Abstract
This paper reviews the possible origin of sperm DNA fragmentation and focuses on the nuclear events associated with spermiogenesis as a potential source of genetic instability and reduced fertilizing potential of the mature male gamete. Recent findings suggest a programmed DNA fragmentation and DNA damage response during the chromatin remodeling steps in spermatids. We also discuss the spermatid DNA repair mechanisms and the possible involvement of condensing proteins, such as transition proteins and protamines, in the process, as this DNA fragmentation is normally not found in late spermatids. We propose that alterations in the chromatin remodeling steps or DNA repair in elongating spermatids may lead to persistent DNA breaks. This vulnerable step of spermiogenesis may provide a clue to the etiology of sperm DNA fragmentation associated with infertility in humans. This vulnerability is further emphasized given the haploid character of spermatids that must resolve programmed double-stranded breaks by an error-prone DNA repair mechanism. Therefore, spermiogenesis has probably been overlooked as an important source of genetic instability.
Abbreviations | ||
AcH4 | = | Hyperacetylated histone H4 |
ART | = | Assisted reproduction technology |
SCSA | = | sperm chromatin structure assay |
BER | = | Base excision repair |
DDR | = | DNA damage response |
DSB | = | Double-strand breaks |
ES | = | Elongating spermatid |
ICSI | = | Intracytoplasmic sperm injection |
MMR | = | Mismatch repair |
NHEJ | = | Non-homologous end joining |
ROS | = | Reactive oxygen species |
SCSA | = | Sperm chromatin structure assay |
TDP1 | = | Tyrosyl-DNA phosphodiesterase 1 |
TP | = | Transition protein |
TUNEL | = | Terminal deoxynucleotidyl transferase-mediated dUTP-nick end labeling |
HR | = | homologous recombination |
TOP2B | = | topoIIβ: topoisomerase IIβ |
Introduction
Male infertility is often associated with a relatively high DNA fragmentation level and an altered or incomplete chromatin packaging in mature spermatozoa [Fatehi et al. [Citation2006]; Irvine et al. [Citation2000]; Muratori et al. [Citation2000]]. In fact, DNA fragmentation is apparently linked to abnormal chromatin packaging in mature sperm [Gorczyca et al. [Citation1993]; Manicardi et al. [Citation1995]]. Assessment of sperm chromatin structure has been shown to have a good predictive value in the assessment of human fertility over the classical parameters recommended by WHO [Virro et al. [Citation2004]]. Alterations in the nuclear integrity of the male gametes have also been associated with de novo genetic disorders, developmental and morphological defects, cancer and miscarriage [Emery and Carrell [Citation2006]; Kleinhaus et al. [Citation2006]; Marchetti and Wyrobek [Citation2005]; Tesarik et al. [Citation2004]]. Sperm DNA fragmentation can also compromise ART (assisted reproduction technology) [Tesarik et al. [Citation2004]].
New tools were developed to assess the nuclear integrity of spermatozoa [for a review, see Evenson and Wixon [Citation2006]]. These include the sperm chromatin structure assay (SCSA) and the terminal deoxynucleotidyl transferase-mediated dUTP-nick end labeling (TUNEL) a sensitive tool to label DNA at strand breaks. The origin of DNA fragmentation in human spermatozoa remains unknown and multiple sources have been proposed including abortive apoptosis, abnormal chromatin packaging, generation of reactive oxygen species and premature release of spermatids from Sertoli cells [Muratori et al. [Citation2006]; Oliva [Citation2006]; Sakkas et al. [Citation2002]; Tesarik et al. [Citation2006]]. We have also proposed that the DNA fragmentation detected in mature spermatozoa may originate from the persistence of the transient DNA strand breaks observed at mid-spermiogenesis steps [Laberge and Boissonneault [Citation2005a]]. These transient DNA strand breaks appear to be part of the normal differentiation program of the spermatids and are produced to either support the important change in DNA topology or as a result of a transient exposure to endogenous nuclease during the chromatin remodeling.
Although male infertility is affected by a variety of environmental, behavioral and genetic factors, resulting in the impairment of spermatogenesis at various levels [Toshimori et al. [Citation2004]], the present review focuses on the nuclear events associated with spermiogenesis as a potential source of genetic instability and reduced fertilizing potential of the mature male gamete.
Abortive apoptosis
During spermatogenesis, apoptosis is essential for balancing the Sertoli cell/germ cell ratio. Sakkas and colleagues previously reported the high occurrence of the cell surface protein Fas, in the ejaculates from patients with abnormal semen parameters [Sakkas et al. [Citation1999]]. Apoptosis-like features other than the Fas ligand have also been observed in sperm including ultrastructural defects such as irregular, enlarged or fragmented nuclei with fragmented or marginated chromatin, cytoplasms with aggregated organelles, alteration in mitochondrial integrity, the presence of Bcl-x, caspases and p53 [Baccetti et al. [Citation1997]; Donnelly et al. [Citation2000]; Gandini et al. [Citation2000]; Sakkas et al. [Citation2002]; Weng et al. [Citation2002]]. Clear evidence linking DNA fragmentation as shown by TUNEL to these features has not been presented [Muratori et al. [Citation2006]].
It is becoming clear that TUNEL positivity may not be considered as a specific marker of apoptosis. The origin of sperm DNA fragmentation, determined by this technique, cannot be related to programmed cell death. During spermatogenesis, all meiotic cells demonstrate TUNEL positivity likely because of chromosomal crossover events that necessitate double-strand breaks [Marcon and Boissonneault [Citation2004]].
Reactive oxygen species
The presence of reactive oxygen species (ROS) is associated with a loss of sperm motility and fertilizing potential, but also with DNA damage [Aitken and Krausz [Citation2001]]. Specific ROS-induced damage include loss of sperm motility, often caused by the retention of residual cytoplasm around the midpiece, and alteration of plasma membrane compromising membrane fusion events associated with fertilization. ROS-induced DNA damage was observed in ejaculates of infertile men, reflected by the measurements of 8-hydroxydeoxyguanosine (8-OhdG) [Irvine et al. [Citation2000]]. Secretion of antioxidant molecules or enzymes protects sperm cells against ROS in reproductive tracts [O et al. [Citation2006]]. ROS-induced DNA damage may arise through the imbalance between the endogenous or xenobiotics-induced ROS generation and the natural protection provided by the accessory sex glands. Moreover, use of antioxidants has led to some relative protection of human sperm DNA fragmentation [reviewed by Agarwal et al. [Citation2004]] and improved ICSI outcome [Greco et al. [Citation2005]].
Human spermatozoa are potentially more sensitive to ROS because of their relatively high residual content of histones reaching about 10 to 20 percent [Dadoune [Citation2003]; Wykes and Krawetz [Citation2003]] compared to animal models where about 1% of mouse sperm DNA remains bound to histones [van der Heijden et al. [Citation2006]]. On an evolutionary basis, this may indicate an elevated transmission of epigenetic marks to offspring but this may produce a more vulnerable genome. Protamination protects DNA from many insults as it creates an almost crystal-like chromatin structure, making it almost inaccessible to nucleases and mutagens [Oliva [Citation2006]]. The change in DNA topology during the chromatin remodeling steps may, by itself, account for decreased sensitivity to DNA damage as the linear, non-supercoiled DNA of the condensed spermatid may be less vulnerable to damaging agents. One may hypothesize that the arginine-rich protamines, once bound to DNA, may chemically protect spermatozoa from ROS damage as polycationic molecules (polyamines) are known as free radical scavengers [Chattopadhyay et al. [Citation2003]; Chattopadhyay et al. [Citation2006]; von Deutsch et al. [Citation2005]]. Sotolongo and colleagues [Sotolongo et al. [Citation2003]] proposed a model of sperm chromatin organization by protamines where sperm DNA is condensed by forming toroids, which consist of approximately 50 kilobases attached to the nuclear matrix by a nuclease-sensitive linker. These observations strongly suggest that a limited portion of the genomic DNA of mature sperm is sensitive to nucleases or other genetic insults. Therefore, one can assume that the packaging quality may influence the contribution of ROS to DNA fragmentation.
Incomplete packaging
Post-translational Modifications
Striking chromatin reconstruction takes place during spermiogenesis. Although some differences have been observed among mammals [Dadoune [Citation2003]; Oliva [Citation2006]], chromatin remodeling relies on a conserved sequence of events, that is, the incorporation of histone variants and covalent modifications of nucleosomal histones, such as acetylation and ubiquitination, the displacement of most histones by transition proteins (TPs), followed by the final deposition of protamines. Although little is known about this process, one may surmise that any alteration in this nuclear sequence of events is likely to have significant consequences on the genetic integrity of the gamete. Human homologues of RAD6, a yeast ubiquitin-conjugating enzyme, have been implicated in post-replication repair and damage-induced mutagenesis. In HR6B-null mice, spermiogenesis is not arrested but more than 90% of spermatozoa show aberrant head morphology with deformated flagella, suggesting its role in post-meiotic chromatin remodeling [Roest et al. [Citation1996]]. Decreased acetylation of histone H4 is correlated with infertility [Sonnack et al. [Citation2002]]. Moreover, hyperacetylation seems to be related to the control of transient DNA fragmentation [Bird et al. 2002; Laberge and Boissonneault 2005b; Marcon and Boissonneault [Citation2004]; Martínez-López et al. [Citation2001]]. The association of histone hyperacetylation with an increase in DNA fragmentation is not surprising as previous reports indicate that this post-translational modification of histone H4 in somatic cells enhances sensitivity to endonucleases [Martínez-López et al. [Citation2001]].
Transition proteins
The role of TPs and their structural properties are not well understood, but they play an important role in the condensation of the spermatid nucleus, as mice with deletions of both major transition proteins, TP1 and TP2, are infertile [Shirley et al. [Citation2004]; Zhao et al. [Citation2004]]. As demonstrated by Shirley and colleagues [Shirley et al. [Citation2004]], the interplay between TPs and protamines is quite complex. Although a decrease in the total TP content has a direct effect on fertility, the absence of one can be compensated by the increased expression of the other, suggesting that TPs have redundant functions. However, mutants with two copies of Tnp1 but no Tnp2 (producing 35% of normal TP1 level) have severely defected sperm compared to those with two copies of Tnp2 and no Tnp1 (producing 30% of the normal TP2 level). This indicates a unique role for TP2. It remains clear that the absence of both transition proteins leads to a persistence of endogenous DNA strand breaks in a fraction of ES suggesting that the combined DNA-condensing activity of these proteins is important to the genetic integrity of the developing male gamete. In this regard, it was demonstrated that TP1 facilitates DNA ligation and their detection in the nucleus coincide with a decrease in DNA fragmentation [Caron et al. [Citation2001]; Kierszenbaum [Citation2001]; Levesque et al. [Citation1998]]. In double TP knockout models, it is noteworthy that DNA fragmentation does not persist in some ES and that DNA repair, as seen by a decrease in TUNEL labeling, seems to proceed normally. Hence, the DNA condensing ability of the protamines (discussed below) may compensate for the lack of TPs. Clearly, the potential link between the architectural DNA binding and condensing activity of these proteins and the stimulation of DNA repair needs to be further investigated.
Protamines
Protamines are essential to DNA integrity and the ultimate compaction of the sperm head. The abnormal ratio of protamine 1 (PRM1) and 2 (PRM2) is found in some infertile men, suggesting that the relative levels of protamines are important for complete spermiogenesis. Protamine deficiency potentially has diverse origins. For a recent review, see [Carrell et al. [Citation2007]]. Mutations in the PRM1 and PRM2 genes have been identified in infertile men [Aoki et al. [Citation2006b]; Ravel et al. [Citation2007]]. Lower expression of one or both genes is associated with severe male infertility and also DNA damage in spermatozoa as evidenced by acridine orange staining [Aoki et al. [Citation2006a]; Cho et al. [Citation2003]]. Defects in the regulation of expression of protamines have been observed in infertile men as premature expression or retention of mRNA leads to infertility [Aoki et al. [Citation2006b]; Lee et al. [Citation1995]]. Also, proper post-translational modifications of protamines appear essential. Camk4, a multifunctional serine/threonine protein kinase may play a role in the exchange of TPs to protamine, as CAMK4 phosphorylates PRM2 in vitro and Camk4-null mice demonstrated prolonged retention of TP2 [Wu et al. [Citation2000]]. Moreover, the final step of this chromatin remodeling, the creation of inter- and intra-molecule disulfide bonds, is also known to affect fertility as suggested by the higher sensitivity of DNA damage of marsupial spermatozoa that do not possess such a capacity [Bennetts and Aitken [Citation2005]].
Endogenous DNA fragmentation and repair as part of normal spermiogenesis
Mammalian elongating spermatids (ES) have a unique nuclear status. McPherson and Longo [[Citation1992]] first observed that steps 12 and 13 of spermiogenesis in the rat showed DNAse I hypersensitivity. Even without DNAse treatment, ES were labeled by nick translation, suggesting endogenous DNA fragmentation. They later postulated the implication of type II topoisomerases in the process of relaxing DNA supercoiling for the final protamination of sperm DNA [McPherson and Longo [Citation1993]]. Other reports suggested the involvement of topoisomerases during this process [Chen and Longo [Citation1996]; Cobb et al. [Citation1997]; Roca and Mezquita [Citation1989]]. In agreement with these observations, we demonstrated that 100% of spermatids undergo transient DNA breaks during steps 9 to 12 of mouse spermiogenesis as evidenced by TUNEL positivity [Marcon and Boissonneault [Citation2004]]. As demonstrated earlier by McPherson and Longo [[Citation1993]] and confirmed later by our group, condensed spermatids (steps 14-16 in mouse) and epidimydal spermatozoa, showed little to no DNA fragmentation after deprotamination and decondensation respectively, confirming the transient character of DNA fragmentation during spermiogenesis ().
FIGURE 1 Schematic representation of immunofluorescence expression of different proteins or activity in the nucleus of spermatids during mouse spermiogenesis.
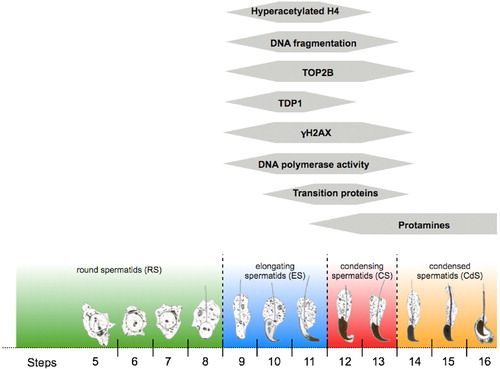
In an effort to identify the enzyme responsible and the nature of these DNA breaks, we first used COMET assays to demonstrate that most of the DNA fragmentation encountered during the chromatin remodeling were double-stranded therefore supporting the involvement of a type II topoisomerase. In addition, we observed a severe decrease in DNA fragmentation when spermatids were incubated with two topoII inhibitors, namely etoposide and suramin [Laberge and Boissonneault [Citation2005a]]. Using confocal microscopy, we recently confirmed the presence of topoisomerase IIβ (TOP2B, topoIIβ) distributed in foci during steps 9 to 13 of mouse spermiogenesis but did not observe the presence of the α isoform at these steps [Leduc et al. unpublished results]. Interestingly, we identified the presence of tyrosyl-DNA phosphodiesterase 1(TDP1), an enzyme known to resolve topoisomerases-mediated DNA damage [Interthal et al. [Citation2005]; Nitiss et al. [Citation2006]; Raymond and Burgin [Citation2006]]. The presence of TDP1 is coincident with the appearance of topoIIβ in ES. Thus, one can hypothesize that TDP1 actively removes topoIIβ cleavable complexes during spermiogenesis, leaving a DSB. TDP1 was first identified by mass spectrometry following co-immunoprecipitation of hyperacetylated histone H4 (AcH4) from sonication resistant spermatid extracts (unpublished results) and later by immunofluorescence and immunoblots. The association of a repair enzyme such as TDP1 with AcH4 is consistent with the known requirement of histone hyperacetylation at sites of damage [Costelloe et al. [Citation2006]].
In this respect, Meyer-Ficca and colleagues [Meyer-Ficca et al. [Citation2005]] demonstrated the presence of poly(ADP)ribosyl polymers in rat ES as well as γH2AX foci. Consistent with these observations, the PARP2 knockout mouse was associated with severely compromised differentiation of spermatids and delays in elongation [Dantzer et al. [Citation2006]]. We confirmed the presence of γH2AX foci in mouse spermiogenesis and correlated this with TUNEL positivity, suggesting a genome-wide DNA damage response (DDR) as part of the normal program of spermiogenesis. Hence, DNA fragmentation shown by TUNEL is not an artefact generated by tissue fixation, as γH2AX is a biological marker of double-strand breaks (DSBs). Because of the presence of TDP1, it is tempting to speculate that part of the topoII-generated double-stranded breaks may not be processed correctly in the context of such an important chromatin remodeling and condensation. The activation of H2AX and the poly(ADP)rybosylation of histones at break sites therefore act as markers of the resulting damage and the DNA repair process may ensue independently from topoII since no fragmentation is observed in condensed spermatids and the presence of DNA polymerase activity at these late steps has been demonstrated [Hecht and Parvinen [Citation1981]]. DNA polymerase activity in non-replicating cells is indeed associated with repair. We have also found endogenous DNA polymerase activity present in elongating and condensing spermatids in accordance with those of others [Hecht and Parvinen [Citation1981]], demonstrating a unique DNA polymerase profile of ES. Interestingly, deletion of pol λ in mice, a member of the X family of DNA polymerase, leads to infertility. However, this is apparently due to a lack of motility rather than genomic instability, as microinjection of pol λ-null mice produces normal offspring [Kobayashi et al. [Citation2002]]. Consequently, considering DNA strand breakage and the signature of the repair activity, higher p53 levels found in ejaculates of infertile men [Sakkas et al. [Citation2002]] may not be related to apoptosis but rather to DNA repair.
Further evidence of a DNA repair system in spermatids
Elongating spermatids, being haploid, cannot undergo homologous recombination (HR). Thus, we have hypothesized that the spermatid must rely on the more error-prone non-homologous end joining (NHEJ) for the repair of their endogenous DSBs [Leduc et al. unpublished results]. Components of NHEJ have been previously observed in spermatids [Goedecke et al. [Citation1999]], although not considered because of their lower level compared to other germinal cell types. We detected the presence of phosphorylated DNApkcs at the condensing steps of spermiogenesis [Leduc et al. unpublished results]. DNApkcs is a member of the phospho-inositide 3-kinase family and usually part of the NHEJ. Evidence from the literature indicates that other DNA repair systems may play a role during spermiogenesis. Elements of the base excision repair (BER) have been identified in elongating and elongated spermatids [Olsen et al. [Citation2001]]. In addition, there is evidence that mismatch repair (MMR) involving the MSH2 protein, is playing a role during spermiogenesis. Using a Huntington disease mouse model, carrying a high number of CAG repeats, McMurray and Kovtun demonstrated that the repeat expansion was occurring between round spermatids and spermatozoa [McMurray and Kortun [Citation2003]]. Interestingly, the deletion of MSH2 in this mice model abolished the expansion [Kovtun and McMurray [Citation2001]], suggesting that MSH2 is active when extensive DNA repair occurs. Additional efforts are needed to identify the proteins involved in the repair process of ES.
Conclusion and perspectives
There is now an increasing interest in the impact of paternal age on fertility and embryo development. Recently, many reports suggested a clear relationship between paternal age and diverse pathologies in offspring such as autism, schizophrenia and Down's syndrome [Fisch et al. [Citation2003]; Malaspina et al. [Citation2001]; Reichenberg et al. [Citation2006]]. Others proposed a link between the age of the father and miscarriage [Kleinhaus et al. [Citation2006]]. Increased DNA fragmentation as determined by SCSA, mutagenesis and defective repair processes that occur with age have been proposed as underlying etiological factors [Marchetti and Wyrobek [Citation2005]; Wyrobek et al. [Citation2006]]. Interestingly, Schmid and colleagues [Schmid et al. [Citation2006]] explored sperm DNA damage and the influence of several lifestyle factors in healthly male non-smokers and found aging to be associated with single-strand breaks. Aside from the age-related effect, they also observed that caffeine consumption produces increased sperm DNA damage associated with DSBs. Quite interestingly, caffeine is a known inhibitor of members of the phospho-inositide 3-kinase family, implicated in many processes in meiotic cells. Recent published results indicate that caffeine may also lead to inactivation of H2AX and DNA repair (NHEJ) [Rybaczek et al. [Citation2007]]. Thus, impairment in signaling and DNA repair during spermiogenesis may likely result in persistent DSBs in mature spermatozoa.
This paper suggests that spermiogenesis has probably been overlooked as an important source of genomic instability. Spermiogenesis may, in fact, be the most crucial period of spermatogenesis because, despite their haploid character, spermatids must resolve these programmed double-stranded breaks. Given the limited repair capacity of the oocyte many idiopathic cases of infertility or embryo loss may be understood by a better knowledge of the chromatin steps of spermiogenesis. One must consider the distribution of strand breakage within the haploid genome rather that by the total number of break sites. Further investigation should provide important clues regarding the genetic consequences of the endogenous DNA strand breaks and repair in spermatids.
Acknowledgments
This work was supported by the Canadian Institutes of Health Research, grant MOP-74500 (Bird et al., MOP-74500) to G.B.
References
- Agarwal A., Nallella K., Allamaneni S., Said T. Role of antioxidants in treatment of male infertility: an overview of the literature. Reprod Biomed Online 2004; 8: 616–627
- Aitken R., Krausz C. Oxidative stress, DNA damage and the Y chromosome. Reproduction 2001; 122: 497–506
- Aoki V., Emery B., Liu L., and Carrell D. Protamine levels vary between individual sperm cells of infertile human males and correlate with viability and DNA integrity. J Androl 2006a; 27: 890–898
- Aoki V., Liu L., Carrell D. A novel mechanism of protamine expression deregulation highlighted by abnormal protamine transcript retention in infertile human males with sperm protamine deficiency. Mol Hum Reprod 2006b; 12: 41–50
- Baccetti B., Strehler E., Capitani S., Collodel G., De Santo M., Moretti E., Piomboni P., Wiedeman R., Sterzik K. The effect of follicle stimulating hormone therapy on human sperm structure (Notulae seminologicae 11). Hum Reprod 1997; 12: 1955–1968
- Bennetts L., Aitken R. A comparative study of oxidative DNA damage in mammalian spermatozoa. Mol Reprod Dev 2005; 71: 77–87
- Caron N., Veilleux S., Boissonneault G. Stimulation of DNA repair by the spermatidal TP1 protein. Mol Reprod Dev 2001; 58: 437–443
- Carrell D., Emery B., Hammoud S. Altered protamine expression and diminished spermatogenesis: what is the link?. Hum Reprod Update 2007; 13: 313–327
- Chattopadhyay M., Tabor C., Tabor H. Polyamines protect Escherichia coli cells from the toxic effect of oxygen. Proc Natl Acad Sci USA 2003; 100: 2261–2265
- Chattopadhyay M., Tabor C., Tabor H. Polyamine deficiency leads to accumulation of reactive oxygen species in a spe2Delta mutant of Saccharomyces cerevisiae. Yeast 2006; 23: 751–761
- Chen J., Longo F. Expression and localization of DNA topoisomerase II during rat spermatogenesis. Mol Reprod Dev 1996; 45: 61–71
- Cho C., Jung-Ha H., Willis W., Goulding E., Stein P., Xu Z., Schultz R., Hecht N., Eddy E. Protamine 2 deficiency leads to sperm DNA damage and embryo death in mice. Biol Reprod 2003; 69: 211–217
- Cobb J., Reddy R., Park C., Handel M. Analysis of expression and function of topoisomerase I and II during meiosis in male mice. Mol Reprod Dev 1997; 46: 489–498
- Costelloe T., Fitzgerald J., Murphy N., Flaus A., Lowndes N. Chromatin modulation and the DNA damage response. Exp Cell Res 2006; 312: 2677–2686
- Dadoune J. Expression of mammalian spermatozoal nucleoproteins. Microsc Res Tech 2003; 61: 56–75
- Dantzer F., Mark M., Quenet D., Scherthan H., Huber A., Liebe B., Monaco L., Chicheportiche A., Sassone-Corsi P., de Murcia G., et al. Poly(ADP-ribose) polymerase-2 contributes to the fidelity of male meiosis I and spermiogenesis. Proc Natl Acad Sci USA 2006; 103: 14854–14859
- Donnelly E., O'Connell M., McClure N., Lewis S. Differences in nuclear DNA fragmentation and mitochondrial integrity of semen and prepared human spermatozoa. Hum Reprod 2000; 15: 1552–1561
- Emery B., Carrell D. The effect of epigenetic sperm abnormalities on early embryogenesis. Asian J Androl 2006; 8: 131–142
- Evenson D. P., Wixon R. Clinical aspects of sperm DNA fragmentation detection and male infertility. Theriogenology 2006; 65: 979–991
- Fatehi A. N., Bevers M. M., Schoevers E., Roelen B. A., Colenbrander B., Gadella B. M. DNA damage in bovine sperm does not block fertilization and early embryonic development but induces apoptosis after the first cleavages. J Androl 2006; 27: 176–188
- Fisch H., Hyun G., Golden R., Hensle T. W., Olsson C. A., Liberson G. L. The influence of paternal age on down syndrome. J Urol 2003; 169: 2275–2278
- Gandini L., Lombardo F., Paoli D., Caponecchia L., Familiari G., Verlengia C., Dondero F., Lenzi A. Study of apoptotic DNA fragmentation in human spermatozoa. Hum Reprod 2000; 15: 830–839
- Goedecke W., Eijpe M., Offenberg H., van Aalderen M., Heyting C. Mre11 and Ku70 interact in somatic cells, but are differentially expressed in early meiosis. Nat Genet 1999; 23: 194–198
- Gorczyca W., Traganos F., Jesionowska H., Darzynkiewicz Z. Presence of DNA strand breaks and increased sensitivity of DNA in situ to denaturation in abnormal human sperm cells: analogy to apoptosis of somatic cells. Exp Cell Res 1993; 207: 202–205
- Greco E., Romano S., Iacobelli M., Ferrero S., Baroni E., Minasi M. G., Ubaldi F., Rienzi L., Tesarik J. ICSI in cases of sperm DNA damage: beneficial effect of oral antioxidant treatment. Hum Reprod 2005; 20: 2590–2594
- Hecht N., Parvinen M. DNA synthesis catalysed by endogenous templates and DNA-dependent DNA polymerases in spermatogenic cells from rat. Exp Cell Res 1981; 135: 103–114
- Interthal H., Chen H., Champoux J. Human Tdp1 cleaves a broad spectrum of substrates, including phosphoamide linkages. J Biol Chem 2005; 280: 36518–36528
- Irvine D. S., Twigg J. P., Gordon E. L., Fulton N., Milne P. A., Aitken R. J. DNA integrity in human spermatozoa: relationships with semen quality. J Androl 2000; 21: 33–44
- Kierszenbaum A. Transition nuclear proteins during spermiogenesis: unrepaired DNA breaks not allowed. Mol Reprod Dev 2001; 58: 357–358
- Kleinhaus K., Perrin M., Friedlander Y., Paltiel O., Malaspina D., Harlap S. Paternal age and spontaneous abortion. Obstet Gynecol 2006; 108: 369–377
- Kobayashi Y., Watanabe M., Okada Y., Sawa H., Takai H., Nakanishi M., Kawase Y., Suzuki H., Nagashima K., Ikeda K., et al. Hydrocephalus, situs inversus, chronic sinusitis, and male infertility in DNA polymerase lambda-deficient mice: possible implication for the pathogenesis of immotile cilia syndrome. Mol Cell Biol 2002; 22: 2769–2776
- Kovtun I., McMurray C. Trinucleotide expansion in haploid germ cells by gap repair. Nat Genet 2001; 27: 407–411
- Laberge R. M., Boissonneault G. Chromatin remodeling in spermatids: a sensitive step for the genetic integrity of the male gamete. Arch Androl 2005a; 51: 125–133
- Laberge R., Boissonneault G. On the nature and origin of DNA strand breaks in elongating spermatids. Biol Reprod 2005b; 73: 289–296
- Lee K., Haugen H., Clegg C., Braun R. Premature translation of protamine 1 mRNA causes precocious nuclear condensation and arrests spermatid differentiation in mice. Proc Natl Acad Sci USA 1995; 92: 12451–12455
- Levesque D., Veilleux S., Caron N., Boissonneault G. Architectural DNA-binding properties of the spermatidal transition proteins 1 and 2. Biochem Biophys Res Commun 1998; 252: 602–609
- Malaspina D., Harlap S., Fennig S., Heiman D., Nahon D., Feldman D., Susser E. S. Advancing paternal age and the risk of schizophrenia. Arch Gen Psychiatry 2001; 58: 361–367
- Manicardi G. C., Bianchi P. G., Pantano S., Azzoni P., Bizzaro D., Bianchi U., Sakkas D. Presence of endogenous nicks in DNA of ejaculated human spermatozoa and its relationship to chromomycin A3 accessibility. Biol Reprod 1995; 52: 864–867
- Marchetti F., Wyrobek A. J. Mechanisms and consequences of paternally-transmitted chromosomal abnormalities. Birth Defects Res C Embryo Today 2005; 75: 112–129
- Marcon L., Boissonneault G. Transient DNA strand breaks during mouse and human spermiogenesis new insights in stage specificity and link to chromatin remodeling. Biol Reprod 2004; 70: 910–918
- Martínez-López W., Folle G., Obe G., Jeppesen P. Chromosome regions enriched in hyperacetylated histone H4 are preferred sites for endonuclease- and radiation-induced breakpoints. Chromosome Res 2001; 9: 69–75
- McMurray C., Kortun I. Repair in haploid male germ cells occurs late in differentiation as chromatin is condensing. Chromosoma 2003; 111: 505–508
- McPherson S., Longo F. Localization of DNase I-hypersensitive regions during rat spermatogenesis: stage-dependent patterns and unique sensitivity of elongating spermatids. Mol Reprod Dev 1992; 31: 268–279
- McPherson S., Longo F. Nicking of rat spermatid and spermatozoa DNA: possible involvement of DNA topoisomerase II. Dev Biol 1993; 158: 122–130
- Meyer-Ficca M., Scherthan H., Burkle A., Meyer R. Poly(ADP-ribosyl)ation during chromatin remodeling steps in rat spermiogenesis. Chromosoma 2005; 114: 67–74
- Muratori M., Marchiani S., Maggi M., Forti G., Baldi E. Origin and biological significance of DNA fragmentation in human spermatozoa. Front Biosci 2006; 11: 1491–1499
- Muratori M., Piomboni P., Baldi E., Filimberti E., Pecchioli P., Moretti E., Gambera L., Baccetti B., Biagiotti R., Forti G., et al. Functional and ultrastructural features of DNA-fragmented human sperm. J Androl 2000; 21: 903–912
- Nitiss K., Malik M., He X., White S., Nitiss J. Tyrosyl-DNA phosphodiesterase (Tdp1) participates in the repair of Top2-mediated DNA damage. Proc Natl Acad Sci USA 2006; 103: 8953–8958
- O W. S., Chen H., Chow P. H. Male genital tract antioxidant enzymes – their ability to preserve sperm DNA integrity. Mol Cell Endocrinol 2006; 250: 80–83
- Oliva R. Protamines and male infertility. Hum Reprod Update 2006; 12: 417–435
- Olsen A., Bjørtuft H., Wiger R., Holme J., Seeberg E., Bjørås M., Brunborg G. Highly efficient base excision repair (BER) in human and rat male germ cells. Nucleic Acids Res 2001; 29: 1781–1790
- Ravel C., Chantot-Bastaraud S., El Houate B., Berthaut I., Verstraete L., De Larouziere V., Lourenčo D., Dumaine A., Antoine J., Mandelbaum J., et al. Mutations in the protamine 1 gene associated with male infertility. Mol Hum Reprod 2007; 13: 461–464
- Raymond A., Burgin A. Tyrosyl-DNA phosphodiesterase (Tdp1) (3’-phosphotyrosyl DNA phosphodiesterase). Meth Enzymol 2006; 409: 511–524
- Reichenberg A., Gross R., Weiser M., Bresnahan M., Silverman J., Harlap S., Rabinowitz J., Shulman C., Malaspina D., Lubin G., et al. Advancing paternal age and autism. Arch Gen Psychiatry 2006; 63: 1026–1032
- Roca J., Mezquita C. DNA topoisomerase II activity in nonreplicating, transcriptionally inactive, chicken late spermatids. Embo J 1989; 8: 1855–1860
- Roest H., van Klaveren J., de Wit J., van Gurp C., Koken M., Vermey M., van Roijen J., Hoogerbrugge J., Vreeburg J., Baarends W., et al. Inactivation of the HR6B ubiquitin-conjugating DNA repair enzyme in mice causes male sterility associated with chromatin modification. Cell 1996; 86: 799–810
- Rybaczek D., Bodys A., Maszewski J. H2AX foci in late S/G2- and M-phase cells after hydroxyurea- and aphidicolin-induced DNA replication stress in Vicia. Histochem Cell Biol 2007; 128: 227–241
- Sakkas D., Mariethoz E., Manicardi G., Bizzaro D., Bianchi P. G., Bianchi U. Origin of DNA damage in ejaculated human spermatozoa. Rev Reprod 1999; 4: 31–37
- Sakkas D., Moffatt O., Manicardi G. C., Mariethoz E., Tarozzi N., Bizzaro D. Nature of DNA damage in ejaculated human spermatozoa and the possible involvement of apoptosis. Biol Reprod 2002; 66: 1061–1067
- Schmid T., Eskenazi B., Baumgartner A., Marchetti F., Young S., Weldon R., Anderson D., Wyrobek A. The effects of male age on sperm DNA damage in healthy non-smokers. Hum Reprod 2006; 22: 180–187
- Shirley C., Hayashi S., Mounsey S., Yanagimachi R., Meistrich M. Abnormalities and reduced reproductive potential of sperm from Tnp1- and Tnp2-null double mutant mice. Biol Reprod 2004; 71: 1220–1229
- Sonnack V., Failing K., Bergmann M., Steger K. Expression of hyperacetylated histone H4 during normal and impaired human spermatogenesis. Andrologia 2002; 34: 384–390
- Sotolongo B., Lino E., Ward W. Ability of hamster spermatozoa to digest their own DNA. Biol Reprod 2003; 69: 2029–2035
- Tesarik J., Greco E., Mendoza C. Late, but not early, paternal effect on human embryo development is related to sperm DNA fragmentation. Hum Reprod 2004; 19: 611–615
- Tesarik J., Mendoza-Tesarik R., Mendoza C. Sperm nuclear DNA damage: update on the mechanism, diagnosis and treatment. Reprod Biomed Online 2006; 12: 715–721
- Toshimori K., Ito C., Maekawa M., Toyama Y., Suzuki-Toyota F., Saxena D. Impairment of spermatogenesis leading to infertility. Anatomical science international/Japanese Association of Anatomists 2004; 79: 101–111
- van der Heijden G., Derijck A., Ramos L., Giele M., van der Vlag J., de Boer P. Transmission of modified nucleosomes from the mouse male germline to the zygote and subsequent remodeling of paternal chromatin. Dev Biol 2006; 298: 458–469
- Virro M., Larson-Cook K., Evenson D. Sperm chromatin structure assay (SCSA) parameters are related to fertilization, blastocyst development, and ongoing pregnancy in in vitro fertilization and intracytoplasmic sperm injection cycles. Fertil Steril 2004; 81: 1289–1295
- von Deutsch A., Mitchell C., Williams C., Dutt K., Silvestrov N., Klement B., Abukhalaf I., von Deutsch D. Polyamines protect against radiation-induced oxidative stress. Gravitational and space biology bulletin: publication of the American Society for Gravitational and Space Biology 2005; 18: 109–110
- Weng S., Taylor S., Morshedi M., Schuffner A., Duran E., Beebe S., Oehninger S. Caspase activity and apoptotic markers in ejaculated human sperm. Mol Hum Reprod 2002; 8: 984–991
- Wu J. Y., Ribar T. J., Cummings D. E., Burton K. A., McKnight G. S., Means A. R. Spermiogenesis and exchange of basic nuclear proteins are impaired in male germ cells lacking Camk4. Nat Genet 2000; 25: 448–452
- Wykes S., Krawetz S. The structural organization of sperm chromatin. J Biol Chem 2003; 278: 29471–29477
- Wyrobek A. J., Eskenazi B., Young S., Arnheim N., Tiemann-Boege I., Jabs E. W., Glaser R. L., Pearson F. S., Evenson D. Advancing age has differential effects on DNA damage, chromatin integrity, gene mutations, and aneuploidies in sperm. Proc Natl Acad Sci USA 2006; 103: 9601–9606
- Zhao M., Shirley C., Hayashi S., Marcon L., Mohapatra B., Suganuma R., Behringer R., Boissonneault G., Yanagimachi R., Meistrich M. Transition nuclear proteins are required for normal chromatin condensation and functional sperm development. Genesis 2004; 38: 200–213