ABSTRACT
Embryo micromanipulation was developed after introduction of microinjection to overcome infertility. Embryo micromanipulation may be performed at any embryo stage from pronuclear to blastocyst. The technique started out as basic and turned out to be increasingly more complex. Embryo micromanipulation at the cleavage-stage includes a wide range of techniques, from opening the zona pellucida in order to improve the chance of implantation, to removing detrimental components from the embryo to enhance embryo development or blastomeres for preimplantation genetic diagnosis and embryo splitting. Evaluating the impact(s) of different micromanipulation techniques on epigenetics of the embryo and considering quality control during these techniques are important issues in this regard. This review aims to discuss the micromanipulation of cleavage-stage embryos in clinical assisted reproductive technology (ART).
Abbreviations: ART: assisted reproductive technology; ICSI: intracytoplasmic sperm injection; IVF: in vitro fertilization; PGD: preimplantation genetic diagnosis; PZD: partial zona dissection; ZP: zona pellucida; SUZI: subzonal insemination; PVS: perivitelline space; AH: assisted hatching; LAH: laserassisted hatching; ZT: zona thinning; UV: ultraviolet; IR: infrared; PCR: polymerase chain reaction; FISH: fluorescent in situ hybridization; NGS: next generation sequencing; QC: quality control; QA: quality assurance
Introduction
Micromanipulation in assisted reproductive technology (ART) has been of great help to treat infertile patients and to understand the basic fertilization and embryo development concepts. In light of the introduction of the successful human in vitro fertilization (IVF) method in 1987, many infertile patients were given hope of becoming parents. However, after several years, there remained many cases with male factor infertility that could not be treated by this standard technique. Thus, micromanipulation techniques were introduced by embryologists to overcome this problem. Micromanipulation techniques include injecting a single sperm into the oocyte to assist fertilization, zona breaching to improve blastocyst hatching, fragment or lysed cell removal to improve embryo development, and embryo biopsy to detect possible chromosomal abnormalities. The first micromanipulation technique was partial zona dissection (PZD) in which the zona pellucida (ZP) was opened by mechanical force in order to facilitate sperm access to the oocyte vestment. Subzonal insemination (SUZI) was the next procedure where sperm cells were directly deposited into the perivitellinespace (PVS). The high rate of polyspermy, due to more than one sperm entering into the oocyte, and low rate of fertilization were the drawbacks of this technique. Next was the injection of a single spermatozoon into an oocyte, called intracytoplasmic sperm injection (ICSI) (Palermo et al. Citation1992). Assisted zona hatching, fragment/debris/lysed cell removal, blastomere biopsy, and finally embryo splitting were introduced successively. Micromanipulation techniques are performed in every stage of development including oocytes, zygote, cleavage-stage embryos, morula, and blastocyst. This review aims to discuss the micromanipulation of the cleavage-stage human embryo in clinical ART. This review also underlines the possible role exerted by micromanipulation in inducing epigenetic alterations and the need for high quality control during micromanipulation.
Assisted hatching
ZP hatching is a natural process caused by serine proteases released from maternal (uterine cells) or embryonic (trophectoderm cells) sources or/and increasing internal pressure due to blastocyst expansion (Cohen Citation1991; O’Sullivan et al. Citation2002; Sathananthan et al. Citation2003). Normally, the embryo escapes from the ZP 5–6 days after fertilization just before implantation. There are some factors that impair hatching, including abnormality in ZP structure, increase in ZP thickness, and zona hardening. Advanced maternal age, ovarian stimulation protocol, sub-optimal in vitro culture and cryopreservation may induce zona hardening (Aston and Weimer Citation2010). Assisted hatching (AH), by creating an opening in the ZP, helps the embryo to escape from the ZP. In human embryos, the site of hatching is in close proximity to the blastocyst inner cell mass (Gonzales et al. Citation1996). Therefore, the site of AH seems important for the initiation of hatching (Miyata et al. Citation2010). The recommended indications for AH include advanced maternal age (≥40 years), elevated FSH level, thick ZP (>15µm), frozen-thawed embryos, and history of implantation failure (≥2) (Cohen et al. Citation1992; Schoolcraft et al. Citation1994; Tao and Tamis Citation1997; Mansour et al. Citation2000; Hammadeh et al. Citation2011). Some AH indications are referred to in vitro culture like zona hardening or lack of produced protease by the embryo (Schiewe et al. Citation1995). Recently, Razi et al. (Citation2013) in a prospective randomized study evaluated the ART outcomes following laser AH (LAH) in patients undergoing their first ICSI treatment due to male factor infertility. They showed that the clinical pregnancy and the live birth rates were similar between patients with AH and the control group (Razi et al. Citation2013).
In theory, AH may increase the chance of implantation of abnormal embryos that otherwise are unable to hatch themselves. There is a lack of comprehensive data on the effect of AH on increased congenital anomalies and possible epigenetic changes on children born following IVF and AH (Das et al. Citation2009; Marchesi et al. Citation2012; Razi et al. Citation2013). It was shown that the rate of congenital anomalies was the same between the LAH group and the control group (Razi et al. Citation2013). Additional well-designed studies focusing on neonatal outcome and a long-term follow-up of children born following AH is necessary in order to draw a solid conclusion on the safety of this procedure.
Multiple pregnancy including monozygotic twinning is considered to be one of the main concerns in AH usage (Schieve et al. Citation2000). A monochorionictriamnionic triplet associated with monoamniotic twins following AH has been reported (Pantos et al. Citation2009). It is suggested to reduce the number of transferred embryos undergoing AH (Martins et al. Citation2011). However, in a systematic review and meta-analysis, it was concluded that AH does not improve multiple pregnancy in fresh embryo transferred to non-poor prognosis patients (Martins et al. Citation2011). There are different types of AH including mechanical, chemical, enzymatical/pronase, and laser procedures.
Mechanical assisted hatching
PZD was the first method of AH using mechanical force to open a hole in the ZP (Malter and Cohen Citation1989; Cohen et al. Citation1990). In this technique, the embryo is held by the holding pipette, and the microneedle penetrates the ZP. Then, the microneedle is pushed until it pierces the opposite site. When the microneedle has entered the embryo, the embryo is released from the holding pipette and the slit is created by rubbing the impaled ZP section on the holding pipette. This technique is more natural compared to other AH methods but requires extensive technical skills in micromanipulation. The size of the hole is not under control and holes of different sizes may be created if the technique is repeated. Pregnancies are also reported from zona thinning (ZT) derived from this technique (Nijs et al. Citation1992).
Mechanical expansion of ZP
This method mimics the natural hatching process in which the increasing internal blastocyst pressure helps to trigger the embryo escape from the ZP. In this technique, the embryo is held by a holding pipette and medium is injected via injection using a microneedle in a blastomere free area and the injecting hydrostatic pressure to assist embryo hatching (Fang et al. Citation2010; Hammadeh et al. Citation2011). Regarding the volume of injection, Fang et al. has suggested to withdraw the medium into the injection microneedle until the length of 200 µm (Fang et al. Citation2010). No ZP dissolving is applied. Therefore, the creation of a hole in the ZP and ZT are not achieved in this method resulting in preventing blastomere loss after hatching. However, ultrastructural changes may occur by applying this technique (Fang et al. Citation2010).
Chemically assisted hatching
Dissolving the ZP by acid Tyrode’s is considered a chemical AH (Cohen et al. Citation1992). In this method, the ZP is thinned (Tucker et al. Citation1993) or a hole is created through the ZP. The needle containing acid Tyrode’s solution is emptied near the embryo. The place for depletion is where the PVS is wider and/or near the cytoplasmic fragments instead of blastomeres (blastomere free area). It is very important to reduce the acid solution entering to the PVS to a minimum amount. Therefore, acid suction is needed after the ZP is breached. It is very important to wash the embryo in several microdroplets to remove the residual acid solution. The amount of acid solution deposited and the time of exposure are important factors affecting the outcome of this method. Also, only well-experienced embryologists should be selected to perform this AH method because rapid manipulation is necessary for this technique.
Pronase treatment
Pronase treatment is the enzymatically treatment to completely dissolve or thin the ZP. The frequency of this technique in clinical setting is less than other AH methods. In this technique, the embryo is exposed to pronase 0.2% for a short period of time (30 seconds to 1 minute). The embryo is checked frequently to evaluate ZP softening and disappearing. The embryo is transferred and washed several times in culture media before complete zona dissolving (Fong et al. Citation1998; Isik et al. Citation2000; Balaban et al. Citation2002).
Laser assisted hatching
LAH was described for the first time in 1991 (Tadir et al. Citation1991). The laser provides an easy way to thin the ZP or creates a hole in a controlled manner in the ZP. There are two modes of using the laser in AH, contact or non-contact. In the contact mode, the laser touches the embryo through a guided optical fiber while in the non-contact mode, the laser reaches the embryo through an optical lens. Initially, both ultraviolet (UV) wavelength and infrared (IR) wavelength were used for LAH. Currently, the non-contact mode using the IR 1.48 μm (1480 nm) diode laser is more common for LAH. This method presents a safe, easy, high accuracy, fast, effective, and repeatable technique for AH (Balaban et al. Citation2002; Hammadeh et al. Citation2011). Clinical data have shown that LAH is correlated with a higher pregnancy rate compared to chemical AH (Hsieh et al. Citation2002). One concern about the using the laser is heat production resulting from ZP ablation. It is important to keep blastomeres as far away as possible from the heating zone displayed on the monitor by the specific software. Laser radiation is highly absorbed by water and this is the reason for increased temperature during drilling. The medium temperature in and near the beam focus area rapidly reaches >100ºC depending on the intensity of the laser beam. Conduction during and after the laser pulse could help to rapidly reduce the heat (Tadir and Douglas‐Hamilton Citation2007). The dissolution of the ZP is due to the increase of temperature near the laser beam resulting from conducted energy (Tadir and Douglas‐Hamilton Citation2007). Three properties of the laser that can impact the embryo and surrounding media include power, wavelength, and pulse length. The power has a direct relationship with the diameter of the hole created in the ZP. It is shown that using different laser pulse lengths for embryo biopsy had no negative impacts on both the procedure and embryo development (Taylor et al. Citation2010). LAH may be performed immediately to minutes or hours before embryo transfer. For LAH, the beam power is set at ≤300 mW with a pulse duration of ≤800 µs and a single opening of 30 µm in length (about 10% of the ZP circumference) is created (Tadir and Douglas‐Hamilton Citation2007). Two to three laser diode pulses are used to thin the ZP or create a hole (10–30 µm). The laser beam focused on the solid surfaces should be avoided ().
Figure 1. Laser assisted hatching in a cleavage-stage embryo. The laser is used for zona pellucida thinning or creation of a hole in the zona pellucida. This technique helps the embryo to escape from zona pellucida before implantation. For laser assisted hatching, the beam power is set at ≤300 mW with a pulse duration of ≤800 µs and a single opening of 30 µm in length (about 10% of the ZP circumference) is created. Two to three laser diode pulses are used to thin the zona pellucida or create a hole (10–30 µm). The laser beam is shot from one side (A) and creates a hole (B).
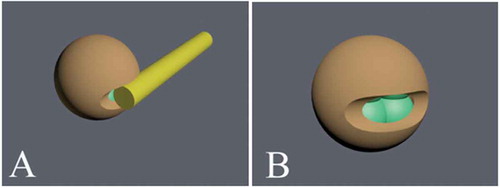
Piezo micromanipulation
In this technique the embryos are individually loaded in a 10µl droplet and a 20 µm diameter hole is created using the piezo micromanipulator (Nakayama et al. Citation1999). This method is very quick (2 seconds for creating the hole and 15 seconds for thinning the ZP) with the possibility of the ZP thinning and drilling simultaneously (Nakayama et al. Citation1998). The embryo is held by a holding pipette. The microneedle is loosely attached to the ZP and is vibrated. Vibration is produced by continuous piezoelectric pulses. The position of the vibrating needle is changed to an adjacent area for ZT.
Zona thinning
Complete perforation of the ZP may be related to the loss of blastomeres or complete loss of the embryo especially when embryo transfer is difficult or in the case of female reproductive tract contractions (Nichols and Gardner Citation1989). Antinori et al. demonstrated that ZT with laser leads to an increased implantation rate in patients with a history of repeated implantation failure (Antinori et al. Citation1996). By ZT, increasing the internal pressure of the embryo occurs in a similar manner and a premature burst of outer coat does not occur and because the inner layer of the ZP in untreated, the embryo is protected from potential harm from the female reproductive tract (Ebner Citation2010). ZT, along with zona hatching, is performed when the ZP is very thick or dark (Tadir and Douglas‐Hamilton Citation2007).
Fragment removal
Embryo fragmentation is a common phenomenon occurring in cleavage-stage in vitro produced embryos and can be the result of either a physiological or a pathological process. Fragments are anucleated portions of the cytoplasm that might contain cytoplasmic organelles. Mitochondria are the main organelles found in the fragments (Halvaei et al. Citation2016a). The majority of fragments are produced during the first mitotic divisions. In theory, fragment removal can improve ART outcomes by several mechanisms including 1) improving cell-cell interaction and restoring the spatial relationship between blastomeres and 2) preventing the lysis of neighboring blastomeres due to the release of harmful compounds from fragments. Fragment removal was introduced at the late 1990s (Alikani et al. Citation1999). Fragment removal is suggested to be performed on embryos with moderate fragmentation (15–35%), because low fragmentation seems to have less detrimental effects on embryo viability and fragments may be reabsorbed following subsequent embryo development (pseudo fragmentation). In contrast, severe embryo fragmentation s (>35%) is associated with a high rate of aneuploidy and fragment removal only changes the embryo morphology score but not the implantation potential.
The effect of fragment removal on ART outcome is a matter of debate. Retrospective studies support the beneficial effects of fragment removal, while the prospective studies do not clearly demonstrate any improvement in embryo development and pregnancy rate. Recently, our group reported that fragment removal in unselected cases does not improve pregnancy and live birth rates compared to the control group in fresh and vitrified-warmed embryos, while the pregnancy rate was significantly improved in cases with repeated implantation failure (Halvaei et al. Citation2015, Citation2016a; Safari et al. Citation2017). The site of ZP opening is important for fragment removal. It is better to open the ZP near the fragments, because it helps remove the fragments easily with minimal blastomere manipulation. After introducing the specific fragment removal pipette (inner diameter: 10–12 µm) through the ZP hole, the fragments around the blastomeres are gently removed (Halvaei et al. Citation2016b). The fragments may be in different spatial positions and continued refocusing is necessary during the procedure (). This technique needs a high level of expertise, patience, and technical skills. Touching blastomeres and aggressive micromanipulation may reduce embryo viability. Nonetheless, fragment removal should be performed as quickly as possible to minimize the time the embryos are kept out of the incubator.
Figure 2. Fragment and debris removal from a cleavage-stage embryo. Firstly, the zona pellucida should be opened by assisted hatching. The embryo is held by the holding pipette and noncontact 1.48 diode laser is used to open the zona pellucida (A). The place of assisted hatching should be precisely selected near the fragments and debris. After opening of the zona pellucida, an appropriate micropipette (10–12 µm) is inserted between blastomeres space (B) and the fragments and debris around the blastomeres are gently removed (C). During fragment and debris removal, continued refocusing should be performed for easy access to fragments and debris. Finally, the micropipette is gently removed and the embryo is released from the holding pipette (D).
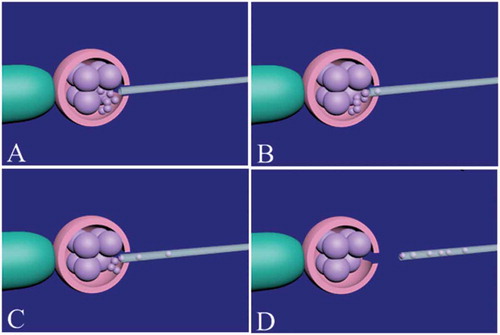
Debris removal
Debris and coarse granulation in the PVS may interfere with the hatching process. Debris and coarse granulation removal can prevent probable adverse effects of these structures during the hatching process. In a recent paper, we described coarse granulation removal and defined fragment removal plus coarse granulation removal as ‘cosmetic micromanipulation’ (Halvaei et al. Citation2015). We reported that in cases with a history of implantation failure, the cosmetic micromanipulation may improve implantation and ongoing pregnancy rates. Beneficial effects of debris and coarse granulation removal on pregnancy and live birth rates has not been verified in a prospective study for unselected patients (Halvaei et al. Citation2016a). The technique is performed the same as fragment removal. It should be noted that some debris may be attached more tightly to the ZP and aggressive manipulation to remove them should be avoided.
Removal of lysed cells
Blastomere lysis after thawing cryopreserved embryos might be seen due to sub-optimal cryopreservation technique or the poor quality of the embryo per se. Lysed cells may impair cell-cell communication between healthy blastomeres necessary for compaction. It was shown that removal of lysed blastomeres in a frozen-thawed embryo is associated with embryo viability and pregnancy rate (Alikani et al. Citation1993; Rienzi et al. Citation2002). It was assumed that necrotic blastomeres after cryopreservation may release toxic metabolites and removal of the necrotic cell(s) can prevent the detrimental effects of the released compounds to the healthy blastomeres (Rienzi et al. Citation2002). It is clear that if the number of lysed cells increases in a frozen-thawed embryo, or if the lysed cells are distributed between the healthy blastomeres, the success rate of this technique decreases. Many studies have reported the beneficial effects of removing lysed blastomeres on embryo viability, embryo development, hatching rate, and implantation of embryo following the cryopreservation (Alikani et al. Citation1993; Rienzi et al. Citation2002; Liu et al. Citation2005; ; Rienzi et al. Citation2005; Elliott et al. Citation2007; Fathi et al. Citation2008) ().
Figure 3. Lysed blastomere removal in a cleavage-stage embryo. Firstly, the embryo is held by the holding pipette. The place of zona pellucida opening should be near the lysed blastomere(s). A hole about 20 µm in diameter is created with a noncontact 1.48 diode laser. After opening of the zona pellucida (A), an appropriate micropipette is inserted near the lysed blastomere (B). The lysed blastomere is gently removed (C) using a × 25 objective. If there is another lysed blastomere, the previous step is repeated. Finally, the embryo is released from the holding pipette.
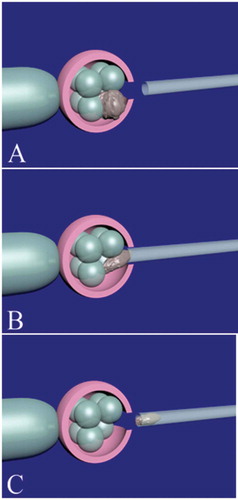
Blastomere biopsy
Embryo biopsy involves removing few blastomeres from the preimplantation embryo for genetic testing to determine the monogenic disease and/or numerical chromosomal abnormalities to avoid transferring an abnormal or aneuploid embryo into a mother’s womb. Embryo biopsy can be performed at different stages of preimplantation embryo development including polar body (zygote), blastomere (cleavage-stage embryo), and, trophectoderm (blastocyst) (Cimadomo et al. Citation2016). The biopsy at each stage has its advantages and disadvantages helping the embryologist to decide at which stage embryo biopsy should be performed. In the recent past, blastomere biopsy at the cleavage-stage embryo has accounted for 90% of preimplantation embryo biopsies (Moutou et al. Citation2014). It was suggested that the blastomere biopsy should be performed on a day 3 embryo that has at least 6 cells and less than 30% fragmentation (Harton et al. Citation2011; Cimadomo et al. Citation2016).
The first step for embryo biopsy is ZP breaching using different methods of AH including mechanical force, acid Tyrode’s solution, and LAH. According to ESHRE preimplantation genetic diagnosis (PGD) consortium, cycles from January to December 2009, the most prevalent method used for zona breaching for PGD cycles was laser (75.7%) followed by acidic Tyrode (17.1%) and mechanical action (7.2%) (Moutou et al. Citation2014). After opening the ZP, the biopsy needle can easily enter the cleavage-stage embryo to remove one or two blastomeres. Viscous fluids can be used to fill the biopsy needle in order to facilitate the suction of blastomeres. ZP opening for embryo biopsy is much bigger as compared to AH (70 µm). It is important that the hole’s size is optimum. A narrow hole increases the risk of blastomere degeneration while a large hole results in loss of more than one blastomere. The culture medium used for blastomere biopsy is a Ca++/Mg++ free medium, suitable for reducing cell-cell adhesion and aiding in the detachment and aspiration of the blastomere. Since Ca++ is very important for compaction, the main concern is Ca++ depletion following the use of Ca++/Mg++ free medium, so it is important to minimize the time the embryo is exposed to this medium, and the biopsy procedure should be performed quickly (). Factors involved in embryo biopsy, such as size and number of holes introduced may affect the hatching process (Cohen and Feldberg Citation1991; Schmoll et al. Citation2003). Abnormal hatching and subsequent embryo development are the main concerns in the embryo biopsy. Time-lapse analysis has shown the blastomere biopsy in PGD cases would cause delayed compaction and change in the hatching mechanism (Kirkegaard et al. Citation2012).
Figure 4. Blastomere biopsy in a cleavage-stage embryo. The embryo should be immobilized by the holding pipette. Noncontact 1.48 diode laser is used for zona pellucida opening (A). It is important to open the zona pellucida near the blastomere which is candidate for biopsy. After assisted zona hatching, the biopsy micropipette (inner diameter: 20–40 µm) is gently entered and brought in close proximity to the blastomere (B). The blastomere with visible nucleus is gently removed (C). The biopsy pipette is removed from the embryo (D). The biopsied blastomere is released to biopsy medium and the embryo is released from the holding pipette.
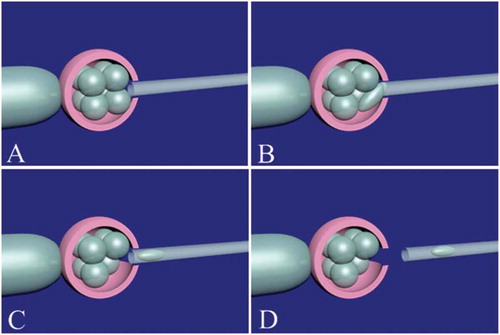
Blastomere biopsy and a single cell analysis may be associated with technical and biological difficulties. In theory, single cell analysis might be associated with amplification failure. To overcome this problem, it is suggested to remove two blastomeres for analysis. Brodie et al. evaluated the PGD results, embryo development and clinical outcomes between groups in which one or two blastomeres were biopsied from cleavage-stage embryos with more than 7 blastomeres on day-3 (Brodie et al. Citation2012). They found that the likelihood of PGD results was significantly higher when 2 blastomeres were biopsied compared to 1 blastomere biopsy (92% vs. 88%, respectively). They also showed that if the embryo has more than 7 blastomeres on day 3, the embryo development and clinical outcome are the same between the two groups. However, there are studies showing detrimental effects of removing more than 1 cell on embryo development and clinical outcomes (Goossens et al. Citation2008; De Vos et al. Citation2009). Removing 2 blastomeres may reduce the implantation rate in comparison with 1 blastomere biopsy (Munné et al. Citation2010). However, the embryologist’s expertise and skill have a crucial role in this regard.
One of the problems associated with blastomere biopsy is chromosomal mosaicism. In this situation, cells with different karyotypes are seen within the same embryo. The rate of chromosomal mosaicism is high in cleavage stage embryo (Wells and Delhanty Citation2000). It is suggested to remove two blastomeres to overcome this problem (Brodie et al. Citation2012; Cimadomo et al. Citation2016). The high rate of mosaicism in cleavage-stage embryos led to introduce trophectoderm biopsy as an alternative option for PGD-A (Harton et al. Citation2011; Vera-Rodriguez and Rubio Citation2017). The diagnostic efficacy, regarding chromosomal mosaicism and PCR accuracy, is high in trophectoderm biopsy (Chang et al. Citation2013; Greco et al. Citation2015). Also, technical limitation(s) and FISH interpretation may lead to overstimulation of abnormalities in the blastomere biopsy (Rubio et al. Citation2009; Vera-Rodriguez and Rubio Citation2017). New techniques, like NGS, are now suggested for comprehensive chromosomal screening with a higher accuracy (Weissman et al. Citation2017).
The presence of blastomere nucleus should be considered at the time of blastomere biopsy. If the biopsied blastomere is lost or an anucleate blastomere is biopsied, the re-biopsy of another blastomere is acceptable if there are enough remaining blastomeres in the embryo (Harton et al. Citation2011). From the molecular point of view, ICSI is the choice method of insemination for cases undergoing blastomere biopsy when the PCR method is applied (Harton et al. Citation2011). Spermatozoa attached to the ZP or non-decondensed sperm cells within the blastomere increase the chance of paternal contamination (Harton et al. Citation2011).
The impact of a blastomere biopsy on embryo gene expression is controversial. It was recently shown that the gene expression pattern may be different in biopsied mouse embryos. In particular, micromanipulation alters gene expression in embryos resulting from a stress response or recovery attempt (Jones et al. Citation2015). Concerns have risen about the effects of embryo biopsy on epigenetic alterations. Animal studies have shown the probable increased risk of neurodegenerative disorders in the offspring following blastomere biopsy in the mouse model (Yu et al. Citation2009). Also, the association between blastomere biopsy and alterations in steroid metabolism has been proposed in mice (Sugawara et al. Citation2012). Moreover, changes in epigenetic patterns in the mouse brain resulting in behavioral disorders has been reported in recent publications (Sampino et al. Citation2014).
Blastomere biopsy at the cleavage stage embryo may lead to reduce the reproductive potential of the embryo of about 40% compared to control (Scott et al. Citation2013a). Scott et al. in a review concluded that trophectoderm biopsy at the blastocyst seems to be safer compared to blastomere biopsy (Scott et al. Citation2013b). In the trophectoderm biopsy, the proportion of the embryo’s total cells removed is fewer compared to the blastomere biopsy (2–3% vs. 13%, respectively) (Scott et al. Citation2013a). The extra-embryonic cells are removed in the trophectoderm biopsy while the fate of removed blastomere is not morphologically predictable in the blastomere biopsy. Because of activation of the embryonic genome, the blastocyst may have more tolerance to micromanipulation in comparison with the cleavage-stage embryo (Scott et al. Citation2013a)
Trophectoderm biopsy is an invasive and expensive technique which requires well-experienced embryologist and increases the workload for IVF clinics and longer follow-up is needed for this technique (Kort and Behr Citation2017). If the facilities of quick report of aneuploidy screening are not available in an IVF clinic, the biopsied blastocyst should be transferred in a frozen-thawed embryo transfer cycle which has its pros and cons.
Embryo splitting
Embryo splitting is one of the ways to produce a genetically identical organism. This technique can be performed at different embryo developmental stages including cleavage-stage and morula/blastocyst (bisection) (Noli et al. Citation2017). Human embryo splitting was introduced for the first time in 1993 (Hall et al. Citation1993). Embryo splitting is the microsurgical separation of the blastomere into equal halves, producing a new embryo. In this technique, half of blastomeres are removed by biopsy micropipette and inserted into an empty ZP. Briefly, the ZP should first be opened (e.g., laser) to remove the blastomeres. The biopsy of blastomeres is performed on the heated stage. The diameter of the hole should be wide enough to reduce the stress to blastomeres during biopsy (30–40 µm). Four blastomeres of an 8-cell embryo are gently removed and placed in an empty ZP prepared from unfertilized/immature oocytes or clinically unsuitable embryos (Noli et al. Citation2015; Halvaei et al. Citation2016b) ().
Figure 5. Embryo splitting technique in a cleavage-stage embryo. A good quality 8-cell embryo is selected for embryo splitting. The embryo is immobilized by the holding pipette. After opening the zona pellucida with a noncontact diode laser (A), half of the blastomeres are gently removed (B) and released to biopsy medium. An empty zona pellucida should be previously prepared from unfertilized/immature oocytes or clinically unsuitable embryos. The embryo is released from the holding pipette and the empty zona pellucida is held instead. The removed blastomeres are gently placed in the empty zona pellucida (C).
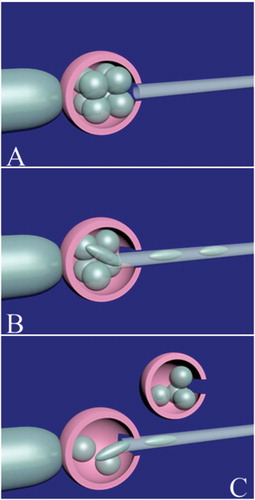
The possible advantages of this technique are 1) a cost-effective method to produce excess embryos and 2) a reduced number of ovarian stimulation cycles to create more embryos. Embryo splitting produces genetically identical embryos for research purposes eliminating the role of genetic background (Noli et al. Citation2017). It is well known that the blastomeres are totipotent and embryo splitting increases the chance of producing more embryos available for infertility treatment or research purposes. There is no general agreement for clinical use of this technique and published articles are controversial. Some studies are in favor of using this technique in the clinical setting, but others believe that these embryos are not suitable for clinical or research purposes (Noli et al. Citation2017). This technique enables infertile couples to have more embryos transferred in a fresh cycle or cryopreserved for future possible transfer. Previous studies have shown that the number of embryos available for transfer and the number of transferred embryos have positive effects on ART outcomes (Pandian et al. Citation2009). The patients who have a low number of embryos benefit from embryo splitting. Advanced maternal age (>37 years), patients with severe pelvic disease(s) like endometriosis, women with poor ovarian reserve, and poor responders are the main indications for embryo splitting (Wood Citation2001). Some points should be taken to account: 1) embryos produced from this group of patients have a reduced likelihood of reaching the blastocyst stage even without any manipulation, so good quality embryos should be selected for embryo splitting and 2) all these patients have more likelihood of having aneuploid embryos, and splitting an abnormal embryo will not help them anyway suggesting that the clinical application of this technique should be performed with extreme caution. Animal studies have shown that although the cell number in split embryos is lower if compared to that of non-split embryos, the size of offspring is normal because the regulation of cell number occurs after blastocyst formation (Escribá et al. Citation2002). However, the size of blastocysts produced from twin embryos is related to the number of blastomeres used for embryo splitting (Noli et al. Citation2015). In addition, morphokinetic data from the same human study have shown that the inner cell mass forms later in the twin embryo compared to the controls and the size and quality of inner cell mass is lower as well (Noli et al. Citation2015). The number of blastomeres is a main indicator of embryo splitting outcome. It was shown that subsequent embryo development following embryo splitting from embryos with 6–8 cells is higher compared to 2–5 cells (Illmensee et al. Citation2010). Since maternal mRNA rapidly disappears after the major activation of the genome at the 4–6 -cell stage and then a new wave of parental mRNA necessary for subsequent embryogenesis is synthesized, considering the 6-cell stage for embryo splitting appears to be rational (Hamatani et al. Citation2006; Illmensee and Levanduski Citation2010). In addition, embryo morphology plays an important role in the efficacy of embryo splitting (Illmensee et al. Citation2010). The Ca++/Mg++free medium is used in this technique like blastomere biopsy procedure but the time needed for exposing is much higher (15 minutes) (Escribá et al. Citation2002). It is suggested to push together the transferred blastomeres inside the ZP to improve cellular contact which is necessary for compaction (Illmensee et al. Citation2010). The ASRM ethic committee has reported that embryo splitting as an acceptable technique (ASRM Citation2004).
Embryo micromanipulation and epigenetic modifications
The term epigenetics was introduced more than 70 years ago referring to ‘the study of changes in gene function that are mitotically and/or meiotically heritable without a change in DNA sequence’ (Waddington Citation1942; Wu and Morris Citation2001). Covalent modifications of DNA bases, histone replacement, posttranslational modifications, and genome imprinting are the main epigenetic modifications (Dupont et al. Citation2009). DNA methylation has several functions including gene imprinting, X-chromosome inactivation, and centromere function (Dean et al. Citation2005; Koerner and Barlow Citation2010). It was shown that different ART procedures and clinically modifiable factors are associated with epigenetics alterations (Ghosh et al. Citation2017; Menezo et al. Citation2017; Roy et al. Citation2017). It also is necessary for early embryo development and diminishes with each division (Qiao et al. Citation2010; Marchesi et al. Citation2012). Imprinting and the methylation pattern have a main role in identification and expression of genes during embryo development. Epigenetic studies in human embryos are associated with ethical restrictions. It is necessary to evaluate the probable epigenetic alterations in human embryos born after different micromanipulation techniques, like ICSI and blastomere biopsy, to elucidate a final conclusion regarding the safety of these techniques. Long-term follow-up should be performed for the safety of such procedures because the effects of epigenetic errors do not necessarily appear in the short-term assessment (Niemitz and Feinberg Citation2004). Moreover, the parents who seek these techniques should receive comprehensive information regarding the safety and the side effects of micromanipulation, including any possible complication (Hansen et al. Citation2013; Ventura-Juncá et al. Citation2015).
Quality control
Quality control (QC) and quality assurance (QA) are crucial foundations of ART, specifically during embryo micromanipulation. In the past, most of the materials used for embryo micromanipulation were prepared in-house. Today, consumables such as different culture media, and microneedles could be purchased from different companies. The commercial materials have passed different QC tests and seem to be more cost effective. There is a relationship between quality control and occurring genetic and epigenetic disorders. It is known that alterations in both contact materials like plasticware, glassware, and embryo culture system (culture media, culture conditions, and incubators) may cause impairment in embryo quality as well (Marchesi et al. Citation2012).
In general, embryo micromanipulation techniques need a highly experienced embryologist. It is suggested to start micromanipulation procedures by first applying ICSI and, if ICSI results are satisfying, the embryologist can perform AH, fragment removal, and blastomere biopsy. The time the embryo is out of incubator should be minimized and micromanipulations should be performed as quickly as possible to maintain pH, temperature, and osmolarity at the optimum level. It is recommended to record the timing need for micromanipulation techniques for QC/QA purposes (Harton et al. Citation2011). Devices and equipment used for embryo micromanipulation need regular maintenance and surveillance. For example, mechanical components should be replaced in case of wear. Hydraulicforce systems should be checked to detect and then adjust any leakage (Malter Citation2016). Electronic devices, like the laser system, should periodically be serviced by an expert technician (Malter Citation2016). It is suggested to write a standard operating procedure for each technique. Providing a log book for documentation of the date of use, operator name, and status of each device is also necessary.
Conclusion
Micromanipulation has been an important procedure in ART to overcome infertility issues and to understand basic science in this field. It should be kept in mind that the embryologist’s expertise and skill have a crucial role in this regard. Embryo micromanipulation cleavage-stage techniques would undoubtedly be developed in the future. Concerns have remained regarding the genomic and epigenomic effects of these techniques. It should be emphasized that the new embryo micromanipulation methods should be applied in the clinic after passing safety evaluations. Any possible complication(s) of micromanipulation techniques should be described to parents seeking these techniques and written informed consent should be taken before applying procedures.
Acknowledgment
The authors would like to thank Dr. Navid Esfandiari for his great help and valuable comments to prepare this paper.
Disclosure statement
No potential conflict of interest was reported by the authors.
Additional information
Notes on contributors
Mohammad Ali Khalili
Concept, analysis, format, revision, and editing: IH, SG, SAN, MAK; Literature search, extraction analysis, and drafted the manuscript: IH. All authors approved the final version of the manuscript.
References
- Alikani M, Olivennes F, Cohen J. 1993. Fertilization and early embryology: microsurgical correction of partially degenerate mouse embryos promotes hatching and restores their viability. Hum Reprod. 8:1723–8.
- Alikani M, Cohen J, Tomkin G, Garrisi GJ, Mack C, Scott RT. 1999. Human embryo fragmentation in vitro and its implications for pregnancy and implantation. Fertil Steril. 71:836–42.
- American Society for Reproductive Medicine. 2004. Embryo splitting for infertility treatment. Fertil Steril. 82:256–257.
- Antinori S, Panci C, Selman H, Caffa B, Dani G, Versaci C. 1996. Zona thinning with the use of laser: a new approach to assisted hatching in humans. Hum Reprod. 11:590–594.
- Aston KI, Weimer KE. 2010. Micromanipulation of human oocytes and embryos: applications of intracytoplasmic sperm injection and assisted hatching in infertility treatment. reproductive endocrinology and infertility. New York: Springer; p. 601–612.
- Balaban B, Urman B, Alatas C, Mercan R, Mumcu A, Isiklar A. 2002. A comparison of four different techniques of assisted hatching. Hum Reprod. 17:1239–43.
- Brodie D, Beyer C, Osborne E, Kralevski V, Rasi S, Osianlis T. 2012. Preimplantation genetic diagnosis for chromosome rearrangements–one blastomere biopsy versus two blastomere biopsy. J Assist Reprod Genet. 29:821–827.
- Chang L-J, Huang -C-C, Tsai -Y-Y, Hung -C-C, Fang M-Y, Lin Y-C, Su Y-N, Chen S-U, Yang Y-S. 2013. Blastocyst biopsy and vitrification are effective for preimplantation genetic diagnosis of monogenic diseases. Hum Reprod. 28:1435–1444.
- Cimadomo D, Capalbo A, Ubaldi FM, Scarica C, Palagiano A, Canipari R, Rienzi L. 2016. The impact of biopsy on human embryo developmental potential during preimplantation genetic diagnosis. Biomed Res Int. 2016:1–10.
- Cohen J, Elsner C, Kort H, Malter H, Massey J, Mayer MP, Wiemer K. 1990. Impairment of the hatching process following IVF in the human and improvement of implantation by assisting hatching using micromanipulation. Hum Reprod. 5:7–13.
- Cohen J. 1991. Assisted hatching of human embryos. J In Vitro Fert Embryo Transf. 8:179–190.
- Cohen J, Alikani M, Trowbridge J, Rosenwaks Z. 1992. Implantation enhancement by selective assisted hatching using zona drilling of human embryos with poor prognosis. Hum Reprod. 7:685–691.
- Cohen J, Feldberg D. 1991. Effects of the size and number of zona pellucida openings on hatching and trophoblast outgrowth in the mouse embryo. Mol Reprod Dev. 30:70–78.
- Das S, Blake D, Farquhar C, Seif MM. 2009. Assisted hatching on assisted conception (IVF and ICSI). Cochrane Database Syst Rev. 2:CD001894.
- De Vos A, Staessen C, De Rycke M, Verpoest W, Haentjens P, Devroey P, Liebaers I, Van De Velde H. 2009. Impact of cleavage-stage embryo biopsy in view of PGD on human blastocyst implantation: a prospective cohort of single embryo transfers. Hum Reprod. 24:2988–2896.
- Dean W, Lucifero D, Santos F. 2005. DNA methylation in mammalian development and disease. Birth Defects Res C Embryo Today. 75:98–111.
- Dupont C, Armant DR, Brenner CA. 2009. Epigenetics: definition, mechanisms and clinical perspective. Semin Reprod Med. 20:164–170.
- Ebner T. 2010. Micromanipulation Techniques in IVF. J Reprod Biotech Fertil. 1:108–119.
- Elliott TA, Colturato LFA, Taylor TH, Wright G, Kort HI, Nagy ZP. 2007. Lysed cell removal promotes frozen–thawed embryo development. Fertil Steril. 87:1444–1449.
- Escribá M, Valbuena D, Remohí J, Pellicer A, Simón C. 2002. New techniques on embryo manipulation. J Reprod Immunol. 55:149–161.
- Fang C, Li T, Miao B-Y, Zhuang G-L, Zhou C. 2010. Mechanically expanding the zona pellucida of human frozen thawed embryos: a new method of assisted hatching. Fertil Steril. 94:1302–1307.
- Fathi R, Valojerdi MR, Eftekhari-Yazdi P. 2008. Effect of laser-assisted hatching and necrotic blastomere removal on the development of vitrified–warmed four-cell mouse embryos. J Assist Reprod Genet. 25:333–339.
- Fong CY, Bongso A, Ng SC, Kumar J, Trounson A, Ratnam S. 1998. Blastocyst transfer after enzymatic treatment of the zona pellucida: improving in-vitro fertilization and understanding implantation. Hum Reprod. 13:2926–2932.
- Ghosh J, Coutifaris C, Sapienza C, Mainigi M. 2017. Global DNA methylation levels are altered by modifiable clinical manipulations in assisted reproductive technologies. Clin Epigenetics. 9:14.
- Gonzales D, Jones J, Pinyopummintr T, Carnevale E, Ginther O, Shapiro S, Bavister BD. 1996. Implantation: trophectoderm projections: a potential means for locomotion, attachment and implantation of bovine, equine and human blastocysts. Hum Reprod. 11:2739–2745.
- Goossens V, De Rycke M, De Vos A, Staessen C, Michiels A, Verpoest W, Van Steirteghem A, Bertrand C, Liebaers I, Devroey P, et al. 2008. Diagnostic efficiency, embryonic development and clinical outcome after the biopsy of one or two blastomeres for preimplantation genetic diagnosis. Hum Reprod. 23:481–492.
- Greco E, Biricik A, Cotarelo RP, Iammarone E, Rubino P, Tesarik J, Fiorentino F, Minasi MG. 2015. Successful implantation and live birth of a healthy boy after triple biopsy and double vitrification of oocyte-embryo-blastocyst. SpringerPlus. 4:22.
- Hall JL, Engel D, Gindoff PR, Mottla G, Stillman R. 1993. Experimental cloning of human polyploid embryos using an artificial zona pellucida. Fertil Steril. 61:S1.
- Halvaei I, Khalili MA, Safari S, Esfandiari N. 2015. Ongoing pregnancies following cosmetic micromanipulation of preimplantation embryos in patients with implantation failure. Case Rep Med. 2015:1–4.
- Halvaei I, Khalili MA, Esfandiari N, Safari S, Talebi AR, Miglietta S, Nottola SA. 2016a. Ultrastructure of cytoplasmic fragments in human cleavage stage embryos. J Assist Reprod Genet. 33:1677–1684.
- Halvaei I, Khalili MA, Nottola SA. 2016b. A novel method for transmission electron microscopy study of cytoplasmic fragments from preimplantation human embryos. Microsc Res Tech. 79:459–462.
- Hamatani T, Ko M, Yamada M, Kuji N, Mizusawa Y, Shoji M, Hada T, Asada H, Maruyama T, Yoshimura Y. 2006. Global gene expression profiling of preimplantation embryos. Hum Cell. 19:98–117.
- Hammadeh ME, Fischer-Hammadeh C, Ali KR. 2011. Assisted hatching in assisted reproduction: a state of the art. J Assist Reprod Genet. 28:119–128.
- Hansen M, Kurinczuk JJ, Milne E, de Klerk N, Bower C. 2013. Assisted reproductive technology and birth defects: a systematic review and meta-analysis. Hum Reprod Update. 19:330–353.
- Harton G, Magli M, Lundin K, Montag M, Lemmen J, Harper J. 2011. ESHRE PGD Consortium/Embryology Special Interest Group—best practice guidelines for polar body and embryo biopsy for preimplantation genetic diagnosis/screening (PGD/PGS). Hum Reprod. 26:41–46.
- Hsieh YY, Huang CC, Cheng TC, Chang CC, Tsai HD, Lee MS. 2002. Laser-assisted hatching of embryos is better than the chemical method for enhancing the pregnancy rate in women with advanced age. Fertil Steril. 78:179–182.
- Illmensee K, Levanduski M, Vidali A, Husami N, Goudas VT. 2010. Human embryo twinning with applications in reproductive medicine. Fertil Steril. 93:423–427.
- Illmensee K, Levanduski M. 2010. Embryo splitting. Middle East Fertil Soc J. 15:57–63.
- Isik A, Vicdan K, Kaba A, Dagli G. 2000. Comparison of zona manipulated and zona intact blastocyst transfers: a prospective randomized trial. J Assist Reprod Genet. 17:135–139.
- Jones D, Paczkowski M, Kuehl T. 2015. Embryo manipulation techniques alter pre-implantation development and gene expression in mouse embryos. Reproduction, Reprod Fertil Dev. 28:182.
- Kirkegaard K, Hindkjaer JJ, Ingerslev HJ. 2012. Human embryonic development after blastomere removal: a time-lapse analysis. Hum Reprod. 27:97–105.
- Koerner MV, Barlow DP. 2010. Genomic imprinting—an epigenetic gene-regulatory model. Curr Opin Genet Dev. 20:164–170.
- Kort J, Behr B. 2017. Biomechanics and developmental potential of oocytes and embryos. Fertil Steril. 108:738–741.
- Liu WX, Zheng Y, Luo MJ, Huang P, Yue LM, Wang L, He Y-P, Zhang J-H. 2005. Effects of removal of necrotic blastomeres from mouse cryopreserved embryos on blastocyst formation and hatching. Theriogenology. 64:1114–1120.
- Malter HE. 2016. Micromanipulation in assisted reproductive technology. Reprod Biomed Online. 32:339–347.
- Malter HE, Cohen J. 1989. Partial zona dissection of the human oocyte: a nontraumatic method using micromanipulation to assist zona pellucida penetration. Fertil Steril. 51:139–148.
- Mansour R, Rhodes C, Aboulghar M, Serour G, Kamal A. 2000. Transfer of zona-free embryos improves outcome in poor prognosis patients: a prospective randomized controlled study. Hum Reprod. 15:1061–1064.
- Marchesi DE, Qiao J, Feng HL. 2012. Embryo manipulation and imprinting. Semin Reprod Med. 30:323–334.
- Martins WP, Rocha IA, Ferriani RA, Nastri CO. 2011. Assisted hatching of human embryos: a systematic review and meta-analysis of randomized controlled trials. Hum Reprod Update. 17:438–453.
- Menezo Y, Dale B, Elder K. 2017. Time to re-evaluate ART protocols in the light of advances in knowledge about methylation and epigenetics: an opinion paper. Hum Fertil. 25:1–7.
- Miyata H, Matsubayashi H, Fukutomi N, Matsuba J, Koizumi A, Tomiyama T. 2010. Relevance of the site of assisted hatching in thawed human blastocysts: a preliminary report. Fertil Steril. 94:2444–2447.
- Moutou C, Goossens V, Coonen E, De Rycke M, Kokkali G, Renwick P, SenGupta SB, Vesela K, Traeger-Synodinos J. 2014. ESHRE PGD Consortium data collection XII: cycles from January to December 2009 with pregnancy follow-up to October 2010. Hum Reprod. 29:880–903.
- Munné S, Wells D, Cohen J. 2010. Technology requirements for preimplantation genetic diagnosis to improve assisted reproduction outcomes. Fertil Steril. 94:408–430.
- Nakayama T, Fujiwara H, Tastumi K, Fujita K, Higuchi T, Mori T. 1998. A new assisted hatching technique using a piezo-micromanipulator. Fertil Steril. 69:784–788.
- Nakayama T, Fujiwara H, Yamada S, Tastumi K, Honda T, Fujii S. 1999. Clinical application of a new assisted hatching method using a piezo-micromanipulator for morphologically low-quality embryos in poor-prognosis infertile patients. Fertil Steril. 71:1014–1018.
- Nichols J, Gardner R. 1989. Effect of damage to the zona pellucida on development of preimplantation embryos in the mouse. Hum Reprod. 4:180–187.
- Niemitz EL, Feinberg AP. 2004. Epigenetics and assisted reproductive technology: a call for investigation. Am J Hum Genet. 74:599–609.
- Nijs M, Vanderzwalman P, Bertin G. 1992. Pregnancies obtained after zona softening of in vitro cultured or frozen–thawed human embryos. Hum Reprod. 7:82.
- Noli L, Dajani Y, Capalbo A, Bvumbe J, Rienzi L, Ubaldi FM, Ogilvie C, Khalaf Y, Ilic D. 2015. Developmental clock compromises human twin model created by embryo splitting. Hum Reprod. 30:2774–2784.
- Noli L, Ogilvie C, Khalaf Y, Ilic D. 2017. Potential of human twin embryos generated by embryo splitting in assisted reproduction and research. Hum Reprod Update. 23:156–165.
- O’Sullivan CM, Liu SY, Karpinka JB, Rancourt DE. 2002. Embryonic hatching enzyme strypsin/ISP1 is expressed with ISP2 in endometrial glands during implantation. Mol Reprod Dev. 62:328–334.
- Palermo G, Joris H, Devroey P, Van Steirteghem AC. 1992. Pregnancies after intracytoplasmic injection of single spermatozoon into an oocyte. The Lancet. 340:17–18.
- Pandian Z, Bhattacharya S, Ozturk O, Serour G, Templeton A. 2009. Number of embryos for transfer following in‐vitro fertilisation or intra‐cytoplasmic sperm injection. Cochrane Database Syst Rev. 15:CD003416.
- Pantos K, Kokkali G, Petroutsou K, Lekka K, Malligiannis P, Koratzis A. 2009. Monochorionic triplet and monoamniotic twins gestation after intracytoplasmic sperm injection and laser-assisted hatching. Fetal Diagn Ther. 25:144–147.
- Qiao J, Chen Y, Yan LY, Yan J, Liu P, Sun QY. 2010. Changes in histone methylation during human oocyte maturation and IVF-or ICSI-derived embryo development. Fertil Steril. 93:1628–1636.
- Razi MH, Halvaei I, Razi Y. 2013. Laser assisted zona hatching does not improve live birth rate in patients undergoing their first ICSI cycles. Iran J Reprod Med. 11:1021–1026.
- Rienzi L, Nagy ZP, Ubaldi F, Iacobelli M, Anniballo R, Tesarik J, Greco E. 2002. Laser-assisted removal of necrotic blastomeres from cryopreserved embryos that were partially damaged. Fertil Steril. 77:1196–1201.
- Rienzi L, Ubaldi F, Iacobelli M, Minasi MG, Romano S, Ferrero S, Sapienza F, Baroni E, Tesarik J, Greco E. 2005. Developmental potential of fully intact and partially damaged cryopreserved embryos after laser-assisted removal of necrotic blastomeres and post-thaw culture selection. Fertil Steril. 84:888–894.
- Roy M-C, Dupras C, Ravitsky V. 2017. The epigenetic effects of assisted reproductive technologies: ethical considerations. J Dev Orig Health Dis. 8:436–442.
- Rubio C, Giménez C, Fernández E, Vendrell X, Velilla E, Parriego M, Rodrigo L. 2009. The importance of good practice in preimplantation genetic screening: critical viewpoints. Hum Reprod. 24:2045–2047.
- Safari S, Khalili MA, Barekati Z, Halvaei I, Anvari M, Nottola SA. 2017. Cosmetic micromanipulation of vitrified-warmed cleavage stage embryos does not improve ART outcomes: an ultrastructural study of fragments. Reprod Biol. 17:210–217.
- Sampino S, Zacchini F, Swiergiel A, Modlinski A, Loi P, Ptak G. 2014. Effects of blastomere biopsy on post-natal growth and behavior in mice. Hum Reprod. 29:1875–1883.
- Sathananthan H, Gunasheela S, Menezes J. 2003. Mechanics of human blastocyst hatching in vitro. Reproductive Biomed Online. 7:228–234.
- Schieve LA, Meikle SF, Peterson HB, Jeng G, Burnett NM, Wilcox LS. 2000. Does assisted hatching pose a risk for monozygotic twinning in pregnancies conceived through in vitro fertilization? Fertil Steril. 74:288–294.
- Schiewe MC, Hazeleger NL, Sclimenti C, Balmaceda JP. 1995. Physiological characterization of blastocyst hatching mechanisms by use of a mouse antihatching model. Fertil Steril. 63:288–294.
- Schmoll F, Schneider H, Montag M, Wimmers K, Rink K, Schellander K. 2003. Effects of different laser-drilled openings in the zona pellucida on hatching of in vitro–produced cattle blastocysts. Fertil Steril. 80:714–719.
- Schoolcraft WB, Schlenker T, Gee M, Jones GS, Jones HW. 1994. Assisted hatching in the treatment of poor prognosis in vitro fertilization candidates. Fertil Steril. 62:551–554.
- Scott KL, Hong KH, Scott RT. 2013b. Selecting the optimal time to perform biopsy for preimplantation genetic testing. Fertil Steril. 100:608–614.
- Scott RT, Upham KM, Forman EJ, Zhao T, Treff NR. 2013a. Cleavage-stage biopsy significantly impairs human embryonic implantation potential while blastocyst biopsy does not: a randomized and paired clinical trial. Fertil Steril. 100:624–630.
- Sugawara A, Sato B, Bal E, Collier AC, Ward MA. 2012. Blastomere removal from cleavage-stage mouse embryos alters steroid metabolism during pregnancy. Biol Reprod. 87:4,1–9.
- Tadir Y, Wright W, Vafa O, Liaw L, Asch R, Berns M. 1991. Micromanipulation of gametes using laser microbeams. Hum Reprod. 6:1011–1016.
- Tadir Y, Douglas‐Hamilton DH. 2007. Laser effects in the manipulation of human eggs and embryos for in vitro fertilization. Methods Cell Biol. 82:409–431.
- Tao J, Tamis R. 1997. Application of assisted hatching for 2-day-old, frozen-thawed embryo transfer in a poor-prognosis population. J Assist Reprod Genet. 14:128–130.
- Taylor TH, Gilchrist JW, Hallowell SV, Hanshew KK, Orris JJ, Glassner MJ, Wininger JD. 2010. The effects of different laser pulse lengths on the embryo biopsy procedure and embryo development to the blastocyst stage. J Assist Reprod Genet. 27:663–667.
- Tucker MJ, Luecke NM, Wiker SR, Wright G. 1993. Chemical removal of the outside of the zona pellucida of day 3 human embryos has no impact on implantation rate. J Assist Reprod Genet. 10:187–191.
- Ventura-Juncá P, Irarrázaval I, Rolle AJ, Gutiérrez JI, Moreno RD, Santos MJ. 2015. In vitro fertilization (IVF) in mammals: epigenetic and developmental alterations. Scientific and bioethical implications for IVF in humans. Biol Res. 48:1–13.
- Vera-Rodriguez M, Rubio C. 2017. Assessing the true incidence of mosaicism in preimplantation embryos. Fertil Steril. 107:1107–1112.
- Waddington CH. 1942. The epigenotype. Endeavour. 1:18–20.
- Weissman A, Shoham G, Shoham Z, Fishel S, Leong M, Yaron Y. 2017. Chromosomal mosaicism detected during preimplantation genetic screening: results of a worldwide Web-based survey. Ferti Steril. 107:1092–1097.
- Wells D, Delhanty JD. 2000. Comprehensive chromosomal analysis of human preimplantation embryos using whole genome amplification and single cell comparative genomic hybridization. Mol Hum Reprod. 6:1055–1062.
- Wood C. 2001. Embryo splitting: a role in infertility? Reprod Fertil Dev. 13:91–93.
- Wu CT, Morris J. 2001. Genes, genetics, and epigenetics: a correspondence. Science. 293:1103–1105.
- Yu Y, Wu J, Fan Y, Lv Z, Guo X, Zhao C, Zhou R, Zhang Z, Wang F, Xiao M, et al. 2009. Evaluation of blastomere biopsy using a mouse model indicates the potential high risk of neurodegenerative disorders in the offspring. Mol Cell Proteomics. 8:1490–1500.