ABSTRACT
One of the necessary ways to achieve the efficient, clean utilization of coal resources is to reduce the amount of coal gangue in raw coal to ensure the development of clean, low-carbon, and green energy in China. The application of conventional coal gangue separation equipment is severely limited due to the large investment in early construction, high production costs, and large water consumption and waste in production. Intelligent coal gangue separation equipment has the advantages of fast speed, high precision, strong modularity, and integration scalability. It also has the advantages of low operation cost and energy consumption, convenient operation and maintenance, and no water use. All of that could effectively improve the utilization rate of coal resources and expand the economic benefits of enterprises. The application of high-speed, high-precision, and anti-interference online element rapid analysis technology in intelligent coal gangue separation equipment will become an inevitable trend of equipment development in the future with the continuous improvement of coal product demand. Online rapid element analysis technology includes laser-induced breakdown spectroscopy, dual-energy X-ray, and transient neutron activation analysis. Improving and applying more high-speed, high-precision algorithms such as partial least squares and Monte Carlo library least squares in the future are important.
Introduction
Coal, a solid, flammable mineral buried underground by ancient plants that underwent complex biochemical and physical chemical changes to gradually form, has been one of the major energy sources used by the human world since the eighteenth century and is a major source of energy (Lv et al. Citation2022; Rocha, Siqueira, and Silva Citation2021). Nevertheless, coal gangue is a dark gray rock, which is less carbonaceous and harder than coal. It has numerous properties, such as low heat production and an excess of deleterious substances (Ashfaq et al. Citation2020; Ding and Laskowski Citation2006). Numerous studies have found that the main components of coal gangue are Al2O3 and SiO2, and they contain various amounts of Fe2O3, Cao, MgO, Na2O, K2O, P2O5, SO3, and trace rare elements (gallium, vanadium, titanium, cobalt) (Li and Wang Citation2019). Given that the carbon resources of coal are continuously and profoundly mined, large quantities of coal gangue are also inevitably extracted, which in turn gravely affects the quality of the product and increases the cost of production (Zhang et al. Citation2017). According to the China Coal Processing and Utilization Association, for every 100 million tons of raw coal enrolled, about 18 million tons of coal gangue can be excluded. Approximately 13 million tons of ash and 3.5 × 105 tons of sulfur could be excluded, and 4.9 × 104 tons of SO2 emissions could be reduced. The efficiency of coal burning could be increased from 10% to 15% by using washed and processed fine coal (Ruan Citation2022; Yan Citation2022). Therefore, reducing the amount of coal gangue in raw coal is one of the necessary ways to achieve efficient clean utilization of coal resources and ensure the development of clean, low-carbon, and green energy in our country.
In coal mining, the automatic removal of coal gangue is an important link in modern coal production and processing. The majority of coal mining and production in China has been screened, and large gangue sizes have been screened manually (Zou et al. Citation2020). Manual gangue selection is a backward method with excessive labor intensity and low production efficiency. The gangue sorting rate fluctuates greatly depending on personnel quality, management level, and other factors (Xie et al. Citation2022). Moreover, minute amounts of coal gangue cannot be separated effectively, leading to waste of coal resources and high gangue content in coal. In recent years, all major coal enterprises have accelerated the construction of coal preparation plants, and the production rate of raw coal has reached 70% by 2017 (Sun et al. Citation2021). To promote the development of coal washing, China’s 13th Five-Year Energy Development Plan has set out detailed requirements for various fields related to energy development. Actively promoting the application of clean coal technology and vigorously developing coal washing and processing in the coal mining sector are essential to achieve a raw coal selection rate of more than 75% by 2020 (Du Citation2017). According to the China Coal Industry Policy, newly built large- and medium-sized coal mines should be equipped with coal preparation plants of corresponding size to encourage the construction of cluster coal preparation plants in the concentrated mining areas of small- and medium-sized coal mines (Zhou Citation2022).
The rate of mining has been greatly accelerated due to the application of large-scale mechanized coal mining techniques. Thin bands of dirt, faults, and collapsed columns are mined together with the coal seam, and mixed into the raw coal of the mine. An increase in the proportion of gangue brought into the raw coal preparation system has resulted in a great increase in the yield of lump gangue (Li, Wang, and An Citation2015). Collecting the waste manually is not suitable, so discharging the waste using machinery is imperative. shown the beneficiation process of mechanical gangue discharge.
In , the mined raw coal is transported by tram to the screening workshop, where the screened product is then placed in a fine ore bin. The product on the screen is crushed by a jaw crusher to a qualified particle size and then deposited in a buffer ore bin. The raw coal is then conveyed to the ore feeder through the bucket feeder and through the conveyor belt. The ore information acquisition system scans the characteristics of the coal mine (such as color, magnetism, and conductivity). The information processing system also feeds the processed signals to the computer, which sends the separation instructions to the separation execution system. After processing by the sorting execution system, the gangue is placed in the designated bin for sorting.
Traditional Coal Gangue Separation Process and Equipment
Water washing and dry coal preparation are the main parts of coal preparation. Spiral separation, jigging, heavy medium separation, and other processes are water washing processes, which have the advantages of mature technology, high separation accuracy, and large processing capacity (Majumder, Barnwal, and Ramakrishnan Citation2004). Dry coal preparation has existed for the past 20 years and is currently developing slowly (Yu et al. Citation2019). Dry coal preparation has existed for the past 20 years and is currently developing slowly (Zheng et al. Citation2016). Conventional coal gangue separation devices include spiral separation equipment, a heavy medium shallow trough separator, and a TBS interference bed separator (Dong Citation2017). Several separators, which essentially enable the preliminary separation of coal gangue, have been applied in all the major coal mines.
Spiral Separation Equipment for Coal Gangue and Its Application
Spiral separation devices are governed by the flow membrane separation principles. A separation equipment that achieves the separation of minerals with different densities under the action of gravity and centrifugal force depending on the characteristics of liquid flow (Meng et al. Citation2021; Yu et al. Citation2022). Deputy the early 1990s, the spiral separator has been used in the coal preparation industry to separate 3.0–0.15 mm coarse slime (Richards et al. Citation2000). Spiral separators have been widely used in the United States and Australia as well as in other coal-producing countries. shows the working principle of the spiral separator. A slurry of thickened coarse slime from the top of the helical separator is fed into the helical groove. As the coals spiral downward, the coals in the spiral groove are primarily affected by gravity, centrifugal force, water flow driving force, and friction force. It is in the upper layers of the current and moves toward the thickest water layer at the outer edge of the spiral. The high-density material is in the lower water layer of the flow and then moves to the thickest part of the water layer at the inner edge of the spiral to achieve separation finally (Khanchi et al. Citation2014).
Spiral separators have the advantage of structural simplicity, a general classification particle size of 1.50–0.15 mm, and are widely used in the Shanxi regions of Shandong, Jincheng, Datong, and Shuozhou (Huang Citation2014; Zhao and Kuang Citation2014). Laixin Clean Coal Company uses a spiral sorting machine to sort +0.8 mm fine coal ore, which can effectively improve the sorting accuracy of heavy medium coal selection and reduce production costs (Wang and Wei Citation2006). A coal preparation plant in Shanxi used a two-stage spiral separator to separate the flotation tailings of No. 1 raw coal (difficult to float coal), achieving effective separation of the flotation tailings of No. 1 raw coal. This process can increase the concentrate yield of fine coal slime to 60.51%, with a quantity efficiency of 92.7%, and a lower separation limit of 0.075 mm (Zhu Citation2023). However, this approach has the disadvantage that the separation density is commonly required to be larger than 1.70 kg/L, which is appropriate for desulfurization. It is not applicable to coking coal preparation plants with separation densities below 1.70 kg/L. The tailing after helical classification is usually 40%–60%, and product quality is not high (Gao and Fan Citation2010).
Heavy Medium Shallow Trough Separator and Its Application
To select coal for the heavy medium shallow trough separator, the coal to be selected passes from the feed end into a tank filled with a dense medium suspension. A portion of the heavy-duty medium suspension enters the tank through the ascending feed pipe. A second portion of the heavy medium suspension enters the tank from an ascending funnel at the bottom of the tank, creating a horizontal and an upward flow (Dong Citation2017; Du Citation2021; Miao and Zhang Citation2014). shows the working principle of the heavy medium shallow trough separator.
During the influence of the upward flow, the coal to be selected is evenly distributed in the tank, and particles with a density less than that of the dense medium suspension float up and are expelled from the discharge end by the horizontal flow. The densities of particles greater than the suspension density of the heavy medium sink to the bottom of the tank and are sent out of the tank by a scraper conveyor (Wei Citation2017; Zhao Citation2016). The Anju Coal Mine Coal Preparation Plant adopts the QG series shallow groove scraper heavy medium sorting machine to replace the original moving screen jig process. Effectively reducing the lower particle size limit of the lump coal discharge system and increasing the washing amount of lump coal discharge. While improving the accuracy of lump coal sorting, it also alleviates the production pressure in the final coal workshop (Qi, Guo, and Lian Citation2018). Depending on the working principle of a heavy medium shallow trough separator, the flow ratio between the upflow and the downflow has a direct effect on its separation effect. When the low flow rate is too high, the position of the gangue in the heavy medium suspension is affected, causing the gangue to mix easily with the clean coal (Yang Citation2018). Consequently, the gangue rate of the light product increases. The heavy medium suspension is directly unstable due to the low flow rate. The actual separation density of the material is reduced, and light products easily enter the gangue. The final result is that the coal carrying rate of the gangue rises. When the horizontal flow rate is too high, the separation time of the raw coal in the tank is shortened, resulting in a decrease in the separation accuracy (Li Citation2019). Given that the horizontal flow is small, the separation time of the raw coal to be sorted in the tank is prolonged, which directly affects the processing capacity of the equipment.
TBS Interference Bed Separator and Its Application
TBS interference bed separator is a kind of interference sedimentation separation equipment that uses the rising water flow to generate turbulence in the tank (Zhang Citation2020). shows the working principle of the TBS interference bed separator.
Figure 4. Working principle of the TBS interference bed separator (Zi Citation2015).
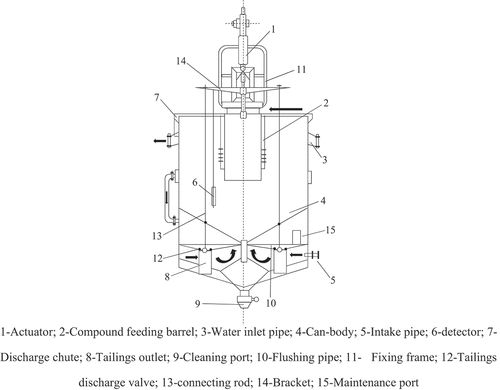
In a tangential direction, the rocks enter the feed well through the feed pipe and form an interference bed in the tank. As a result of the rising water flow, the particles with low density move upward and overflow into the overflow tank. Particles with high density move downward and pass through the bed before concentrating at the bottom of the tank. The density meters are in the middle and lower parts of the bed (Chen Citation2015). When bed density is detected to increase, the underflow discharge valve is opened to discharge the coarse gangue intermittently. When a decrease in the bed density is detected, the underflow discharge valve is gradually closed. TBS interference bed separator is widely used in coking coal preparation plants such as Shanghaimiao in Inner Mongolia, Lu’an in Shanxi, Hejin in Shanxi, and Xingtai in Hebei (Zi Citation2015). The Qixing Coal Preparation Plant of China Pingmei Shenma Group has optimized the TBS interference bed sorting machine to make its operation more stable and significantly improve the sorting efficiency of coal mines (Chen Citation2016). The application effect of TBS interference bed sorting machine in the coarse and clean coal recovery system of Huangling No.1 Coal Mine Preparation Plant is significant. It can handle a large amount of coarse coal slime and reduce the content of coarse and fine coal ash, ensuring the quality of clean coal, and avoiding the adverse phenomenon of heavy medium clean coal and flotation clean coal being the back ash of coarse and clean coal. It can effectively improve the yield of clean coal products and improve the utilization rate of coal resources (Hou and He Citation2015). For long-term use, the separation density of the TBS interference bed separator is higher, generally less than 1.70 kg/L. The ash content of tailings after separation is generally 40%–60%, and the loss of clean coal is relatively serious. This approach is not conducive to the effective recovery and utilization of coal resources.
Development and Application of Intelligent Coal Gangue Separation Equipment
In the past two decades, dry separation has been a coal preparation method that has existed and developed slowly with advantages of no water, simple process, low investment, and low production cost (Guo et al. Citation2021; Li et al. Citation2022). With increasing attention to dry coal separation, intelligent dry separation technology has emerged. It is an intelligent automatic separation technology based on ray, spectral analysis, or image recognition of coal gangue (Li et al. Citation2021). An intelligent dry separation technology has the advantages of no water, a simple process, low investment, and low production costs. Since 2005, this technology has been gradually applied to the coal industry, such as Shenhuo Liangbei Coal Mine and Beijing Muchengjian Coal Mine (He et al. Citation2015; Yu et al. Citation2016). Zhang et al. studied γ applicability of X-ray separation in underground coal gangue separation. An evaluation of the selection process for the underground separation method of coal gangue is given, and requirements for the underground separation system and roadway layout of coal gangue are determined. They discussed the development direction of coal mine full grain classification, and proposes four typical filling mining methods: full mining full filling, full mining partial filling, partial mining partial filling and partial mining full filling. To provide technical reference for the realization of coal mining, underground separation and gangue treatment intensification, and further enrich the technical system of green mining in China’s coal mines (Zhang et al. Citation2020).
X-rays can be used to identify and distinguish between the two because coal and gangue differ substantially in properties such as material composition and density (Li et al. Citation2021). X-ray transmission sorting machine and X-ray fluorescence sorting machine are two different X-ray analysis techniques (Luo et al. Citation2022). The X-ray transmission sorting machine analyzes the composition of substances by measuring their absorption and transmission of X-rays (Robben et al. Citation2020). It detects the energy spectrum of X-rays transmitted from the sample by placing the sample behind the X-ray beam, and determines the presence and content of different elements in the sample based on the peak and intensity information in the energy spectrum. This method is suitable for the analysis of solid samples and can obtain chemical information of the samples in a nondestructive manner. The X-ray fluorescence sorting machine uses the fluorescence radiation generated by the excitation of X-rays to analyze the composition of substances (Li, Ye, and Xiong Citation2020). It irradiates an X-ray beam onto the sample, and the atoms in the sample are excited to emit specific fluorescent X-rays. The composition of the sample is determined by measuring the energy spectrum of the fluorescent X-rays through a detector. This method is suitable for samples of different forms such as solids, liquids, and gases, but requires the samples to be analyzed in a vacuum or low-pressure environment. shows the working principle of the intelligent X-ray gangue sorter. shows the recognition method based on X-ray image of coal gangue separation.
Figure 5. Intelligent X-ray gangue sorter working principles (Li et al. Citation2021).
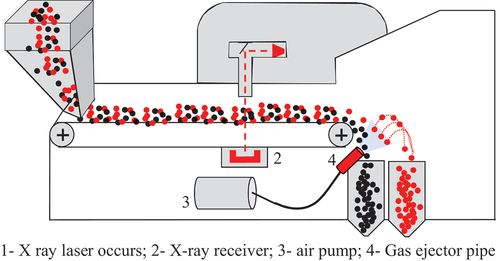
The X-ray identification of gangue does not require separation but is necessary to avoid stacking of material that causes identification errors and reduces classification accuracy. Most of the existing X-ray gangue sorting devices only use a single vibration method for distribution, and the feeding device is also mostly continuous unloading. Large pieces of material are possible when the vibration intensity is inadequate. When continuous unloading is used, effectively distributing the material and effectively dispersing the material is difficult, resulting in a stacking of materials. Thus, the identification accuracy is reduced, and excessive vibration intensity may cause splash problems (Wang et al. Citation2021). Furthermore, when applied to coal gangue sorting by existing electro-optical sorting machines, the coal gangue mixed in the coal pile is not easily irradiated by special light sources illuminated by light-emitting radiation. The gangue is prone to ejection in all directions under the action of high-pressure air currents and is prone to damage by impact during the sorting of the light-emitting tubes. Dust adhering to the surface of a light-emitting tube makes the light irradiated by the tube insufficient, and identifying gangue and useful minerals is difficult (Han and Deng Citation2021).
TCS intelligent coarse slime sorter can realize high density separation of coarse slime (particle size grade: 3–0.15 mm; separation density: 1.40–1.80 kg/L automatic adjustment). After separation, the concentrate product is qualified, and the ash content of tailings reaches 60%–75%, which can be directly discharged with gangue or comprehensively utilized. In production, the speed of upwelling water can be adjusted by adjusting the upwelling water flow. If the upwelling water flow is increased within a reasonable range, the separation density of coarse coal slime increases. If the upwelling water flow is reduced, the separation density of coarse coal slime decreases. Changing the separation density at the same time δ value can increase or decrease the density of the separation area, thus increasing or decreasing the buoyancy of particles, and increasing or decreasing the separation density of coarse coal slime. The ash content of concentrate products and the ash value of tailings products can be controlled by the above adjustment methods. For example, a coal preparation plant has an annual processing capacity of 5 million tons of raw coal, its crude slime content is about 15%, and its annual output of crude slime is about 7.5 × 105 tons. If the spiral separator or TBS separator is used for separation, about 3 × 105 tons of tailings can be produced and discharged into the gangue. Its ash content is about 40%–60%, and the calorific value of the tailings is generally 1500–2500 kcal/kg. If the TCS intelligent coarse slime separator of the invention is adopted, the ash content of tailings can be increased to more than 60%, the annual output of tailings can be reduced to about 1.5 × 105 tons, and the calorific value can be reduced to less than 1000 kcal/kg. This part of tailings can be directly discarded. If the TCS intelligent sorter of the invention is adopted, its one-time investment increases by about 3 million yuan compared with that of the spiral sorter or TBS sorter, but about 1.5 × 105 tons of clean coal products can be recovered in one year. According to the estimated price of clean coal of 150 yuan/ton, the sales income increases by 22.5 million yuan in one year, the increased investment is recovered in two months, and the waste of gangue is reduced (Zhai Citation2022).
Compared with the traditional coal preparation method, photoelectric sorting technology is applied in the field of gangue sorting, which has the advantages of fast speed, high precision, modularity and integration scalability, low operating cost and energy consumption, easy operation and maintenance, and no water use, and has developed rapidly in the coal preparation industry in recent years. The domestic photoelectric sorting industry began in the 90s of the twentieth century. Based on the introduction and digestion of foreign technology, it rapidly entered a period of rapid development, from the beginning of the grain, beans, seeds, and food sorting and processing, gradually expanded to other fields, and began to be used in the field of gangue sorting around 2014 (Fu, Lu, and Zhang Citation2020). In 2014, six coal mines in Pingzhuang of Guodian Group tried the GDRT intelligent dry sorting system. In 2015, Tongmei Group carried out the KRS intelligent coal dry sorting system test on the well, the onsite discharge efficiency reached more than 85%, the equipment was simple, and the economic benefits were evident. In 2016, Hancheng Mining Group conducted the BIRTLEY dual-energy X-ray gangue sorting test on the well, with a sorting accuracy of about 85%, and the test situation was generally good (Gao et al. Citation2021). At present, the TCS intelligent dry separation equipment and KRS intelligent dry separation equipment developed based on X-ray technology have been successfully applied in coal preparation plants and achieved good results.
Prospect of Intelligent Coal Gangue Separation Equipment
The application of coal gangue intelligent separation equipment can better realize the clean, low-carbon, and green development of the coal industry. The research and development of intelligent separation equipment for coal gangue with faster processing capacity and more accurate separation accuracy will promote the reform in the field of coal mining. Some application problems exist in traditional coal gangue separation equipment, such as difficulty in identifying black coal gangue similar to coal. The linear array detector used by the sorting equipment has many pixels and a large amount of data processing, so it needs to be equipped with an industrial computer for special processing. In addition, the commonly used coal gangue intelligent sorting equipment cannot solve the effect of coal mine material particle size and is not suitable for the specific use environment of coal mine (Yan et al. Citation2022). Although X-ray separation equipment has been used to separate target minerals and gangue minerals by analyzing the type and content of elements contained in ores, X-ray sorting is mostly an offline analysis method with control lag, and its operation speed is greatly affected by the environment. If the X-ray protection device of the equipment leaks, it will cause injury to the human body (Liu et al. Citation2012). The online element rapid analysis technology has the advantages of fast, strong online processing ability, high recognition accuracy, and strong adaptability. Therefore, the use of online element rapid analysis technology, which is more effective than X-ray separation, will become the inevitable trend of the development of intelligent separation equipment for coal gangue in the future. At present, the online element rapid analysis system that can be used in industry mainly includes laser induced breakdown spectroscopy (LIBS), dual-energy X-ray recognition technology, and instantaneous emission spectroscopy γ neutron activation analysis (PGNAA).
LIBS focuses the pulse laser on the sample surface, and high-temperature plasma is generated on the sample surface. The plasma emits element spectral lines during cooling. The emission spectral lines correspond to the elements in the measured sample one by one. The elements and their concentrations in the sample to be measured are obtained according to the wavelength and intensity of the emission spectral lines. LIBS can improve the detection accuracy and is suitable for measuring gas, liquid, and solid substances. To date, the detection limit of LIBS for most elements has reached the order of PPM, and for some elements, it has even reached the order of PPB (Fuentes et al. Citation2021; Kasim et al. Citation2022). Hudson et al. used LIBS technology to realize rapid recognition of metal composition. This technology can also strictly control the cleanliness of metal surface, so that the metal can meet the requirements of key applications in automobile, aerospace, and national defense industries (Hudson et al. Citation2017). Aberkane et al. successfully analyzed the depth profile of alumina-coated silicon substrate using LIBS technology, defined coating thickness and chemical composition, and discussed the influence of film structure and surface roughness (Aberkane et al. Citation2018). In recent years, LIBS technology has been widely used in various industrial production fields, such as environmental monitoring, metallurgy, coal quality detection, biomedicine, archeology, art, and other fields, due to its advantages of real-time and rapidity, no pretreatment of samples, adaptability to harsh measurement conditions, and the ability to achieve noncontact online measurement of multi-elements.
X-ray transmission system is classified into single energy and double energy according to the size of the energy of the ray source. The single-energy system is resolved from a macro perspective. Based on few images generated by it, it is recognized according to different shapes of objects (Hyunwoo et al. Citation2022). By contrast, the dual-energy system can distinguish objects from a microscopic perspective. When scanning an object, it can arrange the atomic number of the object and other relevant information, and distinguish the object according to its atomic composition (He et al. Citation2022). Like the single-energy system, the dual-energy system is divided into true dual energy and pseudo dual energy according to the ray source. For the true dual-energy system, the X-ray source used can form two kinds of energy with different sizes detected by the detector. The pseudo dual-energy system uses a single ray source and two groups of detectors to obtain the dual-energy value. shows the recognition method based on dual-energy X-ray image of coal gangue separation.
After the X-ray beam transmits the object, the low-energy part of the attenuated ray is first detected and imaged by the low-energy detector, then the ray passes through the copper filter, the low-energy signal is filtered, and the remaining high-energy X-ray is detected and imaged by the high-energy detector. The image can be processed after the transmission of the two different energy X-rays. Dual-source dual-energy X-ray imaging is adopted, which can accurately distinguish the composition proportion of materials (Fredenberg Citation2018). In combination with Relief and Pso-svm algorithm, the signal is processed by mathematical algorithm to eliminate parameters such as density and thickness, the R-value related to the effective atomic number is obtained, and the material type is determined accordingly. Compared with single-energy imaging, dual-energy imaging has no material differentiation ability (Han et al. Citation2016). Dual-energy imaging is related to the atomic sequence of materials, has no relation to the size of raw coal particles, and has an excellent recognition effect.
PGNAA uses the neutron stream generated by a neutron source to bombard the nuclei of various elements in the sample. The element produces a neutron capture reaction and is de-excited in 10 − 14 s–10 − 12 s, releasing energy of 2 keV–10 MeV γ-ray. An analysis system according to each characteristic γ online qualitative and quantitative analysis of elements is carried out according to the energy and intensity of rays (Zhang et al. Citation2005). The neutron source can be an isotope neutron source or an electrically controllable neutron source. The isotope neutron source includes an Am-Be source or a Cf source. The electrically controllable neutron source comprises a deuterium – tritium neutron generator or a deuterium – deuterium neutron generator (Bacal and Wada Citation2020). Based on PGNAA technology, it can accurately and online detect the characteristic parameters of coal mines with complex components, and provide time-sensitive garbage characteristic parameters, including element content, calorific value, ash content, fixed carbon, and water content (Hei et al. Citation2014). PGNAA technology is fast, accurate, and comprehensive, and achieves multielement analysis at the same time. It is highly suitable for online detection of main elements of ore and can solve the problems of low accuracy and low separation efficiency of current sorting equipment.
Improvement in the information processing algorithm of intelligent coal gangue separation equipment can also enhance the separation efficiency of the equipment. Common spectral analysis methods for intelligent sorters used in production are the peak area discussion method and the artificial neural network method (Hou Citation2019; Li et al. Citation2020). However, the peak area method is a quantitative analysis based on the scaling relation between the net or total area of the characteristic peaks of an element and the concentration of that element. It requires another spectral processing technology to overcome the overlapping peaks, absorption enhancement effect, and peak effect between elements (Luo et al. Citation2001). The artificial neural network method directly uses the content and intensity data of the known samples in many training samples to obtain a specific quantitative function, does not need to consider the complex relationship between the content and ray intensity, and indirectly overcomes the overlapping peaks and absorption enhancement effect between elements (Alfarzaeai et al. Citation2020; Anarghya, Anarghya, and Al Adhoubi Citation2020). However, the artificial neural network method needs a large amount of experimental data as the basis of model training, so it needs many experimental standard samples to be measured in different complex environments, which is time consuming and labor intensive. Partial least squares (PLS) and Monte Carlo Spectral Library Least Squares (MCLLS) are the methods that have attracted more attention in recent years. Giang et al. (Hwang and Cho Citation2020) improved the PLS method and successfully applied it to the analysis of heavy metals and copper ores. Urbański et al (Urbański and Kowalska Citation1995) applied the PLS method to analyze the composition and thickness of iron and zinc coatings in ore powder. The MCLLS method mainly uses the Monte Carlo method to simulate and calculate the single element spectral library and carries out the fitting calculation of the experimental spectrum through multiple cyclic iterations until the optimal solution is obtained (Cavalcanti et al. Citation2021). Both methods have the advantages of automatic energy peak identification, full spectrum fitting, and matrix effect automatic correction, which can greatly improve the separation accuracy and efficiency of intelligent coal gangue separation equipment. The improvement and joint application of more high-speed, high-precision algorithms are an important part of the future development of intelligent ore separation equipment. Application of online element rapid analysis system will promote the development of intelligent coal gangue separation equipment to a new level.
Conclusions
Reducing the amount of coal gangue in raw coal is one of the necessary ways to achieve the efficient, clean utilization of coal resources and ensure the development of clean, low-carbon, and green energy in China. The development of intelligent coal gangue sorting equipment can effectively increase the utilization rate of coal resources and enhance the economic benefits of enterprises. This process is necessary to automate coal mining and dressing.
Conventional coal gangue separation equipment, such as spiral separation equipment, heavy medium shallow trough separation equipment, and TBS interference bed separation equipment have the advantages of mature technology, high separation accuracy, and large processing capacity. However, due to the heavy investment in early construction, high production costs, and large water consumption and waste during production, the application of the equipment is severely limited.
Compared with traditional coal separation methods, photoelectric separation technology is applied in the field of coal gangue separation, which has the advantages of fast speed, high accuracy, strong modularity and integration scalability, low operation cost and energy consumption, convenient operation and maintenance, and no water use. TCS intelligent dry separation equipment and KRS intelligent dry separation device developed based on X-ray technology have been successfully applied in coal preparation plant and achieved good results.
With the continuous improvement of coal product demand and mine automation level, the application of high-speed, high-precision, and anti-interference online element rapid analysis technology in intelligent coal gangue separation equipment will become an inevitable trend of equipment development in the future. Currently, online rapid element analysis technology mainly includes LIBS, dual-energy X-ray, and PGNAA. Moreover, the improvement and joint application of more high-speed, high-precision algorithms are an important part of the future development of intelligent ore separation equipment. The application of PLS and MCLLS will further promote the development of intelligent ore separation equipment.
Acknowledgements
This study was financially supported by the National Natural Science Foundation of China (51874053, 52064016), the Scientific Research Foundation of State Key Laboratory of Coal Mine Disaster Dynamics and Control (2011DA105287-zd201804), Jiangxi Provincial Thousand Talents Plan Project (jxsq2019102082).
Disclosure statement
No potential conflict of interest was reported by the author(s).
Additional information
Funding
References
- Aberkane, S., A. M. Messaoud, K. Yahiaoui, C. Mahieddoune, S. Abdelli-Messaci, and M. A. Harith. 2018. Depth profiling of alumina thin films using laser induced breakdown spectroscopy: Structural and morphological dependence. Thin Solid Films 653 (May 1):293–300. doi:10.1016/j.tsf.2018.03.052.
- Alfarzaeai, M. S., Q. Niu, J. Q. Zhao, R. M. A. Eshaq, and E. Y. Hu. 2020. Coal/gangue recognition using convolutional neural networks and thermal images. Institute of Electrical and Electronics Engineers Access 8:76780–89. doi:10.1109/ACCESS.2020.2990200.
- Anarghya, A., A. Anarghya, and M. Al Adhoubi. 2020. A study on the behavior of CO2 corrosion on pipeline using computational fluid dynamics, experimental and artificial neural network approach. Engineering Research Express 2 (2):025012. doi:10.1088/2631-8695/ab69d6.
- Ashfaq, M., M. Lal, M. Heera, and A. B. Arif. 2020. Carbon footprint analysis of coal gangue in geotechnical engineering applications. Indian Geotechnical Journal 50 (4):646–54. doi:10.1007/s40098-019-00389-z.
- Bacal, M., and M. Wada. 2020. Negative ion source operation with deuterium. Plasma Sources Science and Technology 29 (3):3. doi:10.1088/1361-6595/ab6881.
- Cavalcanti, C., A. Halisson, S. D. B. Melo, D. A. Silva, M. Ricardo, and L. D. Carvalho. 2021. Spectral analysis and optimization of the condition number problem. Computer Physics Communications 258:258. doi:10.1016/j.cpc.2020.107587.
- Chen, L. 2016. The application of TBS interference bed sorter in qixing coal preparation plant. Shandong Industrial Technology 15:40–40. doi:10.16640/j.cnki.37-1222/t.2016.15.35.
- Chen, Q. 2015. The application of TBS interference bed separator for coarse slime in shaqu coal preparation plant. Coal and Chemical Industry (In Chinese) 38 (4):111–13.
- Ding, K. J., and J. S. Laskowski. 2006. Coal reverse flotation. Part I: Separation of a mixture of subbituminous coal and gangue minerals. Minerals Engineering 19 (1):72–78. doi:10.1016/j.mineng.2005.07.011.
- Dong, W. H. 2017. Application of heavy medium shallow trough separator in cold well coal preparation plant. Coal and Chemical Industry 6:86–88. doi:10.19286/j.cnki.cci.2017.06.028.
- Du, Q. 2021. Optimization and transformation of shallow trough heavy medium separator in coal preparation plant. Coal and Chemical Industry (In Chinese) 44 (12):86–90. doi:10.19286/j.cnki.cci.2021.12.025.
- Du, X. W. 2017. The 13th five-year period: A key period of China’s energy low-carbon transition. Electric Power 50 (2):1–4. doi:10.11930/j.issn.l004-9649.2017.02.001.04.
- Fredenberg, E. 2018. Spectral and dual-energy X-ray imaging for medical applications. Nuclear Instruments and Methods in Physics Research, Section A: Accelerators, Spectrometers, Detectors and Associated Equipment 878:74–87. doi:10.1016/j.nima.2017.07.044.
- Fuentes, R., D. Luarte, C. Sandoval, A. K. Myakalwar, J. Yanez, and D. Sbarbaro. 2021. Data fusion of laser induced breakdown spectroscopy and diffuse reflectance for improved analysis of mineral species in copper concentrates. Minerals Engineering 173:107193. doi:10.1016/j.mineng.2021.107193.
- Fu, C. C., F. L. Lu, and G. Y. Zhang. 2020. Discrimination analysis of coal and gangue using multifractal properties of optical texture. International Journal of Coal Preparation & Utilization 42 (7):1925–37. doi:10.1080/19392699.2020.1789974.
- Gao, C., and X. N. Fan. 2010. Application of spiral separator at panyi coal preparation plant. Coal Processing & Comprehensive Utilization 2:25–28. doi:10.16200/j.cnki.11-2627/td.2010.02.014.
- Guo, Y. C., L. He, P. Z. Liu, and X. Wang. 2021. Multi-dimensional analysis and recognition method of coal and gangue dual-energy X-ray images. Journal of China Coal Society (In Chinese) 46 (1):300–09. doi:10.13225/j.cnki.jccs.2020.1626.
- Guo, Y. C., X. Q. Wang, S. Wang, K. Hu, and W. S. Wang. 2021. Identification method of coal and coal gangue based on dielectric characteristics. Institute of Electrical and Electronics Engineers Access 9:9845–54. doi:10.1109/ACCESS.2021.3050196.
- Han, W., and W. M. Deng. 2021. Experimental study and prospect of photoelectric separation in a copper ore. Copper Engineering (In Chinese) 3:40–43.
- Han, H. J., S. H. Wang, K. Gao, Z. L. Wang, C. Zhang, M. Yang, K. Zhang, and P. P. Zhu. 2016. Preliminary research on dual-energy X-ray phase-contrast imaging. Chinese Physics C 40 (4):147–54. doi:10.1088/1674-1137/40/4/048201.
- Hei, D. Q., C. Cheng, W. B. Jia, Q. Shan, and Y. S. Ling. 2014. Preliminary study of sample uniformity impact on the elemental analysis in PGNAA technique. Nuclear Techniques (In Chinese) 37 (6):21–25. doi:10.11889/j.0253-3219.2014.hjs.37.060202.
- He, L., S. Wang, Y. C. Guo, K. Hu, G. Cheng, and X. Q. Wang. 2022. Study of raw coal identification method by dual-energy X-ray and dual-view visible light imaging. International Journal of Coal Preparation & Utilization 43 (2):361–76. doi:10.1080/19392699.2022.2051013.
- He, J. F., Y. M. Zhao, Y. Q. He, Z. F. Luo, and C. L. Duan. 2015. Comparison of coal separation characteristics based on different separating approaches in dry coal beneficiation flowsheet. Journal of Central South University 22 (5):1651–59. doi:10.1007/s11771-015-2683-7.
- Hou, W. 2019. Identification of coal and gangue by feed-forward neural network based on data analysis. International Journal of Coal Preparation & Utilization 39 (1):33–43. doi:10.1080/19392699.2017.1290609.
- Hou, X. X., and C. He. 2015. The application of TBS interference bed sorter in huangling No.1 coal mine coal preparation plant. Coal Quality Technology 2:69–70,72. doi:10.3969/j.issn.1007-7677.2015.02.019.
- Huang, X. Y. 2014. Application study on using of spiral separator in coal slime separation process. Coal Technology 33 (6):220–23. doi:10.13301/j.cnki.ct.2014.06.088.
- Hudson, S. W., J. Craparo, R. De, and A. D. Apelian. 2017. Applications of laser-induced breakdown spectroscopy (LIBS) in molten metal processing. Metallurgical and Materials Transactions B 48 (5):2731–42. doi:10.1007/s11663-017-1032-7.
- Hwang, H., and G. Cho. 2020. Global least squares path modeling: A full-information alternative to partial least squares path modeling. Psychometrika 85 (4):947–72. doi:10.1007/s11336-020-09733-2.
- Hyunwoo, L., C. Hyosung, L. Hunwoo, and J. Duhee. 2022. Quantification of dark-field effects in single-shot grid-based x-ray imaging. Journal of Optics (United Kingdom) 24 (3):035608. doi:10.1088/2040-8986/ac3f93.
- Kasim, A. F. A., M. A. Wakil, K. Grant, M. Hearn, and Z. T. Alwahabi. 2022. Aqueous ruthenium detection by microwave-assisted laser-induced breakdown spectroscopy. Plasma Science & Technology 24 (8):41–48. doi:10.1088/2058-6272/ac6733.
- Khanchi, A. R., H. Sedighi, S. Ansar, and J. Fasihi. 2014. Preconcentration of rare earth elements from Iranian monazite ore by spiral separator using multi-response optimization method. International Journal of Mining Science and Technology 24 (1):117–21. doi:10.1016/j.ijmst.2013.12.020.
- Li, H. 2019. Maintenance and improvement of structural components of shallow trough heavy medium separator in coal preparation plant. Mechanical Management and Development (In Chinese) 34 (10):273–4, 276. doi:10.16525/j.cnki.cn14-1134/th.2019.10.115.
- Li, M., X. L. He, Y. Duan, and M. L. Yang. 2021. Experimental study on the influence of external factors on image features of coal and gangue. International Journal of Coal Preparation & Utilization 42 (9):2770–87. doi:10.1080/19392699.2021.1901692.
- Li, G. Z., B. Klein, C. B. Sun, and J. Kou. 2020. Applying receiver-operating-characteristic (ROC) to bulk ore sorting using XRF. Minerals Engineering 146:106117. doi:10.1016/j.mineng.2019.106117.
- Li, G. Z., B. Klein, C. B. Sun, and J. Kou. 2021. Lab-scale error analysis on X-ray fluorescence sensing for bulk ore sorting. Minerals Engineering 164:164. doi:10.1016/j.mineng.2021.106812.
- Liu, M. B., W. Z. Yin, Y. X. Han, and Z. Q. Sun. 2012. The study on the X-Ray separator and its pre-sorting test of the low grade ore of mo and Ni. Mining and Metallurgy (In Chinese) 21 (4):26–29. doi:10.3969/j.issn.1005-7854.2012.04.007.
- Li, J. Y., and J. M. Wang. 2019. Comprehensive utilization and environmental risks of coal gangue: A review. Journal of Cleaner Production 239. doi:10.1016/j.jclepro.2019.117946.
- Li, L. H., H. J. Wang, and L. An. 2015. Research on recognition of coal and gangue based on image processing. World Journal of Engineering 12 (3):247–54. doi:10.1260/1708-5284.12.3.247.
- Li, G. F., D. Wang, Q. X. Liu, Y. M. Zhao, and C. L. Duan. 2022. Separation performance of 0.5–1 mm fine coal in a gas–solid fluidized bed without dense medium. Fuel 318:123645. doi:10.1016/j.fuel.2022.123645.
- Li, W. Y., W. H. Ye, and T. Z. Xiong. 2020. Metal identification algorithm based on bp neural network for dual energy x-ray transmission. Non-Ferrous Metal Engineering (In Chinese) 10 (8):124–30. doi:10.3969/j.issn.2095-1744.2020.08.020.
- Luo, L. Q., L. Gan, X. J. Wu, A. Ji, and G. L. Liang. 2001. Correction of nonlinear matrix effects by using an algorithm of combining neural networks with fundamental parameters. Analyze Laboratory Journals 20 (1):1–4. doi:10.13595/j.cnki.issn1000-0720.2001.0001.
- Luo, X. P., K. Z. He, Y. Zhang, P. Y. He, and Y. B. Zhang. 2022. A review of intelligent ore sorting technology and equipment development. International Journal of Minerals, Metallurgy & Materials 29 (9):1647–55. doi:10.1007/s12613-022-2477-5.
- Lv, Z. Q., W. D. Wang, K. H. Zhang, W. J. Li, J. D. Feng, and Z. Q. Xu. 2022. A synchronous detection-segmentation method for oversized gangue on a coal preparation plant based on multi-task learning. Minerals Engineering 187:107806–107806. doi:10.1016/j.mineng.2022.107806.
- Majumder, A. K., J. P. Barnwal, and N. Ramakrishnan. 2004. A new approach to evaluate the performance of gravity-based coal washing equipment. International Journal of Coal Preparation & Utilization 24 (5–6):277–84. doi:10.1080/07349340490888995.
- Meng, L. G., S. L. Gao, D. Z. Wei, B. Y. Cui, Y. B. Shen, and Z. G. Song. 2021. Investigation on the evolution of flow field stability in a spiral separator. Minerals Engineering 174:107224. doi:10.1016/j.mineng.2021.107224.
- Miao, X. X., and J. X. Zhang. 2014. Key technologies of integration of coal mining-gangue washing-backfilling and coal mining. Journal of China Coal Society 39 (8):1424–33. doi:10.13225/j.cnki.jccs.2014.9022.
- Qi, Z. Y., J. X. Guo, and T. Lian. 2018. Study on application of QG shallow trough heavy medium separator in lump coal reject discharge. Coal Engineering (In Chinese) 50 (11):71–73. doi:10.11799/ce201811018.
- Richards, R. G., D. M. MacHunter, P. J. Gates, and M. K. Palmer. 2000. Gravity separation of ultra-fine (−0.1mm) minerals using spiral separators. Minerals Engineering 13 (1):65–77. doi:10.1016/S0892-6875(99)00150-8.
- Robben, C., P. Condori, A. Pinto, R. Machaca, and A. Takala. 2020. X-ray-transmission based ore sorting at the San Rafael tin mine. Minerals Engineering 145:105870. doi:10.1016/j.mineng.2019.105870.
- Rocha, H. D., S. Siqueira, and R. J. Silva. 2021. Effects of coal compositions on the environment and economic feasibility of coal generation technologies. Sustainable Energy Technologies and Assessments 47:101500. doi:10.1016/j.seta.2021.101500.
- Ruan, L. J. 2022. Thinking on the future development of coal industry under the situation of “double carbon”. Coal Processing & Comprehensive Utilization 1:55–57. doi:10.16200/j.cnki.11-2627/td.2022.01.010.
- Sun, X. L., Y. H. Yue, C. X. Zhou, and Y. L. Kuang. 2021. The online correction of gamma-ray ash monitor in coal preparation plant. International Journal of Coal Preparation & Utilization 41 (2):144–57. doi:10.1080/19392699.2018.1455667.
- Urbański, P., and E. Kowalska. 1995. Application of partial least-squares calibration methods in low-resolution EDXRS. X-Ray Spectrometry 24 (2):70–75. doi:10.1002/xrs.1300240209.
- Wang, F. D., and G. Y. Wei. 2006. The application of spiral sorter in coal preparation plants. Coal Processing & Comprehensive Utilization 2:15–16. doi:10.3969/j.issn.1005-8397.2006.02.006.
- Wang, Z. X., S. X. Xie, G. D. Chen, W. Z. Chi, Z. H. Ding, and P. Wang. 2021. An online flexible sorting model for coal and gangue based on multi-information fusion. Institute of Electrical and Electronics Engineers Access 9:90816–27. doi:10.1109/ACCESS.2021.3090780.
- Wei, Z. Q. 2017. Analysis on feasibility of washing down to a further reduced size with shallow-trough heavy medium separator at buliangou coal washery. Coal Preparation Technology 1:70–72. in Chinese. doi:10.16447/j.cnki.cpt.2017.01.021.
- Xie, J. C., X. F. Cai, H. Q. Yang, Q. N. Meng, B. H. Li, Y. Yan, and L. Zhao. 2022. Coal gangue data set expansion method based on DCGAN[M]. 2nd International Conference on Consumer Electronics and Computer Engineering, ICCECE 2022, Guangzhou, China, 303–08.
- Yan, B. L. 2022. Analysis on consumption and saving situation of energy in coal. Coal Processing & Comprehensive Utilization 1:43–46. doi:10.16200/j.cnki.11-2627/td.2022.01.007.
- Yang, Q. 2018. Application and fault treatment of heavy medium shallow trough separator in coal preparation plant. Coal Mine Modernization (In Chinese) 6:161–63. doi:10.13606/j.cnki.37-1205/td.2018.06.054.
- Yan, P. C., Q. S. Sun, N. N. Yin, L. L. Hua, S. H. Shang, and C. Y. Zhang. 2022. Detection of coal and gangue based on improved YOLOv5.1 which embedded scSE module*. Measurement: Journal of the International Measurement Confederation 188:110530. doi:10.1016/j.measurement.2021.110530.
- Yu, X. D., Z. F. Luo, G. M. Li, and D. Q. Gan. 2019. Effects of characteristics of bed structure on separation of 6-0 mm high-sulfur fine coal in a compound dry separator. International Journal of Coal Preparation & Utilization 42 (3):286–304. doi:10.1080/19392699.2019.1599872.
- Yu, X. D., Z. F. Luo, H. B. Li, X. L. Yang, E. H. Zhou, H. S. Jiang, J. D. Wu, S. L. Song, and L. H. Cai. 2016. Effect of vibration on the separation efficiency of high-sulfur coal in a compound dry separator. International Journal of Mineral Processing 157:195–204. doi:10.1016/j.minpro.2016.10.008.
- Yu, W., H. L. Zhang, X. B. Wang, Z. U. Rahman, Z. C. Shi, Y. H. Bai, G. S. Wang, Y. Q. Chen, J. J. Wang, and L. J. Liu. 2022. Enrichment of residual carbon from coal gasification fine slag by spiral separator. Journal of Environmental Management 315:115149. doi:10.1016/j.jenvman.2022.115149.
- Zhai, X. 2022. Application of intelligent crude coal slme sorter in lutaishan coal preparation plant. Shanxi Coking Coal Science & Technology (In Chinese) 46 (6):23–5, 28. doi:10.3969/j.issn.1672-0652.2022.06.007.
- Zhang, L. 2020. Study on practical application of TBS interference bed separator in coal washing plant. Mechanical Management and Development (In Chinese) 35 (9):188–90. doi:10.16525/j.cnki.cn14-1134/th.2020.09.079.
- Zhang, J. X., P. Huang, Q. Zhang, M. Li, and Z. W. Chen. 2017. Stability and control of room mining coal pillars—taking room mining coal pillars of solid backfill recovery as an example. Journal of Central South University 24 (5):1121–32. doi:10.1007/s11771-017-3515-8.
- Zhang, J. X., F. Ju, M. Li, N. Zhou, and Q. Zhang. 2020. Method of coal gangue separation and coordinated in-situ backfill mining. Journal of China Coal Society 45 (1):131–40. doi:10.13225/j.cnki.jccs.YG19.1826.
- Zhang, L. Z., B. F. Ni, W. Z. Tian, D. H. Huang, G. Y. Zhang, C. X. Liu, P. S. Wang, L. K. Liu, and D. H. Li. 2005. Status and development of prompt γ-ray neutron activation analysis. Atomic Energy Science and Technology 39 (3):282–88. doi:10.3969/j.issn.1000-6931.2005.03.022.
- Zhao, Y. 2016. The working principle and application of QG series shallow groove scraper heavy medium sorting machine. Coal Processing & Comprehensive Utilization 11:13–14. doi:10.16200/j.cnki.11-2627/td.2016.11.004.
- Zhao, Y. H., and J. H. Kuang. 2014. Discussion on the application of spiral separator in coal preparation plant. Shandong Coal Science & Technology 5:182–84. doi:10.3969/j.issn.1005-2801.2014.05.77.
- Zheng, K. H., C. L. Du, J. P. Li, and B. J. Qiu. 2016. Coal and gangue underground pneumatic separation effect evaluation influenced by different airflow directions. Advances in Materials Science and Engineering 2016:1–13. doi:10.1155/2016/6465983.
- Zhou, J. H. 2022. Thinking on intelligent development stage of coal preparation plan. Coal Processing & Comprehensive Utilization 1:34–39. doi:10.16200/j.cnki.11-2627/td.2022.01.005.
- Zhu, P. 2023. Application of two stage spiral sorter for separating difficult to float fine coal slime process. Energy and Environmental Protection 45 (3):194–98. doi:10.19389/j.cnki.1003-0506.2023.03.033.
- Zi, W. 2015. Application of interference bed separator in slime. Inner Mongolia Coal Economy (In Chinese) 8:182–84. doi:10.13487/j.cnki.imce.007009.
- Zou, L., X. H. Yu, M. Li, M. Lei, and H. Yu. 2020. Nondestructive identification of coal and gangue via near-infrared spectroscopy based on improved broad learning[J]. IEEE Transactions on Instrumentation and Measurement 69 (10):1–1. doi:10.1109/TIM.2020.2988169.