. coli AAA levels at all 15 urine collection time points/participant prior to and after intake of either CJE (one 265 mg capsule) or DM (three 500-mg capsules) every 24 h for 7 days is presented in and Supplementary Table 1. AAA is expressed as 0 = no activity, 1 = 50% activity, 2 = 100% activity. Over the course of the 1-week interventions following CJE intake, all 20 participants responded (expressed both Type 1 and P-type E. coli urinary AAA level of either 50% or 100%) in at least one urine collection time period; however, after DM intake, 15% of participants did not express Type 1 E. coli AAA in any urine collection time period and 90% did not respond when tested for mannose-resistant P-type AAA, as expected.
Figure 3. Urinary Type 1 and P-type E. coli (ex vivo) anti-adhesion activity (AAA) of all participants at each urine collection time point at time 0 and after intake of either cranberry juice extract (CJE) (Ellura®) or D-mannose (DM) over each 1-week study period. AAA level 0 = no activity, 1 = 50% activity, 2 = 100% activity.

Positive AAA in background urines
Urine samples tested for Type 1 AAA (but not P-type AAA) were positive at the time 0 background collection as follows: 20% (4/20) participant urines were positive at time 0 following DM intake, and 15% (3/20) were positive at time 0 after CJE intake resulting in n = 16 for DM and n = 17 for CJE (Supplementary Table 1). Three of the participants had positive Type 1 AAA backgrounds after both DM and CJE intake. Type 1 AAA data from participants with positive background urines was not included in the data analyses to determine average percent AAA at 8 and 24 h following DM or CJE ingestion because it would not have been possible to determine the AAA contributions of the DM or CJE products vs an endogenous inhibitor with Type 1 AAA that was present in the background urines and likely also in other urine collections taken by those participants.
Urinary AAA by time-period
There were no significant trends for increasing or decreasing AAA effect from days 1 to 7 for either treatment, indicating no changes in persistence. Following intake of CJE, average percent P-type urinary AAA remained fairly consistent over all time periods (Supplementary Table 2) but was more variable for Type 1 activity over the course of the 1-week intervention (Supplementary Table 3). At 8 h after each 24-h CJE intake over the week, P-type AAA averaged 69.6% and ranged from 60-80%, while Type 1 AAA averaged 37.5% and ranged from 30%–40% ( and ). At 8 h after each 24-h DM intake, P-type AAA averaged 0.36% ranging from 0%–2.5% while Type 1 AAA averaged 21.9% ranging from 9.4%–37.5%. At 24 h after each intake of CJE, P-type AAA averaged 20% and ranged from 12.5%–25% and Type 1 AAA averaged 12.1% ranging from 7.5%–17.5% ( and ). At 24 h after each intake of DM, P-type AAA averaged 0.36% ranging from 0%–2.5% and Type 1 AAA averaged 4.64% ranging from 0%–18.75%.
Figure 4. Cranberry juice extract (CJE) and D-mannose (DM) P-type and Type 1 E. coli average percent urinary AAA of all participants (ex vivo) at urine collections 8 h after each product intake time period. Urine collections of participants with positive AAA background samples at time 0 (4/20 for Type 1-DM and 3/20 for Type 1-CJE) were removed from the data analysis to allow AAA contributions of the DM or CJE products to be determined without being confounded by endogenous inhibitor with Type 1 AAA that was present in the background urines and likely present in the other urine collections taken by those participants.
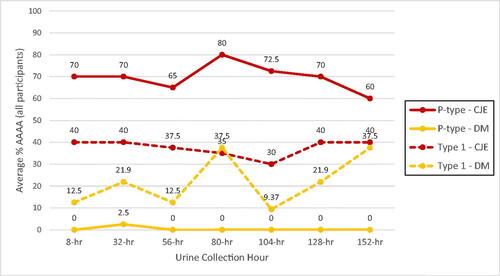
Figure 5. Cranberry juice extract (CJE) and D-mannose (DM) P-type and Type 1 E. coli average percent urinary AAA (ex vivo) of all participants at urine collections 24 h after each product intake time period. Urine collections of participants with positive AAA background samples at time 0 (4/20 for Type 1-DM and 3/20 for Type 1-CJE) were removed from the data analysis to allow AAA contributions of the DM or CJE products to be determined without being confounded by endogenous inhibitor with Type 1 AAA that was present in the background urines and likely present in the other urine collections taken by those participants.
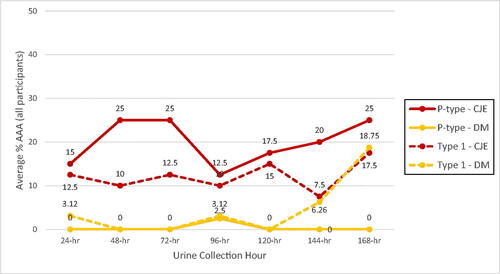
Table 3. P-type and Type 1 E. coli average precent ex vivo anti-adhesion activity (AAA) of urine at each 8-hr and 24-hr urine collection from all participants after each 24-hr intake of CJE and DM investigational products over each 1-week study period, and the total average percent AAA over all time periods and participants combined, and the overall percentage of participants that did not respond to the products in any urine-collection time period.
CJE intake resulted in more urinary AAA scores of 2 (100% AAA) at each urine collection time point than DM against both bacterial types. For P-type bacteria, the difference was highly significant favoring CJE, and for Type 1 bacteria, the difference was significant favoring CJE as well ().
Table 4. Highest median AAA scoreTable Footnote1 reached in participant urine at each urine collection time point following intake of CJETable Footnote2 and DMTable Footnote3 over the 1-week period. Summary of statistical results analyzed using the bootstrap method with a randomization 1-sample randomization t-test and the nonparametric Wilcoxon test.
Urinary AAA by participant
For each participant, the number of times a urinary AAA score of 2 was achieved in each 1-week treatment period (range: 0–14) was higher after CJE intake than after DM intake (). For P-type bacteria, there was a highly significant difference favoring CJE on the number of times AAA scores of 2 were generated during the whole duration of each study period (p < .001). Following DM intake, AAA scores of 2 were never reached. For Type 1 bacteria, there was a trend favoring CJE (.05 < p < .1) for the total number of AAA scores of 2 during the whole period of administration.
Table 5. Total number of times an AAA score of 2Table Footnote1 was achieved following intake of CJETable Footnote2 and DMTable Footnote3 in each 1-week treatment period for each participant. Summary of statistical results analyzed using the bootstrap method with a randomization 1-sample randomization t-test and the nonparametric Wilcoxon test.
Overall AAA by bacterial type
AUC data demonstrated that the overall P-type AAA is about 33% higher after intake of CJE than DM with a p value < .001 (F = 4.54, 19, 139) and about 13% higher Type 1 AAA after CJE than DM (not significant) (Supplementary Tables 2 and 3).
Urinary pH levels over the course of the ex vivo urine collections
Urinary pH did not change significantly from the pre-product intake background collections of all participants when compared to the average pH of urines at each collection after CJE and DM were consumed. Prior to DM intake, background urine samples for all participants averaged 6.06 and after the 7-day DM intervention period, average pH of all urines was 5.89. Prior to CJE intake, background urines averaged 5.99 and after the CJE intervention, pH averaged 5.74.
Discussion
Consumers are faced with an overwhelming number of choices when it comes to selecting a dietary supplement for maintaining urinary tract health. Bacterial anti-adhesion is an important mechanism of action of both cranberry and D-mannose supplements in promoting urinary tract health, but there are still many unanswered questions regarding their effectiveness. It is not only valuable to assess the overall AAA of these products, but also determine their ability to prevent AAA against both types of E. coli bacteria that can lead to UTIs and begin to further the understanding of what compounds or processes may be responsible for the effects. Since it is not possible to know if a future UTI will be caused by P-type or Type 1 E. coli, it is preferable for consumers to rely on a prophylactic product that will have sufficient AAA against both bacterial types (broad spectrum AAA).
Given that studies have shown that cranberry supplement products are most effective clinically when they contain soluble PACs that are derived from cranberry juice, there is ongoing research to determine details of the anti-adhesion mechanism. The structure-activity of PACs is complicated in that they are oligomeric molecules with limited absorption, but this does not prevent the parent PAC molecules in cranberry supplement products from inducing AAA in urine by indirect mechanisms in the gut. Even though only a small fraction of PACs (∼1%) are themselves metabolized to bioactive metabolites with a high degree of interindividual variability based on existing composition of each person’s specific microbiome and dysbiotic state (Diaz et al. Citation2024; Lessard-Lord et al. Citation2024), PACs have a high degree of resistance to the acidic conditions in the gut, where their complex structures interact with the microbiome to encourage growth of beneficial probiotic species, such as Akkermansia which can produce post-biotic metabolites, especially specific valerolactones with bacterial AAA in urine (Monagas et al. Citation2010; Lessard-Lord et al. Citation2024; Déprez et al. Citation2000). They can elicit indirect effects on gut-based immunity and cell signaling in other parts of the body involving processes such as bacterial quorum sensing (Ulrey et al. Citation2014; Feldman et al. Citation2009) and excretion of Tamm-Horsfall glycoprotein (Scharf et al. Citation2019) that can potentially affect adhesiveness of the urine. In one study that discounted the contribution of PACs to AAA (Rafsanjany et al. Citation2015), a cranberry formulation was used in the ex vivo studies that likely contained very low levels of soluble PAC and high amounts of pomace with non-extractable, insoluble PACs that do not contribute to AAA because they are bound to fiber (Roopchand et al. Citation2013; Gullickson et al. Citation2020). In this and other studies, sub-efficacious levels of PAC in cranberry formulations are often not recognized because the European Association for the Valorization of Cranberry (EuraCran) quantitation method was used that overestimates PAC content because it includes the anthocyanin fraction that is not removed prior to spectral analysis (Waterhouse et al. Citation2000; Krueger et al. Citation2013), or HPLC was used with either reverse-phase C18 columns that do not resolve A-type PACs, or normal-phase columns that bind higher oligomeric PACs and elute them in the wash phase which obscures their presence (Sintara et al. Citation2020). Determination of the soluble PAC levels is most accurately done using the DMAC method which is used preferentially in the cranberry industry and is less likely to have interferences from other sample components (Cunningham et al. Citation2002; Krueger et al. Citation2013; Sintara et al. Citation2018; Birmingham et al. Citation2021). These product PAC levels can then be accurately referenced when determining anti-adhesion bioactivity.
In the present study, the more neutral urine pH data following intake of CJE and DM confirmed that the effects were due to the anti-adhesion mechanism and not bacteriostatic. Bioassays were used that have specificity for detecting in vitro and urinary ex vivo AAA against P-type and Type 1 separately, improving the biological relevance of the results. Distinguishing which fimbriae are expressed is important because most strains of uropathogenic E. coli can express both P-type and Type 1 fimbriae, depending on growth conditions (Melican et al. Citation2011). Uroepithelial cell (T24) anti-adherence binding assays (Scharf et al. Citation2020) are not able to distinguish the difference between AAA of P-type and Type 1 strains because bladder cells have surface receptors for both strains. The selective hemagglutination assays used in this study agglutinate different types of red blood cells (RBCs) when E. coli fimbriae bind to their surfaces. P-fimbriated E. coli (PapG) agglutinate human RBCs with A + or O + Rh factor and Type 1-fimbriated E. coli (FimH) induce agglutination of guinea pig RBCs (Hagberg et al. Citation1981). P-type E. coli recognize and bind to P blood group antigens on the surface of human RBCs and Type 1 E. coli recognize and bind to mannose-like structures on guinea pig RBCs (Klinth et al. Citation2012). Bacterial strain selection for use in the assays was also important because there is a high variability in strain expression of fimbrial virulence factors in culturing due to environmental fluctuations such as temperature, pH, osmolarity and nutrient availability (Mulvey Citation2002). Pre-testing the strains using the correct bioassay was done to determine degree of hemagglutination prior to introducing the challenge molecules. For this study, P type and Type 1 strains were selected based on ability to 100% agglutinate their specific RBC in the assay. These strains were also successfully used in a previous study (Bosley et al. Citation2024) and confirmed to produce the correct fimbriae at 100% under controlled culture conditions.
The ex vivo study was designed to evaluate residual urinary AAA in CJE and DM over each 24-h period for 7 days, with each product serving being administered in the mornings each day and urines collected 8 and 24 h after each intake. Absorption and excretion times for DM can be variable but can continue over the course of 8 h (Ala-Jaakkola et al. Citation2022). In previous studies, cranberry juice-based products have exhibited urinary AAA between 3 and 12 h, with residual activity at 24 h (Howell et al. Citation2022; Kaspar et al. Citation2015; Liu et al. Citation2019). Given this variability and the limited number of study participants, collection time points were chosen in the current study to determine if AAA is induced from both products over the entire 24-h period following ingestion and how relatively protective they may be at various time points between daily intake servings.
The significant ex vivo urinary P-type AAA results for CJE were consistent with previous findings on other juice-based cranberry supplement products (Howell et al. Citation2005, Citation2015, Citation2022). High activity at 8 h, some residual activity at 24 h, good responses by all participants to the product, and high number of AAA scores of 2 by participants (representing 100% AAA) suggests a potential protective effect with CJE between daily intakes. The high in vitro AAA of the isolated soluble PACs from CJE, which are at the 36 mg level in the product, and lack of AAA of the other fractions (sugar/acids, anthocyanins/flavonols) are consistent with previous findings that the soluble PACs in products are involved in inducing the urinary AAA, most likely through gut-based mechanisms as opposed to direct metabolic breakdown of PACs. The structural consistencies between the PACs isolated from CJE and from cranberry fruit and the identical high in vitro P-type AAA of both suggest that there were no processing issues that damaged the PAC structures in CJE.
The negative in vitro and urinary P-type AAA of DM and lack of response by all participants indicates that DM does not prevent bacterial adhesion against E. coli with PapG adhesin virulence factors, which are implicated in upper UTI and pyelonephritis kidney infections and may not be protective. These results are not unexpected, given that P-type E. coli, by definition, are mannose resistant.
The higher in vitro Type 1 activity exhibited by DM compared to CJE did not translate to higher ex vivo urine AAA for DM. In fact, CJE had consistently higher and more stable ex vivo activity at both 8 and 24 h than DM and 15% of the participants did not express Type 1 AAA in any urine collection time period after intake of DM. Non-responders of 50% following D-mannose intake were noted in a recent study (Fan et al. Citation2023). More research is needed with additional urine collection time points to get a more complete picture around maximum excretion times and potentially higher ex vivo AAA earlier in the day for DM, especially if DM is dosed more than once in 24 h. But what these results do indicate is that DM may not provide sustained and consistent protection against Type 1 bacterial adhesion between daily intake periods of 24 h. This result is consistent with recent meta-analysis data concluding that, due to poorly controlled clinical study design issues, it has been difficult to determine whether D-mannose intake results in sufficient stable urinary concentrations to elicit an anti-adhesion effect that is biologically relevant for UTI prevention and is consistent enough among multiple demographics (Cooper et al. Citation2022).
The urinary activity exhibited following DM intake may be due to the direct absorption of D-mannose into the urine, but research indicates that monomers of D-mannose may not bind strongly to mannose bladder receptors. A 2017 landmark study found that D-mannose blocked FimH binding in vitro, however treating mice with D-mannose did not alter bacteria levels in vivo. In the study, they showed that by using highly specialized engineered mannosides, they could achieve much better results on prevention of Type 1 adhesion and could be developed as effective pharmaceuticals. They found that biphenyl mannoside FimH inhibitors binding affinity for FimH is ∼100,000-fold higher than D-mannose (Spaulding et al. Citation2017). There are mannose receptors on tissues throughout the entire body which bind mannose residues on many different compounds that control numerous critical biological processes, such as immune responses and glycosylation of proteins (Lee et al. Citation2002; Gazi and Martinez-Pomares Citation2009). There is concern by some researchers that regular high intakes of D-mannose, often multiple times per day over time, on top of the body’s own catabolic production of D-mannose, would saturate mannose receptors and result in side effects with whole-body ramifications related to circulation of toxic end products and immunity (Scharenberg et al. Citation2012). Mydock-McGrane et al. (Citation2016) stated: “Mannose can be a therapeutic, but indiscriminate use can have adverse effects.” Additionally, D-mannose is not recommended for diabetics or those with a history of insulin resistance, since it has been shown in some studies to trigger insulin secretion, making blood sugar control more difficult (Pitkänen Citation1996). Thus D-mannose intake is not a simple process of excretion in the urine followed by receptor binding of Type 1 bacteria happening in a “mechanical” way without the D-mannose molecules interacting with or influencing bodily processes, as has been implicated (Scaglione et al. Citation2023), but rather involves metabolic and pharmacological steps resulting in changes in the adaptive immune system, glycoprotein homeostasis, and insulin secretion. Additional research is needed to further elucidate these effects. There is however a role for engineered mannosides that target mannose receptors at very low dosages and high specificity, thereby minimizing potentially significant side effects while offering effective prevention of Type 1 bacterial adhesion to bladder cells.
Interestingly, in the present study prior to taking the first dose of DM, 20% of the background urines tested for Type 1 AAA were positive and exhibited AAA across some time periods. This likely indicates the presence of the endogenous Type-1 AAA inhibitor Tamm-Horsfall protein (THP) or uromodulin in the urines which is a common highly mannosylated hormone-like glycoprotein produced by the kidneys under different conditions at variable concentrations (Reinhart et al. Citation1990). Water and salt intake, vasopressin hormone secretion and other variables have been linked with variations in THP production (LaFavers et al. Citation2022). Urinary excretion of THP is characterized by significant fluctuations that occur both within and among individuals (Olden et al. Citation2014). Maximum excretion times for THP are extremely variable, according to a population cohort of urine samples from 6500 people (Pruijm et al. Citation2016). THP binds in a dose-dependent manner to the FimH adhesin of Type 1 E. coli decreasing interaction with uroplakin, a FimH-binding protein on bladder cells (Säemann et al. Citation2005). It has many other immunological functions, which are still being elucidated (Weichhart et al. Citation2005). In the current study, calculating the overall percent AAA following DM intake and including the data on participants with positive background urines, there is an overall 20.89% anti-adhesion effect, but after recalculating following removal of data with positive background samples, the overall percent AAA after DM intake drops to 13.1%. Since THP excretion is known to fluctuate (Olden et al. Citation2014; Pruijm et al. Citation2016), the variable data seen here over urine collection time-points after DM intake could be due to THP excretion and not exclusively to D-mannose monomers. Additional studies on D-mannose intake that measure THP in urines could provide more details as to the relative contribution of D-mannose vs. endogenous THP.
CJE ingestion resulted in more muted urinary AAA against Type 1 than against P-type E. coli, however Type 1 AAA was higher for CJE overall and more consistent at all urine collection time points than it was for DM. The overall Type 1 AAA after CJE intake by all 20 participants was 33.93%, and after removing those participants with positive background urines, the overall AAA was 29.2%, indicating possible THP induction by CJE influencing Type 1 AAA in each urine collection. Previous studies have found an increase in THP excretion that positively correlated with Type 1 AAA when cranberry powder was administered to humans (Scharf et al. Citation2019, Citation2020). The researchers speculated that the activity of cranberry against Type 1 bacteria may be due to certain cranberry B-ring substituted flavones and flavonols stimulating expression of THP. Results of the current study did reveal some in vitro Type 1 AAA for the flavonol/anthocyanin fraction which could have resulted in stimulation of THP and/or production of bioactive urinary metabolites. Further studies on the flavonol fraction from cranberry fruit are needed to further elucidate this. The low-moderate in vitro Type 1 AAA of the sugar/acid fraction was likely due to the fructose component, which in a previous study elicited scant AAA that was 10 times lower than the methyl α-mannoside control, but would not result in an in vivo effect in urine due to complete fructose metabolization (Zafriri et al. Citation1989). Other simple sugars in cranberry include (per 100 g of raw cranberries): glucose (3.44 g), fructose (0.67 g), and sucrose (0.16 g) (Nemzer et al. Citation2022), but only fructose has Type 1 in vitro AAA (Zafriri et al. Citation1989). The amount of D-mannose in cranberries is negligible (0.04–0.14% of DW) (Johnson-White et al. Citation2006) (also confirmed for CJE through a Certificate of Analysis provided by the producer of CJE indicating 0.04 g D-mannose/100 g CJE) and bioavailability from dietary sources is poor, so it is also unlikely to be contributing to Type 1 AAA. On the other hand, the PAC fractions from both CJE and cranberry fruit had high in vitro Type 1 AAA and exhibited identical MICs of 0.21 mg/mL in the dilution series in . It is unclear how the PACs are eliciting significant in vitro AAA through a process of competitive inhibition since their structures do not have mannose moieties. It may be that the PACs are binding directly to the bacteria and forming aggregates that inhibit the bacteria’s ability to bind (Urena-Saborio et al. Citation2021). Further research is needed to determine if cranberry PACs are also stimulating the kidneys to excrete THP or promote other anti-adhesive processes to account for a portion of the urinary Type 1 AAA exhibited in the current study. Ideally, ingesting the pure cranberry PACs and obtaining ex vivo urinary data would be ideal, but these compounds in isolated form would require an Investigational New Drug (IND) application.
Given the issues with antibiotic resistance and the consumer need for research-supported dietary supplements with high bacterial AAA that do not promote resistance development, these current results indicate that the cranberry juice-derived supplement product in this study standardized to contain 36 mg soluble PAC could provide more consistent, broad-spectrum AAA against both P-type and Type 1 uropathogenic E. coli with low potential side effects when compared to D-mannose products taken on a daily basis for maintenance of urinary tract health. Additional studies further elucidating the details on how the compounds in cranberry, especially the PACs induce the beneficial effects on bacterial anti-adhesion of both bacterial types are needed.
Supplemental Material
Download MS Word (414.7 KB)Acknowledgements
ABH, CGK, JDR, and BC designed research; ABH, SB and AB conducted research; ABH, JFD, CGK and JDR analyzed data; and ABH, CGK, and JDR wrote the paper. ABH had primary responsibility for final content. All authors read and approved the final manuscript.
Disclosure statement
Scott Bosley and Andrew Birmingham are employed by Complete Phytochemical Solutions, LLC, and Christian G. Kruger, Amy B. Howell and Jess D. Reed have ownership interest.
Data availability statement
Data described in the manuscript will be made available upon request pending approval.
Additional information
Funding
Notes on contributors
Amy B. Howell
Amy B. Howell, PhD, has been an Associate Research Scientist (now retired) at the Marucci Center for Blueberry Cranberry Research at Rutgers University in Chatsworth, NJ, since 1993, and a founding member of Complete Phytochemical Solutions, LLC. She has been engaged in research that targets utilizing cranberry for prevention and management of bacterial diseases, including urinary tract infections (UTIs), stomach ulcers, and periodontal disease. Her primary research focus has been on isolating polyphenolic compounds from cranberry and determining their role in prevention of UTIs and collaborating on ex vivo clinical studies to determine effects of cranberry proanthocyanidins on uropathogenic bacterial adhesion in urine. She studied the pharmacokinetics and bioavailability of the structurally unique cranberry proanthocyanidins in an effort to determine site(s)of action and dose response. Dr. Howell has been involved in method development for powdered cranberry supplements, working closely with regulatory teams from AOAC and USP (US Pharmacopeia) to determine standard methods for quantification of the bioactive compounds in cranberries.
Jean-François Dreyfus
Jean-François Dreyfus, MD, PhD, is currently senior consultant at Dreyfus Research Consulting International (DRCI) in Bouère, France. He was trained as an MD, psychiatrist and neuropsychopharmacologist at Universite Rene Descartes, Paris and obtained a PhD in Computer Science at Faculte des Sciences, Paris. He has been working as head of clinical development for several drug companies and contributed to the development/registration of ten different drugs in CNS, GI, allergy/asthma, diabetes and cancer, as well as dietary supplements for patients with cystic fibrosis. Before retiring, he was appointed, chief methodologist and statistician at Hopital Foch, a private academic institution near Paris with major responsibilities for studies mainly in pharmacoeconomics, anesthesiology and urology. He has published several books and over 100 papers in peer reviewed journals.
Scott Bosley
Scott S. Bosley is a Scientist for Complete Phytochemical Solutions, LLC. Graduated from the University of Wisconsin – Madison in 2020 with a Master of Science in Molecular and Cellular Pharmacology. He is focused on developing assays to test the biological efficacy of dietary supplements.
Christian G. Krueger
Christian G. Krueger has been a Research Program Manager at the University of Wisconsin-Madison since 1995 and a founding member and Chief Executive Officer of Complete Phytochemical Solutions, LLC since 2010. He specializes in the development, validation and harmonization of methods for the authentication and quantification of bioactive compounds in dietary supplements, foods and beverages. Christian has published over 80 peer-reviewed manuscripts and holds numerous patents on natural product technologies. He is a member on several AOAC International Expert Review Panels, an Expert Reviewer with the AOAC Research Institute’s Performance Tested MethodSM Program, a member of the American Herbal Products Association’s Psychedelic Plants and Fungi Committee, a member of the American Botanical Council’s Botanical Adulterants Prevention Program and works closely with the United State Pharmacopeia (USP) to assist in development of monographs for the Dietary Supplement Compendia. He is an invited Advisory Council Member for Sonoran University of Health Sciences. His expertise in natural product chemistry, understanding of natural products supply chain management (growers, processors, formulators, and retail) and research experiences relating phytochemical structures to biologic function provides a unique skill set and background.
Andrew Birmingham
Andrew Birmingham is the Director of Analytic Service at Complete Phytochemical Solutions, LLC. He received his bachelor’s degree in biomedical biology from the University of Wisconsin-La Crosse and a master’s degree from the University of Wisconsin-Madison. While managing the laboratory, he is in the process of developing and maintaining a quality management system at Complete Phytochemical Solutions, LLC for ISO 17025:2017 accreditation.
Jess D. Reed
Jess Reed, PhD, is Emeritus Professor of Nutrition at the University of Wisconsin-Madison with 40 years of postgraduate experience in research on the effects of dietary phytochemicals on the nutrition and health of animals and humans. He also directs the Reed Research Group (RRG) which consists of 3 core programs: Phytochemistry, Cardiovascular and Mucosal Immunity. The main thrust of their Phytochemistry Research Core is to determine the effects of oligomeric polyphenols (tannins) in foods on disease processes. This research effort includes development of phytochemical methods for characterization of structure and their interactions with proteins and polysaccharides, and mechanistic studies on the effects of tannins in cell culture and animal models of disease. He and his group are currently studying the relationship between tannin structure and the ability of tannins to inhibit the adherence, invasion and colonization of mucosal epithelial cells by extra-intestinal pathogenic E. coli and other bacteria that infect the GI tract, the urogenital tract and mammary gland. Dr. Reed is also a founding member and Chief Science Officer of Complete Phytochemical Solutions, LLC (CPS) since 2010. CPS is a provider of contract research and analytical services for the food industry.
Bilal Chughtai
Bilal Chughtai, MD, is currently an Associate Professor of Urology specializing in Female Pelvic Medicine and Reconstructive Surgery and Male Voiding Dysfunction. In addition to a successful clinical practice, he has been an investigator in numerous clinical trials, as well as translational, and outcomes research including National Institute of Health and industry support. He is a reviewer for several prestigious journals such as Lancet, JAMA Surgery, European Urology, Journal of Urology, Urology as well as an editor for several urological journals. He has published over 175 peer-reviewed articles, authored chapters in several urologic texts, published 3 textbooks, and has presented at numerous national meetings.
References
- Ala-Jaakkola R, Laitila A, Ouwehand AC, Lehtoranta L. 2022. Role of D-mannose in urinary tract infections - a narrative review. Nutr J. 21(1):18. doi: 10.1186/s12937-022-00769-x.
- Beachey EH. 1981. Bacterial adherence: adhesin-receptor interactions mediating the attachment of bacteria to mucosal surface. J Infect Dis. 143(3):325–345. doi: 10.1093/infdis/143.3.325.
- Bekiares N, Krueger CG, Meudt JJ, Shanmuganayagam D, Reed JD. 2018. Effect of sweetened dried cranberry consumption on urinary proteome and fecal microbiome in healthy human subjects. OMICS. 22(2):145–153. doi: 10.1089/omi.2016.0167.
- Birmingham AD, Esquivel-Alvarado D, Maranan M, Krueger CG, Reed JD. 2021. Inter-laboratory validation of 4-(dimethylamino) cinnamaldehyde (DMAC) assay using cranberry proanthocyanidin standard for quantification of soluble proanthocyanidins in cranberry foods and dietary supplements, First action official method SM: 2019.06. J AOAC Int. 104(1):216–222. doi: 10.1093/jaoacint/qsaa084.
- Bosley S, Krueger CG, Birmingham A, Howell AB, Reed JD. 2024. Improved in vitro hemagglutination assays utilizing P-Type and Type 1 uropathogenic Escherichia coli to evaluate bacterial anti-adhesion activity of cranberry products. J Diet Suppl. 21(3):327–343. doi: 10.1080/19390211.2023.2276962.
- Chughtai B, Thomas D, Howell A. 2016. Variability of commercial cranberry dietary supplements for the prevention of uropathogenic bacterial adhesion. Am J Obstet Gynecol. 215(1):122–123. doi: 10.1016/j.ajog.2016.03.046.
- Cooper TE, Teng C, Howell M, Teixeira-Pinto A, Jaure A, Wong G. 2022. D-mannose for preventing and treating urinary tract infections. Cochrane Database Syst Rev. 8(8):CD013608. doi: 10.1002/14651858.CD013608.pub2.
- Cunningham DG, Vannozzi S, O’Shea E, Turk R. 2002. Analysis and standardization of cranberry products. In: Ho CT, Zheng QY, editors. Quality management of nutraceuticals. Washington (DC): American Chemical Society; p. 151–166.
- DE Leo V, Cappelli V, Massaro MG, Tosti C, Morgante G. 2017. Evaluation of the effects of a natural dietary supplement with cranberry, Noxamicina® and D-mannose in recurrent urinary infections in perimenopausal women. Minerva Ginecol. 69(4):336–341. doi: 10.23736/S0026-4784.17.04074-6.
- de Llano DG, Esteban-Fernández A, Sánchez-Patán F, Martínlvarez PJ, Moreno-Arribas MV, Bartolomé B. 2015. Anti-adhesive activity of cranberry phenolic compounds and their microbial-derived metabolites against uropathogenic Escherichia coli in bladder epithelial cell cultures. Int J Mol Sci. 16(6):12119–12130.
- Del Popolo G, Nelli F. 2018. Recurrent bacterial symptomatic cystitis: a pilot study on a new natural option for treatment. Arch Ital Urol Androl. 90(2):101–103. doi: 10.4081/aiua.2018.2.101.
- Déprez S, Brezillo C, Rabot S, Philippe C, Mila I, Lapierre C, et al. 2000. Polymeric proanthocyanidins are catabolized by human colonic microflora into low-molecular-weight phenolic acids. J. Nutr. 130:2733–2738.
- Di Martino P, Agniel R, David K, Templer C, Gaillard JL, Denys P, Botto H. 2006. Reduction of Escherichia coli adherence to uroepithelial bladder cells after consumption of cranberry juice: a double-blind randomized placebo-controlled cross-over trial. World J Urol. 24(1):21–27. doi: 10.1007/s00345-005-0045-z.
- Diaz MS, Mertens-Talcott SU, Talcott ST. 2024. Intestinal microbiome metabolism of cranberry (Vaccinium macrocarpon) proanthocyanidin dimers, but not trimers, is altered by dysbiosis in ulcerative colitis ex vivo. J Agric Food Chem. 72(8):4184–4194. doi: 10.1021/acs.jafc.4c00042.
- Esquivel-Alvarado D, Alfaro-Viquez E, Krueger CG, Vestling MM, Reed JD. 2021. Identification of A-type proanthocyanidins in cranberry-based foods and dietary supplements by matrix-assisted laser desorption/ionization time-of-flight mass spectrometry, First Action Method: 2019.05. J AOAC Int. 104(1):223–231. doi: 10.1093/jaoacint/qsaa106.
- Fan E, Dashti M, Fuentes J, Reitzer L, Christie AL, Zimmern PE. 2023. D-mannosuria levels measured 1 h after d-mannose intake can select out favorable responders: A pilot study. Neurourol Urodyn. 42(1):49–55. doi: 10.1002/nau.25059.
- Feldman M, Weiss EI, Ofek I, Steinberg D. 2009. Interference of cranberry constituents in cell-cell signaling system of Vibrio harveyi. Curr Microbiol. 59(4):469–474. doi: 10.1007/s00284-009-9462-3.
- Feliciano RP, Boeres A, Massacessi L, Istas G, Ventura MR, Nunes Dos Santos C, Heiss C, Rodriguez-Mateos A. 2016. Identification and quantification of novel cranberry-derived plasma and urinary (poly)phenols. Arch Biochem Biophys. 599:31–41. doi: 10.1016/j.abb.2016.01.014.
- Feliciano RP, Mills CE, Istas G, Heiss C, Rodriguez-Mateos A. 2017. Absorption, metabolism and excretion of cranberry (poly)phenols in humans: a dose response study and assessment of inter-individual variability. Nutrients. 9(3):268. doi: 10.3390/nu9030268.
- Foo LY, Lu Y, Howell AB, Vorsa N. 2000a. The structure of cranberry proanthocyanidins which inhibit adherence of uropathogenic P-fimbriated Escherichia coli in vitro. Phytochemistry. 54(2):173–181. doi: 10.1016/S0031-9422(99)00573-7.
- Foo LY, Lu Y, Howell AB, Vorsa N. 2000b. A-type proanthocyanidin trimers from cranberry that inhibit adherence of uropathogenic P-fimbriated Escherichia coli. J Nat Prod. 63(9):1225–1228. doi: 10.1021/np000128u.
- Gazi U, Martinez-Pomares L. 2009. Influence of the mannose receptor in host immune responses. Immunobiology. 214(7):554–561. doi: 10.1016/j.imbio.2008.11.004.
- Global antimicrobial resistance and use surveillance system (GLASS) report 2022. 2022. Geneva: World Health Organization.
- Gullickson ER, Krueger CG, Birmingham A, Maranan M, Reed JD. 2020. Development of a cranberry standard for quantification of insoluble cranberry (Vaccinium macrocarpon Ait.) proanthocyanidins. J Agric Food Chem. 68(10):2900–2905. doi: 10.1021/acs.jafc.9b03696.
- Gupta K, Chou MY, Howell A, Wobbe C, Grady R, Stapleton AE. 2007. Cranberry products inhibit adherence of uropathogenic Escherichia coli to primary cultured bladder and vaginal epithelial cells. J Urol. 177(6):2357–2360. doi: 10.1016/j.juro.2007.01.114.
- Hagberg L, Jodal U, Korhonen T, Lidin-Janson G, Lindberg U, Svanborg E. 1981. Adhesion, hemagglutination, and virulence of Escherichia coli causing urinary tract infections. Infect Immun. 31(2):564–570. doi: 10.1128/iai.31.2.564-570.1981.
- Hotchkiss AT, Jr., Nunez A, Strahan GD, Chau HK, White AK, Marais JP, et al. 2015. Cranberry xyloglucan structure and inhibition of Escherichia coli adhesion to epithelial cells. J Agric Food Chem. 63(23):5622–5633. doi: 10.1021/acs.jafc.5b00730.
- Howell A, Souza D, Roller M, Fromentin E. 2015. Comparison of the anti-adhesion activity of three different cranberry extracts on uropathogenic P-fimbriated Escherichia coli: a randomized, double-blind, placebo controlled, ex vivo, acute study. Nat Prod Commun. 10(7):1215–1218.
- Howell AB, Botto H, Combescure C, Blanc-Potard A-B, Gausa L, Matsumoto T, Tenke P, Sotto A, Lavigne J-P. 2010. Dosage effect on uropathogenic Escherichia coli anti-adhesion activity in urine following consumption of cranberry powder standardized for proanthocyanidin content: a multicentric randomized double blind study. BMC Infect Dis. 10(1):94. doi: 10.1186/1471-2334-10-94.
- Howell AB, Dreyfus JF, Chughtai B. 2022. Differences in urinary bacterial anti-adhesion activity after intake of cranberry dietary supplements with soluble versus insoluble proanthocyanidins. J Diet Suppl. 19(5):621–639. doi: 10.1080/19390211.2021.1908480.
- Howell AB, Reed JD, Krueger CG, Winterbottom R, Cunningham DG, Leahy M. 2005. A-type cranberry proanthocyanidins and uropathogenic bacterial anti-adhesion activity. Phytochemistry. 66(18):2281–2291. doi: 10.1016/j.phytochem.2005.05.022.
- Howell AB, Vorsa N, Der Marderosian A, Foo LY. 1998. Inhibition of the adherence of P fimbriated Escherichia coli to uroepithelial-cell surfaces by proanthocyanidin extracts from cranberries. N Engl J Med. 339(15):1085–1086. doi: 10.1056/NEJM199810083391516.
- Hu X, Shi Y, Zhang P, Miao M, Zhang T, Jiang B. 2016. D-Mannose: properties, production, and applications: an overview. Compr Rev Food Sci Food Saf. 15(4):773–785.
- Jepson RG, Williams G, Craig JC. 2012. Cranberries for preventing urinary tract infections. Cochrane Database Syst Rev. 10(10):CD001321.
- Johnson JR, Brown JJ, Ahmed P. 1998. Diversity of hemagglutination phenotypes among P-fimbriated wild-type strains of Escherichia coli in relation to papG allele repertoire. Clin Diagn Lab Immunol. 5(2):160–170. doi: 10.1128/CDLI.5.2.160-170.1998.
- Johnson-White B, Buquo L, Zeinali M, Ligler FS. 2006. Prevention of nonspecific bacterial cell adhesion in immunoassays by use of cranberry juice. Anal Chem. 78(3):853–857. doi: 10.1021/ac051700v.
- Kaspar KL, Howell AB, Khoo C. 2015. A randomized, double-blind, placebo-controlled trial to assess the bacterial anti-adhesion effects of cranberry extract beverages. Food Funct. 6(4):1212–1217. doi: 10.1039/C4FO01018C.
- Klinth JE, Pinkner JS, Hultgren SJ, Almqvist F, Uhlin BE, Axner O. 2012. Impairment of the biomechanical compliance of P pili: a novel means of inhibiting uropathogenic bacterial infections? Eur Biophys J. 41(3):285–295. doi: 10.1007/s00249-011-0784-2.
- Kranjčec B, Papeš D, Altarac S. 2014. D-Mannose powder for prophylaxis of recurrent urinary tract infections in women: a randomized clinical trial. World J Urol. 32(1):79–84. doi: 10.1007/s00345-013-1091-6.
- Krueger CG, Reed JD, Feliciano RP, Howell AB. 2013. Quantifying and characterizing proanthocyanidins in cranberries in relation to urinary tract health. Anal Bioanal Chem. 405(13):4385–4395. doi: 10.1007/s00216-013-6750-3.
- Kyriakides R, Jones P, Somani BK. 2021. Role of D-mannose in the prevention of recurrent urinary tract infections: evidence from a systematic review of the literature. Eur Urol Focus. 7(5):1166–1169. doi: 10.1016/j.euf.2020.09.004.
- LaFavers KA, Micanovic R, Sabo AR, Maghak LA, El-Achkar TM. 2022. Evolving concepts in uromodulin biology, physiology, and its role in disease: a tale of two forms. Hypertension. 79(11):2409–2418. doi: 10.1161/hypertensionaha.122.18567.
- Lavigne JP, Bourg G, Combescure C, Botto H, Sotto A. 2008. In-vitro and in-vivo evidence of dose-dependent decrease of uropathogenic Escherichia coli virulence after consumption of commercial Vaccinium macrocarpon (cranberry) capsules. Clin Microbiol Infect. 14(4):350–355.
- Lee SJ, Evers S, Roeder D, Parlow AF, Risteli J, Risteli L, Lee YC, Feizi T, Langen H, Nussenzweig MC, et al. 2002. Mannose receptor-mediated regulation of serum glycoprotein homeostasis. Science. 295(5561):1898–1901. doi: 10.1126/science.1069540.
- Lessard-Lord J, Roussel C, Guay V, Desjardins Y. 2024. Assessing the gut microbiota’s ability to metabolize oligomeric and polymeric flavan-3-ols from aronia and cranberry. Mol Nutr Food Res. 68(5):e2300641. doi: 10.1002/mnfr.202300641.
- Liu H, Howell AB, Zhang DJ, Khoo C. 2019. A randomized, double-blind, placebo-controlled pilot study to assess bacterial anti-adhesive activity in human urine following consumption of a cranberry supplement. Food Funct. 10(12):7645–7652. doi: 10.1039/C9FO01198F.
- Mannino G, Di Stefano V, Lauria A, Pitonzo R, Gentile C. 2020. Vaccinium macrocarpon (cranberry)-based dietary supplements: variation in mass uniformity, proanthocyanidin dosage and anthocyanin profile demonstrates quality control standard needed. Nutrients. 12(4):992. doi: 10.3390/nu12040992.
- Melican K, Sandoval RM, Kader A, Josefsson L, Tanner GA, Molitoris BA, Richter-Dahlfors A. 2011. Uropathogenic Escherichia coli P and Type 1 fimbriae act in synergy in a living host to facilitate renal colonization leading to nephron obstruction. PLoS Pathog. 7(2):e1001298. doi: 10.1371/journal.ppat.1001298.
- Mena P, Calani L, Bruni R, Del Rio D. 2015. Chapter 6 - Bioactivation of high-molecular-weight polyphenols by the gut microbiome. In: Kieran T, Del Rio D, editors. Diet-microbe interactions in the gut. Academic Press, London; p. 73–101. ISBN 9780124078253, doi: 10.1016/B978-0-12-407825-3.
- Monagas M, Urpi-Sarda M, Sánchez-Patán F, Llorach R, Garrido I, Gómez-Cordovés C, Andres-Lacueva C, Bartolomé B. 2010. Insights into the metabolism and microbial biotransformation of dietary flavan-3-ols and the bioactivity of their metabolites. Food Funct. 1(3):233–253. doi: 10.1039/c0fo00132e.
- Monagas M. 2023. Cranberry fruit juice dry extract, USP Monograph. Pharmacopeial Forum. 48(3):1–4. doi: 10.31003/USPNF_M8506_02_01.
- Mulvey MA. 2002. Adhesion and entry of uropathogenic Escherichia coli. Cell Microbiol. 4(5):257–271. doi: 10.1046/j.1462-5822.2002.00193.x.
- Mydock-McGrane L, Cusumano Z, Han Z, Binkley J, Kostakioti M, Hannan T, et al. 2016. Antivirulence c-mannosides as antibiotic-sparing, oral therapeutics for urinary tract infections. J Med Chem. 59(20):9390–9408.
- Nemzer BV, Al-Taher F, Yashin A, Revelsky I, Yashin Y. 2022. Cranberry: chemical composition, antioxidant activity and impact on human health: overview. Molecules. 27(5):1503. doi: 10.3390/molecules27051503.
- Ofek I, Hasty DL, Sharon N. 2003. Anti-adhesion therapy of bacterial diseases: prospects and problems. FEMS Immunol Med Microbiol. 38(3):181–191. doi: 10.1016/S0928-8244(03)00228-1.
- Olden M, Corre T, Hayward C, Toniolo D, Ulivi S, Gasparini P, Pistis G, Hwang S-J, Bergmann S, Campbell H, et al. 2014. Common variants in UMOD associate with urinary uromodulin levels: a meta-analysis. J Am Soc Nephrol. 25(8):1869–1882. doi: 10.1681/ASN.2013070781.
- Pitkänen E. 1996. Mannose, mannitol, fructose and 1,5-anhydroglucitol concentrations measured by gas chromatography/mass spectrometry in blood plasma of diabetic patients. Clin Chim Acta. 251(1):91–103. doi: 10.1016/0009-8981(96)06284-5.
- Pruijm M, Ponte B, Ackermann D, Paccaud F, Guessous I, Ehret G, Pechère-Bertschi A, Vogt B, Mohaupt MG, Martin P-Y, et al. 2016. Associations of urinary uromodulin with clinical characteristics and markers of tubular function in the general population. Clin J Am Soc Nephrol. 11(1):70–80. doi: 10.2215/CJN.04230415.
- Rafsanjany N, Senker J, Brandt S, Dobrindt U, Hensel A. 2015. In vivo consumption of cranberry exerts ex vivo antiadhesive activity against fimh-dominated uropathogenic Escherichia coli: a combined in vivo, ex vivo, and in vitro study of an extract from Vaccinium macrocarpon. J Agric Food Chem. 63(40):8804–8818. doi: 10.1021/acs.jafc.5b03030.
- Reinhart H, Obedeanu N, Hooton T, Stamm W, Sobel J. 1990. Urinary excretion of Tamm-Horsfall protein in women with recurrent urinary tract infections. J Urol. 144(5):1185–1187. doi: 10.1016/s00225347(17)39687-8.
- Roopchand DE, Krueger CG, Moskal K, Fridlender B, Lila MA, Raskin I. 2013. Food-compatible method for the efficient extraction and stabilization of cranberry pomace polyphenols. Food Chem. 141(4):3664–3669. doi: 10.1016/j.foodchem.2013.06.050.
- Russo E, Montt Guevara M, Giannini A, Mannella P, Palla G, Caretto M, Pancetti F, Genazzani AD, Simoncini T. 2020. Cranberry, D-mannose and anti-inflammatory agents prevent lower urinary tract symptoms in women undergoing prolapse surgery. Climacteric. 23(2):201–205. doi: 10.1080/13697137.2019.1679110.
- Säemann MD, Weichhart T, Hörl WH, Zlabinger GJ. 2005. Tamm-Horsfall protein: a multilayered defense molecule against urinary tract infection. Eur J Clin Invest. 35(4):227–235. doi: 10.1111/j.13652362.2005.01483.x.
- Scaglione F, Minghetti P, Ambrosio F, Ernst B, Ficarra V, Gobbi M, Naber K, Schellekens H. 2023. Nature of the interaction of alpha-d-mannose and Escherichia coli bacteria, and implications for its regulatory classification. a Delphi Panel European consensus based on chemistry and legal evidence. Ther Innov Regul Sci. 57(6):1153–1166. doi: 10.1007/s43441-023-00548-8.
- Scharenberg M, Schwardt O, Rabbani S, Ernst B. 2012. Target selectivity of fimh antagonists. J Med Chem. 55(22):9810–9816. doi: 10.1021/jm3010338.
- Scharf B, Schmidt TJ, Rabbani S, Stork C, Dobrindt U, Sendker J, Ernst B, Hensel A. 2020. Antiadhesive natural products against uropathogenic E. coli: what can we learn from cranberry extract? J Ethnopharmacol. 257:112889. doi: 10.1016/j.jep.2020.112889.
- Scharf B, Sendker J, Dobrindt U, Hensel A. 2019. Influence of cranberry extract on Tamm-Horsfall protein in human urine and its antiadhesive activity against uropathogenic Escherichia coli. Planta Med. 85(2):126–138. doi: 10.1055/a-0755-7801.
- Sintara M, Li L, Cunningham DG, Prior RL, Wu X, Chang T. 2018. Single-laboratory validation for determination of total soluble proanthocyanidins in cranberry using 4 dimethylaminocinnamaldehyde. J AOAC Int. 101(3):805–809. doi: 10.5740/jaoacint.17-0288.
- Sintara M, Wang Y, Li L, Liu H, Cunningham DG, Prior RR, Chen P, Chang T, Wu X. 2020. Quantification of cranberry proanthocyanidins by normal-phase high-performance liquid chromatography using relative response factors. Phytochem Anal. 31(6):874–883. doi: 10.1002/pca.2952.
- Sobota AE. 1984. Inhibition of bacterial adherence by cranberry juice: potential use for the treatment of urinary tract infections. J Urol. 131(5):1013–1016. doi: 10.1016/S0022-5347(17)50751-X.
- Spaulding CN, Klein RD, Ruer S, Kau AL, Schreiber HL, Cusumano ZT, Dodson KW, Pinkner JS, Fremont DH, Janetka JW, et al. 2017. Selective depletion of uropathogenic E. coli from the gut by a FimH antagonist. Nature. 546(7659):528–532. doi: 10.1038/nature22972.
- Ulrey RK, Barksdale SM, Zhou W, van Hoek ML. 2014. Cranberry proanthocyanidins have anti-biofilm properties against Pseudomonas aeruginosa. BMC Complement Altern Med. 14(1):499. doi: 10.1186/1472-6882-14-499.
- Urena-Saborio H, Udayan APM, Alfaro-Viquez E, Madrigal-Carballo S, Reed JD, Gunasekaran S. 2021. Cranberry proanthocyanidins-pani nanocomposite for the detection of bacteria associated with urinary tract infections. Biosensors (Basel). 11(6):199. doi: 10.3390/bios11060199.
- Valentova K, Stejskal D, Bednar P, Vostalova J, Cíhalík C, Vecerova R, Koukalova D, Kolar M, Reichenbach R, Sknouril L, et al. 2007. Biosafety, antioxidant status, and metabolites in urine after consumption of dried cranberry juice in healthy women: a pilot double-blind placebo controlled trial. J Agric Food Chem. 55(8):3217–3224. doi: 10.1021/jf0636014.
- Vicariotto F. 2014. Effectiveness of an association of a cranberry dry extract, D-mannose, and the two microorganisms Lactobacillus plantarum LP01 and Lactobacillus paracasei LPC09 in women affected by cystitis: a pilot study. J Clin Gastroenterol. 48 Suppl 1:S96–S101.
- Waterhouse AL, Ignelzi S, Shirley JR. 2000. A comparison of methods for quantifying oligomeric proanthocyanidins from grape seed extracts. Am J Enol Vitic. 51(4):383–389. doi: 10.5344/ajev.2000.51.4.383.
- Weichhart T, Zlabinger GJ, Säemann MD. 2005. The multiple functions of Tamm-Horsfall protein in human health and disease: a mystery clears up. Wien Klin Wochenschr. 117(9-10):316–322. doi: 10.1007/s00508-005-0353-8.
- Williams G, Hahn D, Stephens JH, Craig JC, Hodson EM. 2023. Cranberries for preventing urinary tract infections. Cochrane Database Syst Rev. 4(4):CD001321.
- Yamabhai M, Sak-Ubol S, Srila W, Haltrich D. 2016. Mannan biotechnology: from biofuels to health. Crit Rev Biotechnol. 36(1):32–42.
- Zafriri D, Ofek I, Adar R, Pocino M, Sharon N. 1989. Inhibitory activity of cranberry juice on adherence of type 1 and type P fimbriated Escherichia coli to eucaryotic cells. Antimicrob Agents Chemother. 33(1):92–98. doi: 10.1128/AAC.33.1.92.