ABSTRACT
Trophoblast phagocytosis has been considered important in pregnancy. However, whether human preimplantation blastocysts possess phagocytic activity is still unclear. In this study, we determined the phagocytosis potential in human trophectoderm cells of blastocysts prior to implantation. Fluorescent microspheres were used as markers for phagocytic analysis under transmission electron microscope (TEM) and fluorescence microscopy. Phagocytosis of 1 μm fluorescent microspheres was observed in most (9/11) day-6 and even some (2/9) day-5 blastocysts. More effective phagocytosis occurred in blastocysts at the morula-blastocyst stage of day-6. Furthermore, we observed an increased trend of phagocytic acitivities in polar trophectoderm. Our findings indicated phagocytic ability exists in human blastocysts prior to implantation and the differentiation between polar and mural trophectoderm may be associated with blastocyst implantation.
Abbreviations: TEM: transmission electron microscope; ZP: zona pellucida; PGD: preimplantation genetic diagnosis; ICM: inner cell mass; FGF4: fibroblast growth factor 4
Introduction
Phagocytosis, defined as the ingestion by cells of large (≥0.5 μm) particles [Flannagan et al. Citation2012], was first observed by Elie Metchnikoff over a century ago [Metchnikoff Citation1884]. It starts with the recognition of the target and then forms a phagocytic cup around the target by membrane protrusion to engulf it into the cell [Swanson Citation2008; Underhill and Goodridge Citation2012].
Phagocytic activity has been described previously in the trophoblast cells, particularly in rodents [Bevilacqua and Abrahamsohn Citation1988; Enders and Schlafke Citation1969; Finn and Lawn Citation1968]. In humans, necrotic decidual cells were found in the phagosomal vacuoles within cytotrophoblast cells at early stage of gestation approximately 7-9 days after fertilization [Hata et al. Citation1981]. Human extravillous trophoblast cells were also demonstrated as phagocytes during early pregnancy [Choy and Manyonda Citation1998; Manyonda and Choy Citation1999]. Moreover, placental syncytiotrophoblast displayed phagocytic uptake to aquire adequate nutrition for developing placenta [Burton et al. Citation2002]. Collectively, these studies suggest the roles of trophoblast phagocytosis in acquisition of nutrition or space for embryo development in tissue remolding during implantation and placentation.
Trophectoderm cells, the first differentiated cell type that arise during blastocyst formation, were reported to possess effective phagocytosis in murine [Drake and Rodger Citation1987]. The phagocytosis potential is triggered during trophectoderm formation at the morula-blastocyst stage and considered a differentiation marker for trophoblast giant cells during embryo implantation in rodents [Red-Horse et al. Citation2004]. A recent study observed active phagocytosis of adjacent epithelium cell debris in the mural trophectoderm cells during murine blastocyst reactivation and attachment [Fu et al. Citation2014]. Furthermore, phagocytosis of a latex compound bead (1-3 μm) has been demonstrated more active at mural trophectoderm than polar pole in mice and researchers observed that implantation was drastically hampered after ingestion of large latex particles by a small number of the mural trophectoderm cells [Rassoulzadegan et al. Citation2000]. However, little is known concerning trophectoderm phagocytosis and a number of issues remain unsettled [Bevilacqua et al. Citation2010]. It has been implicated in the uptake of uterine gland secretions by pig blastocyst trophectoderm [Baumbach et al. Citation1990]. In rodents, phagocytosis is generally considered a marker for trophoblast differentiation in giant cells during embryo implantation. Whether human trophectoderm possesses the phagocytic activity and its connection to implantation have not been elucidated. In this study, we co-cultured human embryos with fluorescent microspheres (1 μm) and investigated the phagocytic behavior of trophectoderm in preimplantation human blastocysts in vitro by using transmission electron microscope (TEM) and fluorescence microscopy.
Results
TEM analysis of phagocytosis on day 5
To assess the phagocytic potential, we used TEM to examine the sections which were sampled from nine, day-5 (D5) blastocysts after overnight co-culture with microspheres. In seven out of nine blastocysts, no evidence of phagocytic activity occurred and the cytoplasm of these trophectoderm was dominated by typical ultrastructural features, with well-developed nuclei and abundant mitochondrions (). Nevertheless, there were one or two phagcytosed microspheres found in trophectoderm derived from two blastocysts on D5, as shown in . These microspheres displayed a uniform density in the cytoplasm of trophectoderm cells and could be easily distinguished from the cellular organelles for the profile of them was round to oval, quite different from that of mitochondrion which varied considerably in size and structure.
Figure 1. Detection of phagocytic activity in day 5 blastocyst under transmission electron microscope. (A) No evidence of phagocytic activity is observed in the cytoplasm of trophectoderm. (B) One microsphere is found within trophectoderm cell. Short arrow shows the phagocytosed microsphere. M: mitochondria. Bars: (A) 2 μm; (B) 2 μm; 49 x 17 mm (300 x 300 DPI).
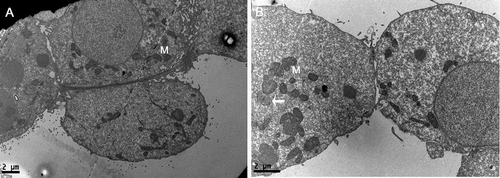
TEM analysis of phagocytosis on day 6
A total of 11 D6 blastocysts were used for TEM analysis and nine blastocysts were observed with the presence of phagocytosed microspheres. As shown in , a large number of microspheres were identified intracellularly rather than being merely adsorbed to the cell surface. It was interesting to note that trophectoderm was able to phagocytize micropheres in spite of their large size, resulting in a marked distortion of the phagocyte (). Moreover, it displayed a target–acquisition phenotype that consisted of membranous protrusions which captured targets in phagocytic cup-like structures (). Interestingly, we detected the phagocytosis of cell debris by trophectoderm which possessed protrusions derived from the apical membrane that faced the blastocyst cavity ().
Figure 2. Phagocytosis by trophectoderm in day 6 blastocyst under transmission electron microscope. (A) Many microspheres are observed within trophectoderm cells after overnight co-culture. Short arrow shows the profile of microspheres. (B) Distorted trophectoderm cell with intracellular microsphere. (C) Phagocytic cup formation. Long arrow points to the membrane protrusions and short arrow indicates a phagocytosed microsphere. (D) Phagocytosis of cell debris. Long arrow shows the membrane protrusions and short arrow indicates cell debris. M: mitochondria. Bars: (A) 2 μm; (B) 0.5 μm; (C) 0.5 μm; (D) 1 μm; 83 x 49 mm (300 x 300 DPI).
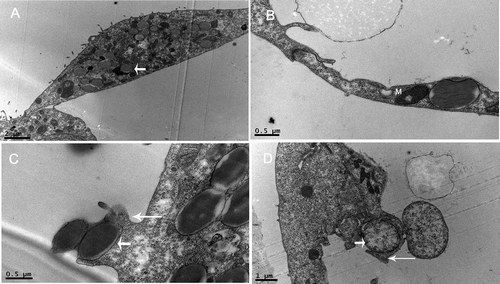
Observation of phagocytosis under fluorescence microscopy
As shown in , the cells of blastocyst exhibited granulated staining of phagocytosed microspheres (green) and all cell nuclei were visualized by DAPI staining (). It was possible to identify the location of inner cell mass (ICM) with more densely packed blue nuclei. Interestingly, a graded pattern was observed in the trophectoderm, from low phagocytic activity in the part immediately adjacent to the mural region to a maximal activity at the polar pole near the ICM ().
Figure 3. Phagocytic analysis of microspheres by day 6 blastocyst under fluorescence microscopy. (A) Granulated green fluorescence emitted by phagocytosed microspheres. Long arrow shows inner cell mass (ICM) region, where green fluorescence intensity is higher. (B) TE and ICM nuclei staining with DAPI. Long arrow shows ICM location, where blue nuclei are densely packed. (C) Merged images of A and B. The polar-mural axis is indicated by the single-headed arrow. P: polar; M: mural; Bar = 20 μm; 140 x 34 mm (300 x 300 DPI).
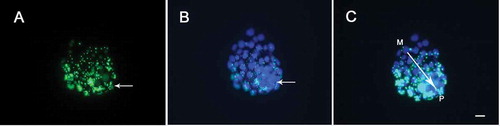
Discussion
Phagocytosis is a complex process that differs in species depending on the phagocytic particle [Mao and Finnemann Citation2015]. It plays an important role in host defense against harmful pathogens and the clearance of dead cells to maintain tissue homeostasis as well as remodeling [Underhill and Goodridge Citation2012]. Trophoblast phagocytosis also integrates different roles which are closely connected to the embryo implantation and placentation process in different species [Bevilacqua et al. Citation2010].
In this study, we used TEM and fluorescence microscopy to detect the phagocytic activity of human trophectoderm cells before implantation in vitro. A previous study found that the human blastocyst was able to phagocytize neighboring cells [Hardy et al. Citation1989]. We extend the phagocytic particles from autologous cells to fluorescence microspheres. To exclude the potential effects of aneuploidy on the development of blastocysts [Li et al. Citation2014; Treff et al. Citation2011], we included thalassemia-positive vitrified embryos in this study. Phagocytosis is a receptor-mediated event and a previous study showed that cryopreservation, connected to mitochondrial changes that serve as an energy supply, may attenuate the process of phagocytosis [Hansen et al. Citation1995; van der Meulen et al. Citation1981]. So, the phagocytosis observed in our study shows that the preimplantation trophectoderm cells do have the ability to phagocytize fluorescent microspheres at the stage of D6 and even D5. The internalization of the microspheres had occurred, rather than just tight binding to the outer cell surface, which was established by transmission electron microscopy. These findings are in agreement with reports of Rassoulzadegan et al. [Citation2000] where they have documented phagocytic ability that appeared after blastocyst cavity formation. In our present study, no phagocytic activity in the ICM was detected under TEM. We speculate that the cohesive epithelium of trophectoderm on the periphery of ICM may act as a physical barrier in preventing the entrance of microspheres.
Increased phagocytosed microspheres of D6 blastocysts in the present study suggest a more active phagocytic ability at the stage of D6 than that of D5. Human blastocyst implants in the endometrium approximately 7-9 days after fertilization [Norwitz et al. Citation2001]. Therefore, we suggest the phagocytic ability of blastocysts may be associated with embryo development and an increased trend of phagocytic activity may exist toward the time of human blastocyst implantation. In rodents, one ultrastructural study indicated that uterine epithelial cells in the attachment site undergo apoptotic changes leading to their phagocytosis by adjacent trophoblast cells during implantation [Parr et al. Citation1987]. In humans, the process of adhesion and penetration has not been elucidated. Some researchers tend to support instrusive implantation in which trophoblastic cytoplasmic extensions penetrate between intact epithelial cells [Bentin-Ley et al. Citation2000; Enders and Chlafke Citation1972]. But others suggested the human blastocyst may regulate endometrium epithelium apoptosis during implantation to breach the epithelial barrier [Galán et al. Citation2000]. This similarity to mice may be useful for the understanding of the behavior of trophectoderm phagocytosis concerning embryonic invasion in humans.
Phagocytosis of cell debris by trophectoderm was observed in our study. Phagocytosis provides a means by which cells clear particles and pathogens from their vicinity [DeMali and Burridge Citation2003]. The clearance of debris contributes to tissue health and homeostasis [Mao and Finnemann Citation2015]. Therefore, we propose the quick and efficient clearance of apoptotic cells is essential in embryonic development and for maintaining normal tissue homeostasis as well as remodeling.
Our preliminary results indicated that increased fluorescence signals were observed in polar trophectoderm of D6 blastocyst under a fluorescent microscope. The FluoSpheres® (Invitrogen, Shanghai, China) microspheres were in the state of aqueous suspensions by Brownian motion based on the manufacturer’s instructions. So blastocysts were maintained in the environment of high density of microspheres and exposed equally to microspheres. According to a previous study, in early blastocysts, there should be a band of evenly sized and distributed cells on the periphery of the embryo and a distinct area of dense cells, the ICM [Scott Citation2003]. In humans, ICM cells double between days 5 and 6, while the number of TE cells remains virtually unchanged [Hardy et al. Citation1989]. In our preliminary results, no phagocytic activity in the ICM was detected under TEM. Therefore, we consider the increased fluorescence signal may be due to the active phagocytic activity in polar TE cells. This suggested differentiation may exist between polar and mural trophectoderm. The variation of phagocytic activity along the polar-mural axis also exists in mice and it is controlled by fibroblast growth factor 4 (FGF4) secreted by inner cell mass [Rassoulzadegan et al. Citation2000]. However, FGF4 is not expressed in human preimplantation embryos [Yan et al. Citation2013]. This indicates the mechanism of phagocytosis in mice cannot be wholly extrapolated to similar events during human development. The results may reveal species variation. The evolutionary divergence in mouse and human might also lead to differences in the mechanism of phagocytosis and its regulation.
In summary, our results demonstrated that distinct phagocytic behavior exists in human trophectoderm before implantation. Moreover, trophectoderm phagocytic ability may increase with blastocyst development and vary between the polar and mural region of trophectoderm cells. However, due to the scarceness of human embryos and the inevitable embryo loss during TEM processing, the sample size was limited in our study. Whether human trophectoderm phagocytosis is a feature of differentiation, especially if it is connected to the biology of embryo implantation, is of interest and we will continue to study in depth. More studies are required to unravel the mechanism underlying this phenomenon.
Materials and methods
Embryo collection and vitrification
This research was performed on embryos of severe thalassemia or failed diagnosis donated by couples who submitted to preimplantation genetic diagnosis (PGD) treatments at the Reproductive Medicine Center of the First Affiliated Hospital of Sun Yat-sen University. All issues concerning the experimental setups and evaluation techniques have been approved by the ethical committee of the First Affiliated Hospital of Sun Yat-sen University. Specimens were collected from enrolled patients whose informed consent was obtained before their participation in this study.
Controlled ovarian stimulation was carried out for all patients by administering GnRH analogue (Dophereline, IPSEN, France) in a long protocol, followed by recombinant follicle stimulating hormone (FSH) (Gonal-F, SeronoSA, Switzerland). Biopsy procedure was performed on the morning of D3 at the cleavage stage with the mechanical approach as described previously [Verlinsky and Kuliev 2000]. PCR analysis for alpha or beta thalassemia was performed as we described previously [Deng et al. Citation2006]. We obtained the results of genetic diagnosis on the morning of D4 or D5. Thus, embryos diagnosed as severe thalassemia or failed of diagnosis were divided into D4 and D5 groups. Embryos with normal speed of development were selected [Familiari et al. Citation2006]. The D4 embryos were at the morula stage and compaction was completed, while the D5 embryos had morula cavitation and formation of the blastocoele. We collected embryos generated from 14 couples undergoing alpha-thalassemia PGD cycles and 16 couples undergoing beta-thalassemia cycles. The mean maternal age was 32. In total, 17 D4 embryos and 23 D5 blastocysts were vitrified, respectively, for the present study.
Co-culture of embryos and microspheres
Before warming of embryos, co-culture dishes (Falcon 1006, Heidelberg, Germany) were prepared with G2 culture medium (Vitrolife, Gothenburg, Sweden), supplemented with 1.0 µm diameter green carboxylate-modified fluorescent microspheres (excitation/emission: 505/515 nm, Invitrogen, Shanghai, China), to a final concentration of 10 μg/ml per droplet. The dishes were overlaid by 5 ml mineral oil (Vitrolife) and equilibrated overnight in triple-gas incubators (ASTEC, Japan) before use.
Only the survival D5 and D6 embryos after thawing were selected. These embryos were freed from zona pellucida (ZP) by 10 IU/ml pronase (Sigma, USA) at 25°C for 1.5 min. After ZP removal, D4 and D5 embryos were incubated individually in prepared co-culture dish overnight. Following overnight culture in vitro, embryos reached D5 and D6 stages, respectively. These blastocysts were washed twice with phosphate-buffered saline (PBS) and treated with 0.02% trypsin (Sigma) diluted by G2 media at 25°C for 5 min to quench the fluorescent microspheres just binding to the outer cell surface according to previous study [Rassoulzadegan et al. Citation2000].
Embryo evaluation
Two-hour extended culture in G2 media was needed for blastocyst re-expansion. Then embryos were evaluated by Gardner’s criteria before fixation [Gardner and Schoolcraft Citation1999]. Only high-quality blastocysts above 3BB were preserved for future use. For TEM, 11 D5 and 14 D6 blastocysts were fixed in the mixture of 2.5% glutaraldehyde and 2% paraformaldehyde. For fluorescence microscopy, D6 blastocysts were fixed in 4% paraformaldehyde. These samples were preserved at 4°C for future use.
TEM
For TEM, D5 and D6 blastocysts were washed in PBS three times for more than 10 min and fixed in 1% aqueous osmium tetroxide for 2 h. After being washed in PBS again for more than 10 min, they were dehydrated in serial dilutions of alcohols (50%, 70%, 80%, 90%, 100%) followed by 100% acetone. These blastocysts were embedded in the mixture of acetone and araldite (1:1) for 3 h and then in the pure araldite overnight before hardening at 60°C. Ultrathin (70 nm) sections were successfully obtained from 9 D5 and 11 D6 blastocysts. The sections were stained with alcoholic uranyl acetate followed by Reynold’s citrate solutions and finally examined by a Tecnai G2 Spirit Twin transmission electron microscope (FEI, Czech Republic).
Fluorescence microscopy
Phagocytosed microspheres were visualized by fluorescence microscopy. These aforementioned fixed D6 blastocysts were rinsed in PBS carefully to avoid shrinkage for more than10 min and mounted on the groove slides with 4, 6-diamidino-2-phenylindole (DAPI, Vector Labs, Peterborough, UK) in the dark for 15 min to stain the cell nuclei. Then samples were examined with an inverted fluorescence microscopy (Olympus IX71, Tokyo, Japan) fitted with specific filter cubes and the images of green microspheres as well as blue nuclei were collected, respectively, when corresponding filter cube was set.
Declaration of interest
All authors read and approved the final manuscript and declare no competing interests.
Acknowledgments
We are very grateful to people’s scientific advice and encouragement in the Reproductive Medical Center of Henan provincial People’s Hospital and the First Affiliated Hospital of Sun Yat-Sen University.
Additional information
Notes on contributors
Yan Li
Conceived the study and designed the experiments: YL, JX; Participated in the study design: G-LZ; Performed overall supervision: C-QZ, C-LZ; Performed the experiments: YL; Wrote the manuscript: YL, JX; All authors revised the manuscript and approved the final version.
Jian Xu
Conceived the study and designed the experiments: YL, JX; Participated in the study design: G-LZ; Performed overall supervision: C-QZ, C-LZ; Performed the experiments: YL; Wrote the manuscript: YL, JX; All authors revised the manuscript and approved the final version.
Can-quan Zhou
Conceived the study and designed the experiments: YL, JX; Participated in the study design: G-LZ; Performed overall supervision: C-QZ, C-LZ; Performed the experiments: YL; Wrote the manuscript: YL, JX; All authors revised the manuscript and approved the final version.
Cui-lian Zhang
Conceived the study and designed the experiments: YL, JX; Participated in the study design: G-LZ; Performed overall supervision: C-QZ, C-LZ; Performed the experiments: YL; Wrote the manuscript: YL, JX; All authors revised the manuscript and approved the final version.
Guang-lun Zhuang
Conceived the study and designed the experiments: YL, JX; Participated in the study design: G-LZ; Performed overall supervision: C-QZ, C-LZ; Performed the experiments: YL; Wrote the manuscript: YL, JX; All authors revised the manuscript and approved the final version.
References
- Baumbach, G.A., Bartley, N.G., Kattesh, H.G. and Godkin, J.D. (1990) Immunolocalization and endocytosis of the uterine secretory protein, uteroferrin, in pre-implantation pig trophectoderm on day 11 of pregnancy. Anat Embryol (Berl) 182:563-568.
- Bentin-Ley, U., Horn, T., Sjögren, A., Sorensen, S., Larsen, J.F. and Hamberger, L. (2000) Ultrastructure of human blastocyst-endometrial interactions in vitro. J Reprod Fertil 120:337-350.
- Bevilacqua, E., Hoshida, M.S., Amarante-Paffaro, A., Albieri-Borges, A. and Gomes, S.Z. (2010) Trophoblast phagocytic program: roles in different placental systems. Int J Dev Biol 54:495-505.
- Bevilacqua, E.M. and Abrahamsohn, P.A. (1988) Ultrastructure of trophoblast giant cell transformation during the invasive stage of implantation of the mouse embryo. J Morphol 198:341-351.
- Burton, G.J., Watson, A.L., Hempstock, J., Skepper, J.N. and Jauniaux, E. (2002) Uterine glands provide histiotrophic nutrition for the human fetus during the first trimester of pregnancy. J Clin Endocrinol Metab 87:2954–2959.
- Choy, M.Y. and Manyonda, I.T. (1998) The phagocytic activity of human first trimester extravillous trophoblast. Hum Reprod 13:2941-2949.
- DeMali, K.A. and Burridge, K. (2003) Coupling membrane protrusion and cell adhesion. J Cell Sci 116:2389-2397.
- Deng, J., Peng, W.L., Li, J., Fang, C., Liang, X.Y., Zeng, Y.H., et al. (2006) Successful preimplantation genetic diagnosis for alpha- and beta-thalassemia in China. Prenat Diagn 26:1021-1028.
- Drake, B.L. and Rodger, J.C. (1987) Phagocytic properties of cultured murine trophoblast. Placenta 8:129-139.
- Enders, A.C. and Chlafke, S. (1972) Implantation in the ferret: epithelial penetration. Am J Anat 133:291-315.
- Enders, A.C. and Schlafke, S. (1969) Cytological aspects of trophoblast uterine interaction in early implantation. Am J Anat 125:1-30.
- Familiari, G., Heyn, R., Relucenti, M., Nottola, S.A. and Sathananthan, A.H. (2006) Ultrastructural dynamics of human reproduction, from ovulation to fertilization and early embryo development. Int Rev Cytol 249:53-141.
- Finn, C.A. and Lawn, A.M. (1968) Transfer of cellular material between the uterine and trophoblast during the early stage of implantation. J Reprod Fertil 15:333-336.
- Flannagan, R.S., Jaumouillé, V. and Grinstein, S. (2012) The cell biology of phagocytosis. Annu Rev Pathol 7:61-98.
- Fu, Z., Wang, B., Wang, S., Wu, W., Wang, Q., Chen, Y., et al. (2014) Integral proteomic analysis of blastocysts reveals key molecular machinery governing embryonic diapause and reactivation for implantation in mice. Biol Reprod 90:52.
- Galán, A., O’Connor, J.E., Valbuena, D., Herrer, R., Remohı, J., Pampfer, S., et al. (2000) The human blastocyst regulates endometrial epithelial apoptosis in embryonic adhesion. Biol Reprod 63:430-439.
- Gardner, R.L. and Schoolcraft, W.B. (1999) In vitro culture of human blastocysts. In: Jansen R.; Mortimer D. (eds) Towards Reproductive Certainty: Fertility and Genetics Beyond. Parthenon Publishing, Carnforth, UK. pp. 378–388.
- Hansen, J.B., Halvorsen, D.S., Haldorsen, B.C., Olsen, R., Sjursen, H. and Kierulf, P. (1995) Retention of phagocytic functions in cryopreserved human monocytes. J Leukoc Biol 57:235-241.
- Hardy, K., Handyside, A.H. and Winston, R.M. (1989) The human blastocyst: cell number, death and allocation during late preimplantation development in vitro. Development 107:597-604.
- Hata, T., Ohkawa, K., Tomita, M. and Kishino, M. (1981) Phagocytosis of human cytotrophoblast cell invading into decidual tissue in early stage of gestation. Acta Obstet Gynaecol Jpn 33:537-544.
- Li, G., Jin, H., Xin, Z., Su, Y., Brezina, P.R., Benner, A.T., et al. (2014) Increased IVF pregnancy rates after microarray preimplantation genetic diagnosis due to parental translocations. Syst Biol Reprod Med 60:119-124.
- Manyonda, I.T. and Choy, M.Y. (1999) Collagen phagocytosis by human extravillous trophoblast: potential role in trophoblastic invasion. J Soc Gynecol Investig 6:158-166.
- Mao, Y. and Finnemann, S.C. (2015) Regulation of phagocytosis by Rho GTPases. Small GTPases 6:89-99.
- Metchnikoff, E. (1884) Über eine Sprosspilzkrankenheit der Daphnei: Betrag zur libre über den Kampf der Phagocyten gegen Darm krankheitserreger. Vischows Arch 96:177-185.
- Norwitz, E.R., Schust, D.J. and Fisher, S.J. (2001) Implantation and the survival of early pregnancy. N Engl J Med 345:1400-1408.
- Parr, E.L., Tung, H.N. and Parr, M.B. (1987) Apoptosis as the mode of uterine epithelial cell death during embryo implantation in mice and rats. Biol Reprod 36:221-225.
- Rassoulzadegan, M., Rosen, B.S., Gillot, I. and Cuzin, F. (2000) Phagocytosis reveals a reversible differentiated state early in the development of the mouse embryo. EMBO J 19:3295-3303.
- Red-Horse, K., Zhou, Y., Genbacev, O., Prakobphol, A., Foulk, R., McMaster, M., et al. (2004) Trophoblast differentiation during embryo implantation and formation of the maternal-fetal interface. J Clin Invest 114:744-754.
- Scott, L. (2003) The biological basis of non-invasive strategies for selection of human oocytes and embryos. Hum Reprod Update 9:237-249.
- Swanson, J.A. (2008) Shaping cups into phagosomes and macropinosomes. Nat Rev Mol Cell Biol 9:639-649.
- Treff, N.R., Northrop, L.E., Kasabwala, K., Su, J., Levy, B. and Scott, R.T.J. (2011) Single nucleotide polymorphism microarray-based concurrent screening of 24-chromosome aneuploidy and unbalanced translocations in preimplantation human embryos. Fertil Steril 95:1606-1612.
- Underhill, D.M. and Goodridge, H.S. (2012) Information processing during phagocytosis. Nat Rev Immunol 12:492-502.
- van der Meulen, F.W., Reiss, M., Stricker, E.A., van Elven,E. and von dem Borne, A.E. (1981) Cryopreservation of human monocytes. Cryobiology 18:337-343.
- Verlinsky, Y. and Kuliev, A. (2003) Current status of preimplantation diagnosis for single gene disorders. Reprod Biomed Online 7:145-150.
- Yan, L., Yang, M., Guo, H., Yang, L., Wu, J., Li, R., et al. (2013) Single-cell RNA-Seq profiling of human preimplantation embryos and embryonic stem cells. Nat Struct Mol Biol 20:1131-1139.