ABSTRACT
There is growing evidence that the spermatozoon’s epigenetic structure is of the utmost importance in the health of the future embryo. Following fertilization, sperm chromatin undergoes epigenetic reprogramming including DNA demethylation and remethylation, which resets gene expression. In some infertile patients, it is inevitable that sperm cells that are not within the range of normal human sperm parameters will be used for intracytoplasmic sperm injection. Understanding the relationship between the human sperm parameters and male pronucleus DNA demethylation seems necessary. We hypothesized that demethylation of the male pronucleus might be altered in zygotes conceived from a spermatozoa obtained from a sample exhibiting an abnormal semen analysis profile. To test the hypothesis, sperm cells from normal and abnormal human semen samples were injected into mouse oocytes. A group of cultured zygotes was fixed before the onset of DNA demethylation and the other group was fixed after DNA demethylation. Both groups were then labeled with a 5 methylcytosine antibody and the level of pronuclei methylation was detected as a function of fluorescent intensity. The level of demethylation was then determined as the difference between 5 methylcytosine fluorescent intensity before and after DNA demethylation. A negative correlation (p<0.05) was observed between sperm motility, morphology, percentage of head defects, protamine deficiency, and DNA demethylation level. However, no correlation was found between the demethylation level and sperm count. In conclusion, these observations suggest that demethylation is altered in the male pronucleus when low quality sperm samples are used.
Introduction
Infertility can be attributed to the male in one half of all cases and is associated with a variety of sperm abnormalities [Cassuto et al. Citation2012]. Intracytoplasmic sperm injection (ICSI) has been widely used in treatment of couples with male factor infertility [Oehninger et al. Citation1995]. There has always been a concern with respect to the risk of abnormalities in the children conceived by ICSI. One of the pathways that could raise the risk of birth defects is through the epigenetics of early embryogenesis [Carrell et al. Citation2015; Estill et al. Citation2016; Wen et al. Citation2012; Wen et al. Citation2010]. Epigenetic factors, including DNA methylation, histone modifications, and chromatin remodeling have been studied during fertilization and early embryonic development. It has been suggested that aberrant epigenetic changes in gametes may underlie some cases of congenital malformation [Carrell et al. Citation2015; Kitamura et al. Citation2015; Montjean et al. Citation2015; Wijers et al. Citation2015] and it has been suggested that sperm abnormalities are responsible for some of the epigenetic disorders [Anway and Skinner Citation2008; Benchaib et al. Citation2003; Oakes et al. Citation2007].
The sperm and oocyte are highly specialized cells and undergo a dramatic reprogramming as the totipotent zygote is formed after fertilization [Zhou and Dean Citation2015]. The sperm protamines are replaced by the maternal histones and then the paternal genome, which has been highly methylated during spermatogenesis is actively demethylated beginning in the pronuclear stage [Zhou and Dean Citation2015]. Active demethylation of the male pronuclear DNA plays a critical role in regulating the transcriptional programs during the embryo development and therefore the consequences of disrupted demethylation might be irreversible [Iqbal et al. Citation2011; Santos et al. Citation2002].
ICSI offers a useful therapeutic option to help couples with infertility. Generally, sperm parameters including count, motility, and morphology are used to evaluate semen samples. Sometimes complimentary semen tests are carried out to determine other sperm parameters such as sperm protamine deficiency and sperm DNA fragmentation. Recent data provide evidence that sperm global DNA methylation level are directly associated with semen parameters including concentration and motility [Montjean et al. Citation2015]. In our recently published work, a higher level of methylation was found in male pronuclei conceived from protamine deficient sperm cells compared to normal sperm cells [Rajabi et al. Citation2016]. The current study was conducted to investigate the importance of the role of DNA demethylation process on reprogramming in ICSI. The relationships between sperm parameters including count, motility, morphology, protamine deficiency, and the level of male pronuclear DNA demethylation following injection into the mouse oocyte were examined.
Results
To evaluate the influence of semen quality on male pronucleus demethylation, 20 human semen samples (8 normal and 12 abnormal) were analyzed and selected spermatozoon were injected into 248 mouse oocytes (98 normozoospermia, 18 oligozoospermia, 28 asthenozoospermia, 26 teratozoospermia, 78 oligoasthenoteratozoospermia). The experimental methods and the sources of analyzed data have been depicted in . A total of 195 oocytes survived after ICSI and were cultured. Following 3 hours and 8 hours after fertilization, 5-methylcytosine (5-mC) fluorescent intensity were determined (; ). There was a significant difference (p<0.05) between the DNA methylation level 3 hours and 8 hours after ICSI in all groups which was considered as the demethylated state.
Table 1. The number of injected oocytes and evaluated male pronuclei.
Figure 1. The experimental methods and the sources of analyzed data. ICSI: intracytoplasmic sperm injection; CMA3: chromomycin A3; IFS: immunofluorescent staining.
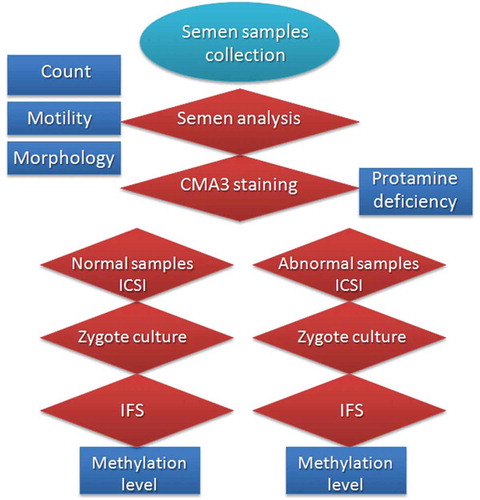
Figure 2. Fluorescent micrograph of zygotes labeled with 5-methylcytosine (5-mC). The pronuclei which are located near the secondary polar bodies, originated from oocytes and the others are the male pronuclei. (a) 3 hours after ICSI for normal semen sample, (b) 8 hours after ICSI for normal sample, (c) 3 hours after ICSI for low quality semen sample, and (d) 8 hours after ICSI for protamine deficient semen sample. Zygotes were also stained with Hoechst H33342, which are shown in the upper panels (corresponding uppercase letters).
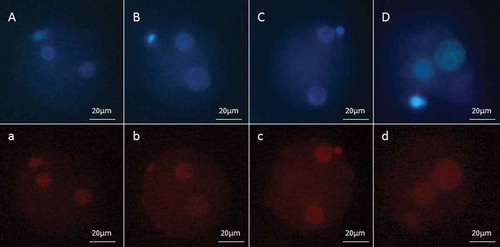
Count and motility
The samples with a concentration equal to or greater than 15 million spermatozoa per mL were considered normal [WHO Citation2010]. The mean sperm count was (62.49 ± 2.22) × 106 in normal and (11.8 ± 1.64) × 106 in the low concentration groups. The results of the study showed that at 3 hours post fertilization there were no differences (p>0.05) between the DNA methylation level in normal and low concentrated samples (). Similarly, no differences (p>0.05) were detected in the level of male pronuclear DNA methylation at 8 hours post fertilization. Also, no difference (p>0.05) was demonstrated between the demethylation level of the male pronuclei in normal and low concentrated samples (). Moreover, Pearson correlation analysis showed no relationship between sperm count and DNA methylation (; ).
Table 2. Number of male pronuclei and mean of fluorescent intensity of 5-mC in normal and abnormal groups.
Table 3. Relationship between sperm parameters and DNA methylation level.
Figure 3. Fluorescent intensity of 5-methylcytosine (5-mC) in male pronucleui, 3 and 8 hours after ICSI, and demethylation level. (A) pronuclei conceived from normal and low count semen samples, (B) pronuclei conceived from normal and low motility semen samples, (C) pronuclei conceived from normal and abnormal morphology semen samples, and (D) pronuclei conceived from normal and protamine deficient semen samples. *Significant difference between normal and abnormal groups.
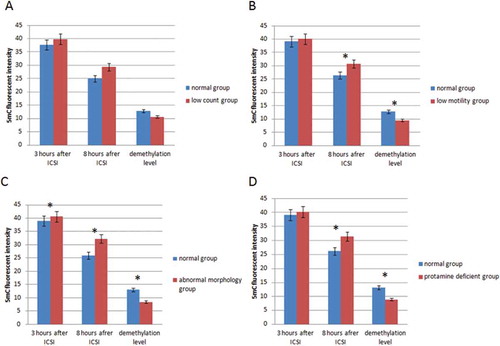
The WHO [Citation2010] reference limit for progressive motility is at least 32%. The semen samples with progressive motility greater than 32% were considered as normal and the semen samples with progressive motility less than 32%, abnormal. The mean of 5-mC fluorescent intensity, 3 hours after fertilization, was (39.1 ± 0.64) in the normal group and (40.07 ± 0.49) in the abnormal group was not different (p>0.05) (). In contrast, 8 hours after fertilization, significant differences in 5-mC fluorescent intensity (p<0.05) were detected when the normal (26.43 ± 0.53) and abnormal groups (30.65 ± 0.67; ) were compared. The DNA methylation level in the normal group was lower than in the abnormal group. Moreover, the level of demethylation was higher in the normal group compared with the lower motility abnormal group (p<0.05) (). Further as shown in and , there was a negative correlation between the DNA methylation and percentage of progressive motility.
Morphology and protamine
Semen samples with greater than or equal to 4% normal morphology forms were considered normal with less than 4% abnormal. The results showed that 5-mC fluorescent intensity was lower (p<0.05) 3 hours post fertilization in the normal group as compared to the abnormal group (). This trend between groups remained up to 8 hours post fertilization, (p<0.05) (). There was a negative correlation between the percent normal morphology sperm and male pronuclei DNA methylation showing that morphologically normal sperm were demethylated to a greater degree ().
In the present study, sperm samples were evaluated as a function of the different forms of human sperm abnormalities (head defects, neck and midpiece defects, tail defects, and excess residual cytoplasm). Pearson correlation analysis revealed that at 8 hours post fertilization there was a direct negative relationship (p<0.05) between the level of DNA methylation and head defects (), but this correlation was not observed (p>0.05) 3 hours after fertilization (). That is, the level of methylated DNA increased at 8 hours post fertilization as the level of normal forms decreased. No correlation was detected (p>0.05) between DNA methylation level and the other forms of sperm abnormalities (neck and midpiece defects, tail defects, excess residual cytoplasm), 3 hours and 8 hours after fertilization (; ).
At 3 hours after fertilization, the level of DNA methylation level was not different when the normal and protamine deficient groups were compared (p>0.05). In contrast, 8 hours after fertilization, a difference (p<0.05) was observed in DNA methylation between normal and protamine deficient groups. The level of methylation was higher in the protamine deficient group as compared to the normal group. Also, the level of demethylation in protamine deficient samples was lower than in normal samples (). Correlation analysis showed a negative relationship between protamine deficiency and demethylation level (; ).
Discussion
ART is responsible for the birth of more than 200,000 babies each year [Aitken Citation2008]. The studies have shown that the children born as a consequence of ART are at a higher risk for birth defects as compared to natural pregnancy [Hansen et al. Citation2005; Loke and Craig Citation2016; Reefhuis et al. Citation2009]. Cytosine methylation is a key part of the epigenetic code [Hochedlinger and Jaenisch Citation2006; Iqbal et al. Citation2011]. Following fertilization, the paternal genome undergoes DNA demethylation [Iqbal et al. Citation2011; Santos et al. Citation2002], as part of its epigenetic reprogramming for subsequent gene expression [Santos et al. Citation2002; Simonsson and Gurdon Citation2004; Surani et al. Citation2007; Wu and Zhang Citation2010]. There are some reports of imprinting errors and other epigenetic anomalies in couples treated with ICSI which may reflect abnormal patterns of DNA methylation [Dong et al. Citation2016; Sanchez Albisua et al. Citation2007].
Interspecies fertilization was used as a surrogate to assess the importance of sperm quality (sperm parameters) on early development following ICSI as a function of DNA demethylation. Our observations showed a significant decrease for the level of methylation at 3 hours to 8 hours post ICSI fertilization. This is consistent with the timing shown in previous studies [Iqbal et al. Citation2011; Santos et al. Citation2002; Wossidlo et al. Citation2010]. Our results revealed that sperm count was not significantly correlated with the level of DNA methylation at 3 or 8 hours post fertilization. Thus demethylation was independent of sperm concentration consistent with previous studies [Rajabi et al. Citation2016].
Houshdaran et al. (Citation2007) have previously studied the relationship between sperm epigenetic defects with semen parameters. They reported that methylation levels were elevated in some sequences of DNA from low motile and poor quality sperm samples [Houshdaran et al. Citation2007]. Another report by Oakes et al. [Citation2007] showed that 5-aza-2′-deoxycytidine, an anticancer agent, increases DNA hypomethylation, which leads to altered sperm morphology and decreased sperm motility [Oakes et al. Citation2007]. These studies showed that there could be a relationship between DNA methylation and sperm motility. In the study presented above the assessment of the level of DNA methylation in male pronuclei 3 hours after fertilization was not dependent on motility until 8 hours post fertilization. At this time the level of DNA methylation in the normal group was lower than the abnormal group, indicating that the DNA demethylation appeared more complete in the male pronucleus conceived from normal motility sperm, compared to abnormal sperm. This is consistent with previous studies suggesting that motility could be a marker for DNA methylation defects.
The quality of sperm development during spermiogenesis is reflected in the shape of the spermatozoa. Therefore, a defect in sperm maturation can be mirrored in sperm morphology [Ghasemian et al. Citation2016]. At the present time, ICSI has been implemented for patients with normal, mildly, or severely abnormal semen parameters [Babayev et al. Citation2014]. Blahová et al. [Citation2014] found that there is a relationship between abnormal sperm and embryo morphology at later stage cleavage. In regard to the importance of sperm morphology on subsequent development, we compared the level of male pronuclear DNA demethylation from semen samples with normal and abnormal morphology. Our observations indicate that although some abnormal spermatozoa are capable of forming the pronucleus, the level of DNA demethylation is less than normal pronucleus. This suggests that when a spermatozoon with a defective head is used the male pronucleus may show a decreased level of DNA demethylation.
The protamines are small basic proteins that are synthesized in the late stage spermatids and bind to DNA, condensing the chromatin into a genetically inactive state [Balhorn Citation2007]. It is well established that there is a relationship between protamine deficiency and sperm DNA damage which leads to male infertility [Ni et al. Citation2016; Rajabi et al. Citation2016]. Our pervious study revealed that, 6 hours after fertilization, protamine deficient semen samples have a higher level of 5-mC in the male pronucleus [Rajabi et al. Citation2016]. In this study, we compared fluorescent intensity of 5-mC before and after onset of DNA demethylation. The results showed that 3 hours after ICSI, which is before DNA demethylation, there is no significant difference between normal and protamine deficient semen samples. Surprisingly, our data revealed that 8 hours after ICSI, which is subsequent to active DNA demethylation 5-mC fluorescent intensity was higher in pronucleus conceived from a protamine deficient spermatozoon as compared to that obtained from normal sperm. This means that in the protamine deficient group the male pronucleus retained somewhat of a DNA demethylated or relatively hypermethylated state. Different studies have shown that during spermiogenesis, sperm chromatin becomes highly methylated, prior to replacing histones by protamines [Bao and Bedford Citation2016]. Since the extensive DNA methylation occurs earlier than the installation of protamines as part of sperm chromatin, it suggests that cytosine methylation is independent of histone-protamine exchange.
Previous investigations have documented that shortly after fertilization, protamines exchange with oocyte histones and then male pronucleus undergoes DNA demethylation [McLay and Clarke Citation2003; Morgan et al. Citation2005; Zhou and Dean Citation2015]. Also, it has been shown that the male pronucleus requires specific histone modifications along with a receptive chromatin configuration for demethylation [Montellier et al. Citation2013]. This receptive chromatin is probably distorted in the male pronucleus formed from a protamine deficient spermatozoon. This is consistent with our finding of a higher level of methylation in the protamine deficient group 8 hours after ICSI.
In summary, this study provided evidence that the level of demethylation is altered in low quality semen samples, which are sometimes used for ICSI treatment. This suggests that it is necessary to develop accurate gamete selection techniques and apply complementary tests to evaluate the epigenetic status of a semen sample. We should keep long term monitoring of the health of children produced by ICSI.
Material and methods
All the materials were purchased from Sigma Chemical Corporation (St. Louis, MO, USA) except where otherwise specified. This project was approved by the ethics committee of Shahid Beheshti University of Medical Sciences, Tehran, Iran.
Sperm preparation
Semen analysis was performed in the in vitro fertilization department of Erfan hospital which is under the supervision of the Ministry of Health, Treatment and Medical Training quality control program. We analyzed semen samples from fertile and infertile male volunteers who had been referred to the infertility center (n=20, 8 normozoospermia, 1 oligozoospermia, 2 asthenozoospermia, 2 teratozoospermia, 7 oligoasthenoteratozoospermia).
The specimens were obtained by masturbation after 2-3 days sexual abstinence and poured into sterile containers. The samples were liquefied within 15-30 min at room temperature. Four hundred spermatozoa were assessed for each parameter and semen parameters including count, motility, and morphology were analyzed according to WHO guidelines [WHO Citation2010]. The lower reference limits were considered 15 × 106 for sperm count, 32% for progressive motility and 4% for normal morphology [WHO Citation2010].
Sperm cells were assessed in two replicate slide from each sample and the percentage of all abnormal forms of spermatozoa including spermatozoa with head defects, midpiece defects, tail defects, and those with excess residual cytoplasm were determined. One hundred µl of the liquefied semen was fixed to evaluate protamine deficiency, using chromomycin A3 staining (CMA3) [Rajabi et al. Citation2016]. The samples were considered normal with equal or less than 30% and protamine deficient with more than 30%. Then a fraction of each sample was washed with Ham’s F10 medium containing 10% human serum albumin (HSA), and used for ICSI.
Oocyte preparation and intracytoplasmic sperm injection
Hybrid B6D2F1 (BDF1) female mice (Pasteur Institute, Tehran, Iran) were superovulated by intraperitoneal injection with 10 IU pregnant mare serum gonadotropin (PMSG), followed by 10 IU human chorionic gonadotropin (HCG) 48 h later. Oocytes were collected from oviduct ampullae 13-14 h after injection of the HCG in flushing holding medium (FHM), and cumulus cells were removed from oocytes with 1% hyaluronidase treatment [Dehghani-Mohammadabadi et al. Citation2014]. After washing in FHM medium, meiosis II oocytes with normal morphology were stored in a potassium simplex optimized medium (KSOM) in an incubator at 37°C with 5% CO2 within the maximum period of 2 h until ICSI. Spermatozoa were injected into oocytes with a PMM-150FU Piezo-actuated micromanipulator (Prime Tech Ltd, Tsuchikura, Japan) at room temperature [Fulka et al. Citation2008]. The surviving oocytes were divided in two groups. One of which was cultured for 3 h and the other for 8 h in a KSOM medium in the incubator. Then, pronuclei formation was evaluated with an inverted microscope. The pronucleus which was located near the secondary polar body, originated from the oocyte and the other one was the male pronucleus (). It has been shown that, within 4 h post fertilization, the male pronucleus undergoes considerable demethylation and within 8 h, it seems almost completely demethylated. This span of time was selected to study the demethylation level and the difference between 5-methylcytosine fluorescent intensity 3 h and 8 h after ICSI was determined as demethylation level.
Immunofluorescence staining
Zygotes with two, male and female, pronuclei were washed in phosphate buffered saline (PBS), fixed for 30 min in 4% paraformaldehyde in PBS, and permeabilized with 0.2% Triton X-100 in PBS for 30 min at room temperature [Fulka et al. Citation2008]. The samples were treated with HCl (2M) for 30 min, then neutralized for 15 min with Tris HCl (pH=8), washed several times with 1% Bovine Serum Albumin (BSA), 0.1% Tween 20 in PBS, and blocked overnight at 4°C in 2% BSA in PBS. The zygotes were incubated for 1 h at 37°C with monoclonal antibody Anti 5-mC (Epigentek, NY, USA) (1:100 dilution) and a secondary antibody coupled with propidium iodide (PI) at 37°C [Fulka et al. Citation2008], and finally were stained with Hoechst H33342. The samples were mounted in glycerol, assessed under fluorescent microscope, (Nikon, Japan) and portrayed. The pronucleus which was located far from the polar body was considered as male pronucleus [Fulka et al. Citation2008].
Observations were performed with a fluorescent microscope and pictures were taken with a digital camera (Cannon, Japan). To evaluate the level of male pronuclei DNA methylation the photographs were analyzed with Image-J software (Version 1.45 s; National Institutes of Health, USA) software. To do so, the fluorescent intensity of the male pronuclei area (A) and the fluorescent intensity of the surrounding area (B) were measured and the fluorescent intensity of male pronuclei calculated by taking away B from A. The difference between 5-methylcytosine fluorescent intensity 3 hours and 8 hours after ICSI was considered as the demethylation level.
Experimental design
During this study, the effect of sperm parameters was evaluated on the demethylation of male pronucleus. Accordingly, semen samples obtained from fertile (normal samples) and infertile (abnormal samples) men were analyzed and sperm parameters including count, motility, morphology, and protamine deficiency were determined in each sample. A sample was considered normal when all sperm parameters were in normal range and considered abnormal when at least one parameter was not in normal range. In most of the abnormal semen samples more than one form of abnormality were detected. A fraction of each sample was prepared and injected to mouse oocytes. A group of zygotes were cultured for 3 h and another group was cultured for 8 h. Subsequently, both groups were fixed, stained with 5-mC, and the methylation level of male pronuclei were determined in all zygotes. For each semen sample, the difference between 5 methylcytosine fluorescent intensity 3 h and 8 h after ICSI was considered as demethylation level.
Statistical analysis
The statistical analysis was carried out using SPSS Ver.20 software (SPSS, Chicago, IL, USA). The means of fluorescent intensity of 5-methylcytosine were compared by a nonparametric analysis test (Mann Whitney). Comparison between parameters was performed using a correlation co-efficient (Pearson correlation). P<0.05 was considered statistically significant.
Declaration of interest
This study was funded by Kharazmi University and Stem Cell Technology Research Center, Tehran, Iran. The authors declare no potential conflicts of interest with respect to the authorship and publication of the article. Also, we declare that none of the authors are otherwise employed in any respect by the Government of Iran.
Acknowledgments
We would like to thank Dr. Abdollah Mohammadi-Sangcheshmeh for his extraordinary support in this project. We are also indebted to Mr. Craig Wilson for language editing the manuscript.
Additional information
Notes on contributors
Hoda Rajabi
Carried out the data collection, performed the study, and wrote the manuscript: HR; Supervised the analysis: HM-K; Helped in the study coordination: MS, FF-N; Took part in data collection: TE-A.
References
- Aitken, R.J. (2008) Just how safe is assisted reproductive technology for treating male factor infertility? Expert Rev Obstet Gynecol 3:267-271.
- Anway, M.D. and Skinner, M.K. (2008) Epigenetic programming of the germ line: effects of endocrine disruptors on the development of transgenerational disease. Reprod Biomed Online 16:23-25.
- Babayev, S.N., Park, C.W. and Bukulmez, O. (2014) Intracytoplasmic sperm injection indications: how rigorous? Semin Reprod Med 32:283-290.
- Balhorn, R. (2007) The protamine family of sperm nuclear proteins. Genome Biol 8:1.
- Bao, J. and Bedford, M.T. (2016) Epigenetic regulation of the histone-to-protamine transition during spermiogenesis. Reproduction 151:R55–R70.
- Benchaib, M., Ajina, M., Lornage, J., Niveleau, A., Durand, P. and Guérin, J.F. (2003) Quantitation by image analysis of global DNA methylation in human spermatozoa and its prognostic value in in vitro fertilization: a preliminary study. Fertil Steril 80:947-953.
- Blahová, E., Máchal, J., Máchal, L., Milaković, I. and Hanuláková, Š. (2014) Eliminating the effect of pathomorphologically formed sperm on resulting gravidity using the intracytoplasmic sperm injection method. Exp Ther Med 7:1000-1004.
- Carrell, D.T., Nyboe Andersen, A. and Lamb, D. (2015) The need to improve patient care through discriminate use of intracytoplasmic sperm injection (ICSI) and improved understanding of spermatozoa, oocyte and embryo biology. Andrology 3:143-146.
- Cassuto, N.G., Hazout, A., Hammoud, I., Balet, R., Bouret, D., Barak, Y., et al. (2012) Correlation between DNA defect and sperm-head morphology. Reprod Biomed Online 24:211-218.
- Dehghani-Mohammadabadi, M., Salehi, M., Farifteh, F., Nematollahi, S., Arefian, E., Hajjarizadeh, A., et al. (2014) Melatonin modulates the expression of BCL-xl and improve the development of vitrified embryos obtained by IVF in mice. J Assist Reprod Gen 31:453-461.
- Dong, H., Wang, Y., Zou, Z., Chen, L., Shen, C., Xu, S., et al. (2016) Abnormal Methylation of Imprinted Genes and Cigarette Smoking Assessment of Their Association With the Risk of Male Infertility. Reprod Sci 2016 May 31: pii (Epub ahead of print).
- Estill, M.S., Bolnick, J.M., Waterland, R.A., Bolnick, A.D., Diamond, M.P. and Krawetz, S.A. (2016) Assisted reproductive technology alters deoxyribonucleic acid methylation profiles in bloodspots of newborn infants. Fertil Steril 106:629-639.
- Fulka, H., Barnetova, I., Mosko, T. and Fulka, J. (2008) Epigenetic analysis of human spermatozoa after their injection into ovulated mouse oocytes. Hum Reprod 23:627-634.
- Ghasemian, F., Mirroshandel, S.A., Monji-Azad, S., Azarnia, M. and Zahiri, Z. (2016) Corrigendum to “An efficient method for automatic morphological abnormality detection from human sperm images”[Comput Methods Programs Biomed 122 (2015) 409–420]. Comput Methods Programs Biomed 129:217.
- Hansen, M., Bower, C., Milne, E., de Klerk, N. and Kurinczuk, J.J. (2005) Assisted reproductive technologies and the risk of birth defects—a systematic review. Hum Reprod 20:328-338.
- Hochedlinger, K. and Jaenisch, R. (2006) Nuclear reprogramming and pluripotency. Nature 441:1061-1067.
- Houshdaran, S., Cortessis, V.K., Siegmund, K., Yang, A., Laird, P.W. and Sokol, R.Z. (2007) Widespread epigenetic abnormalities suggest a broad DNA methylation erasure defect in abnormal human sperm. PloS One 2:e1289.
- Iqbal, K., Jin, S.-G., Pfeifer, G.P. and Szabó, P.E. (2011) Reprogramming of the paternal genome upon fertilization involves genome-wide oxidation of 5-methylcytosine. Proc Natl Acad Sci 108:3642-3647.
- Kitamura, A., Miyauchi, N., Hamada, H., Hiura, H., Chiba, H., Okae, H., et al. (2015) Epigenetic alterations in sperm associated with male infertility. Congenit Anom 55:133-144.
- Loke, Y.J. and Craig, J.M. (2016) Are the effects of IVF on DNA methylation driven by intracytoplasmic sperm injection and male infertility? Epigenomics 8:881-884.
- McLay, D.W. and Clarke, H.J. (2003) Remodelling the paternal chromatin at fertilization in mammals. Reproduction 125:625-633.
- Montellier, E., Boussouar, F., Rousseaux, S., Zhang, K., Buchou, T., Fenaille, F., et al. (2013) Chromatin-to-nucleoprotamine transition is controlled by the histone H2B variant TH2B. Genes Dev 27:1680-1692.
- Montjean, D., Zini, A., Ravel, C., Belloc, S., Dalleac, A., Copin, H., et al. (2015) Sperm global DNA methylation level: association with semen parameters and genome integrity. Andrology 3:235-240.
- Morgan, H.D., Santos, F., Green, K., Dean, W. and Reik, W. (2005) Epigenetic reprogramming in mammals. Hum Mol Gen 14:R47–R58.
- Ni, K., Spiess, A.N., Schuppe, H.C. and Steger, K. (2016) The impact of sperm protamine deficiency and sperm DNA damage on human male fertility: a systematic review and meta‐analysis. Andrology 4:789-799.
- Oakes, C.C., Kelly, T.L., Robaire, B. and Trasler, J.M. (2007) Adverse effects of 5-aza-2′-deoxycytidine on spermatogenesis include reduced sperm function and selective inhibition of de novo DNA methylation. J Pharm Exp Ther 322:1171-1180.
- Oehninger, S., Veeck, L., Lanzendorf, S., Maloney, M., Toner, J. and Muasher, S. (1995) Intracytoplasmic sperm injection: achievement of high pregnancy rates in couples with severe male factor infertility is dependent primarily upon female and not male factors. Fertil Steril 64:977-981.
- Rajabi, H., Mohseni-Kouchesfehani, H., Mohammadi-Sangcheshmeh, A., Farifteh-Nobijari, F. and Salehi, M. (2016) Pronuclear epigenetic modification of protamine deficient human sperm following injection into mouse oocytes. Syst Biol Reprod Med 62:125-132.
- Reefhuis, J., Honein, M., Schieve, L., Correa, A., Hobbs, C. and Rasmussen, S. (2009) Assisted reproductive technology and major structural birth defects in the United States. Hum Reprod 24:360-366.
- Sanchez Albisua, I., Borell Kost, S., Mau Holzmann, U., Licht, P. and Krägeloh Mann, I. (2007) Increased frequency of severe major anomalies in children conceived by intracytoplasmic sperm injection. Dev Med Child Neurol 49:129-134.
- Santos, F., Hendrich, B., Reik, W. and Dean, W. (2002) Dynamic reprogramming of DNA methylation in the early mouse embryo. Dev Biol 241:172-182.
- Simonsson, S. and Gurdon, J. (2004) DNA demethylation is necessary for the epigenetic reprogramming of somatic cell nuclei. Nat Cell Biol 6:984-990.
- Surani, M.A., Hayashi, K. and Hajkova, P. (2007) Genetic and epigenetic regulators of pluripotency. Cell 128:747-762.
- Wen, J., Jiang, J., Ding, C., Dai, J., Liu, Y., Xia, Y., et al. (2012) Birth defects in children conceived by in vitro fertilization and intracytoplasmic sperm injection: a meta-analysis. Fertil Steril 97:1331–1337. e1334.
- Wen, S.W., Leader, A., White, R.R., Léveillé, M.-C., Wilkie, V., Zhou, J., et al. (2010) A comprehensive assessment of outcomes in pregnancies conceived by in vitro fertilization/intracytoplasmic sperm injection. Eur J Obstet Gynaecol Reprod Biol 150:160-165.
- WHO (2010) WHO laboratory manual for the examination and processing of human semen. 5th edition. Geneva: World Health Organization.
- Wijers, C.H., van Rooij, I.A., Rassouli, R., Wijnen, M.H., Broens, P.M., Sloots, C.E., et al. (2015) Parental Subfertility, Fertility Treatment, and the Risk of Congenital Anorectal Malformations. J Epidemiol 26:169–176.
- Wossidlo, M., Arand, J., Sebastiano, V., Lepikhov, K., Boiani, M., Reinhardt, R., et al. (2010) Dynamic link of DNA demethylation, DNA strand breaks and repair in mouse zygotes. EMBO J 29:1877–1888.
- Wu, S.C. and Zhang, Y. (2010) Active DNA demethylation: many roads lead to Rome. Nat Rev Mol Cell Biol 11:607-620.
- Zhou, L.-q. and Dean, J. (2015) Reprogramming the genome to totipotency in mouse embryos. Trends Cell Biol 25:82–91.