Abstract
Extracellular ATP has been implicated in a number of cellular events, including mammalian sperm function. The complement of ATP-dependent sperm proteins includes six subunits of the 26S proteasome, a multi-subunit protease specific to ubiquitinated substrate-proteins. Proteolysis of ubiquitinated proteins by the 26S proteasome is necessary for the success of mammalian fertilization, including but not limited to acrosomal exocytosis (AE) and sperm-zona pellucida (ZP) penetration. The 26S proteasome is uniquely present on the sperm acrosomal surface during mammalian, ascidian, and invertebrate fertilization. The proteasome is a multi-subunit protease complex of ∼2 MDa composed of the 19S regulatory complex and a 20S proteolytic core. Integrity of the 19S complex is maintained by six 19S ATPase subunits (PSMC1 through PSMC6). Consequently, we hypothesized that fertilization will be blocked by the depletion of sperm-surface associated ATP (ssATP). Depletion of ssATP by the Solanum tuberosum apyrase, a 49 kDa, non-cell permeant enzyme, significantly reduced the ATP content measured by an adapted luminescence-ATP assay from which all permeabilizing agents were excluded. Addition of active apyrase to porcine in vitro fertilization (IVF) medium caused a concentration dependent reduction in the overall fertilization rate. No such outcomes were observed in control groups using heat-inactivated apyrase. Apyrase treatment altered the band pattern of 19S ATPase subunits PSMC1 (Rpt2) and PSMC4 (Rpt3) in Western blotting, suggesting that it had an effect on the integrity of the sperm proteasomal 19S complex. Apyrase only altered the proteasomal core activities slightly, since these activities are not directly dependent on external ATP. In contrast, sperm treatment with MG132, a specific inhibitor of the proteasomal core chymotrypsin-like activity, inhibited the target proteolytic activity, but also induced a compensatory elevation in proteasomal peptidyl-glutamyl peptide hydrolase activity. Altogether, the present data provide an important missing piece of evidence in support of the ssATP-dependent, proteasomal-proteolytic model of sperm-ZP interactions.
Abbreviations | ||
AE | = | acrosomal exocytosis |
ZP | = | zona pellucida |
ssATP | = | sperm-surface associated ATP |
IVF | = | in vitro fertilization |
ATP | = | adenosine triphosphate |
PGPH | = | peptidyl-glutamyl peptide-hydrolyzing |
LN | = | liquid nitrogen |
AMC | = | aminomethylcoumarin |
Introduction
Ubiquitin is a highly conserved chaperone protein of 76 amino acid residues which binds covalently to substrate proteins through a multi-step enzymatic pathway, catalyzed by the enzymatic activities of ubiquitin-activating enzyme E1, ubiquitin-conjugating enzyme E2, and a substrate-specific ubiquitin ligase E3. Tandem ligation of ubiquitin molecules, resulting in the formation of polyubiquitin chain, is necessary for substrate degradation by the 26S proteasome [Voges et al. Citation1999; Glickman et al. Citation1998]. The 26S proteasome is a multi-subunit protease composed of a 20S proteasome, a barrel-shaped proteolytic core complex, and one or two 19S regulatory complexes, capping one or both ends of 20S proteasome [Voges et al. Citation1999]. The 19S regulatory complex is an asymmetrical, ∼700 kDa protein complex which facilitates the recognition of ubiquitinated substrates via direct binding to the multi-ubiquitin chains, substrate deubiquitination, substrate remodeling, and substrate translocation to the 20S proteasomal core, where the actual substrate proteolysis occurs. The 19S regulatory complex is composed of at least 17 different subunits, forming two distinct subcomplexes, a base, and a lid. Both subcomplexes are linked to each other via non-ATPase subunit Rpn10 (S5a or PSMD4 in mammals). The base is composed of a ring of six different ATPase subunits of the AAA-type (Rpt1 to Rpt6; Rpt=regulatory particle triple A proteins), which dock on both ends onto the rings of the 20S core and two additional non-ATPase subunits, Rpn1 and Rpn2. The lid is built up from eight different subunits, including Rpn3 and Rpn5 to Rpn11 [Glickman et al. Citation1998; Wolf and Hilt Citation2004]. Consequently, ATP is required for the function and structural integrity of the 19S complex. The 20S proteasome is composed of four stacked heptameric rings enclosing two antechambers and a central catalytic chamber [Groll et al. Citation1997], which does not require ATP for its function or sustenance.
The sperm proteasome participates in many steps in the fertilization process from sperm capacitation to acrosomal exocytosis triggered by the egg coat glycoproteins to penetration of the vitelline coat and fusion with the egg plasma membrane [Mykles Citation1998]. The proteasome facilitates sperm binding and penetration through the vitelline coats of the eggs of a solitary ascidian (Halocynthia roretzi) [Sawada et al. Citation1983, Citation2002a; Saitoh et al. Citation1993]. The sperm proteasomes in H. roretzi are localized on the plasma membrane surface of the sperm head [Sawada et al. Citation2002b]. Human sperm proteasomes are localized on the surface of the acrosomal plasma membrane and are involved in zona pellucida/progesterone-induced acrosomal exocytosis and in the sustained phase of the Ca2+ influx provoked by progesterone [Morales et al. Citation2003, Citation2004]. Sutovsky et al. [Citation2003], have inhibited sperm penetration through porcine ZP using reversible proteasomal inhibitors and antibodies specific for the 20S proteasomal core subunits [Sutovsky et al. Citation2004]. Thus, evidence has been accumulating that the 26S proteasome is a protease that is involved in acrosomal functions and digestion of the egg coat during both mammalian and non-mammalian fertilization [reviewed in Sakai et al. Citation2004; Yi et al. Citation2007a; Baska and Sutovsky Citation2005; Morales et al. Citation2007]. The peculiarity of the sperm borne proteasome is in its exposure onto the acrosomal surface, documented by the studies in which proteasomes were recovered from acrosomal membrane fractions [Morales et al. Citation2004] as well as by studies in which fertilization was altered by non-cell permeant agents such as antibodies [Sutovsky et al. Citation2004], recombinant deubiquitinating enzymes (isopetidase T, ubiquitin C-terminal hydrolase UCHL3 [Yi et al. Citation2007b]) and modified ubiquitins (ubiquitin aldehyde [Yi et al. Citation2007b]). A prerequisite of the 19S proteasomal regulatory complex function is the availability of adenosine triphosphate (ATP) as an energy source. In the case of sperm proteasome, this requirement implies that ATP is available on the sperm acrosomal surface and/or in the acrosomal interior which becomes surface accessible during sperm capacitation and acrosomal exocytosis. To this effect, a recent study of sea urchin fertilization demonstrated that sperm proteasomal function and sea urchin fertilization, which occurs in sea water, can be halted by the addition of Solanum tuberosum apyrase [Yokota and Sawada Citation2007], a large 49 kDa ATPase that, due to its size, is not cell permeant [Molnar and Lorand Citation1961; Kettlun et al. Citation2005].
The aim of the present study was to investigate the sperm-surface ATP (ssATP) content of motile boar spermatozoa and examine whether sperm proteasomal function depends on ATP during in vitro fertilization (IVF) of mammalian, porcine oocytes. Boar is a relevant model for understanding human male fertility, as boar and human spermatozoa share similar proteasomal activities and fertilization mechanisms. Porcine sperm morphology, mode of centrosomal inheritance, and mechanism of anti-polyspermy defense during fertilization are similar to humans and different from rodents. The amount of fresh boar spermatozoa that can be collected non-invasively and in a physiological state (i.e. mixed with seminal plasma) cannot be matched by rodent models, while the access to fresh (i.e. not frozen-thawed/cryocapacitated) human spermatozoa is limited due to logistic and ethical considerations.
Results
In order to measure the level of ssATP of boar sperm, fresh, motile boar spermatozoa were incubated with luciferin-luciferase reagent without sperm permeabilization. In a separate group, spermatozoa were incubated with 0.2 mg/ml Solanum tuberosum apyrase (A6410, Sigma) to deplete ssATP during measurement before and after capacitation. As a negative control, apyrase was boiled at 100°C for 5 min, or heat inactivated at 55°C for 30 min and added into the semen at a concentration identical to the concentration of active apyrase in the experimental group, i.e. 0.2 mg/ml.
As shown in , sperm ATP content was higher in non-capacitated control spermatozoa than in capacitated spermatozoa. Surface-associated ATP content of boar spermatozoa was significantly decreased by apyrase treatment both before and after capacitation ().
After capacitation, 10 μM Ca-ionophore was added to the spermatozoa to induce the acrosome reaction during a 10 min incubation; subsequently, 0.2 mg/ml apyrase or boiled apyrase was added to sperm suspension during ATP measurement. The ssATP was significantly lower in apyrase treatment compared to boiled apyrase treatment and control sperm suspension without treatment (). The rate of acrosomal exocytosis in the presence of the Ca-ionophore was 60% and the motility was reduced from 90% to 50%.
Levels of ATP in frozen-thawed boar spermatozoa were also measured with or without apyrase. The ssATP was higher with boiled apyrase treatment than with active apyrase treatment ().
To examine the ssATP levels after acrosome reaction induced by sperm-egg coat binding, zona pellucida (ZP) protein extracts were added to the capacitated spermatozoa to induce acrosome reaction 10 min before starting the measurements. This treatment resulted in a 40% rate of acrosomal exocytosis, while the sperm motility was fairly constant at 80%. Control, capacitated spermatozoa in all experiments showed less than a 20% rate of acrosomal exocytosis. Similar to ionophore treatment, the ZP-induced acrosomal exocytosis reduced ssATP content only slightly; however, ssATP significantly decreased in ZP extract-apyrase treatment during measurement (P < 0.05; ). Inhibition of proteasomal proteolysis (not ATP-dependent) by the specific proteasomal inhibitor MG132 had no effect on ssATP in control spermatozoa or in the acrosome reacted, ZP-exposed spermatozoa ().
Figure 1 Solanum tuberosum apyrase causes the depletion of sperm surface-associated extracellular adenosine triphosphate (ssATP). Measurement of ssATP in boar spermatozoa by luciferin-luciferase was performed without sperm permeabilization. (A) Spermatozoa from fresh semen were measured before or after sperm capacitation. Apyrase was added into medium during ATP measurement. For negative control, apyrase was boiled at 100°C for 5 min. (B) After capacitation, 10 μM Ca-ionophore was added to spermatozoa. (C) Frozen-thawed boar spermatozoa were measured with or without apyrase. (D) After sperm capacitation, zona pellucida (ZP) extracts (10 ZP/μl) were added to spermatozoa. Values are expressed as the mean percentage±SE. Experiments were repeated 10 times. Different superscripts a, b, c, and d in each group of columns denote a significant difference at P < 0.05.
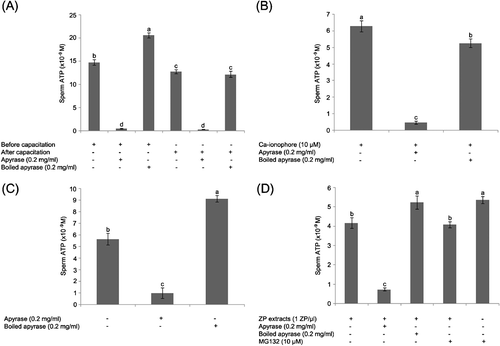
Altogether, the data show that significant levels of ssATP are present in the capacitated spermatozoa that can be depleted efficiently by the addition of active apyrase. Next, the effect of ssATP depletion was examined during porcine IVF. Only the active apyrase reduced the fertilization and polyspermy rates in repeated IVF trials at 0.2 mg/ml (), chosen based on the dose-dependency studies in which porcine oocytes were fertilized in the presence of various concentrations of active apyrase or boiled apyrase (0, 0.1, 0.2 and 0.4 mg/ml; data not shown).
Figure 2 The depletion of sperm surface-associated ATP by apyrase reduces the rate of porcine in vitro fertilization (IVF) in a concentration-dependent manner. Solanum tuberosum apyrase is a ∼49 kDa protein that is not cell permeant, meaning that it can only deplete ssATP in the intact, live cells such as motile spermatozoa. Fertilization parameters at 16 h post IVF are shown. Apyrase that was heat-inactivated at 55°C for 30 min, was used as negative control. Values are expressed as the mean percentage±SE. The numbers of inseminated ova are indicated in parentheses. Different superscripts a, b, and c in each group of columns denote a significant difference at P < 0.05. Oocytes were inseminated in the presence of different concentrations (0.1, 0.2 and 0.4 mg/ml) of apyrase and 0.2 mg/ml boiled apyrase to compare fertilization parameters.
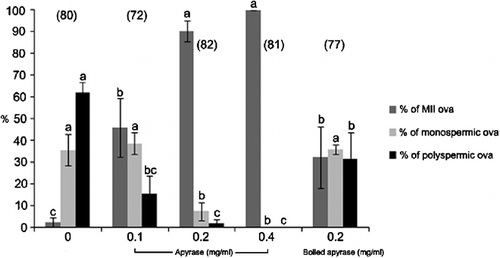
The proteolytic activities of the 20S proteasomal core, including the trypsin-like, chymotrypsin-like, and caspase-like activity, are not directly dependent on ATP. However, given the interaction between the ATPase subunits of the 19S complex and the outer ring subunits of the 20S core, it is possible that ssATP depletion could indirectly affect the 20S core. To measure proteasomal proteolytic activities in the capacitated boar spermatozoa, 1×106 sperm/ml were incubated at 37.5°C with Z-LLL-AMC (fluorometric substrate specific to 20S core chymotrypsin-like activity sensitive to proteasomal inhibitor MG132; final conc. 100 μM) or Z-LLE-AMC (fluorometric substrate specific to 20S core chymotrypsin-like peptidyl-glutamyl peptide-hydrolyzing activity (PGPH) not sensitive to MG132; final conc. 100 μM) for 1 h. In some groups, spermatozoa were treated with 0.2 mg/ml apyrase, 0.2 mg/ml boiled apyrase and/or 10 μM MG132, respectively, during the time-lapse measurement. There were no major differences in the measured proteasomal activities between treatments (Fig. ). However, a major increase in the measurable proteasomal activities was recorded in the capacitated spermatozoa incubated with 10 μM Ca-ionophore. The goal of this treatment was to induce acrosomal exocytosis in as many spermatozoa as possible, thus causing the maximum possible surface exposure of proteasomes present in the acrosomal matrix and on the inner acrosomal membrane. Spermatozoa were incubated again with apyrase, boiled apyrase or MG132. At the 60 min end point, the apyrase reduced the chymotrypsin-like activity of the 20S core by approximately 25%, while it had no effect on PGPH activity. As expected, MG132 knocked-down the chymotrypsin-like activity while it actually stimulated the PGPH activity to a high level, possibly through a compensatory mechanism (Fig. ). In contrast to ionophore-induced, massive acrosomal exocytosis, only a small percentage of capacitated spermatozoa are expected to undergo physiological acrosomal exocytosis when exposed to isolated ZP-protein. Indeed, proteasomal activities in such spermatozoa remained steady and at the basal levels throughout the course of 60 min, and neither apyase nor MG132 had a dramatic effect on sperm proteasomal core activities (Fig. ). Interestingly, boiled apyrase actually seemed to stimulate 20S core activities in the frozen-thawed spermatozoa (Fig. ).
Figure 3 Sperm surface ATP depletion by apyrase alters proteasomal core proteolytic activities in boar spermatozoa. Measurements of proteasomal core-proteolytic activities were conducted in live boar spermatozoa. Spermatozoa (1×106 sperm/ml) were incubated at 37.5°C with Z-LLL-AMC (final conc. 100 μM; A–D) or Z-LLE-AMC (final conc. 100 μM; A′–D′). (A–A′) Spermatozoa were treated with 0.2 mg/ml apyrase, 0.2 mg/ml heat-inactivated apyrase and 10 μM MG132, respectively. (B–B′) Spermatozoa were incubated with 10 μM Ca-ionophore, and then treated with apyrase, boiled apyrase and MG132. (C–C′) Spermatozoa were incubated with zona pellucida (ZP) extracts (10 ZP/μl), and then were treated with apyrase, boiled apyrase, and MG132. (D–D′) Frozen-thawed spermatozoa were treated with apyrase or boiled apyrase. Proteasomal proteolytic activities were measured 3 times every 5 min for 1 h (λex 405 nm and λem 450 nm). The graphs indicate means of fluorescence intensity.
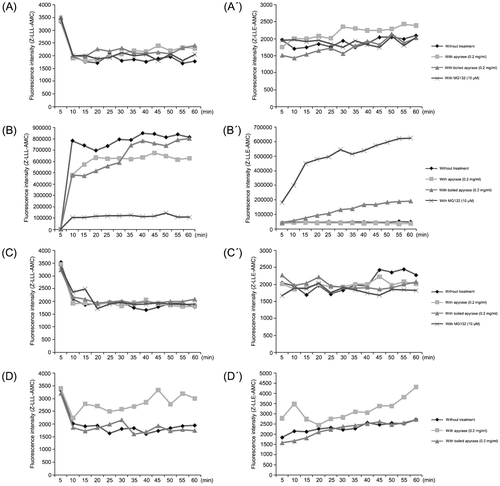
To directly visualize ssATP in boar spermatozoa, modified luciferin/luciferase assay was used, while omitting the cell permeabilization component to avoid the detection of intracellular ATP stores. Prominent fluorescence was detected on the acrosome and postacrosomal sheath, but not on the equatorial segment or in the flagellum of the boar spermatozoa ().
Figure 4 Visualization of ssATP in the boar sperm head by the modified, non-cell permeant CellTitreGlo assay kit. In the presence of ATP, the luciferase component of the kit cleaves luciferin, releasing measurable fluorescence at ∼575 nm wavelength (A). Panel B shows negative control without luciferin.
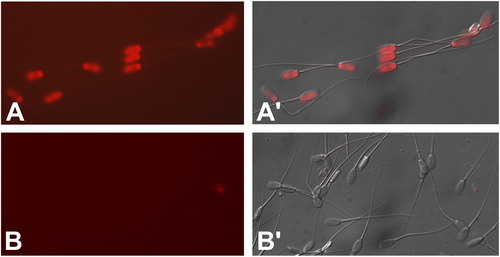
To examine the effect of apyrase treatment on sperm proteasome integrity, we incubated boar spermatozoa for 6 h with 0.4 mg/ml apyrase or 0.4 mg/ml boiled apyrase, and performed western blotting with antibodies recognizing various 19S and 20S proteasomal subunits. The most dramatic change in band pattern was observed for the 19S ATPase subunits Rpt2 () and Rpt3 (). A subtle change of the band pattern was also observed with a polyclonal antibody raised against the whole 20S proteasomal core (), while the antibody against the alpha-type 20S core subunits showed identical band patterns and band densities in all treatments (). No bands were observed in the negative control ().
Figure 5 Effect of a six-hour apyrase treatment on the integrity of the sperm proteasome. Spermatozoa were incubated in the presence of 0.4 mg apyrase (Lane 1), 0.4 mg heat-inactivated apyrase (Lane 2), 100 μM MG132 (Lane 3), or only sperm (Lane 4). Lane 5 is the vehicle without sperm extracts. Western blots were probed with antibodies against the 19S ATPase subunits PSMC1/Rpt2 (A) and PSMC4/Rpt3 (B), with a polyclonal antibody raised against the whole 20S proteasome (C), and with a monoclonal antibody specific to alpha-type 20S core subunits (D). Negative control blot (E) and residual, Coomassie blue stained gel (F) are also shown. Bands unique to active apyrase lane are marked with asterisks.
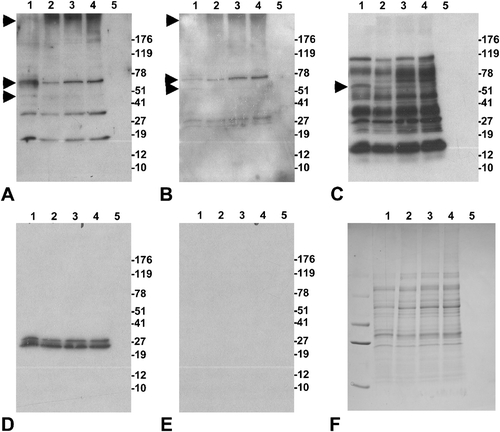
Discussion
Evidence for the involvement of proteasomal proteolysis in sperm capacitation, acrosomal exocytosis and sperm-egg coat penetration has been accruing steadily, coming from model systems as varied as ascidians and echinoderms, and from a range of mammalian species (rodents, ungulates, humans) [reviewed in Sakai et al. Citation2004; Yi et al. Citation2007a; Morales et al. Citation2007]. One apparent discrepancy between the proposed role of sperm proteasome in fertilization and the current knowledge of sperm physiology is the requirement of ATP for the sustenance of the 19S proteasomal regulatory complex and for some steps of ubiquitin-substrate conjugation and substrate remodeling by the 19S complex. Sperm ATP concentration is thought to be high in the sperm midpiece mitochondria and sperm tail principal piece, but not necessarily in the sperm acrosome. There are at least three different sources of ATP generation in the spermatozoa [Rodriguez-Miranda et al. Citation2008], including oxidative phosphorylation in the sperm mitochondria [Halangk et al. Citation1985], glycolysis in the fibrous sheath of the sperm tail principal piece [Krisfalusi et al. Citation2006], and the conversion of ADP to ATP by adenylate kinase [Schoff et al. Citation1989; Foresta et al. Citation1992]. Of particular interest to the present study is the detection of adenylate kinase-dependent KATP-potassium channels in the sperm acrosome [Acevedo et al. Citation2006].
The present study demonstrates that measurable levels of ssATP are present in viable, capacitated spermatozoa, that this ssATP can be knocked down by a non-permeant ATPase (apyrase) and that doing so halts porcine fertilization in vitro. While no dramatic effect of apyrase on the ATP-independent sperm proteasomal core activities was expected, there was a ∼25% reduction in the chymotrypsin-like 20S core activity observed in the presence of apyrase when sperm acrosomal exocytosis was mass-induced by the calcium-ionophore. The most likely mechanisms by which ATP depletion affected the sperm proteasome is through changes in the integrity of the 19S regulatory complex, which depends on six ATPase subunits (PSMC1-6) for its sustenance. This hypothesis is supported by the observed changes in the Western blotting-band pattern of 19S subunits in the apyrase-treated spermatozoa. In addition, sperm surface ATP may be required for the phosphorylation of other, non-ATPase subunits of the 26S proteasome. At least two recent studies showed that proteasomal subunits are phosphorylated during sperm capacitation, including tyrosine phosphorylation of the 20S core subunit α6 in mouse [Arcelay et al. Citation2008] and tyrosine phosphorylation of the 20S α4 subunit in human spermatozoa [Diaz et al. Citation2007]. The control, heat inactivated apyrase had no inhibitory effect on fertilization or ssATP levels. Such a denatured apyrase actually increased the proteasomal PGPH-activity in the spermatozoa exposed to calcium-ionophore, and increased the ssATP levels in non-capacitated spermatozoa. We can only speculate at this moment that the presence of denatured extrinsic protein somehow stimulated sperm proteasomal metabolism, or prevented the loss of ssATP during ionophore-induced or spontaneous acrosome reaction. In any case, the denatured apyrase appeared to be a suitable control for bioactive apyrase in the present series of experiments.
Extracellular/cell surface ATP has been studied in the male reproductive system and many non-gametic systems and is thought to be an important regulatory and signaling factor [reviewed in Zhou et al. Citation2007; Deli and Csernoch Citation2008]. Extracellular ATP likely has many different roles during sperm capacitation, acrosomal exocytosis, and fertilization. In this context, the present results do not directly establish the requirement of extracellular ATP/ssATP for sperm proteasomal function during fertilization. Instead, the experiments described in this communication were designed to provide a missing piece of evidence by showing that ATP is available to the sperm surface-exposed proteasomes, and more specifically to those proteasomes exposed to the acrosomal surface during fertilization. Altogether, our measurements of ssATP bode well with the finding that the addition of extracellular ATP significantly improved the success rate of mouse fertilization in vitro [Rodriguez-Miranda et al. Citation2008; Foresta et al. Citation1992; Luria et al. Citation2002]. It is possible that in addition to a frequently discussed role of extracellular ATP in cellular signaling, boar spermatozoa1 ssATP serves as an energy donor for the 19S proteasomal regulatory complex ATPases during fertilization. Thus, this piece of evidence eliminates an important concern about the theory of sperm proteasomal function during fertilization.
Materials and Methods
Semen Collection
Semen was collected from adult Duroc boars 15–22 months of age. Boars were housed at a pig farm of Chungnam National University in Daejeon. Semen was collected one time per week from each of four Duroc boars. The sperm-rich fraction (30 to 60 ml) of ejaculate was collected into an insulated vacuum bottle by gloved-hand technique. The sperm-rich fractions of ejaculates with greater than 85% motile sperm and normal acrosome were used.
Determination of Semen Volume, Sperm Concentration, Motility, and Acrosomal Integrity
Semen volumes were determined with a graded cylinder. Sperm concentrations were estimated by a hemocytometer. The percentage of motile sperm was estimated at 38.5°C by light microscope at 250×magnification. Spermatozoa were fixed with 1% glutaraldehyde in Beltsville thawing solution (BTS; 3.71 g glucose, 0.60 g tri-sodium citrate, 1.25 g ethylenediamine tetraacetic acid, 1.25 g sodium bicarbonate, 0.75 g potassium chloride, and 100.0 ml distilled water) to examine acrosomal morphology. One hundred spermatozoa per sample were evaluated by phase contrast microscopy at 1,000×magnification. Acrosomes were differentially categorized into four morphological classes: normal apical ridge (NAR), damaged apical ridge (DAR), missing apical ridge (MAR), and loose acrosomal cap (LAC) as described by Pursel et al. [Citation1972]. The sperm-rich fractions of ejaculates with >85% motile sperm and predominantly normal apical ridge (NAR) acrosomes were used in the experiments.
Semen Cryopreservation and Thawing
Semen was slowly cooled to room temperature (RT; 20 to 23°C) within 2 h after collection and centrifuged for 10 min at 800×g. The supernatant was poured off and one volume of concentrated spermatozoa was resuspended in one volume of lactose, egg yolk, and N-acetyl-D-glucosamine (LEN) diluent (the first diluent to contain 1.0×109 sperm/ml) at RT. Semen was cooled in a refrigerator to 4°C over 2 h period and one volume of LEN+4% glycerol diluents+1% Orvus ES paste (Nova Chemical Sales Inc., MA, USA; the second diluent) was added to 1 volume of cooled semen. Straws (Minitüb Gmbh, Landshut, Germany) were immediately filled with 5 ml of semen and sealed with steel or glass balls. The straws were horizontally placed on an aluminum rack and set into a liquid nitrogen tank containing liquid nitrogen (LN). The straws were situated 5 cm above the LN, and kept at that level for 20 min before the straws were transferred into LN storage. For IVF, straws were thawed in 52°C water bath for 40 sec, which brings the temperature of the sperm to 15°C [Yi et al. Citation2002].
Preparation of Solubilized Zona Pellucida Proteins
A modified Bastiaan et al. [Citation1999] protocol was followed. Oocytes were collected and matured as described above. Cumulus cells were removed with 0.1% hyaluronidase in TL-HEPES-PVA medium and washed three times with TL-HEPES PVA. Fifty oocytes were placed in a plastic Eppendorf tube containing TL-HEPES-PVA and centrifuged for 15 min at 1,800×g, supernatant was removed carefully under a microscope, leaving only the oocytes at the bottom of the tube. A total volume of 5 μl of 10 mM HC1 was then added to the oocytes in the tube; solubilization of the ZP was microscopically observed and controlled. Zona-deprived oocytes were left at the bottom of the Eppendorf tube. Following solubilization, 5 μl of 10 mM NaOH was added to the solubilized ZP, to render a final zona volume of 10 μl, containing the protein equivalent of 5 zona per microliter.
Collection and In Vitro Maturation of Porcine Ova
Ovaries were collected from prepubertal gilts at a local abattoir, transported to the laboratory in a warm box, and rinsed in 0.9% NaCl solution containing 75 μg/ml penicillin G and 50 μg/ml streptomycin sulfate at 37.5°C. Cumulus oocyte complexes (COCs) were aspirated from antral follicles (3 to 6 mm in diameter), washed three times in HEPES-buffered Tyrode lactate (TL-HEPES-PVA) medium containing 0.1% (w/v) polyvinyl alcohol (PVA), and three times with the maturation medium [Abeydeera et al. Citation1998]. A total of 50 COCs were transferred to 500 μl of the maturation medium that had been covered with mineral oil in a 4-well multidish (Nunc, Roskilde, Denmark) and equilibrated at 38.5°C, 5% CO2 in air. The medium used for oocyte maturation was tissue culture medium (TCM) 199 (Gibco, Grand Island, NY) supplemented with 0.1% PVA, 3.05 mM D-glucose, 0.91 mM sodium pyruvate, 0.57 mM cysteine, 0.5 μg/ml LH (L 5269, Sigma), 0.5 μg/ml FSH (F 2293, Sigma), 10 ng/ml epidermal growth factor (E 4127, Sigma), 10% porcine follicular fluid, 75 μg/ml penicillin G and 50 μg/ml streptomycin. After 22 h of culture, the oocytes were washed twice and cultured in TCM199 without LH and FSH for 22 h at 38.5°C, 5% CO2 in air.
In Vitro Fertilization and Culture of Porcine Ova
After the completion of in vitro maturation, cumulus cells were removed with 0.1% hyaluronidase in TL-HEPES-PVA medium and washed three times with TL-HEPES-PVA medium and three times with Tris-buffered (mTBM) medium [Abeydeera et al. Citation1998] containing 0.2% (w/v) BSA (A 7888, Sigma). Thereafter, 25–30 oocytes were placed into each 50 μl drop of the mTBM medium, covered with mineral oil in 35 mm polystyrene culture dish. A semen pellet was thawed in PBS containing 0.1% PVA (PBS-PVA) at RT and centrifuged at 1,500×g on a 60% and 40% isotonic Percoll gradient for 10 min. The spermatozoa were resuspended and washed twice in PBS-PVA at 800×g for 5 min, and finally resuspended in mTBM medium. After appropriate dilution, 50 μl of this sperm suspension was added to 50 μl of the medium that contained oocytes to give a final sperm concentration of 1×106 cells/ml where applicable, apyrase was added to fertilization drops at the time of sperm addition. Oocytes were coincubated with spermatozoa for 6 h at 38.5°C, 5% CO2 in air. At 6 h after IVF, oocytes were transferred into 500 μl NCSU-23 culture medium containing 0.4% BSA (A6003, Sigma) for further culture lasting 13–19 h. Fertilization was evaluated using DAPI staining for epifluorescence microscopy and differential interference contrast (DIC) microscopy as described previously [Yi et al. Citation2007b]. Oocytes with two or more pronuclei and at least one sperm tail in the ooplasm were recorded as fertilized.
Capacitation and Acrosome Reaction of Boar Spermatozoa
Spermatozoa isolated from fresh boar semen (2×108 sperm/ml) were incubated in capacitation medium consisting of 35 mM NaCl, 5 mM KCl, 2 mM CaCl2, 1 mM MgCl2, 20 mM HEPES, 10 mM glucose, 15 mM NaHCO3, and 5 mg/ml BSA (pH 7.4) for 1 h at 37.5°C. To induce acrosome reaction, spermatozoa were incubated with 10 μM Ca-ionophore or zona pellucida (ZP) extracts (10 ZP/μl) for 1 h at 37.5°C before measurement.
Measurement and Visualization of Sperm Surface-Associated ATP
Semen was washed twice with PBS at room temperature, collected by centrifugation at 800×g for 5 min and adjusted to concentration of 2×106 sperm/ml with PBS. Sperm ATP content was determined using a luciferase reaction kit without permeabilizing buffer according to the manufacturer's protocol (ATPliteTM, Perkin Elmer Inc., Boston, MA). Standards were prepared from ATP standard (Perkin Elmer) using serial dilutions to obtain concentrations of 1×10−7, 5×10−8, 1×10−8, 5×10−9, 1×10−9, 5×10−10, and 1×10−10 M. Aliquots of the ATP stock solution were stored at −20°C until use, and standard curve dilutions were prepared for each assay. Bioluminescence was measured with an HTS multi label reader (Perkin Elmer) after addition of 100 μl sample and 100 μl luciferin-luciferase reagent. Sperm motility was examined before and after measurement by light microscopy to confirm that the luciferase reagent did not cause sperm damage.
To visualize ssATP in the individual sperm compartments, motile boar spermatozoa were incubated with the modified ATP-reporting CellTitreGlo assay kit (Promega, Madison, WI, USA) from which the cell permeabilizing buffer was omitted. In this assay, as well as in the ATPlite assay, beetle luciferin is cleaved by firefly luciferase in the presence of extrinsic ATP, producing a red-fluorescent oxyluciferin. Spermatozoa were mounted on microscopy slides, exposed for 2 sec to excitation by UV-light (peak wavelength of 366 nm) under a Nikon Eclipse 800 epifluorescence microscope, and immediately photographed using red-fluorescence filter (peak wavelength of 575 nm; exposure time 500 milliseconds). Images were recorded by using a CoolSnap HQ digital camera and MetaMorph imaging software.
Assay of Proteasomal-Proteolytic Activity in Boar Sperm
Spermatozoa isolated from fresh semen were treated with 10 μM Ca-ionophore, then treated with an equivalent of 10 oocyte ZP/μl extract and frozen-thawed sperm were measured. In order to deplete ssATP, 0.2 mg/ml high activity apyrase (A7646, Sigma) was added into the medium in a separate treatment group. For the negative control, 0.2 mg/ml heat inactivated apyrase (heated to 55°C for 30 min) was added into the medium instead of active apyrase. Adjusted and treated spermatozoa (conc. 1×106 sperm/ml) were loaded into a 96-well black plate (Perkin Elmer), and incubated at 37.5°C with Z-LLL-AMC (a specific substrate for 20S chymotrypsin-like activity; final conc. 100 μM; BostonBiochem) or Z-LLE-AMC (a specific substrate for 20S chymotrypsin-like peptidyl-glutamyl-peptide hydrolyzing [PGPH] activity; final conc. 100 μM; BostonBiochem) for 1 h. Fluorogenic proteasomal core substrates are composed of a small peptide (LLL/LLE) coupled to a fluorescent probe, aminomethylcoumarin (AMC). The intact AMC-coupled substrate does not emit fluorescence. In the presence of appropriate 20S core activity, the AMC molecule is cleaved off and becomes fluorescent. This emitted fluorescence was measured every 5 min for a period of one h, yielding a curve of relative fluorescence (no units). Spermatozoa and substrate measurements remained flat, while spermatozoa mixed with the substrates yielded a significant level of fluorescence. Conventional, non-proteasomal proteases (e.g. trypsin) are unable to cleave the above proteasomal substrates, and yielded no fluorescence in the assay (not shown). Fluorescence intensity was measured by HTS multi label reader (Perkin Elmer) between λex 405 nm and λem 450 nm.
Western Blotting
Boar spermatozoa were incubated with 0.4 mg apyrase, 0.4 mg boiled apyrase or with 100 μM MG132 for 6 h at 37.5°C, respectively. After incubation, sperm pellets were boiled with loading buffer (50 mM Tris (pH 6.8), 150 mM NaCl, 2% SDS, 20% glycerol, 5% β-mercaptoethanol, 0.02% bromophenol blue) and gel electrophoresis was performed on 4–20% gradient gels (PAGEr® Precast Gels, Lonza, Rockland, ME). Proteins were transferred to PVDF membranes (Millipore Corp., Bedford, MA) using an Owl wet transfer system (Fisher Scientific, Houston, TX) at a constant 50V for 4 h. The membranes were sequentially incubated with 10% non-fat milk for 1 h, with primary antibody overnight and with an HRP-conjugated goat anti-rabbit or goat anti-mouse IgG (dil. 1/10,000 dilution) for 1 h. The membranes were reacted with chemiluminiscent substrate (Millipore) and visualized by exposing to X-ray film. Primary antibodies, all diluted to 1:2,000 included the rabbit polyclonal antibody to 19S regulator ATPase subunit Rpt2 (Biomol #PW8305), rabbit polyclonal antibody to 19S regulator ATPase subunit Rpt3 (Biomol #PW8250), rabbit polyclonal antibody recognizing multiple 20S core subunits (Biomol #PW 8155; recognized subunits include α5/α7, β1, β5, β5i, β7) and mouse monoclonal antibody against 20S core subunits α1, 2, 3, 5, 6 & 7 (Biomol #PW8195).
Statistical Analysis
Analysis of variance (ANOVA) was carried out using the SAS package in a completely randomized design. Duncan's multiple-range test was used to compare values of individual treatment when the F-value was significant (P < 0.05).
Acknowledgments
This work was supported by the Korea Science and Engineering Foundation (KOSEF) grant funded by the Korea Government (MEST) (No. R11-2002-100-04001-0) to Y-JY and C-SP, by National Research Initiative Competitive Grant # 2007-01319 from the USDA Cooperative State Research, Education and Extension Service to PS, and by seed funding from the Food for the 21st Century Program to the University of Missouri-Columbia to PS.
Declaration of Interest: The authors report no conflicts of interest. The authors alone are responsible for the content and writing of the paper.
References
- Abeydeera L. R., Wang W. H., Prather R. S., Day B. N. Maturation in vitro of pig oocytes in protein-free culture media: fertilization and subsequent embryo development in vitro. Biol Reprod 1998; 58(5)1316–1320
- Acevedo J. J., Mendoza-Lujambio I., de la Vega-Beltran J. L., Trevino C. L., Felix R., Darszon A. KATP channels in mouse spermatogenic cells and sperm, and their role in capacitation. Dev Biol 2006; 289(2)395–405
- Arcelay E., Salicioni A. M., Wertheimer E., Visconti P. E. Identification of proteins undergoing tyrosine phosphorylation during mouse sperm capacitation. Int J Dev Biol 2008; 52(5–6)463–472
- Baska K. M., Sutovsky P. Protein modification by ubiquitination and is consequences for spermatogenesis, sperm maturation, fertilization and pre-implantation embryonic development. New Impact on Protein Modifications in the Regulation of Reproductive System, T. Tokumoto. Research Signpost, KeralaIndia 2005; 83–114
- Bastiaan H., Franken D., Wranz P. G-protein regulation of the solubilized human zona pellucida-mediated acrosome reaction and zona pellucida binding. J Assist Reprod Genet 1999; 16(6)332–336
- Deli T., Csernoch L. Extracellular ATP and cancer-an overview with special reference to p2 purinergic receptors. Pathol Oncol Res 2008; 14(3)219–231
- Diaz E. S., Kong M., Morales P. Effect of fibronectin on proteasome activity, acrosome reaction, tyrosine phosphorylation and intracellular calcium concentrations of human sperm. Hum Reprod 2007; 22(5)1420–1430
- Foresta C., Rossato M., Di Virgilio F. Extracellular ATP is a trigger for the acrosome reaction in human spermatozoa. J Biol Chem 1992; 267(27)19443–19447
- Glickman M. H., Rubin D. M., Coux O., Wefes I., Pfeifer G., Cjeka Z., Baumeister W., Fried V. A., Finley D. A subcomplex of the proteasome regulatory particle required for ubiquitin-conjugate degradation and related to the COP-signalosome and eIF. Cell 1998; 94(5)615–623
- Groll M., Ditzel L., Lowe J., Stock D., Bochtler M., Bartunik H. D., Huber R. Structure of 20S proteasome from yeast at 2.4 A resolution. Nature 1997; 386(6624)463–471
- Halangk W., Bohnensack R., Kunz W. Interdependence of mitochondrial ATP production and extramitochondrial ATP utilization in intact spermatozoa. Biochim Biophys Acta 1985; 808(2)316–322
- Kettlun A. M., Espinosa V., Garcia L., Valenzuela M. A. Potato tuber isoapyrases: substrate specificity, affinity labeling, and proteolytic susceptibility. Phytochemistry 2005; 66(9)975–982
- Krisfalusi M., Miki K., Magyar P. L., O'Brien D. A. Multiple glycolytic enzymes are tightly bound to the fibrous sheath of mouse spermatozoa. Biol Reprod 2006; 75(2)270–278
- Luria A., Rubinstein S., Lax Y., Breitbart H. Extracellular adenosine triphosphate stimulates acrosomal exocytosis in bovine spermatozoa via P2 purinoceptor. Biol Reprod 2002; 66(2)429–437
- Molnar J., Lorand L. Studies on apyrases. Arch Biochem Biophys 1961; 93: 353–363
- Morales P., Diaz E. S., Kong M. Proteasome activity and its relationship with protein phosphorylation during capacitation and acrosome reaction in human spermatozoa. Soc Reprod Fertil Suppl 2007; 65: 269–273
- Morales P., Kong M., Pizarro E., Pasten C. Participation of the sperm proteasome in human fertilization. Hum Reprod 2003; 18(5)1010–1017
- Morales P., Pizarro E., Kong M., Jara M. Extracellular localization of proteasomes in human sperm. Mol Reprod Dev 2004; 68(1)115–124
- Mykles D. L. Intracellular proteinases of invertebrates: calcium-dependent and proteasome/ubiquitin-dependent systems. Int Rev Cytol 1998; 184: 157–289
- Pursel V. G., Johnson L. A., Rampacek G. B. Acrosome morphology of boar spermatozoa incubated before cold shock. J Anim Sci 1972; 34(2)278–283
- Rodriguez-Miranda E., Buffone M. G., Edwards S. E., Ord T. S., Lin K., Sammel M. D., Gerton G. L., Moss S. B., Williams C. J. Extracellular adenosine 5′-triphosphate alters motility and improves the fertilizing capability of mouse sperm. Biol Reprod 2008; 79(1)164–171
- Saitoh Y., Sawada H., Yokosawa H. High-molecular-weight protease complexes (proteasomes) of sperm of the ascidian, Halocynthia roretzi: isolation, characterization, and physiological roles in fertilization. Dev Biol 1993; 158(1)238–244
- Sakai N., Sawada M. T., Sawada H. Non-traditional roles of ubiquitin-proteasome system in fertilization and gametogenesis. Int J Biochem Cell Biol 2004; 36(5)776–784
- Sawada H., Sakai N., Abe Y., Tanaka E., Takahashi Y., Fujino J., Kodama E., Takizawa S., Yokosawa H. Extracellular ubiquitination and proteasome-mediated degradation of the ascidian sperm receptor. Proc Natl Acad Sci USA 2002a; 99(3)1223–1228
- Sawada H., Takahashi Y., Fujino J., Flores S. Y., Yokosawa H. Localization and roles in fertilization of sperm proteasomes in the ascidian Halocynthia roretzi. Mol Reprod Dev 2002b; 62(2)271–276
- Sawada H., Yokosawa H., Hoshi M., Ishii S. Ascidian sperm chymotrypsin-like enzyme; participation in fertilization. Experientia 1983; 39(4)377–378
- Schoff P. K., Cheetham J., Lardy H. A. Adenylate kinase activity in ejaculated bovine sperm flagella. J Biol Chem 1989; 264(11)6086–6091
- Sutovsky P., Manandhar G., McCauley T. C., Caamano J. N., Sutovsky M., Thompson W. E., Day B. N. Proteasomal interference prevents zona pellucida penetration and fertilization in mammals. Biol Reprod 2004; 71(5)1625–1637
- Sutovsky P., McCauley T. C., Sutovsky M., Day B. N. Early degradation of paternal mitochondria in domestic pig (Sus scrofa) is prevented by selective proteasomal inhibitors lactacystin and MG132. Biol Reprod 2003; 68(5)1793–1800
- Voges D., Zwickl P., Baumeister W. The 26S proteasome: a molecular machine designed for controlled proteolysis. Annu Rev Biochem 1999; 68: 1015–1068
- Wolf D. H., Hilt W. The proteasome: a proteolytic nanomachine of cell regulation and waste disposal. Biochim Biophys Acta 2004; 1695(1–3)19–31
- Yi Y. J., Im G. S., Park C. S. Lactose-egg yolk diluent supplemented with N-acetyl-D-glucosamine affect acrosome morphology and motility of frozen-thawed boar sperm. Anim Reprod Sci 2002; 74(3–4)187–194
- Yi Y. J., Manandhar G., Oko R. J., Breed W. G., Sutovsky P. Mechanism of sperm-zona pellucida penetration during mammalian fertilization: 26S proteasome as a candidate egg coat lysin. Soc Reprod Fertil Suppl 2007a; 63: 385–408
- Yi Y. J., Manandhar G., Sutovsky M., Li R., Jonakova V., Oko R., Park C. S., Prather R. S., Sutovsky P. Ubiquitin C-terminal hydrolase-activity is involved in sperm acrosomal function and anti-polyspermy defense during porcine fertilization. Biol Reprod 2007b; 77(5)780–793
- Yokota N., Sawada H. Sperm proteasomes are responsible for the acrosome reaction and sperm penetration of the vitelline envelope during fertilization of the sea urchin Pseudocentrotus depressus. Dev Biol 2007; 308(1)222–231
- Zhou W. L., Zuo W. L., Ruan Y. C., Wang Z., Du J. Y., Xiong Y., Chan H. C. The role of extracellular ATP in the male reproductive tract. Sheng Li Xue Bao 2007; 59(4)487–494