ABSTRACT
Androgen mediating signaling is implicated in regulating the expression of reproductive related genes. Any deviation in the gene expression might be the ignitable precursor for genomic instability that inflames the genomic rearrangements and a leading cause of cancer. The main goal of this study was to determine the relationships between trinucleotide repeats of androgen receptor (AR), sex steroids, and sexual function in men presenting with reduced sperm motility. We investigated the singleton or combinatorial effects of the length of trinucleotide repeats of AR on reproductive hormones, clinical parameters, semen analyses, as well as sexual assessment function of 210 asthenospermic outpatients along with 125 normal subjects. Sexual assessment was executed using the International Index of Erectile Function (IIEF-15 score) which measures erectile function (EF), orgasmic function (OR), sexual desire (SD), intercourse satisfaction (IS), and overall satisfaction. Our findings suggest that long (>26 CAG)n repeats have an inverse correlation with circulatory FSH and T, whereas long (>25 GGC)n repeats have moderated affiliation with reduced sperm concentration. The study revealed a novel finding by exploring the negative correlation between elongated (CAG)n repeats and the cumulative IIEF-15 score, orgasm function (OR), and erectile function (EF) in asthenospermic men. This study examines the tri-nucleotide correlation with sexual function in Punjabi men enhancing our understanding of the regulatory mechanisms of sexual performance.
Abbreviations: AR: androgen receptor; IIEF-15 score: International Index of Erectile Function; EF: erectile function; OR: orgasmic function; SD: sexual desire; IS: intercourse satisfaction; FSH: follicular stimulating hormone; T: testosterone; NTD: N-terminal transactivation domain; DBD: DNA-binding domain; LBD: ligand binding domain; TNR: tri-nucleotide repeat
Introduction
The androgen receptor (AR), i.e., NR3C4 ligand-gated transcription factor, belongs to nuclear receptor subfamily 3, group C and is member 4. It is a cornerstone in a suite of genetically complex diseases [Matsumoto et al. Citation2013]. The 90 kb - 8 exon 919 amino acid human androgen receptor gene is located on the long arm of the X chromosome at Xq 11-12. Previous studies have shown that the androgen receptor has a dynamic role, regulating differentiation and cell growth. Structurally, the AR protein has three distinct functional domains: i) a hypervariable N- terminal highly flexible transactivation domain (NTD) that comprises approximately 60% of AR protein [Mostaghel et al. Citation2014]; ii) a highly conserved central DNA-binding domain (DBD); and iii) a hinge region (HR) and C- terminal ligand binding domain (LBD). Plasticity and flexibility are two major characteristics of the LBD [Bousnane et al. Citation2017]. This cofactor binding groove provides a ligand induced protein-protein interaction surface that has an ability to interact with the FxxLF motif of NTD. This plays a dynamic role in regulating the folding and structuring of the NTD [Sakkiah et al. Citation2016]. Transcriptional modulation targets the AF-1 pocket of NTD, facilitating a hormone dependent positive interaction with the AF-2 pocket in the LBD of AR [Kalkan et al. Citation2016].
Androgen receptor mediating signaling is triggered by the binding of endogenous testosterone (T) or dihydrotestosterone. This is a primary element in regulating embryonic sexual differentiation, growth, maturation of secondary sexual phenotypes, as well as normal spermatogenesis. Mechanistic down regulation of endogenous androgen is one cause of abnormal and defective spermatogenesis [Giagulli et al. Citation2014]. The broad distribution of the AR permits a wide-range of tissue targets guiding various processes throughout the body. It initiates an intra-cytoplasmic cascade of reactions governed by the dissociation of accessory proteins. These successively increase transcription initiation of androgen responsive genes [Shiraishi and Matsuyama Citation2017]. AR is extensively communicates and radiates androgen signals among the various body tissues, including mitotically active hotspot regions, especially sustentacular sertoli cells, prostate cells, seminal vesicle, keratinocytes, melanocytes, hepatocytes, adipocytes, myocytes, and neuronal cells [O’Hara and Smith Citation2015]. AR mutations are responsible for deleterious overwhelming effects on spermatogenesis markedly down-regulating a vast systematic array of genes and their co-regulators [Li et al. Citation2017a] .
AR receptor displays two polymorphic hyper-expansions in the N-terminal domain (TAD) of exon 1. These are characterized by e-signatures of the varied copy number of (CAG)n and (GGC)n repeats transcribed into different numbers of poly-glutamine (poly-Q) and poly-glycine (poly-G), respectively [Querin et al. Citation2017]. The (CAG)n repeats are 12 to 36 in length that have an average of 22 residues, whereas (GGC)n tract harbors 10 to 27 repeats with a mean of 23 residues. Several studies have already been conducted to estimate the association between (GGC)n and (CAG)n stretches in relation with male infertility, but remain provocative [Pastuszak et al. Citation2012; Andersen et al. Citation2011].
The most updated meta-analysis suggests a significant association between long (CAG)n stretches of infertile men [Peng et al. Citation2014]. In comparison, short stretches of (CAG)n have a low probability of male infertility. These trinucleotides repeats have been associated with decreased AR N-terminal domain activity and signify a high risk of male infertility [Li et al. Citation2017b]. This outcome might be a result of an ethnic dissimilarity provoking in the microsatellite polymorphism of (CAG)n repeats. It has been noted in previous studies that (CAG)n repeats are of short span in Africans [Wang et al. Citation2005], intermediate order in Caucasians [Hickey et al. Citation2002], and excessively long in Asians [Thangaraj et al. Citation2002]. The extensive degree of variation in the number of repeats might be a consequence of DNA polymerase slippage at the site of the replication fork. Previous data suggests that longer poly-amino acid tracts down regulate AR activity which causes pubertal infertility in males, whereas shorter tracts parallel over expression that leads to tumorigenesis [Shimada et al. Citation2016].
Population based studies have suggested that phenotypic expansion of (CAG)n stretches are directly linked with male infertility in Caucasians, [Eendebak et al. Citation2016], Chinese [Hsing et al. Citation2000], and Americans [Ingles et al. Citation1997], but not in Egyptians [Younes et al. Citation2016]. Additional meta-analysis revealed a high potential risk of aggressive prostate cancer, associated with short (CAG)n stretches prior to their expansion. The potential role of (GGC)n repeats in transcriptomes that may lead to testicular cancer has been proposed [Weng et al. Citation2017]. However, they remain to be characterized with respect to male infertility and its functional consequences on clinical parameters. To address these issues the effect of gonadal hormones, sexual function, and the correlation of semen parameters in infertile asthenospermic men (n = 210; aged 25-45 years) were examined as a function of (CAG)n and (GGC)n AR repeats.
Results and discussion
One of the important role of the AR receptor is to maintain the integrity of male phenotype and spermatogenesis through T regulation [Levin and Hammes Citation2016]. A key component resides between amino acid residues141 and 338, the transactivation domain (TAD). This is highly polymorphic and inherent variations therein may affect androgen receptor mediated gene regulation of androgen receptor responsive elements/genes. The extent of variation of AR size is in part because polymorphic trinucleotide repeats reside in the TAD within the first exon.
Tri-nucleotide repeat (TNR) status in asthenospermic men
AR (CAG)n and (GGC)n repeats are within a very short distance (~1000bp) of each other, on the opposite junctions of exon 1 of the androgen receptor gene. The characteristics of an infertile and fertile cohort have been summarized in , in a standardized format [Traven et al. Citation2017]; sequence analysis revealed a total of 21 (CAG)n and 17 (GGC)n allelic variants, ranging from 9-28 and 10-28 repeats, respectively. The mean number of (CAG)n repeats were 22 in case subjects and 23 in control subjects, while the mean number of (GGC)n repeats were 21 and 22 in case and control subjects, respectively (). The total distribution of (CAG)n and (GGC)n stretches were not significantly different, despite the fact that they are positively correlated. It has been observed that AR with long (CAG)n stretches tend to have longer (GGC)n stretches.
Table 1. Characteristics of the infertile (N = 210) and fertile(N = 125) cohort.
Table 2. Distribution of (CAG)n and (GGC)n repeats of androgen receptor in infertile and fertile subjects.
The smallest allele size was (CAG)n 9, observed in asthenospermic men at a frequency of 1.90%. The most frequent (CAG)n allele observed in the fertile (18.57%) and infertile men (14.28%) was 23. We also observed that (GGC)n 22 was the leading allele (17.14%) among asthenospermic men, while the fertile subjects exhibited an extensively high ratio (27.2%) of (GGC)n 23 allele. Moreover, some (CAG)n alleles (9, 13, and 14) were only observed in the asthenospermic subjects. In comparison the highest repeat size 33, was observed at a very low frequency in both asthenospermic (0.95%) and normal fertile men (0.8%). Comparatively, the (GGC)n allele portrays a somewhat different scenario among asthenospermic men, exhibiting a low frequency of alleles 10,12,13,15 and a high frequency (4.76% and 3.33%) 18 and 19 alleles whereas, allele 18 and 19 were completely absent in normal fertile subjects.
Correlation of tri-nucleotide repeats (TNR) and asthenospermic male reproductive hormonal profile
TNR, FSH, and LH correlations were examined using bivariate graph analysis and are summarized in . The abscissa presents a potential score of the TNR and Y as a function of FSH and LH concentration. The graph revealed a significant negative linear relationship among (CAG)n, FSH and LH concentration (ρ = 0.003, β coefficient = -0.56). Longer (≥26) AR (CAG)n repeats were associated with a lower serum FSH level and also present a weak but significantly negative correlation with T (ρ = 0.001, β coefficient = -0.38) (). A similar negative association is observed for longer (>25 GGC)n repeats and sperm concentration ().
Table 3. Clinical, biochemical, and genetic parameters of infertile and normal fertile men based on androgen receptor (CAG)n repeats.
Figure 1. Long repeats of (CAG)n and (GGC)n of androgen receptors are associated with male reproductive hormonal parameters in Punjabi men. A) Scatter plot exhibited a weak but significant negative correlation between longer (CAG)n repeats of AR and total testosterone (T) concentration (ng/ml). B) Scatter plot statistically exhibited a negative correlation between sperm concentration (mill/ml) and longer (GGC)n repeats of androgen receptor in asthenospermic men.
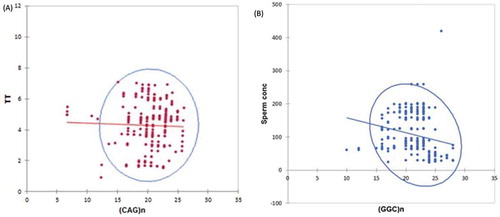
Correlation of sexual performance and elongated (CAG)n repeats
It is noteworthy that age presented a negative correlation with the overall International Index of Erectile Function (IIEF-15 score). However, all sexual parameters exhibited a positive correlation between total T and estradiol levels in asthenospermic men. Correspondingly the (CAG)n repeat length was inversely related to the total IIEF-15 score, whereas (GGC)n alleles showed no significant relationship with sexual parameters ().
Table 4. Clinical, biochemical, and genetic parameters of infertile and normal fertile men based on androgen receptor (GGC)n repeats.
Cluster analysis (CA) separated the parameters into three clusters (). Cluster I: International index of Erectile Function-15 score, EF, IS, OS, SD; Cluster II: Sperm concentration, Sperm count; LH, FSH, TT, BMI, Age, Estradiol, SHBG; and Cluster III: (CAG)n, (GGC)n repeats. Principal component analysis (PCA) emphasized a positive significant correlation between (CAG)n and (GGC)n repeats, while, sperm count showed a close association with (GGC)n repeats rather than (CAG)n repeats ().
Figure 2. Clustering dendrogram (Heat map) showing samples in rows and parameters in columns. Cluster analysis (CA) aligned all studied parameters into three clusters. Cluster I: International index of Erectile Function-15 score, EF, IS, OS, SD; Cluster II: Sperm concentration, Sperm count; LH, FSH, TT, BMI, Age, Estradiol, SHBG; and Cluster III: (CAG)n, (GGC)n.
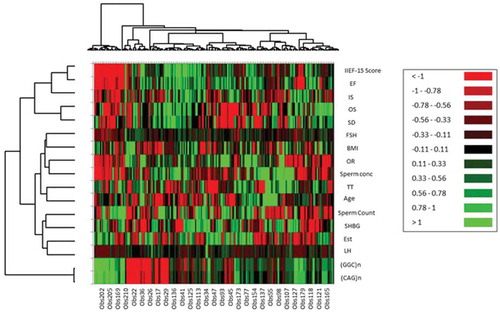
Figure 3. Principal component analysis (PCA) based on frequencies of clinical, hormonal, and genetic parameters, whereas correlation circle showing projection of the initial variables in the factor space. The Coordinate F1 accounts for 19.78 % variations and Coordinate F2 accounts for 10.64% variation.
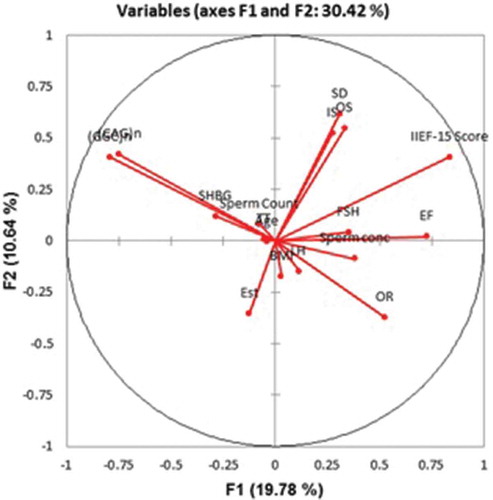
The present study examined an asthenospermic cohort (N= 210) characterized with respect to the individual or combinatorial effect of (CAG)n and (GGC)n alleles of the AR receptor as a function of hormonal profile and semen parameters. This data renders a direct evidence that repeated stretches of the androgen receptor (CAG)n and (GGC)n microsatellite are potentially involved in the ligand-binding transactivation capacity. This is in line with the published data in Iranian [Zare-Karizi et al. Citation2016] and European populations [Dale et al. Citation2016]. Furthermore, the positive correlation among both tri-nucleotide repeats is in agreement with previous study [Tirabassi et al. Citation2016].
On one hand, these studies along with others support a weak inverse association between (CAG)n and (GGC)n repeats [Grigorova et al. Citation2017; Bogaert et al. Citation2009]. On the other hand, the Indian population suggested an insignificant relationship between (CAG)n and (GGC)n repeats [Sharma et al. Citation2014]. Presumably, the existence of inconsistencies between the previous studies might be the result of inter-ethnic diversities and varied origin of the populations. Another salient feature of the present study was to report that longer (CAG)n tracts (>26) were closely associated with a moderately impaired function of AR in asthenospermic men. In this context, the longer (CAG)n repeats have shown significant correlations with sexual parameters such as orgasm function (OR) and erectile function (EF). To date this is the first study that captured the comprehensive snapshot between trinucleotide repeats and sexual dysfunction in Punjabi men. Notably, the study begins to suggest that (CAG)n repeats are involved in the regulation of different interconnected metabolic pathways that might have had indirect effect on sexual dysfunction of men [Tirabassi et al. Citation2015a].
We have demonstrated a negative correlation between long (CAG)n repeats (>26) and erectile dysfunction in the subjects having a relatively low serum T. Thus, it is in agreement with several previous studies which have reported a negative correlation between long (CAG)n repeats and the concentration of T [Cauci et al. Citation2017]. The paucity of T might have a risk for developing T deficiency syndrome which is successively associated with erectile dysfunction [Jääskeläinen Citation2017]. No doubt a potential source of conflict may also be found in previous related studies which often validated a non-significant association in compliance with long (CAG)n repeats and IIEF 15 score [Tirabassi et al. Citation2015b]. This was also demonstrated by assimilating the record of 85 men visiting outpatient clinics expounding a negative correlation between T, cumulative IIEF-15 score, and long (CAG)n repeats [Härkönen et al. Citation2003]. Further support is garnered from the work of Anderson et al. [Citation2011], who demonstrated an inverse association between extended (CAG)n repeats and T within a group of 213 randomly selected men of 41-45 years of age. Epidemiological data on 79 men with erectile dysfunction (ED) compared with 340 normal subjects, showed insignificant association with elongated (CAG)n repeats and T levels in infertile men [Canale et al. Citation2005].
There are certain limitation of our study. The main limitation is that participants completed the questionnaires in front of medical staff, which might have triggered mental discomfort feelings, resulting in a negative impact on the precision of the results. Secondly, 210 subjects and 125 healthy men were registered in this study; this small sample size might have had an effect on the outcomes. Third, medicated patients with depression and injuries could affect the association between AR (CAG)n and other hormonal variables.
In summary, our results confirm that length variations of (CAG)n and (GGC)n polymorphism in the transactivation domain of AR, significantly influence hormonal profile, semen parameters, and sexual functions of asthenospermic subjects by down regulating the expression of AR mediating signaling.
Materials and methods
Subjects
This study was approved by the Institutional Ethical Committee (IEC) of the University of Health Sciences, Lahore, Pakistan, in accordance with Helsinki Declarations. We also obtained a written informed consent from each participant. Personal interviews with each subject were conducted following an elaborative questionnaire to get accurate information regarding ethnicity, congenital diseases, age, and topographical origin.
Patients with any congenital defect, hypogonadism, hypertension, cardiovascular disease, diabetes, psychological illness, alcoholic addiction, and infectious disease were excluded from the study. The patients of our study comprised of 210 physically normal infertile asthenospermic men who visited different private poly-clinics exclusively under the aegis of the Lahore Institute of Fertility and Endocrinology, Hameed Latif Hospital Lahore (born and live in Lahore, Punjab). Moreover, all male participants were unable to induce a normal pregnancy for at least 2.5 years in spite of unprotected sexual intercourse. While female partners had no unidentified clinical cause of infertility and entirely fulfill the scoring criteria of Female Sexual Function Index (FSFI). Control group subjects with proven fertility (N = 125) were selected with normal spermatogenesis.
(CAG)n and (GGC)n allelic copy number
Genomic DNA was isolated from peripheral blood through revised salting out method. AR exon 1 repeats (CAG)n and (GGC)n were amplified from genomic DNA in 50 µl reaction mixture, having 10ng genomic DNA,10µM (2.5 mmol) Mgcl2, 0.5µl of each primer and AmpliTaq Gold® (Applied Bios systems, USA) master mix were used in accordance to the manufacturer’s instructions. Amplification of (CAG)n repeats was done by forward primer sequence GTGGTTGCTCCCGCAAGTTTCC and reverse primer GCTCCCACTTCCTCCAAGGACAATTA. While the automated sequencing was done through GCTGTGAAGGTTGCTGTTCCTC; for (GGC)n repeats forward primer was CAGCAAGAGACTAGCCCCAG and reverse was CCAGAACACAGAGTGACTCTGCC. (GGC)n repeats were sequenced with primer GGACTGGGATAGGGCACTCTGCTCAACC [Ferlin et al. Citation2004]. PCR extension was accomplished using BioRad™ MJ Mini thermal cycler (Applied Biosystems, USA) under standard conditions: initial denaturation at 95°C for 10 min, followed by 35 cycles of 94°C for 1 min, annealing at 58°C for 30 s, extension at 72°C for 1 min and final extension 72°C for 10min. ExoSAP-IT® (USB, Cleveland, OH, USA) was employed for refinement of PCR product. The sequencing was carried out by 3500 Genetic Analyzer (Applied Biosystems, USA) by using Big DyeTM Terminator version 3.1 cycle sequencing kit (Applied Biosystems, USA) according to the manufacturer’s instructions.
Estimation of clinical parameters
Clinical parameters such as age and BMI were calculated according to standard protocol [Wang et al. Citation1994].
Assessment of endocrine measurements
The blood sample was collected between 8 to 10 am from cubital vein and serum was separated instantly, stored at -20°C till the performance of hormonal assay including FSH, LH, T, Estradiol, and SHBG through electrochemiluminenscence Immunoassay according to the manufacturer’s instructions (Elecsys® Roche Diagnostics, Indianapolis, USA).
Semen analysis and physical examination
A clinical investigator physically examined the subjects before taking blood samples, whereas semen samples were obtained by masturbation after three to four days abstinence period and were collected in sterile containers, which were further incubated at 37°C for 30 min until liquefaction. All semen values were evaluated according to the guidelines and criteria of the World Health Organization (WHO) [Cooper et al. 2015] i.e., negative for Chlamydia and Ureaplasma urealyticum infection and devoid of mixed antiglobulin reaction (MAR). To determine the sperm concentration, samples were diluted in a buffer solution of 0.6 mol/L sodium-bi-carbonate along with 0.4% (V/V) formaldehyde. The baseline for spermatozoa analysis in infertile subjects were normal morphology; regressive motility i.e., less than 25 % motile spermatozoa; more than 20 million/ml sperm count. The selection criteria for control subjects based on normal structure; morphology; more than 50% motility and more than 40 million/ml sperm count.
Sexual performance was evaluated according to the IIEF-15 [Seftel Citation2017], which is categorize on the basis of the specific score assigned for erectile function i.e. sexual desire (SD) ranging from score 2-10, intercourse satisfaction (IS) estimated from 0-15, orgasm (O) ranging 0-10, and overall satisfaction (OS) ranging from 2 -10 score, while erectile dysfunction (ED) can be normal 26-30, slightly impaired 17-25, appropriately impaired 11-16, severely impaired ≥11.
Statistical analysis
Statistical analysis was done using statistical package SPSS (version 21; SPSS Inch., Chicago, IL, USA) and XLSTAT 2017 software. Differentiation in (CAG)n and (GGC)n were determined by nonparametric Wilcoxon’s rank sum test, χ2 test. Fisher’s exact test was used to find the relationship between categorical and categorized variables. Pearson’s product-moment correlations was used to find the association between number of (CAG)n and (GGC)n repeats while, Spearman correlation analysis was used to analyze the relationship among clinical, hormonal, and genetic variables. However, categorical continuous variables which were significantly correlated with sexual parameters at bivariate correlation but differ in sexual profile were further subjected into logistic regression analysis. The significant statistical difference was considered p<0.05.
Accession numbers
The entire data set has been submitted to NCBI Genbank (BankIt: 2014764) and is accessible online under Accession Numbers: MF072725 - MF072934.
Delaration of interest
The authors declared no conflict of interest.
Acknowledgments
We acknowledge the research initiative of the University of Health Sciences, Lahore and gratefully thank Professor, M. H. Qazi, Vice Chancellor, The University of Lahore, for providing research facilities.
Additional information
Notes on contributors
Haroon Latif Khan
Performed all the experimental work and wrote the paper: SB; Planned and supervised the work: MA; Edited the manuscript: HLK, YLK; Assisted in sample collection and PCR amplification: SA; Contributed in hormonal assay, sequencing, and statistical analysis: AAS, HHK, and MST.
References
- Andersen, M.L., Guindalini, C., Santos‐Silva, R., Bittencourt, L.R. and Tufik, S. (2011) Androgen receptor cag repeat polymorphism is not associated with erectile dysfunction complaints, gonadal steroids, and sleep parameters: Data from a population‐based survey. J Androl 32(5): 524–529.
- Bogaert, V., Vanbillemont, G., Taes, Y., De Bacquer, D., Deschepper, E., Van Steen, K. and Kaufman, J.M. (2009) Small effect of the androgen receptor gene GGN repeat polymorphism on serum testosterone levels in healthy men. Eur J Endocrinol 161(1): 171–177.
- Bousnane, N.E.H., May, S., Yahia, M. and Abu Alhaija, A.A. (2017) Association of CAT–262C/T with the concentration of catalase in seminal plasma and the risk for male infertility in Algeria. Syst Biol Reprod Med 63(5): 303–310.
- Canale, D., Caglieresi, C., Moschini, C., Liberati, C., Macchia, E., Pinchera, A., et al. (2005) Androgen receptor polymorphism (cag repeats) and androgenicity. J Clin Endo 63(3): 356–361.
- Cauci, S., Chiriacò, G., Cecchin, E., Toffoli, G., Xodo, S., Stinco, G., et al. (2017) Androgen receptor (ar) gene (cag) n and (ggn) n length polymorphisms and symptoms in young males with long-lasting adverse effects after finasteride use against androgenic alopecia. Sex Med 5(1): e61–e71.
- Cooper, T.G., Noonan, E., von Eckardstein, S., Auger, J., Baker, H.W., Behre, H.M., et al. (2010) World Health Organization reference values for human semen characteristics. Hum Reprod Update 16(3): 231–245.
- Dale, M., Maltby, J., Shimozaki, S., Cramp, R., Rickards, H. and REGISTRY Investigators of the European Huntington’s Disease Network. (2016) Disease stage, but not sex, predicts depression and psychological distress in huntington’s disease: A European population study. J Psychosom Res 80: 17–22.
- Eendebak, R.J., Huhtaniemi, I.T., Pye, S.R., Ahern, T., O’Neill, T.W., Bartfai, G., et al. (2016) The androgen receptor gene cag repeat in relation to 4-year changes in androgen-sensitive endpoints in community-dwelling older european men. Europ J Endo 175(6): 583–593.
- Ferlin, A., Bartoloni, L., Rizzo, G., Roverato, A., Garolla, A. and Foresta, C. (2004) Androgen receptor gene cag and ggc repeat lengths in idiopathic male infertility. Mol Hum Reprod 10(6): 417–421.
- Giagulli, V., Carbone, M., De Pergola, G., Guastamacchia, E., Resta, F., Licchelli, B., et al. (2014) Could androgen receptor gene cag tract polymorphism affect spermatogenesis in men with idiopathic infertility? J Assist Reprod and Genet 31(6): 689–697.
- Grigorova, M., Punab, M., Kahre, T., Ivandi, M., Tõnisson, N., Poolamets, O., et al. (2017) The number of CAG and GGN triplet repeats in the Androgen Receptor gene exert combinatorial effect on hormonal and sperm parameters in young men. Andrology 5(3): 495–504.
- Härkönen, K., Huhtaniemi, I., Mäkinen, J., Hübler, D., Irjala, K., Koskenvuo, M., et al. (2003) The polymorphic androgen receptor gene cag repeat, pituitary–testicular function and andropausal symptoms in ageing men. Int J Androl 26(3): 187–194.
- Hickey, T., Chandy, A and Norman, R. (2002) The androgen receptor cag repeat polymorphism and x-chromosome inactivation in australian caucasian women with infertility related to polycystic ovary syndrome. J Clin Endocrinol Metab 87(1): 161–165.
- Hsing, A.W., Gao, Y.T., Wu, G., Wang, X., Deng, J., Chen, Y.L., et al. (2000) Polymorphic cag and ggn repeat lengths in the androgen receptor gene and prostate cancer risk: A population-based case-control study in china. Cancer Res 60(18): 5111–5116.
- Ingles, S.A., Ross, R.K., Mimi, C.Y., Haile, R.W., Irvine, R.A., La Pera, G., et al. (1997) Association of prostate cancer risk with genetic polymorphisms in vitamin D receptor and androgen receptor. J National Cancer Inst 89(2): 166–170.
- Jääskeläinen, J. (2017) Androgen Resistance. Endocrinology of the Testis and Male Reproduction, pp. 1–24.
- Kalkan, R., Özdağ, N., Bundak, R., Çirakoğlu, A. and Serakinci, N. (2016) A unique mosaic Turner syndrome patient with androgen receptor gene derived marker chromosome. Syst Biol Reprod Med 62(1): 77–83.
- Levin, E.R. and Hammes, S.R. (2016) Nuclear receptors outside the nucleus: extranuclear signalling by steroid receptors. Nat Rev Mol Cell Biol 17(12): 783–797.
- Li, J., Xiao, F., Zhang, Y., Lan, A., Song, Q., Zhang, R., et al. (2017a) Shorter ggn repeats in androgen receptor gene would not increase the risk of prostate cancer. Technol Cancer Res Treat 16(2): 159–166.
- Li, L., Liu, W.M., Liu, M.X., Zheng, S.Q., Zhang, J.X., Che, F.-Y., et al. (2017b) A missense mutation in the androgen receptor gene causing androgen insensitivity syndrome in a chinese family. Asian J Androl 19(2): 260.
- Matsumoto, T., Sakari, M., Okada, M., Yokoyama, A., Takahashi, S., Kouzmenko, A., et al. (2013) The androgen receptor in health and disease. Annual Rev Phys 75: 201–224.
- Mostaghel, E.A., Plymate, S.R. and Montgomery, B. (2014) Molecular pathways: Targeting resistance in the androgen receptor for therapeutic benefit. Clin Cancer Res 20(4): 791–798.
- O’Hara, L. and Smith, L.B. (2015) Androgen receptor roles in spermatogenesis and infertility. Best Pract Res Clin Endocrinol Metab 29(4): 595–605.
- Pastuszak, A., Badhiwala, N., Lipshultz, L. and Khera, M. (2012) Androgen receptor CAG repeat length correlates with sexual function and hypogonadal symptoms in men. J Sex Med 9: 205–206.
- Peng, C.Y., Xie, H.J., Guo, Z.F., Nie, Y.L., Chen, J., Zhou, J.M., et al. (2014) The association between androgen receptor gene cag polymorphism and polycystic ovary syndrome: A case-control study and meta-analysis. J Assist Reprod Genet 31(9): 1211–1219.
- Querin, G., Martinelli, I., Bertolin, C., Pegoraro, E., Pennuto, M. and Sorarù, G. (2017) The role of ar polyq tract in male breast carcinoma: Lesson from an sbma case. Ann Oncol 28(5): 1160–1161.
- Sakkiah, S., Ng, H.W., Tong, W. and Hong, H. (2016) Structures of androgen receptor bound with ligands: Advancing understanding of biological functions and drug discovery. Expert Opin Ther Targets 20(10): 1267–1282.
- Seftel, A.D. (2017) Re: Rasch Analysis of the Premature Ejaculation Diagnostic Tool (PEDT) and the International Index of Erectile Function (IIEF) in an Iranian Sample of Prostate Cancer Patients. J Urol 197(6): 1534–1535.
- Sharma, V., Thangaraj, K. and Jyothy, A. (2014) A novel androgen receptor gene mutation in a patient with congenital adrenal hyperplasia associated with penoscrotal hypospadias. J Transl Res 164(2): 149–152.
- Shimada, M.K., Sanbonmatsu, R., Yamaguchi-Kabata, Y., Yamasaki, C., Suzuki, Y., Chakraborty, et al. (2016) Selection pressure on human str loci and its relevance in repeat expansion disease. Mol Genet Genomics 291(5): 1851–1869.
- Shiraishi, K. and Matsuyama, H. (2017) Gonadotoropin actions on spermatogenesis and hormonal therapies for spermatogenic disorders [review]. Endocr J 64(2): 123–131.
- Thangaraj, K., Joshi, M.B., Reddy, A.G., Gupta, N.J., Chakravarty, B. and Singh, L. (2002) Cag repeat expansion in the androgen receptor gene is not associated with male infertility in Indian populations. J Androl 23(6): 815–818.
- Tirabassi, G., Cignarelli, A., Perrini, S., Furlani, G., Gallo, M., Pallotti, F., et al. (2015a) Influence of cag repeat polymorphism on the targets of testosterone action. Int J Endocrinol 2015: 2015:298107.
- Tirabassi, G., Corona, G., Biagioli, A., Buldreghini, E., Muti, N., Maggi, M., et al. (2015b) Influence of androgen receptor cag polymorphism on sexual function recovery after testosterone therapy in late‐onset hypogonadism. J Sexual Med 12(2): 381–388.
- Tirabassi, G., Corona, G., Falzetti, S., Maggi, M. and Balercia, G. (2016) Influence of androgen receptor gene cag and ggc polymorphisms on male sexual function: A cross-sectional study. Int J Endocrinol 2016: 5083569.
- Traven, E., Ogrinc, A. and Kunej, T. (2017) Initiative for standardization of reporting genetics of male infertility. Syst Biol Reprod Med 63(1): 58–66.
- Wang, J., Thornton, J. C., Russell, M., Burastero, S., Heymsfield, S. and Pierson, R. N. (1994) Asians have lower body mass index (BMI) but higher percent body fat than do whites: comparisons of anthropometric measurements. Am J Clin Nutr 60(1): 23–28.
- Wang, W., John, E.M. and Ingles, S.A. (2005) Androgen receptor and prostate-specific antigen gene polymorphisms and breast cancer in African-American women. Cancer Epidemiol Biomarkers Prev 14(12): 2990–2994.
- Weng, H., Li, S., Huang, J.-Y., He, Z.-Q., Meng, X.-Y., Cao, Y., et al. (2017) Androgen receptor gene polymorphisms and risk of prostate cancer: a meta-analysis. Scientific Reports 7: 40554. http://doi.org/10.1038/srep40554.
- Younes, A.K., Hamed, H., Mohamed, E.E., Makki, M., Gaber, N. and Mohamed, H. (2016) Oestrogen receptors beta genotype in infertile egyptian men with nonobstructive azoospermia. J Androl 48(10): 1289–1293.
- Zare-Karizi, S., Amin-Beidokhti, M., Rahimi, M. and Mirfakhraie, R. (2016) Analysis of the androgen receptor cag repeats length in Iranian patients with idiopathic non-obstructive azoospermia. Asian Pacific J Reprod 5(1): 71–74.