ABSTRACT
This study aimed to investigate the protective role of resveratrol (RES) against cisplatin (Cis)-induced testicular damage and reproductive dysfunction in rats and to examine the underlying mechanisms of protection including its effect on endoplasmic reticulum (ER) stress, P53, extracellular signal-regulated kinase (ERK)-1/2, stress-activated protein kinase/c-Jun N-terminal kinase (SAPK/JNK), and Protein kinase B (Akt) signaling. Eight-week-old Rats were divided into four groups (n = 12 each) of 1) control group: received normal saline (i.p.) as vehicle for 45 days, 2) RES-treated group: received RES (20 mg/kg, i.p) for 45 days, 3) Cis-treated group: received Cis (3 mg/kg) for 3 days and then continued on normal saline, and 4) Cis + RES-treated group: received Cis for the first 3 days and then continued on RES for the next 45 days. Serum sex hormones levels, sperm parameters, and levels of testicular antioxidant potential and inflammatory mediators were assessed in all rats. In addition, activation of ER stress, P53, ERK1/2, JNK, and Akt and markers of apoptosis were evaluated in rats’ testis. Cis lowered sperm count and motility and increased sperm morphological abnormalities. Testis of Cis-treated rats had low expression of antioxidant enzymes including SOD, CAT, and GPx and decreased the level of GSH. Concomitantly, Cis upregulated levels of cleaved caspase-3, P53, calpain-1/cleaved caspase-12, p-ERK1/2, and p-SAPK/p-JNK. However, RES administration post-Cis administration restored all sperm parameters and prevented testicular apoptosis mediated by inhibition of all above-mentioned apoptotic pathways. Moreover, RES enhanced testosterone, FSH, and LH levels and upregulated p-Akt/p-Bad levels in both control and Cis-treated rats. In conclusion, RES protects against Cis-induced testicular damage and reproductive dysfunction via improving testosterone levels, increasing sperm count, reducing testicular apoptosis via an antioxidant potential, inhibition of ER stress, P53, ERK1/2, JNK, and activation of Akt.
Abbreviations: RES: resveratrol, Cis: cisplatin; ER: endoplasmic reticulum; ERK1/2: extracellular signal-regulated kinase1/2; SAPK/JNK: stress-activated protein kinase/c-Jun N-terminal kinase; Akt: protein kinase B; HPG axis: hypothalamic-pituitary-gonadal axis; PUFAs: polyunsaturated fatty acids; FSH: Follicular stimulating hormone; LH: Luteinizing hormone; PBS: phosphate buffered saline; GSH: reduced glutathione; GSSG: glutathione disulfide; TNF-α: tumor necrosis factor-α; IL-6: interleukin-6; GRx: glutathione reductase; SOD: superoxide dismutase; CAT: catalase; 4HNE: 4-hydroxynonenal.
Introduction
Cisplatin (Cis) is a platinum-containing anticancer drug that is widely used to treated treat solid neoplasms, including head, neck, lung, colorectal, hematologic, ovarian, and testicular cancer (Prestayko et al. Citation1979; Lebwohl et., Citation1998; Koster et al. Citation2013). The antineoplastic cytotoxic effects of Cis and its analogs are mediated by the formation of Cis-DNA adducts and DNA damage which eventually culminates in irreversible apoptosis (Delmastro et al. Citation1997; Desoize and Madoulet Citation2002).
DNA damage activates various pro-apoptotic signaling pathways including P53 (Persons et al. Citation2000), P73 (Gong et al. Citation1999), and mitogen-activated protein kinases (MAPKs) (Omar et al. Citation2016). All three MAPKs including extracellular signal-regulated kinase (ERK)-1/2, stress-activated protein kinase/c-Jun N-terminal kinase (SAPK/JNK), and P38 MAPK (Sanchez-Perez and Perona, Citation1998; Nowak et al. Citation2002; Wang et al. Citation2003; Zhang et al. Citation2007) were shown to be deferentially activated by Cis in different cell types. Moreover, Cis can be catalytically converted into Cis-thiol conjugates by Glutathione (GSH)-S-transferaseπ, leading to the rapid depletion of GSH stores, generation of reactive oxygen species (ROS), and inhibition of antioxidant enzymes (Sakamoto et al. Citation2001).
However, Cis treatment is coupled with cellular resistance and only 15–20% of patients respond to the treatment (Siddik Citation2003). This has been attributed to the activation of some survival signaling pathways and inactivation of Cis by the thiol-conjugation (Siddik Citation2003). Hence, a dose scale is necessary to overcome this resistance (Omar et al. Citation2016). Unfortunately, this leads to severe cytotoxicity in normal non-target tissues, including, ototoxicity (Erdem et al. Citation2012), hepatotoxicity (Waseem et al. Citation2015), nephrotoxicity (dos-Santos et al. Citation2012), and reproductive toxicity (Amin et al. Citation2012).
In this regard, the reproductive toxicity Cis therapy is well reported in both animals (Cherry et al. Citation2004; Ateşşahin et al. Citation2006; Amin et al. Citation2008) and humans (Anderson et al. Citation1995; Boekelheide, Citation2005). Reduced sperm viability, count, and motility as well as increased abnormal sperm morphology, increased germ cells apoptosis, compromised testicular endocrine function, and temporary or permanent azoospermia have been described in Cis-treated patients (Colpi et al. Citation2004; Jahnukainen et al. Citation2011). Similarly, severe testicular damage, reduced testicular weight, germ cell apoptosis, Leydig cell dysfunction, and steroidogenic dysfunction, reduced testicular antioxidant enzymes activities, as well as disturbed testicular endocrine function, have been described in Cis-treated animals (Vawda Citation1994). Furthermore, some studies showed that Cis extends to accessory sex organs such as epididymis and vas deferens, affecting sperm maturation (Narayana Citation2010; Reddy et al. Citation2016).
However, most of the established work has generally ascribed oxidative stress mediated by increased ROS generation and depletion of antioxidants as the main mechanism by which Cis induces toxicity in mammalian testis (Cherry et al. Citation2004; Ateşşahin et al. Citation2006; Amin et al. Citation2008; Narayana Citation2010; Reddy et al. Citation2016). Hence, the sustained ratio of affected people despite antioxidant therapy suggests a lack of knowledge about the exact molecular mechanisms by which Cis causes reproductive toxicity and these mechanisms remain to be elucidated for a better therapeutic outcome (Cherry et al. Citation2004; Ateşşahin et al. Citation2006; Amin et al. Citation2008).
Within this field, much effort has been performed to search for novel protective agents to overcome Cis-induced reproductive toxicity and much of the work was oriented to use natural antioxidants (Salem et al. Citation2012; Surendran et al. Citation2012). Resveratrol (trans-3,5,4ʹ-trihydroxystilbene; RES) is a well-known natural compound that has been used to alleviate numerous disease conditions (Baur and Sinclair Citation2006). The beneficial effects of RES have been referred to its capability to act as a chemopreventive (Wolter et al. Citation2004), anti-inflammatory (Donnelly et al. Citation2004), and a potent antioxidant agent (Cai et al. Citation2003). In healthy rodents, RES has a good impact on most semen parameters, sperm production, testosterone levels, and penile erection in control or stress conditions (Juan et al. Citation2005; Shin et al. Citation2008; Eleawa et al. Citation2014). In an experiment performed on animal models of cadmium chloride-induced reproductive toxicity, RES protected rat’s testis and restored steroidogenesis and reproductive function via an antioxidant potential and inhibition of apoptosis (Eleawa et al. Citation2014). Interestingly, in both rats and mice, RES protected against Cis-induced testicular damage by reducing oxidative stress and lipid peroxidation and boosting testicular antioxidant potential and antioxidant enzyme levels (Reddy et al. Citation2016; Singh et al. Citation2017). However, those authors have suggested further research to understand the mechanisms by which RES affords protection against Cis-induced reproductive dysfunction.
The mechanisms by which Cis induces testicular damage and reproductive toxicity are still interesting and need further investigation. Hence, this study was designed to investigate the molecular mechanisms by which Cis induces testicular apoptosis and by which RES affords its protection. In this regard, endoplasmic reticulum (ER) stress, MAPKs, and Akt were targeted.
Results and discussion
This study confirms the protective action of RES against Cis-induced reproductive toxicity, alterations in sperm parameters, and testicular apoptosis. Mechanisms of action afforded by RES at least attributed to its potent antioxidant potential, activation of hypothalamic-pituitary-gonadal (HPG) axis, activation of some survival pathways such as p-Akt/p-Bad survival pathway, and inhibition of Cis-induced-activation of major apoptotic pathways including ERK1/2, SAPK/JNK, and ER stress.
In general, Cis adversely affects the hypothalamus and/or pituitary and inhibits the HPG axis leading to a state of hypergonadotropic hypogonadism which is clinically characterized by reduced circulatory levels of FSH, LH, and testosterone and subsequent reduction in sperm count (Pogach et al. Citation1989; Tousson et al. Citation2014). Previous reports have shown increased levels of FSH, LH, and testosterone in control rats after RES therapy (Eleawa et al. Citation2014). Consistent with these findings, data of the current study show that levels of testosterone, FSH, and LH were significantly increased in the serum of control rats treated with RES (p > 0.001), decreased in the serum of Cis-treated rats (p ≤ 0.005), and returned to their baseline levels in the serum of Cis + RES-treated rats, as compared to control rats (). The stimulatory effect of RES on HPG axis could be explained by the ability of RES to bind estrogen receptor (ER) as a mixed weak agonist/antagonist without estrogenic properties leading to hypophisary stimulation and HPG axis (Juan et al. Citation2005). In addition, this could represent the protective effect of RES on the hypothalamus and/or pituitary due to its antioxidant potential, which was not confirmed in this study and needs further investigation.
Figure 1. Levels of testosterone (A), follicular stimulating hormone (FSH) (B) and luteinizing hormone (LH) (C) in the serum of all experimental groups of rats. All measurements were done by ELISA. Values are expressed as Mean ± SD for 12 rats in each group. Values were considered significantly different at p < 0.05. aSignificantly different when compared to the control group, bsignificantly different when compared to resveratrol (RES)-treated group and csignificantly when compared to cisplatin (Cis)-treated group.
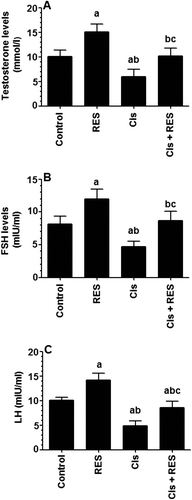
Cis therapy can adversely affect sperm parameters including count, motility and morphology, and testicular weight, resulting in severe testicular damage (Colpi et al. Citation2004; Jahnukainen et al. Citation2011). The initial observations related to the protective role of RES on male fertility in this study were the observations of enhanced testicular weights and sperm parameters in Cis + RES-treated rats. While administration of RES to control rats did not affect final body and testis weights nor sperm parameters, RES administration to Cis-treated rats significantly increased the final body and testis weights () and sperm count and motility (p > 0.001) () and significantly decreased the percentages of headless, tailless, and tail-coiled sperm, resulting in fewer total abnormalities (p > 0.001) (), as compared to Cis-treated rats that received the vehicle. Such increase in testicular weights in Cis-treated rats after RES therapy suggest a protective role of RES on testicular structural proteins (Rajkumar and Srinivasan, Citation1991) and could be related to increased testosterone levels and synthesis (as discussed before), enhanced spermatogenesis and sperm production, and/or lowered germ cell apoptosis (Katoh et al. Citation2002; Prahalathan et al. Citation2004; Pandya et al. Citation2012). Interestingly, sperm count and motility were significantly increased in control rats treated with RES (), which is in accordance with some previous reports (Juan et al. Citation2005; Shin et al. Citation2008; Eleawa et al. Citation2014).
Table 1. Testes and final body weights in all groups of rats.
Table 2. Characterization of epididymal sperm morphology in groups of rat.
Figure 2. Average sperm count and motility in all groups of rats. Sperm analysis was done using the right cauda epididymis. The total number of sperm and sperm motility were calculated using a hemocytometer. For sperm morphological analysis, sperm structures were visualized under a light microscope after adding a drop of Eosin to sperm suspension. Values were considered significantly different at p < 0.05. aSignificantly different when compared to the control group, bsignificantly different when compared to resveratrol (RES)-treated group and csignificantly when compared to cisplatin (Cis)-treated group.
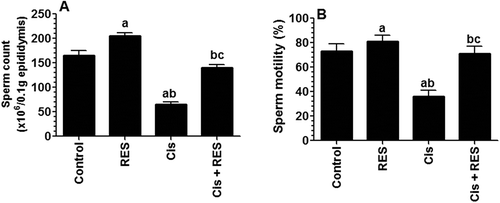
These findings prompted us to further investigate the mechanism of RES protection against Cis-induced alterations in sperm parameters and reproductive dysfunction. Hence, we investigated the effect of RES on testicular oxidative stress and downstream signaling of major death pathways including MAPKs and ER stress as well as some survival pathways. This has been proposed due to four observations. First, the testis is one of the most sensitive organs to oxidative stress due to the high content of germ cell and sperm of polyunsaturated fatty acids (PUFAs) (Koksal et al. Citation2003). Second, oxidative stress-induced germ cell apoptosis has been shown to be a major mechanism of Cis-induced sperm toxicity and reduced motility (Vawda et al. Citation1994; Ateşşahin et al. Citation2006; Amin et al. Citation2008; Chirino and Pedraza-Chaverri Citation2009; Reddy et al. Citation2016). Third, Cis can directly act as an activator of various stress-signaling pathways within the cell at the levels of the cell membrane, cytoplasm, and nucleus to induce apoptosis (Boulikas et al. Citation2003; Wang and Lippard Citation2005). Fourth, RES is a potent scavenger of hydroxyl, superoxide, and metal-induced radicals that was shown to successfully prevent and ameliorate Cis and other reproductive toxic agents-induced testicular and other damage and alterations in sperm parameter in laboratory animals (Amin et al. Citation2012; Juan et al. Citation2005; Ateşşahin et al. Citation2006; Shin et al. Citation2008; Ilbey et al. Citation2009; Rezvanfar et al. Citation2013; Eleawa et al. Citation2014; Keshtmand et al. Citation2014; Waseem et al. Citation2015; Eid et al. Citation2016; Singh et al. Citation2017).
ROS can induce lipid peroxidation and rapid loss of ATP, both of which lead to decrease sperm motility, axonemal damage, morphologic defects, and apoptosis (Sharma and Agarwal Citation1996; Sikka et al. Citation1996). Cis can conjugate with GSH, leading to rapid depletion of GSH stores (Sakamoto et al. Citation2001) and significant reductions in antioxidant enzymes levels including SOD and GPx, which ultimately increases the levels of testicular and sperm ROS (Amin et al. Citation2012). Moreover, Cis-induced ROS can induce TNF-α (Amin et al. Citation2012; Ilbey et al. Citation2009; Sherif et al. Citation2014; Eid et al. Citation2016; Hamza et al. Citation2016), which can also exaggerate levels of ROS and vice versa (Yao et al. Citation2007).
In support, significant increases in the levels of TNF-α and IL-6 (p > 0.0001), 4-HNE (a marker of PUFA oxidation) (p > 0.002), GSSG (p > 0.0001), with concomitant significant decreases in the levels of GSH (p > 0.0001) and protein levels of SOD, CAT, and GPx (p > 0.001) were seen in the testis of Cis-treated rats as compared to control rats ( and ). This supports the trigger mechanism of Cis-induced reductions in sperm count parameters as well as testicular cells apoptosis, as discussed later. Interestingly, testicular levels of these chemical endpoints were returned to their basal levels in Cis + RES-treated rats ( and ). Among all and in control rats, RES therapy only increased the expression of SOD with no effect on all other antioxidant parameters. This suggests that RES mechanism of action is mediated at least by the upregulation of SOD in both control and Cis-intoxication conditions and/or ROS scavenging under stress conditions. In support, Eleawa et al. (Citation2014) have also shown an upregulatory effect of RES on testicular SOD levels but in a different animal model of cadmium chloride-induced testicular damage.
Figure 3. Levels of reduced glutathione (GSH), glutathione disulfide (GSSG) (A), tumor necrosis factor-α (TNF-α) (B) and interleukin-6 (IL-6) (C) in the testis homogenates of all groups of rats. Levels of GSH and GSSG were measured by colorimetric-based kits were as levels of TNF-α and interleukin-6 (IL-6) were measured by ELISA. Values are expressed as Mean ± SD for 6 rats in each group. Values were considered significantly different at p < 0.05. aSignificantly different when compared to the control group, bsignificantly different when compared to resveratrol (RES)-treated group and csignificantly when compared to cisplatin (Cis)-treated group.
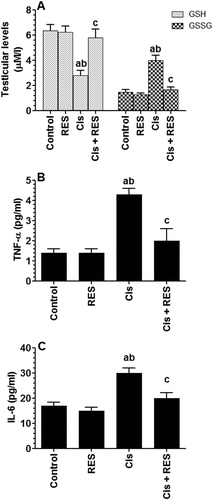
Figure 4. Protein levels of glutathione reductase (GRx), superoxide dismutase (SOD), catalase (CAT) and 4-hydroxynonenal (4HNE) in the testis of all groups of rats as relatively expressed to the reference protein, GAPDH. For western blotting, equal volumes of protein sample (60 µg protein/well) were separated by an SDS electrophoresis, transferred to nitrocellulose membrane and then incubated with the primary and corresponding horseradish peroxidase-conjugated antibodies. Antigen-antibody complexes were then visualized using a Pierce ECL kit and images were quantified using Image J software. Values are expressed as Mean ± SD for 6 rats in each group. Values were considered significantly different at p < 0.05. aSignificantly different when compared to the control group (lane 1), bsignificantly different when compared to resveratrol (RES)-treated group (Lane 2) and csignificantly when compared to cisplatin (Cis)-treated group (Lane 3). Lane 4: Cis +RES-treated group.
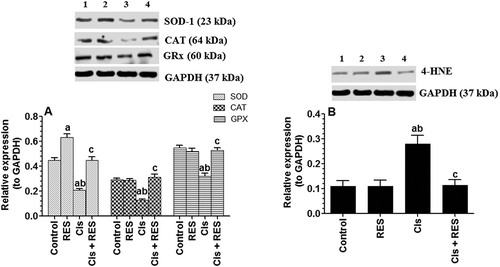
Associated with the increased oxidative stress response of this study, apoptosis was confirmed in the testis of Cis-treated rats by the increased expression of cleaved caspase-3 and reduced its expression after RES therapy (). Several factors including ROS, inflammatory cytokines, DNA damage, and sex hormonal deprivation (testosterone and FSH) can initiate apoptosis in rodents’ testis (Blanco-Rodriguez and Martinez-Garcia Citation1998, Citation2000; Yan et al. Citation2000; Cottet et al. Citation2002; Wang et al. Citation2003; Zhang et al. Citation2007). All these factors were observed in Cis-treated rats. This suggests that the amelioration of the above-mentioned pathways by RES resolve why these rats have reduced cleaved caspase-3 in their testis.
Figure 5. Protein levels of components of intrinsic (mitochondrial) apoptotic pathway in the testis of all groups of rats as relatively expressed to the reference protein, GAPDH. For western blotting, equal volumes of protein sample (60µg protein/well) were separated by an SDS electrophoresis, transferred to nitrocellulose membrane and then incubated with the primary and corresponding horseradish peroxidase-conjugated antibodies. Antigen-antibody complexes were then visualized using a Pierce ECL kit and images were quantified using Image J software. Values are expressed as Mean ± SD for 6 rats in each group as compared to corresponding GAPDH levels. Values were considered significantly different at p < 0.05. aSignificantly different when compared to control the group (lane 1), bsignificantly different when compared to resveratrol (RES)-treated group (Lane 2) and csignificantly when compared to cisplatin (Cis)-treated group (Lane 3). Lane 4: Cis+ RES-treated group. L: Ladder.
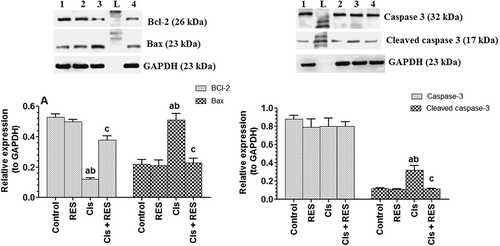
In comparison, sustained ERK activation was shown to mediate Cis-induced apoptosis in various cell lines and in Cis-induced renal damage (Wang et al. Citation2000; Yeh et al. Citation2002; Choi et al. Citation2004; Jo et al. Citation2005; Kim et al. Citation2005; Dhanasekaran and Reddy Citation2008). Hence, it was worth in this study to investigate the role of this pathway in Cis-induced testicular apoptosis. In this study, levels of p-p42-MAPK and p-p45-SAPK/JNK were significantly increased (p > 0.05) in Cis-treated rats, indicating activation of these pathways. Similar to our findings (), Wang et al. (Citation2000) have reported an activation of both pathways in tumor cells after Cis treatment. However, while RES administration did not significantly alter the testicular protein levels of p-p42/p44 MAPKs (ERK1/2) and p-p45/p54 SAPK/JNK in treated control rats, levels of phosphorylated protein of both pathways were returned to their normal levels in the testis Cis + RES treated rats (), indicating that RES acts directly (modulation) or indirectly (reduction in ROS) by inhibition of ERK1/2 and JNK pathways.
Figure 6. Protein levels of phospho-p42 and phosphor-p44 mitogen-activated protein kinases (ERK1/2) and phospho-P45 and phosphor-P54 stress-activated protein kinase/Jun-amino-terminal kinase (SAPK/JNK) pathway in the testis of all groups of rats as expressed to the reference protein, GAPDH. For western blotting, equal volumes of protein sample (60µg protein/well) were separated by an SDS electrophoresis, transferred to nitrocellulose membrane and then incubated with the primary and corresponding horseradish peroxidase-conjugated antibodies. Antigen-antibody complexes were then visualized using a Pierce ECL kit and images were quantified using Image J software. Values are expressed as Mean ± SD for 6 rats in each group. Values were considered significantly different at p < 0.05. aSignificantly different when compared to control group (lane 1), bsignificantly different when compared to resveratrol (RES)-treated group (Lane 2) and csignificantly when compared to cisplatin (Cis)-treated group (Lane 3). Lane 4: Cis + RES--treated group.
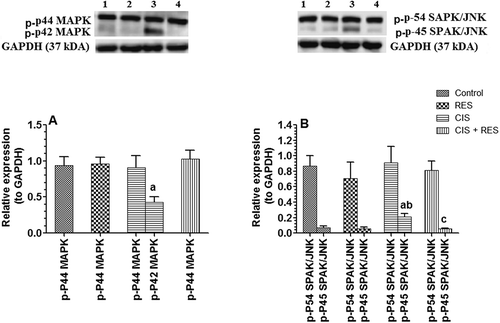
In association, stable baseline levels of Bcl-2, Bax, and cleaved caspase-3 were detected in the testis of control rats administered RES (). Significant decreases in the protein levels of Bax and cleaved caspase-3 and significant increases in protein levels of 6Bcl-2 were seen in Cis + RES compared to Cis-treated rats received the vehicle (p > 0.0001) () were observed. Interestingly, protein levels of Bcl-2, Bax, and cleaved caspase-3 were not significantly different when Cis + RES rats were compared to control rats.
Moreover, significant increases in protein levels of Bax (p > 0.0001) () and P53 (p = 0.006) () with a parallel decrease in protein levels of Bcl-2 (p > 0.0001) () were seen in the testis of Cis-treated rats, suggesting activation of intrinsic cell death. This could be caused by the direct effect of ROS, increases in P53, and induced ER stress by Cis, as shown later in this study. Indeed, ROS can directly induce Bax and inhibit Bcl-2 expression in numerous cells (Chang et al. Citation2002; Hildeman et al. Citation2003; Pugazhenthi et al. Citation2003). However, significant decreases in protein levels of Bax (p < 0.0001) () and P53 (p = 0.002) () and significant increases in the protein levels of Bcl-2 (p > 0.0001) () were seen in Cis + RES whereas stable baseline levels all these markers were seen in the testis of control + RES-treated rats (). These data suggest that inhibition of ERK1/2, JNK, Bax, and P53 as are secondary to inhibition of ROS generation after RES treatments in Cis-treated rats.
Figure 7. Protein levels of P53 in the testis of all groups of rats. Values are expressed as Mean ± SD for 6 rats in each group as relatively expressed to the reference protein, GAPDH. For western blotting, equal volumes of protein sample (60µg protein/well) were separated by an SDS electrophoresis, transferred to nitrocellulose membrane and then incubated with the primary and corresponding horseradish peroxidase-conjugated antibodies. Antigen-antibody complexes were then visualized using a Pierce ECL kit and images were quantified using Image J software. Values were considered significantly different at p < 0.05. aSignificantly different when compared to the control group (lane 1), bsignificantly different when compared to resveratrol (RES)-treated group (Lane 2) and csignificantly when compared to cisplatin (Cis)-treated group (Lane 3). Lane 4: Cis +RES–treated group.
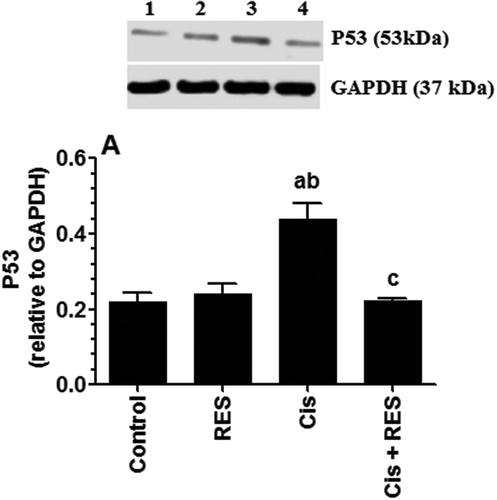
Cis-induced testicular damage was also shown to be mediated by activation of ER stress (Soni et al. Citation2016). Indeed, ROS and accumulation of unfolded protein can imitate ER with all cells and the reverse is correct (Harding et al. Citation2003; Cullinan and Diehl Citation2004; Haynes et al. Citation2004; Mauro et al. Citation2006). Accordingly, testis of Cis-treated rats had significantly higher protein levels of cleaved caspase-12 (p > 0.0001) and calpain-1 (p = 0.003) as compared to control rats (). Caspase-12 is a key player of ER stress-induced caspase-3-induced apoptosis (Rao et al. Citation2002). Calpain-1 is the key protein facilitating caspase-12 activation through cleavage of Bcl-XL (Nakagawa and Yuan, Citation2000). CHOP, a transcription factor induced under ER stress mediates cell apoptosis mainly by reducing the expression of Bcl-2 (McCullough et al. Citation2001). Similarly, sustained activation of inositol-requiring enzyme-1 (IRE-1), another mediator of ER stress, recruits TRAF2 and activates it downstream JNK signaling to inhibit Bcl-2 expression (Auf et al. Citation2010). These two factors could be an alternative source explaining the increases in JNK signaling and the decreases of Bcl-2 protein levels observed in the testis of Cis-treated rats (Harding et al. Citation2003; Cullinan and Diehl Citation2004; Haynes et al. Citation2004; Mauro et al. Citation2006). Administration to control rats did not cause any significant changes in the testicular levels of cleaved caspase-12 nor calpain-1, as compared to control rats, received the vehicle, testis of Cis-treated rats had significantly higher protein levels of cleaved caspase-12 (p > 0.0001) and calpain-1 (p = 0.003) as compared to Cis-treated rat (), confirming that such effect is mainly mediated by the antioxidant potential of RES.
Figure 8. Protein levels of total and cleaved caspase-12 as well calpain-1 in the testis of all groups of rats as compared to corresponding GAPDH levels as relatively expressed to the reference protein, GAPDH. Values are expressed as Mean ± SD for 6 rats in each group. For western blotting, equal volumes of protein sample (60µg protein/well) were separated by an SDS electrophoresis, transferred to nitrocellulose membrane and then incubated with the primary and corresponding horseradish peroxidase-conjugated antibodies. Antigen-antibody complexes were then visualized using a Pierce ECL kit and images were quantified using Image J software. Values were considered significantly different at p < 0.05. aSignificantly different when compared to the control group (lane 1), bsignificantly different when compared to resveratrol (RES)-treated group (Lane 2) and csignificantly when compared to cisplatin (Cis)-treated group (Lane 3). Lane 4: Cis + RES--treated group.
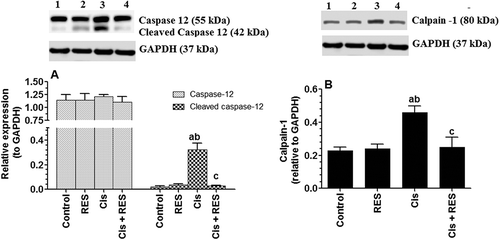
Interestingly, in the current study, Cis has no effect on p-Akt/p-Bad proteins levels, major components of PI3K/Akt/Bad survival pathway (). However, RES treatment significantly increased protein levels of p- P-Akt (Ser473) and p-BAD (Ser112) in the testis of both the control and Cis-treated rats, suggesting a regulatory role of RES on this pathway (). Activation of this pathway is known to induce cell survival and inhibition of intrinsic apoptosis machinery (Datta et al. Citation1997). In this pathway, p-Akt stimulates the phosphorylation of Bad, which results in its dissociation from Bcl-XL and association with the adapter protein 14-3-3 (Datta et al. Citation1997). The free Bcl-XL promotes cell survival. Moreover, another downstream regulator of p-Akt is procaspase 9, which is inactivated by phosphorylation (Cardone et al. Citation1998). These data suggest an activation of p-Akt/p-Bad antiapoptotic pathway by RES which are mainly effective under stress conditions. A brief summary of the mechanism of action of RES in this study is shown in . Four Cis-treated rats died which contradicts the previous findings of Reddy et al. (Citation2016) who showed the safety of this dose in rats. The only variation between our study and his study is the source of Cis as well as the age of the rats. The latter is the most likely variation that resulted in the death of this animal. In Reddy’s study, the animal age was 3 months and their average weight was 201 ± 10 g. However, the average weight of the animals in this study was 180 ± 10 g (8 weeks old). Hence, it could be possible that the age of the rats plays a role in Cis systemic toxicity. Until now, we are not confident of this hypothesis and further studies with respect to this aspect are required. A post-mortem examination revealed increases in blood urea and lowered protein levels with no alterations in liver function enzymes or hematological parameters (data not shown), indicating kidney dysfunction/and damage, which were all normal in the remaining survival rats of the same group. However, RES is a potent nephroprotective agent against numerous oxidative stress conditions and Cis-induced nephrotoxicity. This could explain the increased survival rate of Cis + RES-treated animals. Together, this study provides evidence for the protective role of RES against Cis-induced alteration in sperm parameters and testicular apoptosis in rats. Such findings support considering RES therapy in clinical trials against some common reproductive disorders.
Figure 9. Protein of Akt, phosphor-Akt and Bad pathway in the testis of all groups of rats as expressed to the reference protein, GAPDH.Values are expressed as Mean ± SD for 6 rats in each group. For western blotting, equal volumes of protein sample (60 µg protein/well) were separated by an SDS electrophoresis, transferred to nitrocellulose membrane and then incubated with the primary and corresponding horseradish peroxidase-conjugated antibodies. Antigen-antibody complexes were then visualized using a Pierce ECL kit and images were quantified using Image J software. Values were considered significantly different at p < 0.05. aSignificantly different when compared to the control group (Lane 1), bsignificantly different when compared to resveratrol (RES)-treated group (Lane 2) and csignificantly when compared to cisplatin (Cis)-treated group (Lane 3). Lane 4: Cis +RES–treated group. L: Ladder.
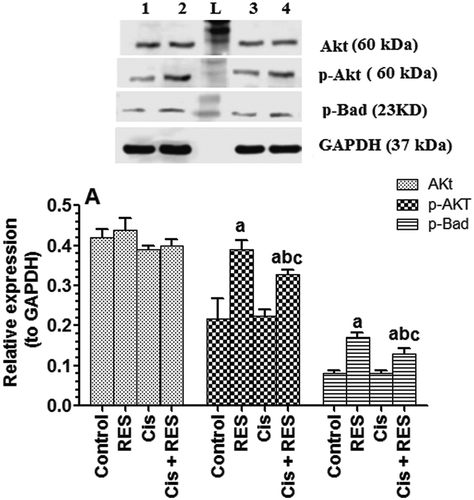
Materials and methods
Antibodies
Antibodies against P-SAPK/JNK (p46 and p54, Thr183/Tyr185) (cat. no. 9251), Akt (cat. no. 9272, 60 kDa), p-Akt (Ser473) (cat. no. 9271, 60 kDa), p-Bad (Ser112) (cat. no. 9291, 23 kDa), Caspase 3 (32 kDa) and its resulted cleaved large fragment (17 kDa) (cat. no. 9662), Caspase-12 (55 kDa), and its cleaved fragment (42 kDa) (cat. no. 2202) and GAPDH (cat. no 2118) were purchased from Cell Signaling Technology, USA. Antibodies against Calpain 1 (cat. no. H-65/sc-13990, 80 kDa), Bax (cat. no. sc-6236, 23 kDa), Bcl-2 (cat. no. C2/sc-7382, 26 KDa), p-53 (cat. no. FL-393/sc-6243), 4-hydroxynonenal (4HNE, cat. no. sc-130083), glutathione reductase (GRx, cat. no. sc-32886, 55–65 kDa), SOD-1 (cat. no. C-17/sc-8637, 23 kDa), and catalase (cat. no. sc-34,285, 64 kDa) were purchased from Santa Cruz Biotechnology, CA, USA.
Animals
This study was approved by the Ethical Committee of the College of Science, King Khalid University, Abha, Saudi Arabia. The guidelines for the care and use of laboratory animals published by the US National Institutes of Health (NIH publication No. 85-23, revised 1996) were followed. Pathogen-free male Wistar rats in their late puberty (8 weeks old; 180 ± 10 g) were supplied from the animal house of the College of Science, King Khalid University, Abha. During habituation and the experimental procedure, all animals were lived in plastic cages (4/cage) under a controlled ambient temperature of 23 ± 1°C with controlled 12-h light/12-h dark cycles. They were always fed standard rat pellets and allowed free access to water.
Experimental procedures
After the adaptation period of 2 weeks, a total of 48 rats were randomly divided into four groups (n = 12 each) as follows: 1) control group: received 1 ml of normal saline (0.9% NaCl), 2) A RES-treated group: received 1 ml of RES at a dose of 20 mg/kg body weight (bwt), 3) Cis-treated group: received 1 ml cisplatin at a dose of 3 mg/kg for the first 3 days of the study period and then received 1 ml of normal saline for the rest of the days (Reddy et al. Citation2016), and 4) Cis + RES-treated group: received Cis as in group 3 and then received RES (1 ml, 20 mg/kg) daily for the rest of the treatment period. All treatments were given intraperitoneally (i.p.) and the experimental procedures were carried out for consecutive 45 days. Cis (cat. no. 232120, ˃98 purity) and RES (cat. no R5010, ˃99 purity) and 2-hydroxypropyl – β-cyclodextrin (cat. no. 332607, ˃99 purity) were purchased from Sigma Aldrich, USA. Cis was dissolved in normal saline whereas RES was dissolved in a saline solution (0.9% NaCl) of 20% of 2-hydroxypropyl – β-cyclodextrin.
This regimen of Cis treatment was selected based on published work showing that long-term failure of spermatogenesis and germ cell apoptosis with the complete survival of all animals without over toxicity in rodents treated with 3 mg/kg Cis i.p. for the first 3 days (Reddy et al. Citation2016). Moreover, the dose of RES and i.p. route of administration were selected based on the studies of (Reddy et al. Citation2016) and (Eleawa et al. Citation2014) who showed that RES at this dose and route of administration are effective in exerting their protective effects in rat’s testis intoxicated with Cis or cadmium. The treatment period of 45 days was chosen because the study aimed to evaluate the effect of RES and Cis on complete testicular spermatogenic cycle, as shown by Clermont (Citation1972).
Serum and tissue collection
Twelve hours after the last treatment, all rats were weighed and anesthetized using sodium pentobarbital (60–70 mg/kg, i.p.) and blood samples were collected by cardiac puncture to collect serum, which was used later to determine the levels of sex hormones. Then, all animals were killed by cervical decapitation, and both testes were removed and transferred into Petri dishes where they were cleaned from the surrounding adipose tissues, connective tissues, and blood vessels and weighted. The left testis was dissected into smaller parts and stored at −80°C and used for biochemical and western blot evaluation. The right epididymis of all rats (n = 12 rats/group) were also collected, weighted, and used fresh for sperm count and analysis.
Measurements of hormones levels
Special rats ELISA kits were used to measure the serum levels of testosterone (cat. no. E0930Ra, Shanghai Crystal Day Biotech Co., Ltd. China) follicular stimulating hormone (FSH) (cat. no. ab108641, Cambridge, UK) and luteinizing hormone (LH) (cat. no. CSB-E12654r, Cusabio Biotech Co., Ltd., China). Absorbance was read using the 800TM TS absorbance/plate reader and supported Gen5 software. All procedures followed the manufacturer’s instructions.
Semen analysis
Sperm count was performed according to the procedure followed by Eleawa et al. (Citation2014). In brief, the right cauda epididymis from each rat diluted in 1:20 with normal saline and kept for 5 min at 37°C in Petri dish after being minced with a scalpel. The total number of sperm was counted at 400× in 5 small squares of the main central area of a glass hemocytometer. A dilution factor of 20 and a correction factor of 50 were applied to calculate total sperm count per milliliter and converted to 0.1 g weight. Samples were analyzed in duplicate. Furthermore, motile and immotile sperm were counted in a total of 400 sperm sample, and the results were expressed in per cent. For sperm morphological analysis, sperm structures were visualized under a light microscope after adding a drop of eosin to sperm suspension. For this procedure, four fields of each sample (each of 200 sperm) were visualized and counted and average abnormality was calculated and presented as a percentage. The absence of head, the absence of tails, tail bending, tail coiling, mid-piece curving, and mid-piece bending were observed. All procedures were analyzed in duplicate and mean readings were used for each rat.
Biochemical measurements in the testis
Half of the left testis obtained from 6 rats/group were washed with ice-cold phosphate-buffered saline (PBS), pH 7.4, containing 0.16 mg/ml of heparin to remove any red blood cells (erythrocytes) and clots. Then they were homogenized with an ultrasonic homogenizer in cold phosphate buffer, pH 7.0 for the determination of glutathione/glutathione disulfide (GSH/GSSG) content using a commercially available kit supplied by Cayman Chemical, Ann Arbor, MI, USA (cat. no. 703002). Levels of tumor necrosis factor-α (TNF-α) were measured using ELISA kit purchased from Abcam, Cambridge, MA, USA (cat no. ab46070). The levels of interleukin-6 (IL-6) were measured using an ELISA kit supplied by RayBio, MO, USA (cat no. ELR-IL6-001). Absorbance was read using the 800TM TS absorbance/plate reader and supported Gen5 software. All procedures followed the manufacturer’s instructions.
Western blot analysis
For western blot analysis, whole cell homogenates (n = 6) including nuclear and membrane proteins were extracted from the frozen other half of the left testis by RIPA buffer (150 mM sodium chloride, 1.0% NP-40 or Triton X-100, 0.5% sodium deoxycholate 0.1% SDS and 50 mM Tris, pH 8.0) to which protease (cat. no. P8340, Sigma-Aldrich, St. Louis, MO, USA) and phosphatase (PhosSTOP, cat. no. PHOSS-RO Sigma-Aldrich, St. Louis, MO, USA) inhibitor cocktails were added. The protein concentrations were measured using the Bradford assay. The sample, diluted in loading buffer and heated at 95°C for 5 min, was then subjected to electrophoresis on 10% SDS-PAGE gel at 100 V for 2 h (60 µg protein/well). After the transfer of the proteins to nitrocellulose membrane, the membranes were rinsed, blocked, and incubated with the primary monoclonal and polyclonal antibodies for 2 h then with the corresponding secondary corresponding horseradish peroxidase-conjugated antibodies for another 2 h. Antigen-antibody complexes were then visualized using a Pierce ECL kit (ThermoFisher, USA, Piscataway, NJ) photographed and bands intensities were quantified using Image J software (1.46R, NIH, USA). All experiments were performed in triplicate. Protein levels were presented relatively to GAPDH and data were represented as the mean ± SD of six samples/group.
Statistical analysis
Statistical analyses were performed by using GraphPad Prism statistical software package (version 6). Data were presented as means with their standard Deviation (mean ± SD). Normality and homogeneity of the data were confirmed before analysis of variance (ANOVA), differences among the experimental groups were assessed by one-way ANOVA followed by Tukey’s t-test. Values were considered significantly different when p ˂ 0.05.
Disclaimers
The views expressed in this submitted article are own by Professor Ali A. Shati and not an official position of any institution or funder.
Acknowledgments
The author would like to thank the technical staff of the animal house and the technical staff at the Department of Biochemistry and Physiology at King Khalid University for their valuable help and measurements of some parameters of the current study.
Disclosure statement
No potential conflict of interest was reported by the author.
Additional information
Funding
Notes on contributors
Ali A. Shati
Professor Ali A. Shati designed the study and the experimental procedure, supervised the experimental treatments and the measurements of the biochemical parameters and performed the semen analysis. He performed all western blot experiments and analysis and performed the statistical analysis. In addition, he wrote the paper and revised the final version of the manuscript. The contributions of other staff members to the current study are further mentioned in the acknowledgment.
References
- Amin A, Abraham C, Hamza A, Abdalla Z, Al-Shamsi S, Harethi S, Daoud S. 2012. A standardized extract of Ginkgo biloba neutralizes Cisplatin -mediated reproductive toxicity in rats. J Biomed Biotechnol. Article Id:362049.
- Amin A, Hamza AA, Kambal A, Daoud S. 2008. Herbal extracts counteract cisplatin-mediated cell death in rat testis. Asian J Androl. 10(2):291–297.
- Anderson D, Bishop JP, Garner RC, Ostrosky-Wegman P, Selby PB. 1995. Cyclophosphamide, review of its mutagenicity for an assessment of potential germ cell risks. Mutat Res-Fund Mol M. 330(1–2):115–181.
- Ateşşahin A, Şahna E, Türk G, Çeribaşi AO, Yılmaz S, Yüce A, Bulmuş Ö. 2006. Chemoprotective effect of melatonin against cisplatin‐induced testicular toxicity in rats. J Pineal Res. 41(1):21–27.
- Auf G, Jabouille A, Guérit S, Pineau R, Delugin M, Bouchecareilh M, Magnin N, Favereaux A, Maitre M, Gaiser T, et al. 2010. Inositol-requiring enzyme 1alpha is a key regulator of angiogenesis and invasion in malignant glioma. Proc Natl Acad Sci USA. 2010 Aug 31;107(35):15553–15558.
- Baur J, Sinclair D. 2006. Therapeutic potential of resveratrol, the in vivo evidence. Nat Rev Drug Discov. 5(6):493–506.
- Blanco-Rodriguez J, Martinez-Garcia C. 1998. Apoptosis precedes detachment of germ cells from the seminiferous epithelium after hormone suppression by short-term oestradiol treatment of rats. Int J Androl. 21(2):109–115.
- Boekelheide K. 2005. Mechanisms of toxic damage to spermatogenesis. J Natl Cancer Inst Monogr Monographs. 34:6–8.
- Boulikas T, Vougiouka M. 2003. Cisplatin, and platinum drugs at the molecular level (Review). Oncol Rep. 10(6):1663–1682.
- Cai Y, Fang J, Ma L, Yang L, Liu Z. 2003. Inhibition of free radical-induced peroxidation of rat liver microsomes by resveratrol and its analogues. Biochim Biophys Acta. 1637(1):313–318.
- Cardone MH, Roy N, Stennicke HR, Salvesen GS, Franke TF, Stanbridge E, Frisch S, Reed JC. 1998. Regulation of cell death protease caspase-9 by phosphorylation. Science. 282(5392): 1318–1321.
- Chang WK, Yang KD, Chuang H, Jan JT, Shaio MF. 2002. Glutamine protects activated human T cells from apoptosis by up-regulating glutathione and Bcl-2 levels. Clin Immunol. 104:151–160.
- Cherry SM, Hunt PA, Hassold TJ. 2004. Cisplatin disrupts mammalian spermatogenesis but does not affect recombination or chromosome segregation. Mutat Res-Gen Tox En. 564(2):115–128.
- Chirino YI, Pedraza-Chaverri J. 2009. Role of oxidative and nitrosative stress in cisplatin-induced nephrotoxicity. Exp Toxicol Pathol. 61:223–242.
- Choi BK, Choi CH, Oh HL, Kim YK. 2004. Role of ERK activation in Cisplatin-induced apoptosis in A172 human glioma cells. Neurotoxicology. 25(6):915–924.
- Clermont Y. 1972. Kinetics of spermatogenesis in mammals, seminiferous epithelium cycle and spermatogonial renewal. Physiol Rev. 52(1):198–236.
- Colpi GM, Contalbi GF, Nerva F, Sagone P, Piediferro G. 2004. Testicular function following chemo-radiotherapy. Eur J Obstet Gynecol Reprod Bio. 113(1):S2–S6.
- Cottet S, Dupraz P, Hamburger F, Dolci W, Jaquet M, Thorens B. 2002. cFLIP protein prevents tumor necrosis factor-α–mediated induction of caspase-8–dependent apoptosis in insulin-secreting βTc-Tet cells. Diabetes. 51(6):1805–1814.
- Cullinan SB, Diehl JA. 2004. PERK-dependent activation of Nrf2 contributes to redox homeostasis and cell survival following endoplasmic reticulum stress. J Biol Chem. 279:20108–20117.
- Datta SR, Dudek H, Tao X, Masters S, Fu H, Gotoh Y, Greenberg ME. 1997. Akt phosphorylation of BAD couples survival signals to the cell-intrinsic death machinery. Cell. 91(2):231–241.
- Delmastro DA, Li J, Vaisman A, Solle M, Chaney S. 1997. DNA damage inducible-gene expression following platinum treatment in human ovarian carcinoma cell lines. Cancer Chemoth Pharm. 39(3):245–253.
- Desoize B, Madoulet C. 2002. Particular aspects of platinum compounds used at present in cancer treatment. Crit Rev Oncol Hemat. 42(3):317–325.
- Dhanasekaran D, Reddy E. 2008. JNK signaling in apoptosis. Oncogene. 27(48):6245–6251.
- Donnelly LE, Newton R, Kennedy GE, Fenwick PS, Leung RH, Ito K, Russell RE, Barnes PJ. 2004. Anti-inflammatory effects of resveratrol in lung epithelial cells: molecular mechanisms. Am J Physiol Lung Cell Mol Physiol. 287:L774–L783.
- dos-Santos NA, Ma R, Nm M, Dos Santos AC. 2012. Cisplatin-induced nephrotoxicity and targets of nephroprotection, an update. Arch Toxicol. 86(8):1233–1250.
- Eid AH, Abdelkader NF, Abd El-Raouf OM, Fawzy HM, El-Denshary ES. 2016. Carvedilol alleviates testicular and spermatological damage induced by cisplatin in rats viamodulation of oxidative stress and inflammation. Arch Pharm Res. 39(12):1693–1702.
- Eleawa SM, Alkhateeb MA, Alhashem FH, Bin-Jaliah I, Sakr HF, Elrefaey HM, Elkarib AO, Alessa RM, Haidara MA, Shatoor AS, et al. 2014. Resveratrol reverses cadmium chloride-induced testicular damage and sub-fertility by down-regulating P53 and bax and upregulating of gonadotropins and Bcl-2 gene expression. J Reprod Dev. 60(2):115–127.
- Erdem T, Bayindir T, Filiz A, Iraz M, Selimoglu E. 2012. The effect of resveratrol on the prevention of Cisplatin ototoxicity. Eur Arch Otorhinolaryngol. 269(10):2185–2188.
- Gong J, Costanzo A, Yang HQ, Melino G, Kaelin WG, Levrero M, Wang JY. 1999. The tyrosine kinase c-Abl regulates p73 in apoptotic response to cisplatin-induced DNA damage. Nature. 399(6738):806–809.
- Hamza AA, Elwy HM, Badawi AM. 2016. Fenugreek seed extract attenuates cisplatin-induced testicular damage in Wistar rats. Andrologia. 48:211–221.
- Harding HP, Zhang Y, Zeng H, Novoa I, Lu PD, Calfon M, Sadri N, Yun C, Popko B, Paules R, et al. 2003. An integrated stress response regulates amino acid metabolism and resistance to oxidative stress. Mol Cell. 11:619–633.
- Haynes CM, Titus EA, Cooper AA. 2004. Degradation of misfolded proteins prevents ER-derived oxidative stress and cell death. Mol Cell. 15:767–776.
- Hildeman DA, Mitchell T, Aronow B, Wojciechowski S, Kappler J, Marrack P. 2003. Control of Bcl-2 expression by reactive oxygen species. Proc Natl Acad Sci USA. 100(25):15035–15040.
- Ilbey YO, Ozbek E, Simsek A, Otunctemur A, Cekmen M, Somay A. 2009. Potential chemoprotective effect of melatonin in cyclophosphamide- and cisplatin-induced testicular damage in rats. Fertil Steril. 92:1124–1132.
- Jahnukainen K, Ehmcke J, Hou M, Schlatt S. 2011. Testicular function and fertility preservation in male cancer patients. Best Pract Res Clin Endocrinol Metab. 25(2):287–302.
- Jo SK, Cho WY, Sung SA, Kim HK, Won NH. 2005. MEK inhibitor, U0126, attenuates Cisplatin-induced renal injury by decreasing inflammation and apoptosis. Kidney Int. 67(2):458–466.
- Juan ME, González-Pons E, Munuera T, Ballester J, Rodríguez-Gil JE, Planas JM. 2005. trans-Resveratrol, a natural antioxidant from grapes, increases sperm output in healthy rats. J Nutr. 135(4):757–760.
- Katoh C, Kitajima S, Saga Y, Kanno J, Horii I, Inoue T. 2002. Assessment of quantitative dual-parameter flow cytometric analysis for the evaluation of testicular toxicity using cyclophosphamide- and ethinylestradiol-treated rats. J Toxicol Sci. 27(2):87–96.
- Keshtmand Z, Oryan S, Ghanbari A, Khazaei M. 2014. Protective effect of tribulus terrestris hydroalcoholic extract against cisplatin-induced cytotoxicity on sperm parameters in male mice. Int J Morphol. 32(2):551–557.
- Kim YK, Kim HJ, Kwon CH, Kim JH, Woo JS, Jung JS, Kim JM. 2005. Role of ERK activation in Cisplatin-induced apoptosis in OK renal epithelial cells. J Appl Toxicol. 25(5):374–382.
- Koksal IT, Usta M, Orhan I, Abbasoglu S, Kadıoglu A. 2003. Potential role of reactive oxygen species on testicular pathology associated with infertility. Asian J Androl. 5:95–99.
- Koster R, Van Vugt M, Timmer-Bosscha H, Gietema JA, de Jong S. 2013. Unravelling mechanisms of Cisplatin sensitivity and resistance in testicular cancer. Expert Rev Mol Med. 15:e12.
- Lebwohl D, Canetta R. 1998. Clinical development of platinum complexes in cancer therapy, a historical perspective, and an update. Eur J Cancer. 34(10):1522–1534.
- Mauro C, Crescenzi E, De Mattia R, Pacifico F, Mellone S, Salzano S, de Luca C, D’Adamio L, Palumbo G, Formisano S, et al. 2006. Central role of the scafford protein TNF-receptor associated factor 2 in regulating endoplasmic reticulum stress-induced apoptosis. J Biol Chem. 281:2631–2638.
- McCullough KD, Martindale JL, Klotz LO, Aw TY, Holbrook NJ. 2001. Gadd153 sensitizes cells to endoplasmic reticulum stress by down-regulating Bcl2 and perturbing the cellular redox state. Mol Cell Biol. 21(4):1249–1259.
- Murgu´Ia S-PJ, Perona R. 1998. Cisplatin induces a persistent activation of JNK that is related to cell death. Oncogene. 16(4):533–540.
- Nakagawa T, Zhu H, Morishima N, Li E, Xu J, Yankner BA, Yuan J. 2000. Caspase-12 mediates endoplasmic-reticulum-specific apoptosis and cytotoxicity by amyloid-beta. Nature. 403(6765):98–103.
- Narayana K. 2010. Cisplatin induces duplex 3ʹ overhangs and 5ʹ blunt ends in the epididymal epithelium in a Bax-dependent manner without any protection from L-ascorbic acid. Eur J Pharmacol. 641(2–3):238–245.
- Nowak G. 2002. PKC-α and ERK1/2 mediate mitochondrial dysfunction, decreases in active Na+ transport, and cisplatin-induced apoptosis in renal cells. J Bio Chem. 277(45):43377–43388.
- Omar HA, Mohamed WR, Arab HH, Arafa ES. 2016. Tangeretin alleviates cisplatin-induced acute hepatic injury in rats, targeting MAPKs and apoptosis. Plos one. 11(3):e0151649.
- Pandya C, Pillai P, Nampoothiri LP, Bhatt N, Gupta S. 2012. Effect of lead and cadmium co-exposure on testicular steroid metabolism and antioxidant system of adult male rats. Andrologia. 44:(S1):813–822.
- Persons DL, Yazlovitskaya E, Pelling JC. 2000. Effect of extracellular signal-regulated kinase on p53 accumulation in response to Cisplatin. J Bio Chem. 275(46):35778–35785.
- Pogach LM, Lee Y, Giglio W, Naumoff M, Huang HF. 1989. Zinc acetate pretreatment ameliorates cisplatin-induced Sertoli cell dysfunction in Sprague-Dawley rats. Cancer Chemother Pharmacol. 24(3):177–180.
- Prahalathan C, Selvakumar E, Varalakshmi P. 2004. Remedial effect of DL-alpha-lipoic acid against adriamycin-induced testicular lipid peroxidation. Mol Cell Biochem. 267(1–2):209–214.
- Prestayko AW, D’Aoust JC, Issell BF, Crooke ST. 1979. Cisplatin (cis-diamminedichloroplatinum II). Cancer Treat Rev. 6(1):17–39.
- Pugazhenthi S, Nesterova A, Jambal P, Audesirk G, Kern M, Cabell L, Eves E, Rosner MR, Boxer LM, Reusch JEB. 2003. Oxidative stress-mediated down-regulation of bcl-2 promoter in hippocampal neurons. J Neurochem. 84:982–996.
- Rajkumar L, Srinivasan N, Balasubramanian K, Govindarajulu P. 1991. Increased degradation of dermal collagen in diabetic rats. Indian J Exp Biol. 29(11):1081–1083.
- Rao RV, Castro-Obregon S, Frankowski H, Schuler M, Stoka V, Del Rio G, Bredesen DE, Ellerby HM. 2002. Coupling endoplasmic reticulum stress to the cell death program. an Apaf-1-independent intrinsic pathway. J Biol Chem. 277:21836–21842.
- Reddy KP, Madhu P, Reddy PS. 2016. Protective effects of resveratrol against Cisplatin-induced testicular and epididymal toxicity in rats. Food Chem Toxicol. 91:65–72.
- Rezvanfar MA, Rezvanfar MA, Shahverdi AR, Ahmadi A, Baeeri M, Mohammadirad A, Abdollahi M. 2013. Protection of cisplatin-induced spermatotoxicity, DNA damage and chromatin abnormality by selenium nano-particles. Toxicol Appl Pharmacol. 266(3):356–365.
- Sakamoto M, Kondo A, Kawasaki K, Goto T, Sakamoto H, Miyake K, Koyamatsu Y, Akiya T, Iwabuchi H, Muroya T, et al. 2001. Analysis of gene expression profiles associated with cisplatin resistance in human ovarian cancer cell lines and tissues using cDNA microarray. Hum Cell. 14(4):305–315.
- Salem E, Salem N, Maarouf A, Serefoglu E, Hellstrom W. 2012. Selenium and lycopene attenuate Cisplatin-induced testicular toxicity associated with oxidative stress in Wistar rats. Urology. 79(5):1184.
- Sharma RK, Agarwal A. 1996. Role of reactive oxygen species in male infertility. Urology. 48:835–850.
- Sherif IO, Abdel-Aziz A, Sarhan OM. 2014. Cisplatin-induced testicular toxicity in rats: the protective effect of arjunolic acid. J Biochem Mol Toxicol. 28:515–521.
- Shin S, Jeon JH, Park D, Jang MJ, Choi JH, Choi BH, Joo SS, Nahm SS, Kim JC, Kim YB. 2008. trans-Resveratrol relaxes the corpus cavernosum ex vivo and enhances testosterone levels and sperm quality in vivo. Arch Pharm Res. 31(1):83–87.
- Siddik ZH. 2003. Cisplatin. mode of cytotoxic action and molecular basis of resistance. Oncogene. 22(47):7265–7279.
- Sikka SC. 1996. Oxidative stress and role of antioxidants in normal and abnormal sperm function. Front Biosci. 1:78–86.
- Singh I, Goyal Y, Ranawat P. 2017. Potential chemoprotective role of resveratrol against cisplatin induced testicular damage in mice. Chem Biol Interact. 273:200–211.
- Soni KK, Kim HK, Choi BR, Karna KK, You JH, Cha JS, Shi YS, Lee SW, Kim CY, Park JK. 2016. Dose-dependent effects of cisplatin on the severity of testicular injury in Sprague Dawley rats: reactive oxygen species and endoplasmic reticulum stress. Drug Des Devel Ther. 10:3959–3968.
- Surendran D, Geetha C, Mohanan P. 2012. Amelioration of melatonin on oxidative stress and genotoxic effects induced by Cisplatin in vitro. Toxicol Mech Method. 22(8):631–637.
- Tousson E, Hafez E, Masoud A, Hassan AA. 2014. Abrogation by curcumin on testicular toxicity induced by cisplatin in rats. J Cancer Res Treat. 2(3):64–68.
- Vawda AI. 1994. Effect of testosterone on Cisplatin-induced testicular damage. Arch Andrology. 32(1):53–57.
- Wang D, Lippard SJ. 2005. Cellular processing of platinum anticancer drugs. Nat Rev Drug Discov. 4(4):307–320.
- Wang X, Martindale J, Holbrook N. 2000. Requirement for ERK activation in Cisplatin-induced apoptosis. JBiol Chem. 275(50):39435–39443.
- Wang X, Sharma RK, Sikka SC, Thomas AJ, Falcone T, Agarwal A. 2003. Oxidative stress is associated with increased apoptosis leading to spermatozoa DNA damage in patients with male factor infertility. Fertil and Steril. 80(3):531–535.
- Waseem M, Bhardwaj M, Tabassum H, Raisuddin S, Parvez S. 2015. Cisplatin hepatotoxicity mediated by mitochondrial stress. Drug ChemToxicol. 38(4):452–459.
- Wolter F, Ulrich S, Stein J. 2004. Molecular mechanisms of the chemopreventive effects of resveratrol and its analogs in colorectal cancer: the key role of polyamines? J Nutr. 134(12):3219–3222.
- Yan W, Samson M, Jégou B, Toppari J. 2000. Bcl-w forms complexes with Bax and Bak, and elevated ratios of Bax/Bcl-w and Bak/Bcl-w correspond to spermatogonial and spermatocyte apoptosis in the testis. Mol Endocrinol. 14(5):682–699.
- Yao X, Panichpisal K, Kurtzman N, Nugent K. 2007. Cisplatin nephrotoxicity: a review. Am J Med Sci. 334:115–124.
- Yeh PY, Chuang SE, Yeh KH, Song YC, Ea CK, Cheng AL. 2002. Increase of the resistance of human cervical carcinoma cells to Cisplatin by inhibition of the MEK to ERK signaling pathway partly via enhancement of anticancer drug-induced NFκB activation. Biochem Pharmacol. 63(8):1423–1430.
- Zhang X, Sanmun D, Hu L, Fadeel B, Ernberg I. 2007. Epstein-Barr virus-encoded LMP1 promotes cisplatin-induced caspase activation through JNK and NF-κB signaling pathways. Biochem Bioph Res Co. 360(1):263–268.