ABSTRACT
We sought to determine serum AMH levels in the maternal circulation, and the umbilical artery and vein, in normal women and women with PCOS, and their neonates at time of delivery. This represents a cross-sectional study of 57 pregnant patients who presented to the labor and delivery suite and subsequently delivered. We obtained maternal, as well as fetal blood from both, umbilical artery and vein. We measured serum concentrations of estradiol, AMH, testosterone and FSH. A total of 30 patients delivered a female and 27 a male neonate. Of them, 18/30 and 18/27 had a diagnosis of PCOS by NIH criteria. Mean age, BMI, weight gain in pregnancy, and gestational age did not differ between the two groups of mothers. AMH serum levels were statistically higher in women with PCOS (p < 0.005) and in their fetuses, independently of gender. Testosterone was higher in women with PCOS (p < 0.001), but there was no PCOS-related difference in their fetuses. FSH levels were significantly lower in PCOS than non-PCOS mothers carrying a male (p = 0.022), but not a female, fetus. AMH was positively correlated with maternal serum testosterone (p = 0.001) and negatively with fetal serum FSH (p < 0.026). In PCOS pregnancies, AMH was negatively correlated with maternal BMI (p = 0.019), menstrual cycle length (p = 0.035), and fetal uterine vein FSH (p = 0.021). In conclusion, at time of delivery, fetuses of women with PCOS had higher AMH levels and similar testosterone levels compared to fetuses from non-PCOS mothers, irrespective of gender. Our results may help explaining developmental differences in offspring of PCOS women.
Trial registration: ClinicalTrials.gov identifier: NCT02431884.
Introduction
Embryonal and fetal hormonal milieu and trans-placental exchange define the optimal conditions for early life to develop. The development of several conditions, including polycystic ovary syndrome (PCOS), has been associated with hormonal changes during the intrauterine life (Cattrall et al. Citation2005). Women with PCOS have high androgen levels, which, besides hirsutism, are correlated with the presence of increased blood pressure readings and insulin resistance (Detti et al. Citation2015a). In addition, PCOS women have high serum anti-Müllerian hormone (AMH) levels from increased production (Pellat et al. Citation2007; Catteau-Jonard et al. Citation2008; Das et al. Citation2008). In addition, AMH levels correlate with metabolic risks, such as decreased HDL-cholesterol, and increased glucose, HOMA-IR, body mass index (BMI), and blood pressure (Pigny et al. Citation2003; Lin et al. Citation2011; Detti et al. Citation2015b; Feldman et al. Citation2017). AMH is known to regulate regression of the Müllerian structures in the male embryo and, in the female, it exerts an inhibitory action on primordial follicles (Durlinger et al. Citation2002), preventing the transition to primary follicles. In fact, in the absence of AMH the pool of primordial follicles is rapidly depleted (Visser et al. Citation2007). AMH was shown to influence FSH-dependent follicular growth (Durlinger et al. Citation2002) by down-regulating FSH and LH receptors in the ovarian cortex (Detti et al. Citation2017a). In addition, AMH correlates positively with testosterone levels and negatively with body mass index (BMI) (Laven et al. Citation2004; Piouka et al. Citation2009).
Our group previously hypothesized that AMH could have a role in the development of non-complex Müllerian anomalies such as subseptate uterus (Detti Citation2014; Detti et al. Citation2017b), which confirmed epigenetic changes as the most plausible cause, as no genetic abnormalities have been found, to date. However, little is known about the pathophysiology of Müllerian anomalies, as well as PCOS. Maternal hyperandrogenism was suggested to affect the metabolic and endocrine development of female offspring, leading to the development of PCOS-like phenotype and increased AMH later in life (Dumesic et al. Citation1997; Abbott DH et al Citation2005; Hart et al. Citation2010; Daan et al. Citation2017). Steroids cross the placenta in both directions but are metabolized en route mostly by aromatase, thus reducing the passage of androgens between the maternal and fetal compartments (Fisher et al. Citation1986; Petraglia et al. Citation1991; Kuijper et al. Citation2013). Most studies reporting on trans-placental hormone milieu have not compared PCOS and non-PCOS pregnant women, and did not specifically address the relationship between androgens, estrogens, AMH, and pituitary hormones in the mothers and their own fetuses (Debieve et al. Citation2000; Troisi et al. Citation2003a, Citation2003b; Gol et al. Citation2004; van de Beek et al. Citation2004; Carlsen et al. Citation2005; Tuutti et al. Citation2011; Vanky et al. Citation2012; Caanen et al. Citation2016). It is known that peptide hormones are virtually unable to pass the placenta to reach the fetal circulation. In pregnancy, women with PCOS have increased AMH levels compared to normal women (Tata et al. Citation2018). Since women with PCOS have higher AMH levels than women without the condition, we wanted to test the hypotheses that pregnant PCOS will have higher AMH levels than pregnant non-PCOS women, and that fetuses from women with PCOS will have higher AMH levels than fetuses of normal pregnancies. We thus determined serum levels of AMH, estradiol, testosterone, and FSH, in the maternal circulation, and the umbilical artery and vein in fetal cord blood at time of delivery, in women with, and without PCOS.
Results
We obtained blood specimens from 70 consecutive laboring patients and their newborns’ umbilical artery and vein, however, because of the difficulty obtaining a sizable umbilical blood specimen, only 57 pregnant women and their cord samples were available for our analyses. Of them, 45 were Black, 10 were White, and 2 were Hispanic. Thirty-six women had a diagnosis of PCOS (29 Black, 6 White, and 1 Hispanic) and 21 did not. Clinical characteristics of the study population are reported in , further subdivided in pregnancies with a female, or a male fetus. There was no gender-related difference between newborns from PCOS and non-PCOS pregnant women for any of the variables evaluated.
Table 1. Maternal and neonatal clinical characteristics.
AMH levels were higher in the serum of pregnant women with PCOS (1.310 ng/ml, Q1 0.363, Q3 2.200) than without PCOS women (0.553 ng/ml, Q1 0.337, Q3 0.949; p = 0.003). reports the serum hormone levels under investigation in the maternal and fetal circulation, grouped by PCOS and gender status. Women with PCOS, regardless of their offspring’s gender, had higher serum AMH levels than pregnant women without PCOS (100% increase; p < 0.005; ). In women carrying a female fetus, AMH levels were significantly higher than their fetuses’ levels. As expected, serum AMH was higher in the umbilical vessels of male than female fetuses (p < 0.001) and higher in male fetuses than in their mothers’ levels, but there was no difference in levels between male fetuses’ umbilical artery and vein. In female fetuses, however, umbilical artery AMH levels were higher than in the vein, although the difference did not reach significance (p = 0.052). There were increased AMH levels in female and male fetuses from PCOS, vs. fetuses from non-PCOS women (vein p = 0.003, artery p = 0.010 in female, and vein p = 0.010, artery p = 0.011, in male fetuses). Testosterone serum levels were significantly higher in PCOS than non-PCOS pregnant women (p < 0.001, ). In the fetal compartment, there was no difference in testosterone levels between female and male fetuses, and the levels were independent from their mothers’ PCOS status (). FSH levels were low in our maternal population, as expected. Levels were not different in PCOS and non-PCOS pregnant women, whether carrying a female or a male fetus (). In addition, levels were statistically lower in mothers than fetuses, irrespective of gender (p < 0.02), and they were significantly higher in male than female fetuses, irrespective of their mothers’ PCOS status (). Maternal estradiol was not different between PCOS and non-PCOS women (), but it was significantly higher than the fetal estradiol in both, the umbilical artery and vein (p < 0.038). In addition, there was no difference in serum estradiol between fetuses from PCOS or non-PCOS women, regardless of fetal gender. is a graphical representation of the maternal hormones’ serum concentrations in PCOS, and non-PCOS women, clustered by fetal gender.
Table 2. Hormone serum levels in the maternal and fetal circulation in pregnant PCOS and non-PCOS women.
Figure 1. Graphic representation of the investigated hormones’ serum concentrations in pregnant PCOS and non-PCOS women, clustered by fetal gender. PCOS Female = PCOS mother carrying a female fetus; Non-PCOS Female = non-PCOS mother carrying a female fetus; PCOS Male = PCOS mother carrying a male fetus; Non-PCOS Male = non-PCOS mother carrying a male fetus.
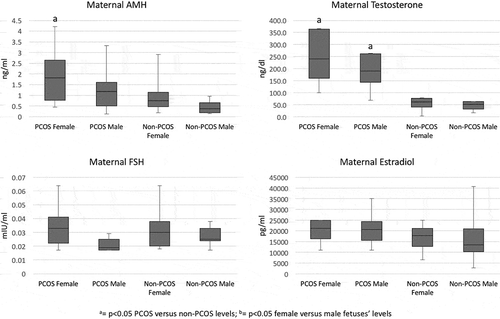
Spearman correlation results are reported as follows. Maternal AMH level was negatively correlated with maternal BMI (cc = −0.401, p = 0.019), menstrual cycle length (cc = −0.362, p = 0.035), and fetal uterine vein FSH (cc = −0.451, p = 0.021) in PCOS women. In non-PCOS women, maternal AMH was positively correlated with maternal testosterone (cc = 0.526, p = 0.014) and negatively with fetal AMH (cc = −0.699, p = 0.011) and FSH (cc = −0.649, p < 0.016). There was no correlation with the other parameters evaluated, including weight gain in pregnancy, birth weight, or gestational age and Apgar scores at delivery.
Discussion
To our knowledge, concurrent AMH, testosterone, and FSH serum levels in maternal and fetal (males and females) circulation at term of pregnancy in women with, and without PCOS, has never been reported. We found that, at time of delivery, women with PCOS have higher AMH and testosterone levels compared to women without PCOS and their female fetuses have higher AMH, but not testosterone, concentrations. Based on the fact that AMH is higher in the male fetus than in the mother, we infer that there is no passage of AMH from the fetus to the mother, similar to what occurs with insulin. The increased AMH and FSH serum concentrations found in the fetuses of pregnant PCOS could be due to a combination of placental passage from the mother and increased production in the fetal compartment. However, transplacental AMH passage has not been recently endorsed (Tata et al. Citation2018).
AMH serum levels were significantly higher in pregnant women with, than without, PCOS, whether they carried a female or a male fetus. AMH is lower during pregnancy and it seems to decrease throughout the three trimesters of pregnancy (La Marca et al. Citation2005; Pankhurst et al. Citation2016). The mechanism is not clear: we believe this could be due to hemodilution, rather than a decreased production; in fact, in pregnancy the ovaries continue their normal follicular development, even though they do not produce ovulatory follicles. As expected, serum AMH was higher in the umbilical vessels of males. However, when carrying a female fetus, maternal AMH was significantly higher than fetal AMH. This probably represents the reduced female fetal production secondary to the lower number of secondary follicles in the fetal ovary, compared to the post-pubertal ovary. Irrespective of gender, we found a PCOS-related significantly increased AMH concentration in the fetus. Even though Caanen et al. (Caanen et al. Citation2016) also found a higher serum AMH in fetuses from PCOS mothers compared to fetuses from non-PCOS mothers (2.11 ng/ml vs. 1.26 ng/ml in females and 46.8 ng/ml vs. 44.31 ng/ml in males, respectively), the difference was not significant, probably due to the low number of fetuses (14 vs. 38 females and 6 vs. 45 males). It is not clear why the fetus from a PCOS mother has higher AMH serum levels, but we could speculate that genetic inheritance of PCOS by the fetus causes increased AMH production by the embryonal/fetal granulosa and Sertoli cells. Indeed, in PCOS women, granulosa cells seem to have a boosted AMH production (Pellatt et al. Citation2007; Das et al. Citation2008). To corroborate the hypothesis of an increased fetal production, we found a non-significantly increased AMH serum levels in the umbilical artery in both genders. The higher AMH levels in the female fetus from a PCOS mother could hamper Müllerian duct development, possibly contributing to the development of milder (late-onset) forms of Müllerian anomalies, such as subseptate uteri. If we combine this finding with the increased incidence of PCOS in women with subsepate uteri (Detti et al. Citation2014, Citation2017b), we add power to the hypothesis that PCOS women from PCOS mothers are at higher risk for developing Müllerian anomalies. Higher AMH concentrations could thus delay/arrest Müllerian duct fusion and inner wall reabsorption, influencing the final uterine fundus’ silhouette, ensuing a bicornuate or a subseptate uterus. Indeed, Vanky et al. (Vanky and Carlsen Citation2012), found that AMH was measurable in cord blood as early as 21 weeks’ gestation, when the Müllerian structures complete their development.
Our estradiol results confirmed previously published levels and the lack of difference between fetal genders (Troisi et al. Citation2003a, Citation2003b). However, cord estradiol levels were significantly lower than maternal levels. Contrary to what was reported by Anderson et al. (Anderson et al. Citation2010), but consistent with Caanen et al. (Caanen et al. Citation2016), in our study PCOS does not appear to influence estradiol levels in the fetus. Our results are supported by the fact that there was no difference in testosterone levels, substrate for placental estradiol production, between females and males, as well as between fetuses from PCOS and non-PCOS pregnant women. This shows, first, that testosterone even though it can cross the placenta, is quickly degraded and converted by placenta’s aromatase into estradiol; second, at the end of pregnancy, fetuses from PCOS women do not produce more testosterone than fetuses from non-PCOS women. Our results suggest epigenetic changes preponderance over genetics in the development of PCOS. Recently, it was shown in mice that prenatal administration of AMH triggered hyperandrogenism in the mothers, which produced masculinization of the brain in the offspring with a negative impact on their future estrous cycle length and litter size (Tata et al. Citation2018). Testosterone, however, was directly measured in the offspring rather than in utero. Our findings support the lack of association between prenatal hyperandrogenism and prevalence of PCOS found in 244 adolescents in an Australian study (Hickey et al. Citation2009). There was no difference in maternal testosterone levels between mothers carrying a female versus a male fetus. Our results confirm Carlsen et al. (Carlsen et al. Citation2005), Gol et al, (Gol et al. Citation2004), van de Beek et al. (van de Beek et al. Citation2004), and Tuutti et al’s results (Tuutti et al. Citation2011), where no gender-related difference in maternal testosterone levels were found.
FSH levels were lower in our maternal population than in their fetuses, regardless of their gender, and lower than in non-pregnant women with or without PCOS, which confirmed previous studies (Fowler et al. Citation1998; Detti et al. Citation2015a). This is due to the negative feed-back of the high estradiol production by the placenta with the hypothalamus and pituitary (Fowler et al. Citation1998). FSH levels were significantly higher in male than female fetuses, irrespective of maternal PCOS status. While this confirms previous studies (Debieve et al. Citation2000; Bergada et al. Citation2002), this cannot be easily explained, since male fetuses’ testosterone and estradiol levels were not different from female fetuses.
Spearman’s correlation showed an unexpected negative, or absent, relationship between AMH, testosterone, and cycle length, in our population (except for the positive relationship with testosterone levels in non-PCOS women). It was interesting noticing that there was no correlation with the other parameters, including weight gain in pregnancy, birth weight, or gestational age and Apgar scores at delivery. While we can explain the relationship between testosterone and AMH, both increased in women with PCOS, we do not have a good explanation for the concurrent decrease in fetal FSH, as our data precludes plausible inferences.
Our study has some limitations, including the small population sample, the high BMI, the specific timing at the end of pregnancy, and the paucity of hormones evaluated, which could decrease the ability to make inferences. The menstrual cycle range was similar in the PCOS and non-PCOS groups, in fact PCOS women were able to ovulate and spontaneously conceive. We would assume that anovulatory PCOS women would have more striking differences than the ones here reported, however, our results may not be applicable to all the PCOS phenotypes. In addition, despite using the new generation assays to quantitate the protein – ECLIA – and steroid hormones – mass spectrometry – some of the results were close to the lower limit of detection. The study also presents strengths, including the concurrent sampling of the mothers with their term fetuses, the homogeneity of the population, and the young maternal age, which would virtually exclude low AMH levels from a concealed decreased ovarian reserve. Fetal AMH varies with gestational age, but in our population this effect was mitigated by including only patients at 38, or greater, weeks of gestation.
In conclusion, we proved the initial hypotheses that, at the time of delivery, women with PCOS have higher AMH levels compared to women without PCOS, and that AMH levels are higher in the female fetus and could contribute to the development of Müllerian abnormalities of the uterine fundus. Our results may contribute to the understanding of the genetic inheritance of PCOS and to explain developmental differences in offspring of PCOS women, which could be linked to the genetic inheritance of PCOS rather than to the hormonal (hyperandrogenic) environment in the mother.
Materials and methods
This was a cross-sectional study of pregnant women who consecutively presented to our hospital in labor, between May 2015 and April 2016. Patients were presented the details of the study and signed an informed consent form prior to being enrolled. The study was approved by the University of Tennessee Health Science Center (UTHSC) Human Investigation Committee (HIC) and is currently registered at ClinicalTrials.gov (NCT02431884). All patients were enrolled soon after presenting to the labor and delivery suite at our tertiary perinatal center, Rout Center at Regional One Health in Memphis, TN. Only women with term pregnancies (≥ 38 weeks of gestation) were asked to participate. After completing a questionnaire aimed at identifying PCOS (menstrual irregularities, presence of hirsutism, prior diagnosis of PCOS) patients were consecutively included for both, PCOS and non-PCOS, cohorts. PCOS was diagnosed with the use of NIH criteria such that all women had experienced ovulatory dysfunction/irregular cycles with clinical and/or biochemical signs of hyperandrogenism (Zawadski et al. Citation1992). Indeed, most patients had experienced at least one episode of ‘delayed menses’ within the two years prior to the index pregnancy. Other PCOS-classifying criteria could not be applied as an ovarian ultrasound was not feasible, nor reliable, in pregnancy. Other possible etiologies such as congenital adrenal hyperplasia, androgen-secreting tumors, Cushing’s syndrome were excluded. We were confident in the patients’ ability to recall a history of irregular periods and unwanted hair. The latest guidelines published by ASRM and ESHRE do support an a posteriori diagnosis of PCOS based on patient’s recollection and allow for a diagnosis of PCOS even in post-menopausal patients. (Teede et al. Citation2018) The diagnosis was determined before entering the study. If enrolled, the results of pre-pregnancy lab work were obtained, when available, to corroborate the diagnoses. Exclusion criteria were multiple gestation, assisted reproduction, type 2 diabetes, gestational diabetes, thyroid disease, and history of fertility treatments or other hormonal abnormalities. Measurements from the physical examination, such as age, blood pressure, height, weight, pre-pregnancy calculated body mass index (BMI) were recorded from the subject’s initial evaluation for all participants.
Specific instructions were given to the doctors dealing with the delivery about how to collect the blood samples. Maternal venous blood samples were drawn from an antecubital vein together with the remainder of their routine pre-delivery lab work as soon as they were admitted to the laboring suite. Immediately after cord clamping and before the placenta was delivered after either a cesarean or a vaginal delivery, the umbilical cord was clamped close to the umbilicus and the umbilical arteries and vein were punctured separately, and blood samples were collected in separate test tubes. Arteries were sampled first because it is more difficult to obtain blood from the umbilical artery than the vein. The goal was to obtain as much blood as possible from each vessel, or at least 4 ml, assuming that with a mean hematocrit of 45–50% in the term fetus, we would obtain about 2 ml of serum for the requested testing. Samples were allowed to clot at 4°C, centrifuged, and the serum stored at −80°C within 12 h from collection. All testing was performed by a centralized clinical laboratory (Esoterix Laboratories, part of LabCorp Laboratories, Endocrine Sciences, Calabasas Hills, CA 91,301), which assured homogeneity of the intra-assay and inter-assay variability. A minimum of 0.5 ml (1.0 ml for estradiol) of serum was required to carry out each assay. AMH was measured with an electrochemiluminescent immunoassay (ECLIA) [range: 0.11–262.69 ng/ml, lower limit of quantitation (LLQ): 0.015 ng/ml; intra-assay coefficient of variation (CV): 2.6%; inter-assay CV: 12.9]. The assay uses a clinically validated pair of monoclonal antibody raised against the associated form of human AMH. The amount of signal (relative light units) generated is directly proportional to the AMH concentration present in the sample. Each assay includes standards and controls for quality assurance. LabCorp Laboratories developed the ECLIA methodology to obviate two problems that were encountered using the second generation ELISA: a. low end was not precise or sensitive; b. no age-correlation was possible. Before releasing ECLIA for general use, the methodology was validated against results from a second generation ELISA and it was found to correlate greater than 95%. FSH was measured by ECLIA (range: 0.16–12.8 mIU/ml; LLQ: 0.017 mIU/ml; intra-assay and inter-assay CV: < 10%); estradiol was measured by high performance liquid chromatography/mass-spectrometry assay (HPLC/MS) (pregnancy range: 215 to > 4,300 pg/ml; LLQ: 1.0 pg/ml; intra-assay and inter-assay CV: < 10%); and total testosterone was measured by HPLC/MS (the investigators endorsed the male Tanner II range to encompass all expected serum concentrations in our population: < 3 to 432 ng/dl; LLQ: 2.5 ng/dl; intra-assay CV: 5.9%; inter-assay CV: 11.5%). For serum estradiol and testosterone, validated dilution factors were applied as necessary to allow expansion of the upper limit of reportable results.
Statistical analysis
We performed a power analysis expecting a 40% increase in serum AMH levels between PCOS and non-PCOS pregnant women (based on unpublished clinical data), with a power of 80% and the probability of a type 1 error of 0.05. The requested number of subjects per group was 14. All our variables were continuous and were summarized as median and 25% and 75% quartiles (quartile 1, 3). Because of the skewed distribution, we decided to use non-parametric tests for our analyses. We performed Mann-Whitney U test to compare distributions between groups. Relationships between continuous variables were tested with the use of Spearman correlations and a correlation coefficient (cc) and p value were reported. We used SPSS v24 for Windows (SPSS, Chicago, Illinois); a p value of < 0.05 was considered the threshold for statistical significance.
Clinical trial registration
This study is currently registered at ClinicalTrials.gov (NCT02431884).
Disclosure statement
No potential conflict of interest was reported by the authors.
Additional information
Funding
Notes on contributors
Laura Detti
Research idea, statistical analysis, figures, and manuscript writing: LD; Conducted research and manuscript writing: MEC, LF, GM; Conducted research: GI; Manuscript writing: MPD; Conducted research, statistical analysis, and manuscript writing: AMT.
References
- Abbott DH, Barnett DK, Bruns CM, Dumesic DA. 2005. Androgen excess fetal programming of female reproduction: a development aetiology for PCOS? Hum Reprod Update. 11:357–374.
- Anderson H, Fogel N, Grebe SK, Singh RJ, Taylor RL, Dunaif A. 2010. Infants of women with polycystic ovary syndrome have lower cord blood androstenedione and oestradiol levels. J Clin Endocrinol Metab. 95:2180–2186.
- Bergada I, Ballerini GM, Ayuso S, Groome NP, Bergada C, Campo S. 2002. High serum concentrations of dimeric inhibins A and B in normal newborn girls. Fertil Steril. 77:363–365.
- Caanen MR, Kuijper EA, Hompes PG, Kushnir MM, Rockwood AL, Meikle WA, Homburg R, Lambalk CB. 2016. Mass spectrometry methods measured androgen and estrogen concentrations during pregnancy and in newborns of mothers with polycystic ovary syndrome. Eur J Endocrinol. 174:25–32.
- Carlsen SM, Romundstad P, Jacobsen G. 2005. Early second-trimester maternal hyperandrogenemia and subsequent preeclampsia: a prospective study. Acta Obstet Gynecol Scand. 84:117–121.
- Catteau-Jonard S, Sp J, Leclerc A, Gonzalès J, Dewailly D, Di Clemente N. 2008. Anti-Mullerian hormone, its receptor, FSH receptor, and androgen receptor genes are overexpressed by granulosa cells from stimulated follicles in women with polycystic ovary syndrome. J Clin Endocrinol Metab. 93:4456–4461.
- Cattrall FR, Vollenhoven BJ, Weston GC. 2005. Anatomical evidence for in utero androgen exposure in women with polycystic ovary syndrome. Fertil Steril. 84,1689–1692.
- Daan NM, Koster MP, Steegers-Theunissen RP, Eijkemans MJ, Fauser BC. 2017. Endocrine and cardiometabolic cord blood characteristics of offspring born to mothers with and without polycystic ovary syndrome. Fertil Steril. 107(261–268.e3).
- Das M, Gillott DJ, Saridogan E, Djahanbakhch O. 2008. Anti-Mullerian hormone is increased in follicular fluid from unstimulated ovaries in women with polycystic ovary syndrome. Hum Reprod. 23:2122–2126.
- Debieve F, Beerlandt S, Hubinont C, Thomas K. 2000. Gonadotropins, prolactin, inhibin A, inhibin B, and activin A in human fetal serum from midpregnancy and term pregnancy. J Clin Endocrinol Metab. 85:270–274.
- Detti L. 2014. Ultrasound assessment of uterine cavity remodeling after surgical correction of subseptations. Am J Obstet Gynecol. 210:262–265.
- Detti L, Fletcher NM, Saed GM, Uhlmann RA, Peregrin-Alvarez I, Uhlmann RA. 2017a. Anti-Mullerian Hormone (AMH) may stall ovarian cortex function through modulation of hormone receptors other than the AMH receptor. Reprod. Sci. Epub ahead of print. doi:10.1177/1933719117737850.
- Detti L, Jeffries-Boyd HE, Williams JL, Diamond MP, Uhlmann RA. 2015a. Fertility biomarkers to estimate metabolic risks in women with polycystic ovary syndrome. J Assist Reprod Genetics. 32:1749–1756.
- Detti L, Norwick H, Levi D’Ancona R, Wright A, Christiansen ME. 2017b. Relevance of uterine subseptations. What Length Should Warrant Hysteroscopic Resection? J Ultrasound Med. 36:757–765.
- Detti L, Norwick H, Osborne SE, Carter C, Williams LJ, Uhlmann RA. 2015b. Anti-mullerian hormone (AMH) serum measurements reflect cycle length and ovarian follicle number in women with polycystic ovary syndrome (PCOS). Abstract Obstet Gynecol. 125:110S.
- Dumesic DA, Abbott DH, Eisner JR, Goy RW. 1997. Prenatal exposure of female rhesus monkeys to testosterone propionate increases serum luteinizing hormone levels in adulthood. Fertil Steril. 67:155–163.
- Durlinger ALL, Visser JA, Themmen APN. 2002. Regulation of ovarian function: the role of anti-Mullerian hormone. Reprod. 124:601–609.
- Feldman RA, O’Neill K, Butts SF, Dokras A. 2017. Antimüllerian hormone levels and cardiometabolic risk in young women with polycystic ovary syndrome. Fertil Steril. 107:276–281.
- Fisher DA. 1986. The unique endocrine milieu of the fetus. J Clin Invest. 78:603–611.
- Fowler PA, Evans LW, Groome NP, Templeton A, Knight PG. 1998. A longitudinal study of maternal serum inhibin-A, inhibin-B, activin-A, activin-AB, pro-alphaC and follistatin during pregnancy. Hum Reprod. 13:3530–3536.
- Gol M, Altunyurt S, Cimrin D, Guclu S, Bagci M, Demir N. 2004. Different maternal serum hCG levels in pregnant women with female and male fetuses: does fetal hypophyseal–adrenal–gonadal axis play a role? J Perinat Med. 32:342–345.
- Hart R, Sloboda DM, Doherty DA, Norman RJ, Atkinson HC, Newnham JP, Dickinson JE, Hickey M. 2010. Circulating maternal testosterone concentrations at 18 weeks of gestation predict circulating levels of antimullerian hormone in adolescence: a prospective cohort study. Fertil Steril. 94:1544–1547.
- Hickey M, Sloboda DM, Atkinson HC, Doherty DA, Franks S, Norman RJ, Newnham JP, Hart R. 2009. The relationship between maternal and umbilical androgen levels and polycystic ovary syndrome in adolescence: a prospective cohort study. J Clin Endocrinol Metab. 94:3714–3720.
- Kuijper EAM, Ket JCF, Caanen MR, Lambalk CB. 2013. Reproductive hormone concentrations in pregnancy and neonates: a systematic review. Reprod Biomed Online. 27:33–63.
- La Marca A, Giulini S, Orvieto R, De Leo V, Volpe A. 2005. Anti-mullerian hormone concentrations in maternal serum during pregnancy. Hum Reprod. 20:1569–1572.
- Laven JS, Mulders AG, Visser JA, Themmen AP, de Jong FH, Fauser BC. 2004. Anti- mullerian hormone serum concentrations in normoovulatory and anovulatory women of reproductive age. J Clin Endocrinol Metab. 89:318–323.
- Lin YH, Chiu WC, Wu CH, Tzeng CR, Hsu CS, Hsu MI. 2011. Antimullerian hormone and polycystic ovary syndrome. Fertil Steril. 96:230–235.
- Pankhurst MW, Clark CA, Zarek J, Laskin CA, McLennan IS. 2016. Changes in circulating proAMH and total AMH during healthy pregnancy and post-partum: a longitudinal study. PLoS One. 11(9):e0162509.
- Pellatt L, Hanna L, Brincat M, Galea R, Brain H, Whitehead S, Mason H. 2007. Granulosa cell production of anti-Mullerian hormone is increased in polycystic ovaries. J Clin Endocrinol Metab. 92:240–245.
- Petraglia F, Angioni S, Coukos G, Uccelli E, DiDomenica P, De Ramundo BM, Genazzani AD, Garuti GC, Segre A. 1991. Neuroendocrine mechanisms regulating placental hormone production. Contrib Gynecol Obstet. 18:147–156.
- Pigny P, Merlen E, Robert Y, Cortet-Rudelli C, Decanter C, Jonard S, Dewally D. 2003. Elevated serum level of AMH in patients with PCOS: relationship to the ovarian follicle excess and to the follicular arrest. J Clin Endocrinol Metab. 88:5957–5962.
- Piouka A, Farmakiotis D, Katsikis I, Macut D, Gerou S, Panidis D. 2009. Anti-mullerian hormone levels reflect severity of PCOS but are negatively influenced by obesity: relationship with increased luteinizing hormone levels. Am J Physiol Endocrinol Metab. 296:E238–43.
- Tata B, Mimouni NEH, Barbotin AL, Malone SA, Loyens A, Pigny P, Dewailly D, Catteau-Jonard S, Sundström-Poromaa I, Piltonen TT, et al. 2018. Elevated prenatal anti-Müllerian hormone reprograms the fetus and induces polycystic ovary syndrome in adulthood. Nat Med. 24:834–846. Epub ahead of print. doi:10.1038/s41591-018-0035-5
- Teede HJ, Misso ML, Costello MF, Dokras A, Laven J, Moran L, Piltonen T, Norman RJ, Network IPCOS. 2018. Recommendations from the international evidence-based guideline for the assessment and management of polycystic ovary syndrome. Fertil Steril. 110:364–379.
- Troisi R, Potischman N, Harger G, Markovic N, Cole B, Lykins D, Siiteri P, Hoover RN. 2003a. Correlation of serum hormone concentrations in maternal and umbilical cord samples. Cancer Epidemiol Biomarkers Prev. 12:452–456.
- Troisi R, Potischman N, Roberts J, Siiteri P, Daftary A, Sims C, Hoover RN. 2003b. Associations of maternal and umbilical cord hormone concentrations with maternal, gestational and neonatal factors. Cancer Causes Control. 14:347–355.
- Tuutti EK, Hamalainen EK, Sainio SM, Hiilesmaa VK, Turpeinen UL, Alfthan HV, Stenman UH. 2011. Serum testosterone levels during early pregnancy in patients developing preeclampsia. Scand J Clin Lab Invest. 71:413–418.
- van de Beek C, Thijssen JH, Cohen-Kettenis PT, van Goozen SH, Buitelaar JK. 2004. Relationships between sex hormones assessed in amniotic fluid, and maternal and umbilical cord serum: what is the best source of information to investigate the effects of fetal hormonal exposure? Horm Behav. 46:663–669.
- Vanky E, Carlsen SM. 2012. Androgens and antumullerian hormone in mothers with polycystic ovary syndrome and their newborns. Fert Steril. 97:509–515.
- Visser JA, Durlinger AL, Peters IJ, van Den Heuvel ER, Rose UM, Kramer P, de Jong FH, Themmen AP. 2007. Increased oocyte degeneration and follicular atresia during the estrous cycle in anti-Müllerian hormone null mice. Endocrinology. 148:2301–2308.
- Zawadski JK, Dunaif A. 1992. Diagnostic criteria for polycystic ovary syndrome: towards a rational approach. In: Dunaif A, Givens JR, Haseltine F, editors. Polycystic Ovary Syndrome. Boston (MA): Boston Scientific; p. 377–384.