Abstract
This study was designed to determine whether HCO3− and Cl− are required for the activation of the GABAA receptor/Cl− channel (GBRC) by GABA and the subsequent capacitation of rat sperm. Spermatozoa from adult Sprague Dawley rats were incubated in four different media: modified complete rat fertilization medium (mRFM), Cl−-deficient (Cl−-DF) mRFM, HCO3−-DF mRFM, and Cl−-DF HCO3−-DF mRFM, with or without GBRC agonists (GABA and progesterone) or GBRC antagonists (bicuculline and picrotoxin) for 0–6 h under capacitating conditions. Sperm capacitation and hyperactivation were assessed by chlortetracycline staining and computer-assisted sperm analysis, respectively. The results showed that GABA added to the mRFM accelerated capacitation and hyperactivation, followed by increase in the acrosome reaction, reaching maximum value after 5 h. Progesterone also accelerated sperm capacitation and hyperactivation. Bicuculline and picrotoxin, antagonists of GABA, blocked the effects of both GABA and progesterone acceleration of sperm capacitation and hyperactivation. Sperm capacitation required both Cl− and HCO3−. These results indicate that activation of GBRC may contribute to sperm capacitation and hyperactivation, and that both HCO3− and Cl− are essential. This is the first report of a close relationship between HCO3−/Cl− transport and the activation of GBRC in rat sperm capacitation and hyperactivation.
Abbreviations | ||
GBRC | = | GABAA receptor/Cl-channel |
mRFM | = | modified rat fertilization medium |
Cl−-DF | = | Cl−-deficient |
CTC | = | chlortetracycline |
CASA | = | computer-assisted sperm analysis |
Introduction
Mammalian spermatozoa must undergo a period of physiological preparation in the female reproductive tract in order to acquire the ability to fertilize an egg [Austin Citation1952; Chang Citation1951]. The changes that confer fertilizing ability are known as capacitation. Only capacitated spermatozoa are able to undergo either the spontaneous or induced acrosome reaction [Florman and Babcock Citation1991; Yanagimachi 1994; Jaiswal and Eisenbach Citation2002]. Capacitation is reversible, but the acrosome reaction is not [Yanagimachi 1994].
The two main anions in sperm capacitation media are HCO3− and Cl−. The optimal concentration of HCO3− varies from species to species but it plays a central role in sperm capacitation [Lee and Storey Citation1986; Neill and Olds-Clarke Citation1987; Shi and Roldan Citation1995a; Visconti et al. Citation1995; Citation1998; Citation2002; Wang et al. Citation2003]. Recent studies have shown that bicarbonate directly stimulates the soluble adenylyl cyclase (sAC) activity of spermatozoa. This elevates intracellular pH, increasing sperm intracellular cAMP, and activating protein kinase A. In turn this initiates a downstream signal cascade that enhances tyrosine phosphorylation. Increased tyrosine phosphorylation is considered to be an indication that spermatozoa are undergoing capacitation [Chen et al. Citation2000; Visconti et al. Citation1995; Citation1998; Citation2002]. However, it is not clear whether activation of GABAA receptor/Cl-channel (GBRC) on the rat sperm by GABA and subsequent capacitation and hyperactivated motility involve extracellular HCO3−.
In addition, it is known that Cl− and its channels play important roles in the maintenance of sperm cellular volume [Yeung et al. Citation2004], intracellular pH [Zeng et al. Citation1996], resting membrane potential and excitability [Chan et al. Citation1997; Zhang and Shi Citation1998]. For example, the sperm cystic fibrosis transmembrane conductance regulator (CFTR) is a cAMP-activated Cl− channel which is involved in the transport of HCO3− that is important for sperm capacitation and fertilization [Hernandez-Gonzalez et al. Citation2007; Xu et al. Citation2007]. In addition, Cl− has been reported to be required for the progesterone-, GABA- and zona pellucid-initiated acrosome reaction in mouse, porcine, hamster and human spermatozoa [Espinosa et al. Citation1998; Melendrez and Meizel Citation1995; Shi et al. Citation1997; Wistrom and Meizel Citation1993; Yashimatsu and Yanagimachi 1988]. However, it is not clear whether extracellular Cl− is required for sperm capacitation and hyperactivated motility.
In vitro studies demonstrated that GABA induces the acrosome reaction in human, mouse and rat sperm [Wistrom and Meizel Citation1993; Roldan et al. Citation1994; Shi and Roldan Citation1995b; 1997; Hu et al. Citation2002a; Meizel Citation2004]. These studies suggested that the GABAA receptor/Cl− channel and a receptor linked perhaps to a Ca2+ channel [Calogero et al. Citation1999; Roldan et al. Citation1994; Shi and Roldan Citation1995b; Turner et al. Citation1994] or progesterone receptor [Falkenstein et al. Citation1999] participated. The ability of GABA or progesterone to induce the acrosome reaction was abolished in the presence of the GBRC antagonists, bicuculline and picrotoxin [Shi et al. Citation1997; Calogero et al. Citation1999; Zhang et al. Citation2000]. Together these studies suggest that GABA or progesterone interacted with the GBRC to induce the acrosome reaction and functional integrity of the chloride channel is vital for this effect [Calogero et al. Citation1999].
On the basis of in vitro studies, sperm GBRC may also be involved in two other important fertilization events, capacitation and hyperactivated motility. It has been shown that GABA increases capacitation in guinea pig and ejaculated human sperm and ram sperm [De las Heras et al. Citation1997; Zhang et al. Citation2000]. Moreover, GABA also increases hyperactivated motility in ejaculated human spermatozoa [Calogero et al. Citation1996; Ritta et al. Citation1998; Zhang et al. Citation2000]. Importantly, in some cases stimulation by a GABAA receptor agonist or inhibition by a GABAA receptor antagonist was also observed [Calogero et al. Citation1996; Ritta et al. Citation1998; Zhang et al. Citation2000]. As discussed above, progesterone also increase capacitation and hyperactivated motility of ejaculated human and porcine sperm, but data linking the effect to the GBRCs have not been reported [Meizel Citation2004].
The rat oviduct contains more than twice as much GABA as the whole brain [Erdo et al. Citation1982; Celotti et al. Citation1986]. Extremely high levels of GABA are concentrated in the oviduct mucosa that exceed by ten-fold, those in brain [Murashima and Kato Citation1986]. GABA acts as an inhibitory neurotransmitter when it binds to GBRCs in central and peripheral nervous system postsynaptic neurons [Kaila Citation1994]. There is abundant evidence supporting a GABAergic system in the female genital tract that provides a physiological environment for sperm transport. Perhaps, GABA may be a modulator for rat sperm function. The GBRC is a plasma membrane multi-subunit receptor complex linked to the chloride channel. Its activation results in channel opening, leading to a transmembrane flux of chloride ions and consequent membrane hyperpolarization or depolarization, depending on the cell types [Calogero et al. Citation1999; Ashcroft Citation2000; Sieghart Citation2006]. The presence of GBRC on porcine and ram sperm membranes has been demonstrated using radioligand assays [Erdo and Wekerle Citation1990; Ritta et al. Citation1998]. GABAA receptor subunits have been identified by immunocytochemical staining on the equatorial segment of the human sperm head [Ewert et al. Citation1990; Wistrom and Meizel Citation1993] and on the ventral surface of the acrosome of rat sperm [Hu et al. Citation2002b; Kanbara et al. Citation2005]. The specific subunits of GBRC with molecular weight approximate 53 KDa and 75 KDa were identified by Western blot analysis [Ewert et al. Citation1990; Wistrom and Meizel Citation1993; Hu et al. Citation2002b]. These studies provide evidence for the presence of this receptor in sperm, suggesting that there may be a link between the GABA receptor and the anion channel [Sieghart Citation2006]. As both HCO3− and Cl− are permeable in nerve cell GBRC [Isomura et al. Citation1994; Staley and Proctor Citation1999], we hypothesized that GBRC may be involved in GABA- and progesterone-induced sperm capacitation and hyperactivated motility through HCO3− and Cl− transport. This study was conducted to test this hypothesis.
Results
Patterns of CTC Staining and the Time Course of Sperm Capacitation
As shown in , different CTC staining patterns were observed that can be resolved into three sequential stages. The first stage was marked by the CTC fluorescence pattern, designated as the “F” pattern and characteristic of uncapacitated, acrosome-intact spermatozoa. The second stage was marked by the CTC fluorescence designated as the “B” pattern, characteristic of capacitated, acrosome-intact spermatozoa with the fluorescence-free band in the postacrosomal region. The third and final stage, designated the “AR” pattern, was characteristic of capacitated sperm, with fluorescence only over the thin band in the anterior region of the head. This corresponded to spermatozoa that have undergone the acrosome reaction.
Figure 1 Chlortetracycline staining patterns in rat spermatozoa during capacitation. This figure shows the three patterns of staining seen in rat spermatozoa after incubation in mRFM for 5 h (see Materials & Methods): the “F” pattern, characteristic of noncapacitated, acrosome-intact spermatozoa; the “B” pattern, characteristic of capacitated, acrosome-intact spermatozoa with null staining in the postacrosome area; and the “AR” pattern, characteristic of capacitated acrosome reaction spermatozoa.
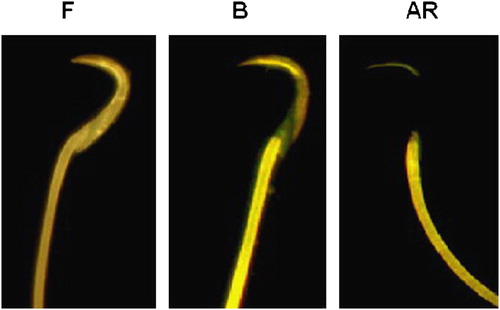
To ascertain the time course of sperm capacitation in rat, 5 μM GABA was added to sperm suspension and incubated for 0–6 hrs in the complete modified rat fertilization medium (mRFM) under 5% CO2 in air at 37°C. The sperm suspensions were then washed by centrifugation to remove GABA at different time points and a 50 μl aliquot was stained by chlortetracycline (CTC). As shown in , a2 sperm suspension incubated with GABA for 6 hrs resulted in a significant decrease in the mean percentage of F pattern spermatozoa (from 87.2±3.7% down to 31.6±4.1%, P < 0.01) and an increase both in the percentage of B pattern spermatozoa (from 6.5±0.2% up to 32.4±1.5%, P < 0.01) and AR pattern spermatozoa (from 6.3±0.2% up to 36.0±2.1%). After 6 hrs of incubation in the spermatozoa following GABA-free treated (), the proportions of B pattern spermatozoa (from 1.1±0.6% up to 30.2±2.4%) and the AR pattern spermatozoa (from 0.2±0.1% up to 18.0±2.5%) also increased. However, there is no difference in the B pattern spermatozoa when GABA-treated () and GABA-free treated were compared. However, the percent of the AR pattern spermatozoa in GABA-treated () is highly significant when compared to that of the GABA-free treated spermatozoa (, P < 0.01). These results suggest that GABA promotes F pattern to B pattern transition, followed by an increase in the AR pattern spermatozoa. The proportion of spermatozoa that were capable of undergoing capacitation in response to GABA increased as pre-incubation was extended. The induced response reached a maximum value when pre-incubated for 5 h (B+AR patterns: 72.2±2.9%) and then decreased slightly (68.4±1.8%) at 6 h incubation. For this reason, subsequent experiments were carried out by pre-incubating spermatozoa for 5 h. In the control, spermatozoa can also be capable of undergoing spontaneous capacitation and the proportions of spermatozoa showing B and AR patterns were lower (B+AR patterns: 49.2±2.5%, ) indicative of spontaneous capacitation as compared to GABA treated induced capacitation (72.2±2.9%, P < 0.01 ).
Figure 2 Initiation of capacitation in rat spermatozoa by GABA. Sperm suspensions prepared in mRFM were incubated with 5 μM GABA (A) or GABA's solvent as control (B) in mRFM at 37°C under 5% CO2 in air for 0–6 hours, washed by centrifugation and assessed using chlortetracycline staining. The data are mean±SEM of five different experiments. Different superscripts denote significant differences between varied time points for each pattern, at the same time-points, a vs. a*, P < 0.05; vs. b*, P < 0.05; c vs. c*, P < 0.01.
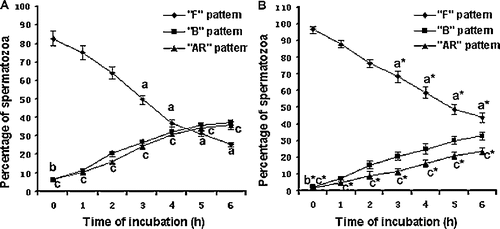
Effects of GBRC Agonists and Antagonists on Sperm Capacitation and Hyperactivation
To examine the effects of GBRC agonists and antagonists on sperm capacitation and hyperactivated motility, 5 μM GABA and 5 μM progesterone or 10 μM bicuculline and 100 μM picrotoxin were added to different aliquots of the sperm suspension prepared in complete mFRM. PBS or 0.1% DMSO solvent for progesterone, bicuculline and picrotoxin (this final concentration does not affect sperm viability and motility), provided a negative control. After incubation for 5 h, the spermatozoa were washed and then evaluated for capacitation and motility. As a result of study, GABA and progesterone facilitates capacitation () and hyperactivated motility () in rat spermatozoa as compared to controls (P < 0.05), whereas, bicuculline and picrotoxin both GBRC antagonists, block sperm capacitation () and hyperactivated motility () as compared to agonist treated, (P < 0.05) suggesting that GABA- and progesterone-initiated rat sperm capacitation and hyperactivation were mediated by activation of the GBRC. GABA is able to modulate sperm kinematic parameters in rat spermatozoa. GABA and progesterone increased significantly sperm curvilinear velocity (VCL) and amplitude of lateral displacement (ALH) and decreased LIN (which is derived from the ratio of VSL/VCL multiplied by 100, ) as compared to the controls (P < 0.05). GBRC antagonists, bicuculline and picrotoxin also inhibit hyperactivated motility initiated by GABA and progesterone as compared to GABA and progesterone alone, (P < 0.05). This suggests that bicuculline or picrotoxin function through antagonizing the GABA and progesterone binding site on the GABAA receptors to suppress the capacitation and hyperactivated motility. This may reflect its ability to block the Cl− channels. GABAA receptors have been shown to open and close Cl− channels when activated by GABA and competitively inhibited by bicuculline and non-competitively inhibited by picrotoxin.
Figure 3 Effect of GABA, progesterone, bicuculline and picrotoxin on capacitation in rat spermatozoa. Sperm suspensions were incubated for 5 h in mRFM with GABA (G, 5 μM) or progesterone (P, 5 μM), and bicuculline (Bic, 10 μM) or picrotoxin (Pic, 100 μM) or without agonists and antagonists of GABA as control (CTL). Suspensions were then washed by centrifugation, resuspended in fresh mRFM and stained with chlortetracycline to evaluate capacitation. The data are mean±SEM of five different experiments. As a, b and c compared separately to control (CTL), P < 0.01. As a*, b* and c* compared to a, b and c, P < 0.01, respectively; As Bic and pic (a**, b**and c**) compared with control, P < 0.05; As bic/p and pic/p compared to bic and pic, P ≤ 0.05.
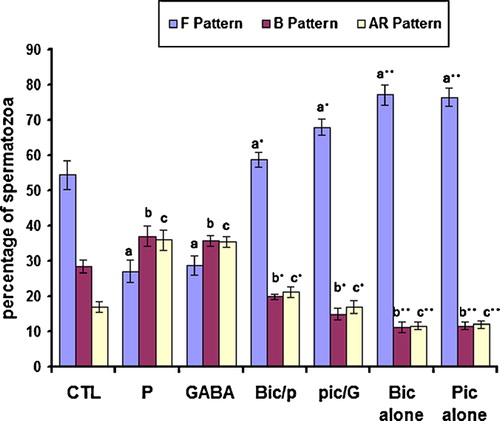
Effect of GABA, Progesterone, Bicuculline and Picrotoxin on Motion Parameters of Rat Spermatozoa in mRFM
Effect of GABA, Progesterone, Bicuculline and Picrotoxin on Motion Parameters of Rat Spermatozoa in the Four Different mRFM Media
Effects of Extracellular Cl− and HCO3− on Sperm Capacitation and Hyperactivation
To ascertain whether extracellular Cl− and HCO3− affect sperm capacitation and hyperactivation, spermatozoa were separately incubated in Cl−-DF- or HCO3− DF-mRFM for 5 hrs under capacitation conditions. Sperm capacitation was significantly reduced when the spermatozoa were exposed to the Cl− DF-mRFM ( blue bars), as compared to the complete mRFM (; P < 0.01). Hyperactivated motility, i.e., CL and ALH were greatly attenuated compared to spermatozoa in the complete mRFM. This shows that sperm capacitation and hyperactivation require extracellular Cl−. Similarly, bicuculline and picrotoxin blocked sperm capacitation () and hyperactivated motility () in the Cl−-DF mRFM. Interestingly, sperm capacitation was restored after incubation for 2 h in the complete mRFM substituted for Cl−-DF mRFM (the sum of B patterns increases from 12.3±1.7% up to 32.0±3.7%). This further supports that sperm capacitation and hyperactivation require extracellular Cl−.
Figure 4 Effect of GABA, progesterone, bicuculline and picrotoxin on capacitation of rat sperm in Cl−-DF mRFM. Sperm suspensions were incubated for 5 h in Cl−-DF mRFM with or without GABA (G, 5 μM), progesterone (P, 5 μM), bicuculline (Bic, 10 μM) and picrotoxin (Pic, 100 μM). Control (CTL) suspension was treated with solvent only. Suspensions were washed by centrifugation and then stained with CTC to evaluate capacitation. The data are mean±SEM of five different experiments. As P and G compared to control (CTL), P < 0.05. As bic/P and pic/G compared to either P/G or control, P > 0.05; As both Bic and Pic compared to either Bic/P or Pic/G, P < 0.05.
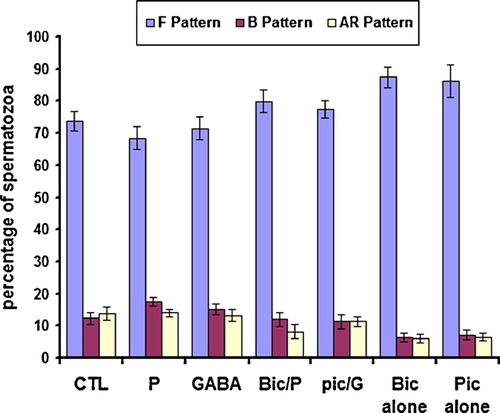
Modified Compositions of the Capacitation Media for Rat Sperm (mRFM)
GABA and progesterone failed to initiate rat sperm capacitation (, the sum of “B+AR” was 10.6±2.3%–13.3±2.5%) and hyperactivation () in the HCO3−-DF mRFM. There was no significant difference in sperm capacitation between the varied treatments (). In contrast hyperactivated motility was absent when sperm were incubated in HCO3−-DF mRFM (), indicating that extracellular HCO3− is necessary for activation of GBRC by GABA and progesterone. However, sperm capacitation was almost restored (the percentage of B pattern increased from 6.9±1.5% up to 29.3±3.7%) when 15 mM NaHCO3 was added to the HCO3−-DF mRFM after a 2 h incubation. However, when spermatozoa were re-suspended in medium lacking both Cl− and HCO3−, neither GABA and progesterone agonists nor bicuculline and picrotoxin antagonists elicited any response to capacitation () and hyperactivation. Therefore, activation of GBRC by GABA and progesterone and subsequent initiation of sperm capacitation and hyperactivation requires HCO3− and Cl−. At this stage sperm viability was 76%–82% which did not fluctuate in the various media ().
Figure 5 Effect of GABA, progesterone, bicuculline and picrotoxin on capacitation of rat spem in HCO3−-DF mRFM Sperm suspensions were incubated for 5 h in HCO3−-DF mRFM with or without GABA (G, 5 μM), progesterone (P, 5 μM), bicuculline (10 μM) and picrotoxin (Pic, 100 μM ). Control (CTL) suspension was treated with solvent only. Sperm suspensions were washed by centrifugation and then stained with CTC to evaluated capacitation. The data are mean±SEM of four different experiments. There is nostatistical significance between various treatments and the control, P > 0.05.
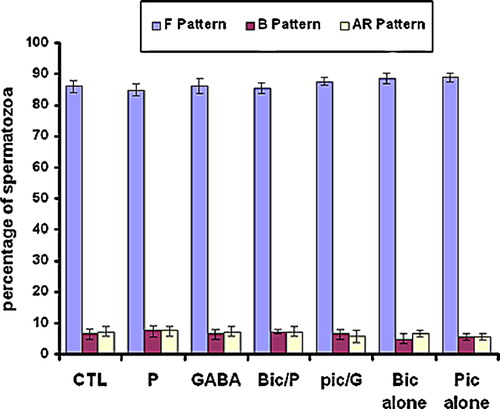
Discussion
In this study, we observed that rat sperm are present in the B pattern during capacitation. This is a characteristic of capacitated sperm in agreement with the results described by Oberlander et al. [Citation1996] and Hu et al. [Citation2002a]. Additionally they proposed that an intermediate pattern would reflect differences between capacitated sperm and uncapacitated as well as acrosome-reacted sperm.
It has been emphasized that capacitation should include a consideration of events occurring both in the head (i.e., the acrosome reaction) and in the tail (i.e., motility changes) [Yanagimachi 1994; Barboni et al. Citation1995; DasGupta et al. Citation1994; Visconti et al. Citation1998; Citation2002]. Only capacitated spermatozoa are able to undergo the acrosome reaction in response to natural inducers ZP, progesterone or GABA [Roldan et al. Citation1994; Shi and Roldan Citation1995b; Shi et al. Citation1997; Yanagimachi 1994]. This has been considered to be indicative of the completion of capacitation [Florman and Babcock Citation1991; Jaiswal and Eisenbach Citation2002; Visconti et al., Citation1998; Yanagimachi 1994]. Therefore, the sum of “B+AR” patterns of spermatozoa during incubation that undergo capacitation is generally taken as an indicator for completion of capacitation [Barboni et al. Citation1995; DasGupta et al. Citation1994; Visconti et al. Citation1998; Wang et al. Citation2003; Xu et al. Citation2007]. Although there was no significant difference (P > 0.05) observed in the B pattern between GABA-treated () and GABA-free treated () spermatozoa, the percentage of the acrosome reacted spermatozoa of the former was significantly higher than that of the latter (P < 0.01). In other words, the number of capacitated cells (“B+AR” patterns) in GABA-treated media were significantly greater than that of GABA-free media.
This study shows that the response to GABA and progesterone may involve activation of GBRC leading to increased rat sperm capacitation and hyperactivation. However, these responses were significantly inhibited by GBRC antagonists, bicuculline and picrotoxin. We also demonstrated that the responses initiated by GABA and progesterone are required for HCO3− and Cl− extracellular transport. GBRC will not be activated if either Cl− or HCO3− or both are deficient in mRFM. Capacitation and hyperactivation do not take place if HCO3− and Cl− conductance is prevented. The mechanism of their conductance through GBRC to induce sperm capacitation and hyperactivation remains to be defined.
In many species capacitated spermatozoa display hyperactivation, of which the main characteristic is increased ALH and VCL but decreased LIN. In vivo, this type of movement occurs in the oviduct, enhancing the capacity of sperm to penetrate viscoelastic media such as the cumulus and matrix complex that surrounds the ovum [Suarez and Dai 1988]. Rat sperm express this pattern at the site of fertilization, the oviductal ampulla [Shalgi and Phillips Citation1988].
Interestingly, hyeractivation is not expressed in vitro [Oberlander et al. Citation1996; Yeung et al. Citation1995]. In this study, rat sperm incubated in mRFM medium for 5 h under capacitating conditions displayed hyperactivation, in agreement with that reported by Cancel et al. [Citation2000]. As discussed above, the concentration of GABA in the oviduct in the rat [Murashima and Kato Citation1986] and the levels of progesterone in the cumulus matrix in hamster [Yuan et al. Citation1998; Meizel Citation2004] are higher than that of other body fluid suggesting that rat spermatozoa can be hyperactivated in vitro. CASA was performed to capture sperm at 60 Hz on digital one second tracks. We used a modified rat fertilization medium of which there was an increased concentration of sodium, but decreased concentration of potassium (see ) as compared with the medium used by Oberlander et al. [Citation1996] and Shalgi [Citation1991]. It has been shown that Na+, K+, Ca+, HCO3−, BSA and energy substrates are important for hyperactivated sperm motility [Burkman et al. Citation1984; Fraser Citation1983; Hyne et al. Citation1985; Suarez Citation1996; Yanagimachi 1994]. The reason for the apparent conflict may reflect, at least in part, the use of different sperm capacitating media and the fact that different studies measured hyperactivation by different techniques which measure different stages of capacitation.
It is well established that HCO3− plays a pivotal role in sperm capacitation. However, how HCO3− regulates sperm capacitation is not well understood. In this study, our results showed that extracellular HCO3− is required for sperm capacitation and hyperactivation. This is consistent with the results obtained in earlier studies using spermatozoa from various species [Lee and Storey Citation1986; Shi and Roldan Citation1995a; Visconti et al. Citation1995; Citation2002]. Our previous studies have demonstrated that the involvement of CFTR in uterine bicarbonate secretion and the fertilizing capacity of sperm were important [Wang et al. Citation2003]. Previously, some studies proposed that a crucial role of the CFTR is to conduct both Cl− and HCO3− [Poulsen et al. Citation1994; Reddy and Quinton Citation2001]. The Cl− ion and its channel, and transportation during sperm capacitation provoked us to investigate this issue.
Recently we showed that CFTR is vital to sperm fertilizing capacity and male fertility [Xu et al. Citation2007]. Disruption of CFTR function in sperm by inhibitors or antibodies was shown to reduce the sperm capacitation, as well as to affect a number of capacitation-associated HCO3− dependent events. In addition, Hernandez-Gonzalez et al. [Citation2007] have also shown that CFTR is present in sperm and necessary for capacitation. They further showed capacitation is accompanied by CFTR activation and epithelial Na+ channel closure resulting in an intracellular loading of Cl−. Hence they suggested that HCO3− can enter sperm coupled to Cl− loading. However, it is not clear whether CFTR is directly conducting HCO3− or working in parallel with a Cl−/HCO3− exchanger. In HCO3−-DF medium, neither GBRC agonists nor antagonists were able to elicit the response seen in complete mRFM. Moreover, sperm capacitation was just about restored by adding back 15 mM HCO3− to HCO3−-DF mRFM. This indicates that HCO3− transport is closely associated with GBRC because the effects of GBRC agonists and antagonists on sperm capacitation and hyperactivation depend on the presence of HCO3− in the medium. It has been shown that HCO3− directly activates a bicarbonate sensitive soluble adenylyl cyclase which in turn triggers the downstream signal transduction cascade leading to capacitation [Chen et al. Citation2000; Cann Citation2004; Chan et al. Citation2006]. Activation of GBRC not only depends on the stimulation of GABA or progesterone, but also relies upon the conductance of HCO3− and Cl−. These results further support that HCO3− and Cl− are required for both sperm capacitation and hyperactivation for spontaneous initiation in the capacitating medium. This is the first demonstration that there is a link between HCO3− conductance and activation of GBRC involvement in the events of sperm capacitation and hyperactivation.
Little is known about how the GBRC regulates sperm capacitation and hyperactivation. In neurons, the Cl− channel and the GABAA receptor co-exist as a GBRC complex which acts as an independent functional unit and the GABAA receptor activation in postsynaptic neurons results in a Cl− influx leading to membrane hyperpolarization and inhibitory neurotransmission [Kaila Citation1994; Ashcroft Citation2000]. It can also be excitatory in presynaptic nerve terminals and other somatic cells. Activation of GABAA receptors can stimulate the exocytosis by causing membrane depolarization and thereby opening voltage-activated Ca2+ channels [Fassio et al. Citation1999; Williams et al. Citation2000; Hoger et al. Citation2008]. The roles for GABA in capacitation and hyperactivation has been demonstrated in vitro. As mentioned above, high concentrations of GABA are found in the oviductal fluids. It is feasible that spermatozoa in the oviduct are exposed to high levels of GABA and thus spermatozoa undergo or complete capacitation together with hyperactivated motility. This suggests that GABA may be a modulator of sperm capacitation and hyperactivated motility in vivo. These findings may be a clue to elucidating the functional mechanism of the sperm GBRC in vitro.
It has been shown that GABA increases sperm capacitation and hyperactivated motility in ram [De las Heras et al. Citation1997], human [Calogero et al. Citation1996; Ritta et al. Citation1998; Zhang et al. Citation2000] and guinea pig [Zhang et al. Citation2000]. Importantly, some of these studies also ascertained activation by a GBRC agonist or inhibition of the GABA effects by a GBRC antagonist [Calogero et al. Citation1996; Ritta et al. Citation1998; Zhang et al. Citation2000]. Progesterone also increases capacitation and hyperactivation in animal and human sperm as mentioned above, but experiments linking the effect to the GBRC were not identified [Uhler et al. Citation1992; DasGupta et al. Citation1994; Barboni et al. Citation1995; Meizel Citation2004]. In this study, we clearly demonstrated that the activation of GBRC in sperm by GABA and progesterone could initiate rat sperm capacitation and hyperactivation. These effects were attenuated by GBRC antagonists, bicuculline and picrotoxine. Moreover, we further demonstrated that stimulation or inhibition of sperm capacitation and hyperactivation by GBRC agonists or antagonists did not occur in the Cl−-DF mRFM or HCO3−-DF mRFM or media deficient in both Cl− and HCO3. These results support the view that the activation of GBRC induced rat sperm capacitation and hyperactivation by GABA- or progesterone require Cl− and HCO3−. Further they suggest that Cl− and HCO3− transport are important for GABA- or progesterone- initiated capacitation and hyperactivated motility. To our knowledge, this is the first report that there is a close relationship between Cl− and HCO3− transport and activation of GBRC in two important fertilization related events, sperm capacitation and hyperactivation. These results suggest that this neurotransmitter may be regarded as an important modulator of sperm function.
In conclusion, we provide evidence that Cl− and HCO3− are essential for inducing rat sperm capacitation and hyperactivated motility by GABA or progesterone through GABAA receptor/Cl− channel.
Materials and Methods
Reagents
Sodium-gluconate, potassium-gluconate, calcium gluconate and GABA, progesterone, bicuculline, picrotoxin, chlortetracycline (CTC), HEPES, Hoechst 33258, Tris-base, L- cysteine, BSA (Fraction V), sodium pyruvate, sodium L-lactate and paraformaldehyde were obtained from Sigma (St Louis, MO, USA). DMSO was purchased from E. Merck. PercollTm was obtained from GE Healthcare Bio-Sciences AB (Uppsala, Sweden).
Media
The capacitation medium used in this study was a modified rat fertilization medium (mRFM) from that of Shalgi [Citation1991] and Oberlander et al. [Citation1996] (see ). Briefly, the complete mRFM:NaCl was increased from 70.32 mM up to 95.32 mM and KCl was decreased from 25 mM to 8.08 mM, respectively, and 25 mM HEPES was descended in the original medium to 10 mM Hepes. In the medium designated as HCO3−-DF mRFM, 25 mM NaHCO3 was replaced by 30 mM gluconate and in the medium designated as Cl−-DF mRFM, the relevant D-gluconate salt was used which substituted for all Cl− in the original mRFM (26 μM extracellular Cl− was detected by Cl− sensitive-electrode of Roche Inc. USA; N=3). The three media above had a final osmolality of 305–310 mOsmol/kg and a pH of 7.5 at 25°C. PH in HCO3−-DF medium was maintained with NaOH-Hepes buffer under 5% CO2 in air (for more detail, see footnote in ).
Collection and Preparation of Spermatozoa
Three-month old Sprague Dawley rats (body weight 380±20g) were purchased from the Centers for Experiment Animal of Zhejiang Province and for Chinese University of Hong Kong. All animal procedures were conducted in accordance with the Experimental Animal Act by the Local Ethics Committee Approval of Zhejiang Province [1996] and the Chinese University of Hong Kong. Animals were sacrificed by CO2 asphyxiation followed by cervical dislocation. The caudal epididymis and vasa deferentia were incised, and their contents were released into 3 ml Cl−-DF and HCO3−-DF mRFM. Spermatozoa were separated by two layer Percoll (50%–75%) density gradient centrifugation for 15 min at 600×g to eliminate dead sperm and other cells, the pellets were washed by centrifugation in the same medium, then resuspended in fresh mRFM, Cl−-DF mRFM, HCO3−-DF mRFM, as well as Cl−-DF mRFM and HCO3−-DF mRFM, respectively. Spermatozoa were adjusted to a final concentration of 20–30×106 cells/ml, and then incubated with or without desire reagents in polythene tubes for 6 h under 5% CO2 in air at 37°C. At this stage 80%–85% viable cells were found.
Evaluation of Capacitation by CTC Staining
For the assessment of capacitation, spermatozoa were stained with a vital stain to discriminate between the live and dead cells. Sperm were stained with Hoechst 33258 solution for 2 min in the dark at 37°C (final concentration of Hoechst 1 μg/ml). Only samples with motility >80%, viability >85% were used in the study. Sperm were washed and resuspended in fresh mRFM. For the assessment of the capacitation status of the rat spermatozoa, CTC staining was carried out as described previously by Oberlander et al. [Citation1996] and Hu et al. [Citation2002a]. Following CTC staining, samples were immediately examined at ×1000 by using phase-contrast and fluorescence microscopy. An excitation beam passed through a 380 nm and DM 420 nm fluorescence emission (Nikon 80i, Tokyo, Japan) was used in each sample, a total of 200 cells were assessed by two individuals. The degree of capacitation was evaluated by the incidence including B and AR patterns by the spontaneous or inducer with GABA or progesterone.
Assessment of Sperm Motility by CASA
Sperm motility and viability at this stage were approximately 80–85% as estimated using a trypan blue exclusion test and phase contrast microscopy after incubation of 5 h. Hamilton Thorne Research Motility Analyzer (HTM-IVOS, with software Version 10.8, Beverly, MA, USA) was used to record the sperm hyperactivated motility and motion parameters by computer-assisted sperm analysis (CASA), performed as described previously by Oberlander et al. [Citation1996] and Cancel et al. [Citation2000]. At the end of the incubation, a 20 μl aliquot of the sperm suspension was placed onto a pre-warmed microcell slide (37°C), with a 22 mm×22 mm coverslip and a chamber depth of 80 μm. For each sample, 10 randomly-selected fields containing 300 tracks were examined. Tracks were recalled later for analysis at 60 Hz. Curvilinear velocity (VCL), straight-line velocity (VSL), average-path velocity (VAP), amplitude of lateral head displacement (ALH), linearity% (LIN, VSL/VCL multiplied by 100) and percentage of motility (motile) were recorded. A spermatozoon was designated as being hyperactive if it had a VCL≥105 μm/s and ALH≥25 μm/s.
Statistical Analyses
Statistical analyses were carried out using the SPSS 13.0 software. Results are expressed as mean±SEM. All the percentages are transformed into arcsin . To compare the different time-points during treatment and recovery phases, the one-way ANOVA test was applied. The differential impact of sperm hyperactivation, capacitation and the acrosome reactions within different treatment groups was calculated using a multiple regression analysis.
Acknowledgments
This work was supported by National Natural Sciences Foundation of China Grants (30270531 and 30600217); National 973 project of China (2006CB504002), WJK (2005204701), Medical Science Research Fund of Zhejiang Province (2006B002) and Li Ka Shing Institute of Health Sciences of the Chinese University of Hong Kong.
Declaration of Interest: The authors report no conflicts of interest. The authors alone are responsible for the content and writing of the paper.
Notes
J-Y Jin and C X Zhou contributed equally to this work.
References
- Ashcroft, F. M. Ion Channels and Disease. Acadermic Press, LondonUK 2000
- Austin C. R. The “capacitation” of the mammalian sperm. Nature 1952; 170: 326
- Barboni B., Mattioli M., Seren E. Influence of progesterone on boar sperm capacitation. J Endocrinol 1995; 144: 13–18
- Burkman L. J., Overstreet J. M., Katz D. F. A possible role for potassium and pyruvate in the modulation of sperm motility in the rabbit oviductal isthmus. J Reprod Fertil 1984; 71: 367–376
- Calogero A. E., Burerello N., Ferrara E., Hall J., Fishei S., D'agata R. γ-Aminobutyric acid (GABA) A and B receptor mediate the stimulatory effects of GABA on the human sperm acrosome reaction: interaction with progesterone. Fertil Steril 1999; 71: 930–936
- Calogero A. E., Hall J., Fishel S., Green S., Hunter A., D'Agata R. Effects of gamma-aminobutyric acid on human sperm motility and hyperactivation. Mol Hum Reprod 1996; 2: 733–738
- Cancel A. M., Lobdell D., Mendola P., Perreault S. D. Objective evaluation of hyperactivated motility in rat spermatozoa using computer-assisted sperm analysis. Hum Reprod 2000; 6: 1322–1328
- Cann M. Bicarbonate stimulated adenylyl cyclases. IUBMB Life 2004; 56: 529–534
- Celotti F., Apud J. A., Rovescalli A. C., Metcangi R. C., Negri-Cesi P., Racagni G. The GABAergic extrinsic innervation of the rat fallopian tubes; biochemical evidence and endocrine modulation. Adv Biochem Psychopharmacol 1986; 42: 251–264
- Chan H. C., Shi Q. X., Zhou C. X., Wang X. F., Xu W. M., Chen W. Y., Chen A. J., Ni Y., Yuan Y. Y. Critical role of CFTR in uterine bicarbonate secretion and the fertilizing capacity of sperm. Mol Endocrinol 2006; 250: 106–113
- Chan H. C., Zhou T. S., Fu W. O., Wang W. P., Shi Y. L., Wang P. Y. D. Cation and anion channels in rat and human spermatozoa. Biochim Biophys Acta 1997; 1323: 117–129
- Chang M. C. Fertilizing capacity of spermatozoa deposited in fallopian tubes. Nature 1951; 168: 997–998
- Chen Y., Cann M. J., Litvin T. N., Iourgenko V., Sinclair M. L., Levin L. R., Buck J. Soluble adenyl cyclase as an evolutionarily conserved bicarbonate sensor. Science 2000; 289: 625–628
- DasGupta S., O'Toole C., Mills C. L., Frasser L. R. Effect of pentoxifylline and progesterone on human sperm capacitation and acrosomal exocytosis. Hum Reprod 1994; 9: 2103–2109
- De las Heras M. A., Valcarcel A., Perez L. J. In vitro capacitating effect of gamma-aminobutyric acid in ram spermatozoa. Biol Reprod 1997; 56: 964–968
- Espinosa F., De la Vega-Beltran J. L., Lopez-Gonzalez I., Delgado R., Labarca P., Darszon A. Mouse sperm patch-clamp recordings reveal single Cl− channels sensitive to niflumic acid, a blocker of the sperm acrosome reaction. FEBS Letters 1998; 426: 47–51
- Erdo S. L., Rosdy B., Szporny L. Higheer GABA concentrations in fallopian tube than in brain of the rat. J Neurochem 1982; 38: 1174–1176
- Erdo S. L., Wekerle L. GABAA type binding sites on membranes of spermatozoa. Life Sci 1990; 47: 1147–1151
- Ewert M., Shivers B. D., Luddens H., Mohler H., Seeburg P. H. Subunit selectivity and epitope characterization of mAbs directed against the GABAA/benzodiazepine receptor. J Cell Biol 1990; 110: 2043–2048
- Falkenstein E., Heck M., Gerdes D., Grube D., Christ M., Weigel M., Buddhikot M., Meizel S., Wehling M. Specific progesterone binding to a membrane protein and related nongenomic effects on Ca2+-fluxes in sperm. Endocrinol 1999; 140: 5999–6002
- Fassio A., Rossi F., Bonanno G., Raiteri M. GABA induces norepinephrine exocytosis from hippocampal noradrenergic axon terminals by a dual mechanism involving different voltage-sensitive calcium channels. J Neurosci Res 1999; 57: 324–331
- Florman, H. M. and Babcock, D. F. (1991) Progress toward understanding the molecular basis of capacitation. In: Wassarman, P. M. (Ed.), Elements of Mammalian Fertilization, vol. 1. Boca Raton: CRC Press; pp. 105–132
- Fraser L. R. Potassium ions modulate expression of mouse sperm fertilizing ability, acrosome reaction and Hyperactivated motility in vitro. J Reprod Fertil 1983; 69: 539–353
- Hernandez-Gonzalez E. O., Trevino C. L., Castellano L. E., Vega-Beltran J. L., Ocampo A. Y., Visconti P. E., Darszon A. Involvement of Cystic Fibrosis Transmembrane Conductance Regulator in mouse sperm capacitation. J. Biol. Chem 2007; 232: 24397–24406
- Hoger P. U., French A. S., Torkkeli P. H. Contributions of Voltage-and Ca2+-activated conductances to GABA-induced depolarization in spider mechanosory neurons. J Neurophysiol 2008; 99: 1596–1606
- Hu J. H., He X. B., Wu Q., Yan Y. C., Koide S. S. Biphasic effect of GABA on rat sperm acrosome reaction: involvement of GABA (A) and GABA (B) receptors. Arch Androl 2002a; 48: 369–378
- Hu J. H., He X. B., Wu Q., Yan Y. C., Koide S. S. Subunit composition and function of GABAA receptors of rat spermatozoa. Neurochem Res 2002b; 27: 195–199
- Hyne R. V., Edwards K. P., Lopata A., Smith J. D. Changes in guinea pig sperm intracellular sodium and potassium content during capacitation and treatment with monovalent ionophores. Gamete Res 1985; 12: 65–73
- Isomura Y., Sugimoto M., Fujiwara-Tsukamoto Y., Yamamoto-Muraki S., Yamada J., Fukuda A. Synaptically activated Cl− accumulation responsible for depolarizing GABAergic responses in mature hippocampal neurons. J Neurophysiol 2003; 90(4)2752–2756
- Jaiswal B. S., Eisenbach M. Capacitation. Fertilization, D. M. Hardy. Academic Press, San Diego 2002; 57–117
- Kaila K. Ionic basis of GABAA receptor channel function in the nervous system. Progress in Neurobiology 1994; 42: 489–537
- Kanbara K., Okamoto K., Nomura S., Kaneko T., Shigemoto R., Azuma H., Katsuoka Y., Watanabe M. Cellular localization of GABA and GABAB receptor subunit proteins during spermiogenesis in rat testis. J Andro 2005; 26: 485–493
- Lee M. A., Storey B. T. Bicarbonate is essential for fertilization of mouse eggs: mouse sperm require it to undergo the acrosome reaction. Biol Reprod 1986; 34: 349–356
- Meizel S. The sperm, a neuron with a tail: ‘neuronal’ receptors in mammalian sperm. Biol Rev 2004; 79: 713–732
- Melendrez C. S., Meizel S. Studies of porcine and human sperm suggesting a role for a sperm glycine receptor/Cl− channel in the zona pellucida-initiated acrosome reaction. Biol Reprod 1995; 53: 676–683
- Murashima Y. L., Kato T. Distribution of gamma-aminobutyric acid and glutamate decarboxylase in the layers of rat oviduct. J Neurochem 1986; 46: 166–172
- Neill J. M., Olds-Clarke P. A computer-assisted assay for mouse sperm hyperactivation demonstrates that bicarbonate but not bovine serum albumin is required. Gamete Res 1987; 18: 121–140
- Oberlander G., Yeung C. H., Cooper T. G. Influence of oral administration of ornidazole on capacitation and the activity of some glycolytic enzymes of rat spermatozoa. J Reprod Fertil 1996; 106: 231–239
- Poulsen J. H., Fischer H., Illek B., Machen T. E. Bicarbonate conductance and pH regulatory capability of cystic fibrosis transmembrane conductance regulator. Proc Natl Acad Sci USA 1994; 91: 5340–5344
- Reddy M. M., Quinton P. M. Selective activation of cystic fibrosis transmembrane conductance regulator Cl− and HCO3− conductance. J Pancreas 2001; 2: 212–218
- Ritta M. N., Calamera J. C., Bas D. E. Occurrence of GABA and GABA receptors in human spermatozoa. Mol Hum Reprod 1998; 4: 769–773
- Roldan E. R. S., Murase T., Shi Q. X. Exocytosis in spermatozoa in response to progesterone and zona pellicida. Science 1994; 266: 1578–1581
- Shalgi R. A Comparative Overview of mammalian Fertilization. Fertilization in Rat, B. S. Dunbar, M. G. O'Rand. Plenum Press, New York 1991; 245–255
- Shalgi R., Phillips D. M. Motility of rat spermatozoa at the site of fertilization. Biol Reprod 1988; 39: 1207–1213
- Shi Q. X., Roldan E. R. S. Bicarbonate/CO2 is not required for zona pellucida- or progesterone-induced acrosomal exocytosis of mouse spermatozoa but is essential for capacitation. Biol Reprod 1995a; 52: 540–546
- Shi Q. X., Roldan E. R. S. Evidence that a GABAA-like receptor is involved in progesterone-induced acrosomal exocytosis in mouse spermatozoa. Biol Reprod 1995b; 52: 373–381
- Shi Q. X., Yuan Y. Y., Roldan E. R. S. gamma-Aminobutyric acid (GABA) induces the acrosome reaction in human spermatozoa. Mol Hum Reprod 1997; 3: 677–683
- Sieghart W. Structure, pharmacology and function of GABAA receptor subtypes. Adv Pharmacol 2006; 54: 231–263
- Staley K. J., Proctor W. R. Modulation of mammalian dendritic GABA(A) receptor function by the kinetics of Cl− and HCO3− transport. J Physiol 1999; 519.3: 693–712
- Suarez S. S. Hyperactivated motility in sperm. J Androl 1996; 17: 331–335
- Suarez S. S., Dai X. Hyperactivation enhances mouse sperm capacity for penetrating viscoelastic media. Biology of Reproduction 1992; 46: 686–691
- Turner K. O., Garcia M. A., Meizel S. Progesterone initiation of the human sperm acrosome reaction: the obligatory increase in intracellular calcium is independent of the chloride requirement. Mol Cell Endocrinol 1994; 101: 221–225
- Uhler M. L., Leung A., Ghan S. Y., Wang G. Direct effects of progesterone and antiprogesterone on human sperm hyperactivated motility and acrosome reaction. Fertil. Steril. 1992; 58: 1191–1198
- Visconti P. E., Bailey J. L., Moore G. D., Pan D., Olds-Clarke P., Kopf G. S. Capacitation of mouse spermatozoa. I. Correlation between the capacitation state and protein tyrosine phosphorylation. Development 1995; 121: 1129–1137
- Visconti P. E., Galantinohomer H., Moore G. D., Bailey J. L., Ning X. P., Fornes M., Kopf G. S. The molecular basis of sperm capacitation. J Androl 1998; 19: 242–248
- Visconti P. E., Westbrook V. A., Chertithin O., Demarco I., Sleight S., Diekman A. B. Novel signaling pathways involved in sperm acquisition of fertilizing capacity. J Reprod Immunol 2002; 53: 133–150
- Wang X. F., Zhou C. X., Shi Q. X., Yuan Y. Y., Yu M. K., Ajonuma L. C., Ho L. S., Lo P. S., Tsang L. L., Liu Y., et al. Involvement of CFTR in uterine bicarbonate secretion and the fertilizing capacity of sperm. Nat Cell Biol 2003; 5: 902–906
- Williams B., Bence M., Evert H., Forrest-Owen W., Lightman S. L., Mcardle C. A. GABAA receptor mediated elevation of Ca2+ and modulation of gonadotropin-release hormone action in alpha T3-1 gonadotropin. J Neroendocrinol 2000; 12: 159–166
- Wistrom C. A., Meizel S. Evidence suggesting involvement of a unique human sperm steroid receptor/Cl− channel complex in the progesterone-initiated acrosome reaction. Dev Biol 1993; 159: 679–690
- Xu W. M., Shi Q. X., Chen W. Y., Zhou C. X., Ni Y., Rowlands D. K., Lui G. Y., Zhu H., Ma Z. G., Wang X. F., et al. Cystic fibrosis transmembrane conductance regulator is vital to sperm fertilizing capacity and male fertility. Proc Natl Acad Sci 2007; 104: 9816–9821
- Yanagimachi R. Mammalian fertilization. Physiology of Reproduction, E. Knobil, J. Neill. Raven Press, New York 1994; 189–317
- Yashimatsu N., Yanagimachi R. Effects of cations and other medium components on the zona-induced acrosome reaction of hamster spermatozoa. Dev Growth Differ 1988; 30: 51–659
- Yeung C. H., Barfield J. P., Anapolski M., Cooper T. G. Volume regulation of mature and immature spermatozoa in a primate model, and possible ion channels involved. Hum. Reprod 2004; 19: 2587–2593
- Yeung C. H., Oberländer G., Cooper T. G. The effects of the male antifertility agent ornidazole on sperm function in vitro and in the female genital tract. J Reprod Fertil 1995; 103(2)257–264
- Yuan Y. Y., Zhang Z. H., Shi Q. X. Initiation of the acrosome reaction in Chinese Hamster sperm by cumulus oophorus and matrix. Acta Anat Sin 1998; 29: 404–409
- Zeng Y., Oberdorf J. A., Florman H. M. PH regulation in mouse sperm: identification of Na+-, Cl−-, HCO3−-dependent and arylaminobenzoate-dependent regulatory mechanisms and characterization of their roles in sperm capacitation. Dev Biol 1996; 173: 510–520
- Zhang G. P., Shi Y. L. Progress in the studies of background chloride channels. Progress in Biochem Biophys 1998; 25: 324–328
- Zhang Z. H., Chen W. Y., Shi Q. X., Yuan Y. Y., Lou Y. C. Capacitating action of GABA and progesterone in spermatozoa of human and guinea pig in vitro. Acta Physiol Sin 2000; 52: 179–184