ABSTRACT
Therapeutic radiation of the pelvic region has been shown to cause damage to testicular germ cells. In this study we aimed to evaluate the effects of a low therapeutic dose of 1 Gy on the induction of cellular and histological damage in early-stage testicular germ cells and the impact of this radiation on offspring sex ratio. Unirradiated and irradiated male rats were mated with unirradiated female rats. Female rats were followed and the sex of the offspring was determined. The male rats were sacrificed at the end of the second week, and the testicular germ cells were subjected to genetic analysis along with cytological and histopathological examination. Sperm DNA was amplified with primers specific to testis-specific Y-linked protein, rat actin beta and testis-specific X-linked genes. The resulting products were separated by capillary electrophoresis. Histopathological changes were investigated by light microscopy along with the TUNEL assay and immunohistochemical staining for caspase-3. There was no significant difference between the two groups for sex ratio and size of offspring. The number of sperm cells bearing X or Y chromosomes’ did not differ significantly between these two groups. However, a 1 Gy dose of radiation caused significant cytopathological and histopathological changes in the testicular tissue. In the irradiated group, edematous regions were evident. The number of caspase-3 positive cells in the germinal epithelium of the seminiferous tubules was also significantly higher in the irradiated group. Our results showed that low-dose radiation induced apoptosis and caused significant cyto- and histopathological changes in the testicular tissue. Further research is required to fully elucidate their contribution to apoptosis and if low-dose radiation may potentially lead to long-term effects in the offspring. These results may also lead us to develop a new technique using the caspase-3 staining to monitor the susceptibility to low dose radiation.
Introduction
Radiotherapy is toxic to the gonads acutely affecting reproductive health (Meistrich Citation2013). The effects of cancer radiotherapy on gametes and reproductive organs have not been fully elucidated. A reduced male to female offspring sex ratio away from 1:1 has been considered to be a marker of exposure to a reproductive hazard (Terrell et al. Citation2011). A few studies have proposed a long-term effect of pelvic ionizing radiation in male reproductive tissues by examining the sex ratio the offspring (Hawkins Citation1991; Byrne et al. Citation1998; Winther et al. Citation2003) in ionizing radiation treated childhood cancer survivors. Only limited evidence for a slightly skewed sex ratio was reported (Hawkins Citation1991; Byrne et al. Citation1998; Winther et al. Citation2003).
The biological effects of low-dose radiation have long been noted by researchers (Cai and Liu Citation1990; Cai et al. Citation1993; Cai and Wang Citation1995; Feinendegen Citation2005; Khan et al. Citation2015). Due to the multifaceted effects of radiation exposure and the complex and resistant nature of tissues and systems, adverse effects of low dose radiation remain to be understood (Hasegawa et al. Citation1998; Feinendegen Citation2005; Fang et al. Citation2013; Khan et al. Citation2015; Fatehi et al. Citation2018). The complexity of cellular metabolism and defense systems present a challenge towards understanding this phenomenon (Dewey et al. Citation1995; Hasegawa et al. Citation1997, Citation1998; Seong et al. Citation2001). Testicular tissue presents a dense apoptotic response to ionizing radiation (Sapp et al. Citation1992; Hasegawa et al. Citation1997; Cordelli et al. Citation2003; Feinendegen Citation2005; Khan et al. Citation2015; Da Silva Mansano et al. Citation2018; Fatehi et al. Citation2018) that is attributed to the death of germ cells (Coureuil et al. Citation2010; Khan et al. Citation2015; Silva et al. Citation2016; Da Silva Mansano et al. Citation2018). Spontaneous apoptosis is a physiological process which occurs during germ cell development to eliminate damaged cells in response to a number of external stimuli such as ionizing radiation, chemotherapy, and hormonal manipulations (Dewey et al. Citation1995; Hasegawa et al. Citation1997, Citation1998; Zhang et al. Citation2001; Liu et al. Citation2007; Fang et al. Citation2013). Necrotic effects may also emerge (Hasegawa et al. Citation1998; Feinendegen Citation2005; Fang et al. Citation2013; Fatehi et al. Citation2018). Induction of apoptosis and the process are controlled by numerous mediators. These include ions like calcium; molecules, ceramide; genes, c-myc, p53; enzymes, caspases, and organelles like the endoplasmic reticulum and mitochondria (Zeiss Citation2003; Elmore Citation2007). Caspase-3 is a key effector in the apoptosis pathway which amplifies the signal from the initiator caspases (Thornberry and Lazebnik Citation1998). Immunohistochemical and biochemical detection of a growing number of apoptotic effectors can be performed.
Fertility problems in patients exposed to low-dose radiation and genetic changes in early-stage treatment with ionizing radiation to meet the goal of long-term survival even though detoxifying mechanisms are activated to protect the cell population from harmful effects. Patients with a testicular tumor or pelvic tumor are exposed to a dose below 1 Gray (Gy) due to scattered radiation despite the activation of these testicular protective detoxifying mechanisms (Bieri et al. Citation1999). Similarly, health care workers are exposed to occupational radiation and the effects of this low dose cannot be easily detected (Bush et al. Citation1985; McParland et al. Citation1990; Ramsdale et al. Citation1990). However, the affect offspring gender a sensitive marker of toxic exposure has not received as much attention.
In the present study, we aimed to perform a histopathological evaluation of damage caused by 1 Gy pelvic radiation in testicular germ cells and to investigate whether the number of spermatozoa with X and Y chromosomes following irradiation varies, i.e., the offspring sex ratio. To our knowledge, this is the first study that combines fluorescence-based capillary electrophoresis to evaluate X and Y chromosome content of semen samples and also caspase-3 and TUNEL assays to examine apoptosis in rat testicular tissue exposed to 1 Gy pelvic radiation.
Results and discussion
The primer pairs specific for TSPY (185 bp), RActβ (193 bp) and TSX (201 bp) genes on chromosomes Y, 12 and X, respectively, were designed, and these regions were amplified from sperm DNA and control DNA (whole blood sample from female rat) by PCR. The PCR products were analyzed by determining the relative proportions (TSPY/TSX) of peak heights for each sample on the results obtained by capillary electrophoresis (). The mean peak height in the sperm DNA samples was 0.98 ± 0.13 in the unirradiated rats and 0.90 ± 0.11 in the irradiated rats. However, no significant difference (p = 0.38) () was found between the groups in terms of the number of sperm cells having an X or Y chromosome, as measured in sperm cells by the relative fluorescence unit (RFU).
Figure 1. Quantitative fluorescence multiplex PCR results and the TSPY/TSX ratio. (A). Analyses of Y and X chromosome-specific gene regions of TSPY, RActβ and TSX genes in the rat sperm genome in unirradiated (n = 7) and irradiated (n = 7) groups in the presence of a female genomic DNA sample. PCR products amplified using individual primer pairs designed for TSPY, RActβ and TSX genes were separated by capillary electrophoresis to determine the estimated sizes. The PCR products were labeled with FAM. Peak heights were used to quantify the results. The x-axis shows amplicon sizes and the y-axis shows the relative fluorescence unit derived from the number of amplicons of the target region. It was confirmed by the DNA sample in the peripheral blood of the female rat that there was no amplification in the TSPY specific region. Representative electrophoretic data is shown for one rat from each group are shown. Four replicates were analysed for each rat. (B). Ratio of TSPY/TSX relative fluorescence unit in rat sperm samples. The ratio of TSPY/TSX relative fluorescence unit in rat sperm samples in the unirradiated and irradiated groups obtained after irradiation. The data in the bar graph shows the mean ± standard deviation values of four replicate assays conducted for each rat. Data were analyzed using the unpaired t-test. p = 0.38. FAM: fluorescein amidite, PCR: polymerase chain reaction, RActβ: rat actin beta, TSPY: testis-specific protein Y-linked, TSX: testis-specific X-linked.
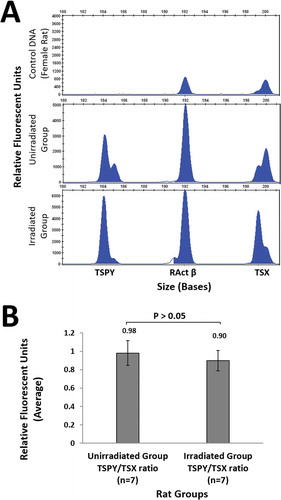
Female rats mated for two weeks with irradiated or control unirradiated rats were followed for another three weeks in order to determine the sex of the offspring. As a result, the sex of the offspring was determined two weeks after birth.
The sex of the offspring of each rat and the total number of offspring are shown in for each group. The number of offspring ranged from 0–13 in females mated with unirradiated males, and 1–16 in irradiated rats. The total number of offspring in unirradiated and irradiated groups were 70 (35 female and 35 male) and 74 (37 female and 37 female), respectively. In both groups, it was determined that the number of offspring was equal for both sexes.
Table 1. Sex and total number of offspring in both groups.
Light microscopic examination of the tissue sections obtained from the testicles of the unirradiated group showed regular seminiferous tubules with normal histological structural features (). In the testicular samples of the irradiated group, there were edematous regions which might be associated with the germinal epithelial cell death. In addition to the loss of germinal epithelial cells, spermatogonia and atypical spermatocytes with condensed chromatin was observed. However, by histopathology, mature spermatids in the lumen were not significantly different in the irradiated group, compared to the unirradiated group. Vascular congestion in the interstitial regions in the irradiated group was also prominent ().
Figure 2. Histopathological evaluation of seminiferous tubules. (A) (x200). Seminiferous tubules (St) demonstrated normal structure in sections from the unirradiated group. In addition, spermatogonia (Spg), primary spermatocytes (PS), spermatids (Spt), Sertoli cells (SC) and Leydig cell (LC) is typically visible in the seminiferous tubules (St). (B) (x200). Sections from the irradiated group show moderate damage were seen in the testis tissue. Vascular congestion (arrowhead) and edematous fields (*) can be seen in interstitial areas.
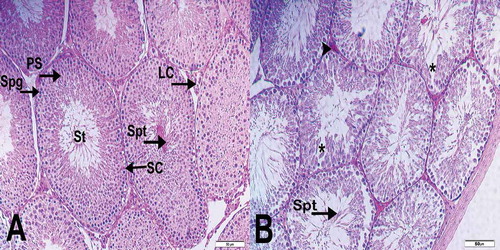
In the irradiated group, the number of TUNEL and Caspase-3-positive cells in the germinal epithelium of the seminiferous tubules, an apoptotic indicator, was significantly higher, compared to the unirradiated group (p = 0.001; p = 0.015; respectively) (). Representative images for the number of TUNEL and Caspase-3 positive cells in the controls and irradiated group are shown in and . The number of TUNEL- and Caspase-3-positive cells in the germinal epithelium of the seminiferous tubules was found to be statistically higher in the irradiated group exposed to 1 Gy pelvic radiation compared to the unirradiated. Areas of loss of germinal epithelial cells, spermatogonia and atypical spermatocytes with condensed chromatin were observed. However, the histopathology of the mature spermatids in the lumen were not found to be significantly different in both groups. This likely reflects the histology of the developmental stages of spermatogenesis. Spermatogenesis begins with the proliferation of the spermatogonium (stem cell), a germ cell located on the basement membrane of the seminiferous tubule, and ends with the formation of mature spermatozoa (sperm cell) in three stages. Spermatogenesis is a series of events that involves mitosis, meiosis and development of new cells. If we look at this process in detail, unlike the others, germinal spermatogonia develop into primary spermatocytes with mitosis. This is thought to be the most sensitive cell cycle phase to radiation i.e., mitosis (Bergonie and Tribondeau Citation1959). The damage occurs mainly in the germinal epithelium as a first response to low-dose radiation. Accordingly, apoptosis would begin by the increasing number of Caspase-3-positive cells. It is not surprising that this region was found to be more sensitive to 1 Gy radiation as described here. As previously reported in several studies, testicular germ cells are known to be the most sensitive cells to radiation (Dewey et al. Citation1995; Chen et al. Citation2000; Seong et al. Citation2001; Cordelli et al. Citation2003). Moreover, spermatogonial cells are reported to be relatively more radiosensitive than spermatocytes, spermatids and spermatozoa (Erickson Citation1976). Thus, the germinal epithelium might be the first affected area in radiation exposure, which may explain high caspase-3 activity in this region. In a recent study, Khan et al. (Khan et al. Citation2015) reported radiation-induced perturbation in both testicular cells and sperm at a sub-lethal dose of 5 Gy. Loss of germ cells after exposure to ionizing radiation has been associated with apoptosis (Sapp et al. Citation1992; Zhang et al. Citation2001). Recently, it has been suggested that gamma irradiation is associated with dose-dependent apoptosis in the impaired seminiferous tubule (Silva et al. Citation2016). Preclinical studies have also shown significant radiation-induced damage to testicular tissue and DNA at a sublethal dose of 2 Gy (Zelefsky et al. Citation2002; Coureuil et al. Citation2010; Khan et al. Citation2015; Fatehi et al. Citation2018). Similarly, Lee et al. reported that pelvic 2 Gy irradiation-induced TUNEL positivity was mostly confined to apoptotic spermatogonia and primary spermatocytes (Lee et al. Citation2015). Khan et al. reported that 2 Gy radiation induced an increase in the caspase-3 immunoreactivity in spermatogonia and spermatocytes (Khan et al. Citation2015). As stated above, certain stages of spermatogenesis are more susceptible to X-ray exposure, and apoptosis is induced more by DNA damage caused by ionizing radtiation in actively dividing spermatogonia (Chen et al. Citation2000; Cordelli et al. Citation2003). Khan et al. showed that radiation induced severe testicular atrophy through the depletion of spermatogonia, spermatocytes, and spermatid in the seminiferous tubules (Khan et al. Citation2015). In their study, 5 Gy whole-body irradiation was used as compared to 1 Gy pelvic region radiation. This might explain our result showing a significant apoptotic effect of radiation on germ cells but not in all cells of spermatogenesis.
Table 2. Caspase-3- and TUNEL-positive density.
Figure 3. Light microscopy image of Caspase-3 staining. (A) (x400). Unirradiated group sections show normal spermatogonium (spgn) and primary spermatocytes (ps). Seminiferous tubule (st). (B) (x400). Irradiated group sections show Caspase-3-positive spermatogonium (black arrow) and primary spermatocytes (blue arrow).
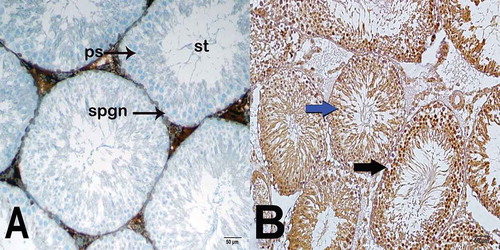
Figure 4. Light microscopy image of TUNEL staining. (A) (x200) – (B) (x400). Unirradiated group sections show normal spermatogonium (spgn) and primary spermatocytes (ps). Seminiferous tubule (st). (C) (x200) – (D) (x400). Irradiated group sections show apoptotic spermatogonium (black arrow) and primary spermatocytes (blue arrow).
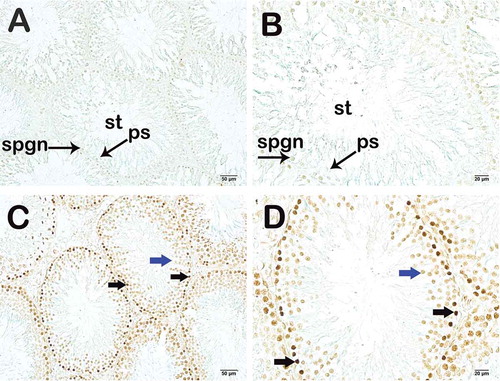
In general we are exposed to various doses of occupation dependent ionizing radiation (Bush et al. Citation1985). Low dose occupational radiation exposure cannot be easily detected. In the literature, a methodology based on caspase-3 measurement has been proposed for the determination of radiotherapy sensitivity of lymphocytes isolated from peripheral blood (SANTOS et al. Citation2017). However, in our study, the sensitivity to radiation was evaluated by measuring TUNEL and caspase-3 positivity in the germinal epithelium exposed to radiation. Our results suggest that this may provide an alternative method for measuring radiation-induced testicular tissue damage associated with therapy and other environmental exposures. The harmful effects of exposure to radiation are classified as somatic and hereditary. While somatic effects of radiation exposure induced gene mutations are not transmitted to the next generation, direct radiation of the reproductive organs may have more serious consequences of an hereditary effect transmitted to offspring (Russell Citation1976). The biological effects following radiation are classified as deterministic and stochastic dependent on dose and time of exposure. Deterministic effects are expected to occur only above a certain dose known as the threshold dose and the severity increases with dose. In stochastic effects, the probability of occurrence increases with dose. However, occurrence is considered to be ‘all or none’, and the effect itself does not worsen with dose (Son et al. Citation2011). Hereditary impact is also considered stochastic (Hall Citation1991). The ‘all or none’ principle, which has been an ongoing hypothesis since the 1940s, describes the occurrence of the harmful consequences of the radiation on inheritance, in a dose-independent manner (Delbruck Citation1940). It refers to the elimination of the harmful effects of radiation to prevent heritable genetic damage in tissues exposed to radiation. For example, if a woman is exposed to radiation within the first two weeks of pregnancy, ‘all or none’ principle is followed. This means that exposure to radiation can cause miscarriages or no problems. However, embryos in the earliest stages are most susceptible to very low doses of radiation. For example, an exposure of 0.1 Gy in the first two weeks of gestation may lead to the death of the embryo even before the clinical pregnancy is confirmed (mShaw et al. Citation2011).
The stochastic effects that lead to biological damage can be induced by low-dose radiation exposure. (McParland et al. Citation1990; Ramsdale et al. Citation1990). Spermatogenesis can be compromised at dose levels between 0.5 Gy to 5 Gy. Radiation doses exceeding 5 Gy lead to significant and possibly permanent injury (Demir et al. Citation2015). Despite the use of gonadal shielding, patients irradiated from infra diaphragmatic portals still receive almost 0.5 Gy scattered irradiation to the remaining testicle while the non-shielded doses were calculated between 1.7 to 5 Gy (Bieri et al. Citation1999). Radiation exposure affects fertility in young patients with a very long life expectancy. There are many studies that represent therapeutic options to compensate for the harmful effects of radiation on fertility (Meistrich et al. Citation2000; Shetty et al. Citation2000). These approaches include pre-treatment with spermatogenesis-suppressing hormones, stimulation of stem cell counts, and testosterone treatments (Meistrich Citation1998).
Testosterone, secreted by interstitial Sertoli and Leydig cells, has a maturing effect on spermatogenesis. Sertoli and Leydig cells were reported to show no histological changes at low doses of irradiation, because of their relatively radioresistant nature (Mettler Citation1985). In many studies, testosterone has been used to reverse deteriorating functions for therapeutic purposes, since the testosterone blood level decreases after irradiation (Zagars and Pollack Citation1997; Shetty et al. Citation2000; Nichols et al. Citation2017). However, testosterone treatment was not recommended in a study in rats after exposure to low-dose ionizing radiation since normal testicular functions was reported within two months after irradiation of groups independent of testosterone treatment (Demir et al. Citation2015). Sertoli and Leydig cell, are relatively radioresistant, the short duration after irradiation and the use of low-dose radiation are not expected to the disrupt hypothalamic-pituitary-gonadalaxis (HPG axis) and Gonadotropin-releasing hormone (GnRH)-Follicle-stimulating Hormone (FSH)/Luteinizing-Hormone (LH) (GnRH-FSH/LH) pathways excluding the necessity to assess blood testosterone GnRH, FSH and LH levels were not examined.
Many features of spermatogenesis are very similar in humans and laboratory animals, especially in mice and rats (Clermont and Harvey Citation1965; Meistrich and Brown Citation1983). The length of the spermatogenic cycle in rats is approximately 12.9 days (Clermont and Harvey Citation1965; Meistrich and Brown Citation1983). Accordingly, rats were sacrificed 14 days after irradiation to cover one cycle of spermatogenesis. Acute effects related to radiation in rats occur hours after the radiation exposure, and late effects occur after Week10.
The susceptibility of SRY (sex-determining region on the Y chromosome) gene to radiation induced damage or the selective damage on Y-chromosome-bearing spermatocytes may increase the percentage of female offspring (Joffe Citation2003). We, therefore, investigated the number of X and Y chromosomes in sperms subjected to radiation in the early period and evaluated the effects on the sex of the offspring. For this purpose, the rats were sacrificed before the end of the seminiferous epithelial cycle. In our study, X/Y chromosome ratio, which is normally 1:1 in semen samples, was not significantly different with the unirradiated and irradiated groups were compared. Although there is a slight preponderance of Y to X in the sperms of the irradiated group, the difference between the two groups was not found to be statistically significant. This is in agreement with previous studies which either found a little or no effect of ionizing radiation on the offspring sex ratio (Hawkins Citation1991; Byrne et al. Citation1998; Winther et al. Citation2003). It is well-known that damage to chromosomes due to radiation exposure induces apoptosis (Sakkas and El-Fakahany Citation2018). This is consistent with apoptotic elimination of low-dose radiation-affected cells in the germinal epithelium. This could also reflect the minimal chromosomal damage caused by low-dose radiation which can be controlled by programmed cell death and, thus, not transferred to the offspring. In this case only the surviving healthy cells could be developed into the cells of the spermatozoa. Previously it has been suggested that the division and differentiation of the surviving cells following exposure to radiation were responsible for the repopulation of the seminiferous epithelium (STEINBERGER et al. Citation1973).
There are several limitations to our study. First, caspase-8 and −9 as initiators of apoptotic signaling and activator for executioner caspases (e.g. caspase-3) were not evaluated. However, the levels of their downstream effector, caspase-3 was assessed to measure the degree of apoptosis. Since apoptosis is a complex molecular process, changes (such as mitochondrial calcium accumulation and alterations in membrane permeability) in those pathways could be evaluated by other methods. Second, testicular caspase-3 activity can be developed as a method for determining radiation exposure when additional dose response data becomes available. For example, studies below 1Gy (such as 10 mGy, 20 mGy, 50mGy) should be considered to establish a radiation threshold for workers exposed to ionizing radiation. Semen quality was not assessed in our study although a study reported no effect on semen quality at low doses of radiation (Demir et al. Citation2015). Data is available for doses above 1 Gy (Abuelhija et al. Citation2013; Ahmad and Agarwal Citation2017).
Pelvic radiation of 1 Gy induces significant testicular germinal epithelial cell apoptosis as a response to the associated cellular damage. Irradiated rats showed no alterations in the sex ratio of their offspring suggested that sex ratio assessment might be a poor indicator for the evaluation of the vulnerability of testicular germ cells in animal models for 1 Gy radiation exposure. Based on the ‘all or none’ principle the effect of the radiation in the offspring gender ratio might not be apparent due to the increased spermatogonial stem cell apoptosis and the elimination of the damaged cells. Of note, it should be kept in mind that potential side effects and genetic changes may occur in the long-term after radiation exposure and that the pathophysiology of early and late effects may be different. Therefore, large-scale and long-term studies are needed to understand these effects. Considering the susceptibility of the testicular germ cells to radiation, of caspase-3 activity may provide a method to monitor early effects of radiation exposure.
Materials and methods
Ethical consent was obtained from the local Ethics Committee for Animal Experiments (Decision No. 2014/21 and Date: 10.03.2014.) A total of 28 (14 females and 14 males) Sprague-Dawley rats weighing 275 ± 35 g and aged three to four months were used. All animals were maintained and fed in a sterile, 12 h light and 12 h dark conditions in the experimental animal unit environment at 55 to 60% humidity and 22ºC room temperature. Food and tap water were supplied ad libitum. The irradiated group comprised male rats which received 1 Gy radiation to the pelvis area and unirradiated female rats that were left unexposed to irradiation. The radiation doses used in our study were based on the doses used in previous radiation-induced infertility studies (Cordelli et al. Citation2003; Feinendegen Citation2005; Liu et al. Citation2006; Fatehi et al. Citation2018). The rats were irradiated by the isometric method with 6 MV from back and front using a bolus of 1 cm in an area of 20 cm x 40 cm, and a gantry 0 and 180 degrees. Photon irradiation (X-ray) was administered using a digital linear accelerator (Elekta Synergy; Elekta, Crawley, United Kingdom) at 6 MV at a dose rate of 4 Gy/min. The CMS-XIO radiotherapy treatment planning system (version 13.2) was used. Placing of irradiated and unirradiated male rats with females was done immediately after the irradiation process. Sperm samples were obtained after sacrification by externally pressurising the ductus defference area. To detect the early effects of radiation, testicular tissues from rats sacrificed at the end of the second week after irradiation, were assessedimmunohistochemically, histopathologically, and genetically. In addition, female rats were followed, until the sex of the offspring was determined.
DNA isolation
For DNA extraction, 60 µL of sperm sample was rinsed with 500 µL physiological buffer (75 mm NaCl, 25 mM EDTA) and centrifuged at 906 x g. After removal of the supernatant, the pellet was incubated at 56°C for 45 min in a shaking incubator with lysis buffer containing 250 µL of TE-9 buffer (500 mM Tris-HCl pH 9.0, 20 mM EDTA, 10 mM NaCl), 80 µL of 10% SDS and 60 µL of Proteinase K (20 mg/mL) was added. At the end of the incubation, 10 µL of beta-mercaptoethanol was added and vortexed, followed by a 10 min-incubation at room temperature. 150 µL of 6 M salt solution was added and proteins were precipitated by centrifugation for 10 min at 15,542 x g. This phase was repeated twice. The supernatant was transferred to a fresh tube, while the pellet was discarded. After the addition of 700 µL of 100% ethanol, it was centrifuged at 15,542 x g. The pellet was rinsed three times with 70% ethanol, which was subsequently allowed to air-dry. After 10 min of drying at room temperature, it was dissolved in 200 µL of sterile dH2O, which was left at 4°C overnight, until molecular analysis. Protein salting-out method applied to genomic DNA extraction from peripheral whole blood sample of a female rat (Miller et al. Citation1988).
Multiplex polymerase chain reaction (PCR) amplification
The total reaction volume was 25 µL per PCR reaction. All assays were performed in a 25 µl reaction volume containing 1µl of DNA template (75 ng for sperm DNA and 2 ng for female rat DNA), 2.5 µl of GeneAmp 10X PCR reaction buffer with MgCl2 (1.5 mM) (Applied Biosystems, Foster City, CA, USA), 0.5 µl of AmpliTaq Gold DNA Polymerase (Applied Biosystems, Foster City, CA, USA), 0.2 µl of dNTP mix (25 mM) and 0.5 µl of each primer (10 mM). Each primer set contains an oligonucleotide labeled with fluorescence at the 5ʹ end. Primer sets consist of the following sequences: forward 5ʹ-gCCTCTggTAATATgTggTCC-3ʹ and reverse 5ʹ-TgCTgATCTTgTTCACCTTCC-3ʹ for the testis-specific protein Y-linked (TSPY) gene, forward 5ʹ-gTgTTgTCCCTgTATgCC-3ʹ and reverse 5ʹ-gTggTggTgAAgCTgTAg-3ʹ for the rat actin beta (RActβ) gene, and forward 5ʹ-AggTACATTCTAgTTgCTAAggC −3ʹ and reverse 5ʹ-ACCATCCATAAggAAgAgTgTg −3ʹ for the testis-specific X-linked (TSX) gene. Amplifications were performed in ThermoCycler (Gene Amp PCR Systems 9700, Applied Biosystems Inc., Foster City, CA, USA) at 95°C for 4 min, and for 30 cycles at 95°C for 1 min, at 56°C for 1 min and at 72°C for 1 min, followed by a final extension step at 72°C for 5 min. The sizes of PCR amplicons are 185 bp, 193 bp and 201 bp for the TSPY, RActβ and TSX genes, respectively.
Capillary electrophoresis and relative peak analysis
The ABI Prism 310 genetic analyzer (Applied Biosystems Inc., Foster City, CA, USA) was used for capillary electrophoresis. 1.5 µL of amplicon, 20 µL of formamide (Hi-Di Formamide, Applied Biosystems Inc., Foster City, CA, USA), 0.5 µL of internal size standard, and GeneScan-500 LIZ size standard (Applied Biosystems, Warrington, UK) were mixed and rapidly cooled after incubation for 2 min at 96°C. It was injected for 5 sec using 36 cm capillaries containing POP-4 polymer (Applied Biosystems Inc., Foster City, CA, USA). Electrophoresis was performed at 15 kV, 60°C for 32 min. The Gene Scan Analyzer buffer containing EDTA was used as the running buffer. Data were expressed in peaks and analyzed by the ABI Gene Scan analysis software (Applied Biosystems Inc., Foster City, CA, USA).
Histopathological analysis procedure
Testicular sections of rats were fixed for 72 h using the Bouin fixator (Sigma Aldrich, St. Louis, MO, USA). Tissue blocks were embedded in paraffin using routine procedures (Merck, Darmstadt, Germany). 3–4 μm sections were obtained with a microtome (Leica, RM2125RT, Germany), which were stained with hematoxylin (Harris hematoxylin, Merck, Germany) and Eosin (H&E) (Eosin G, Merck, Germany). Stained sections were examined by light microscopy (Olympus BX51, Olympus Corporation, Tokyo, Japan) and photographed using Olympus DP71 (Olympus Corporation, Tokyo, Japan).
Immunohistochemistry (IHC) analysis procedure
To determine apoptotic cells in testicular sections, antibodies to caspase-3 (Abcam Rabbit polyclonal to active Caspase-3, UK) and TUNEL Assay Kit – HRP-DAB (ab206386, Abcam, USA) were utilized. The sections mounted on positively charged slides (Patolab, China) were deparaffinized. Endogenous peroxidase was blocked with 3% H2O2 for 15 mins. Sections were blocked for non-specific binding for 20 mins before the application of the primary antibody (anti-Caspase-3 at 1/50 dilution) for 60mins. After incubation with the secondary antibody (Goat Anti-Rabbit IgG H&L (HRP) (ab205718, Abcam, United Kingdom) chromogen (diaminobenzidine chromogen, Abcam, United Kingdom) was added. After counter-staining for hematoxylin, the sections were sealed. TUNEL assay was performed according to the instructions of the manufacturer. Finally, after counter-staining for Methyl Green the sections were sealed.
Stereological analysis
For stereological examination, the Stereo Investigator (version 9, Micro brightfield, USA) was used. For each section, 60 different measurement areas were sampled systematically and randomly using the fractionator probe. The caspase-3 numerical density and positive cells were counted at x40 magnification and the sections were examined as previously described (Hausdorf et al. Citation2008; Mercantepe et al. Citation2016).
Statistical analysis
Statistical analysis was performed using the Statistical Package for the Social Sciences (SPSS) version 18.0 (SPSS Inc., Chicago, IL, USA). The descriptive data were expressed in mean ± standard deviation (SD). The unpaired t-test was used to analyze the significance of the difference of means between the groups. A p-value of ≤ 0.05 was considered statistically significant.
Authors' contributions
Conceived and supported the study: SYR, HSN, LT; Designed and drafted the manuscript: SYR, HSN; Performed the experiments: SYR, HSN, AIG, LT; Analyzed the data: SYR, HSN, LT; Collected samples: SYR, HSN, LT; Provided analysis methods: SYR, HSN, TM.
Acknowledgments
We thank the physicists and technicians working at the Radiotherapy Department of the Teaching and Research Hospital of Rize, Recep Tayyip Erdogan University, Faculty of Medicine.
Disclosure statement
No potential conflict of interest was reported by the authors.
Additional information
Funding
References
- Abuelhija M, Weng CC, Shetty G, Meistrich ML. 2013. Rat models of post‐irradiation recovery of spermatogenesis: interstrain differences. Andrology. 1(2):206–215.
- Ahmad G, Agarwal A. 2017. Ionizing radiation and male fertility. Male infertility. New Delhi, India: Springer; p. 185–196.
- Bergonie J, Tribondeau L. 1959. Interpretation of some results of radiotherapy and an attempt at determining a logical technique of treatment/De Quelques Résultats de la Radiotherapie et Essai de Fixation d’une Technique Rationnelle. Radiat Res. 11(4):587–588.
- Bieri S, Rouzaud M, Miralbell R. 1999. Seminoma of the testis: is scrotal shielding necessary when radiotherapy is limited to the para-aortic nodes? Radiother Oncol. 50(3):349–353. eng.
- Bush WH, Jones D, Brannen GE. 1985. Radiation dose to personnel during percutaneous renal calculus removal. Am J Roentgenology. 145(6):1261–1264.
- Byrne J, Rasmussen SA, Steinhorn SC, Connelly RR, Myers MH, Lynch CF, Flannery J, Austin DF, Holmes FF, Holmes GE, et al. 1998. Genetic disease in offspring of long-term survivors of childhood and adolescent cancer. Am J Hum Genet. 62(1):45–52. eng
- Cai L, Jiang J, Wang B, Yao H, Wang X. 1993. Induction of an adaptive response to dominant lethality and to chromosome damage of mouse germ cells by low dose radiation. Mutat Res. 303(4):157–161. eng.
- Cai L, Liu SZ. 1990. Induction of cytogenetic adaptive response of somatic and germ cells in vivo and in vitro by low-dose X-irradiation. Int J Radiat Biol. 58(1):187–194. eng.
- Cai L, Wang P. 1995. Induction of a cytogenetic adaptive response in germ cells of irradiated mice with very low-dose rate of chronic gamma-irradiation and its biological influence on radiation-induced DNA or chromosomal damage and cell killing in their male offspring. Mutagenesis. 10(2):95–100. eng.
- Chen SL, Cai L, Meng QY, Xu S, Wan H, Liu SZ. 2000. Low-dose whole-body irradiation (LD-WBI) changes protein expression of mouse thymocytes: effect of a LD-WBI-enhanced protein RIP10 on cell proliferation and spontaneous or radiation-induced thymocyte apoptosis. Toxicol Sci. 55(1):97–106. eng.
- Clermont Y, Harvey S. 1965. Duration of the cycle of the seminiferous epithelium of normal, hypophysectomized and hypophysectomized-hormone treated albino rats. Endocrinology. 76(1):80–89.
- Cordelli E, Fresegna AM, Leter G, Eleuteri P, Spano M, Villani P. 2003. Evaluation of DNA damage in different stages of mouse spermatogenesis after testicular X irradiation. Radiat Res. 160(4):443–451. eng.
- Coureuil M, Ugolin N, Tavernier M, Chevillard S, Barroca V, Fouchet P, Allemand I. 2010. Puma and Trail/Dr5 pathways control radiation-induced apoptosis in distinct populations of testicular progenitors. PLoS One. 5(8):e12134. eng.
- Da Silva Mansano N, Jorge IF, Chies AB, Viani GA, Spadella MA. 2018. Effects of telmisartan and losartan on irradiated testes. Life Sci. 194:157–167. eng.
- Delbruck M. 1940. Radiation and the hereditary mechanism. Am Nat. 74(753):350–362.
- Demir A, Tanidir Y, Karadag MA, Atasoy BM, Bozkurt S, Cecen K, Türkeri L. 2015. Effects of testosterone treatment on recovery of rat spermatogenesis after irradiation. J Pak Med Assoc. 65(3):300–305.
- Dewey WC, Ling CC, Meyn RE. 1995. Radiation-induced apoptosis: relevance to radiotherapy. Int J Radiat Oncol Biol Phys. 33(4):781–796. eng.
- Elmore S. 2007. Apoptosis: a review of programmed cell death. Toxicol Pathol. 35(4):495–516. eng. <emory_human_genetics_environmental_exposures_during_pregnancy.pdf>
- Erickson BH. 1976. Effect of 60Co gamma radiation on the stem and differentiating spermatogonia of the postpuberal rat. Radiat Res. 68(3):433–448. eng.
- Fang F, Gong PS, Zhao HG, Bi YJ, Zhao G, Gong SL, Wang ZC. 2013. Mitochondrial modulation of apoptosis induced by low-dose radiation in mouse testicular cells. Biomed Environ Sci. 26(10):820–830. eng.
- Fatehi D, Mohammadi M, Shekarchi B, Shabani A, Seify M, Rostamzadeh A. 2018. Radioprotective effects of Silymarin on the sperm parameters of NMRI mice irradiated with gamma-rays. J Photochem Photobiol B. 178:489–495. eng.
- Feinendegen LE. 2005. Evidence for beneficial low level radiation effects and radiation hormesis. Br J Radiol. 78(925):3–7. eng.
- Hall E. 1991. Scientific view of low-level radiation risks. Radiographics. 11(3):509–518.
- Hasegawa M, Wilson G, Russell LD, Meistrich ML. 1997. Radiation-induced cell death in the mouse testis: relationship to apoptosis. Radiat Res. 147(4):457–467. eng.
- Hasegawa M, Zhang Y, Niibe H, Terry NH, Meistrich ML. 1998. Resistance of differentiating spermatogonia to radiation-induced apoptosis and loss in p53-deficient mice. Radiat Res. 149(3):263–270. eng.
- Hausdorf J, Lemmens MA, Kaplan S, Marangoz C, Milz S, Odaci E, Korr H, Schmitz C, Maier M. 2008. Extracorporeal shockwave application to the distal femur of rabbits diminishes the number of neurons immunoreactive for substance P in dorsal root ganglia L5. Brain Res. 1207:96–101. eng.
- Hawkins MM. 1991. Is there evidence of a therapy-related increase in germ cell mutation among childhood cancer survivors? J Natl Cancer Inst. 83(22):1643–1650. eng.
- Joffe M. 2003. Infertility and environmental pollutants. Br Med Bull. 68:47–70. eng.
- Khan S, Adhikari JS, Rizvi MA, Chaudhury NK. 2015. Radioprotective potential of melatonin against ⁶⁰Co γ-ray-induced testicular injury in male C57BL/6 mice. J Biomed Sci. 22(1):61. eng.
- Lee W, Son Y, Jang H, Bae MJ, Kim J, Kang D, Kim JS. 2015. Protective effect of administered rolipram against radiation-induced testicular injury in mice. World J Mens Health. 33(1):20–29. eng.
- Liu G, Gong P, Bernstein LR, Bi Y, Gong S, Cai L. 2007. Apoptotic cell death induced by low-dose radiation in male germ cells: hormesis and adaptation. Crit Rev Toxicol. 37(7):587–605. eng.
- Liu G, Gong P, Zhao H, Wang Z, Gong S, Cai L. 2006. Effect of low-level radiation on the death of male germ cells. Radiat Res. 165(4):379–389. eng.
- McParland BJ, Nosil J, Burry B. 1990. A survey of the radiation exposures received by the staff at two cardiac catheterization laboratories. Br J Radiol. 63(755):885–888.
- Meistrich ML. 1998. Hormonal stimulation of the recovery of spermatogenesis following chemo‐or radiotherapy. Apmis. 106(1‐6):37–46.
- Meistrich ML. 2013. Effects of chemotherapy and radiotherapy on spermatogenesis in humans. Fertil Steril. 100(5):1180–1186. eng.
- Meistrich ML, Brown CC. 1983. Estimation of the increased risk of human infertility from alterations in semen characteristics. Fertil Steril. 40(2):220–230. eng.
- Meistrich ML, Wilson G, Kangasniemi M, Huhtaniemi I. 2000. Mechanism of protection of rat spermatogenesis by hormonal pretreatment: stimulation of spermatogonial differentiation after irradiation. J Androl. 21(3):464–469. eng.
- Mercantepe T, Unal D, Selli J, Mercantepe F, Unal B, Karabiyik TN. 2016. Protective effects of estrogen and bortezomib in kidney tissue of post-menopausal rats: an ultrastructural study. Ren Fail. 38(7):1129–1135. eng.
- Mettler FA. 1985. Medical effects of ionizing radiation. New York, NY: Grune and Stratton.
- Miller SA, Dykes DD, Polesky HF. 1988. A simple salting out procedure for extracting DNA from human nucleated cells. Nucleic Acids Res. 16(3):1215. eng.
- Nichols RC, Hu C, Bahary JP, Zeitzer KL, Souhami L, Leibenhaut MH, Rotman M, Gore EM, Balogh AG, McGowan D, et al. 2017. Serum testosterone changes in patients treated with radiation therapy alone for prostate cancer on NRG oncology RTOG 9408. Adv Radiation Oncol. 2(4):608–614. eng
- Ramsdale ML, Walker WJ, Horton PW. 1990. Extremity doses during interventional radiology. Clin Radiol. 41(1):34–36. eng.
- Russell W. 1976. Comments on mutagenesis risk estimation. Tenn.(USA): Oak Ridge National Lab.
- Sakkas D, El-Fakahany HM. 2018. Apoptosis in ejaculated spermatozoa and in the normal and pathological testes: abortive apoptosis and sperm chromatin damage. A clinician’s guide to sperm DNA and chromatin damage. Cham: Springer; p. 197–218.
- SANTOS NFD, Silva RF, Pinto MM, SILVA EB, Tasat DR, Amaral A. 2017. Active caspase-3 expression levels as bioindicator of individual radiosensitivity. Anais Da Academia Brasileira De Ciências. 89(1):649–659.
- Sapp WJ, Philpott DE, Williams CS, Williams JW, Kato K, Miquel JM, Serova L. 1992. Comparative study of spermatogonial survival after x-ray exposure, high LET (HZE) irradiation or spaceflight. Adv Space Res. 12(2–3):179–189. eng.
- Seong J, Kim SH, Pyo HR, Chung EJ, Suh CO. 2001. Effect of low-dose irradiation on induction of an apoptotic adaptive response in the murine system. Radiat Environ Biophys. 40(4):335–339. eng.
- Shaw P, Duncan A, Vouyouka A, Ozsvath K. 2011. Radiation exposure and pregnancy. J Vasc Surg. 53(1):28S–34S.
- Shetty G, Wilson G, Huhtaniemi I, Shuttlesworth GA, Reissmann T, Meistrich ML. 2000. Gonadotropin-releasing hormone analogs stimulate and testosterone inhibits the recovery of spermatogenesis in irradiated rats. Endocrinology. 141(5):1735–1745.
- Silva AM, Correia S, Casalta-Lopes JE, Mamede AC, Cavaco JE, Botelho MF, Socorro S, Maia CJ. 2016. The protective effect of regucalcin against radiation-induced damage in testicular cells. Life Sci. 164:31–41. eng.
- Son BK, Lee KT, Kim JS, Lee SO. 2011. Lack of radiation protection for endoscopists performing endoscopic retrograde cholangiopancreatography. Korean Journal Gastroenterol. 58(2):93–99.
- STEINBERGER E, ROOT A, FICHER M, SMITH KD. 1973. The role of androgens in the initiation of spermatogenesis in man. J Clin Endocrinol Metab. 37(5):746–751.
- Terrell ML, Hartnett KP, Marcus M. 2011. Can environmental or occupational hazards alter the sex ratio at birth? A systematic review. Emerg Health Threats J. 4:7109. eng.
- Thornberry NA, Lazebnik Y. 1998. Caspases: enemies within. Science. 281(5381):1312–1316. eng.
- Winther JF, Boice JD Jr., Thomsen BL, Schull WJ, Stovall M, Olsen JH. 2003. Sex ratio among offspring of childhood cancer survivors treated with radiotherapy. Br J Cancer. 88(3):382–387. eng.
- Zagars GK, Pollack A. 1997. Serum testosterone levels after external beam radiation for clinically localized prostate cancer. Int J Radiat Oncol Biol Phys. 39(1):85–89. eng.
- Zeiss CJ. 2003. The apoptosis-necrosis continuum: insights from genetically altered mice. Vet Pathol. 40(5):481–495. eng.
- Zelefsky MJ, Fuks Z, Hunt M, Yamada Y, Marion C, Ling CC, Amols H, Venkatraman ES, Leibel SA. 2002. High-dose intensity modulated radiation therapy for prostate cancer: early toxicity and biochemical outcome in 772 patients. Int J Radiat Oncol Biol Phys. 53(5):1111–1116. eng.
- Zhang X, Yamamoto N, Soramoto S, Takenaka I. 2001. Cisplatin-induced germ cell apoptosis in mouse testes. Arch Androl. 46(1):43–49. eng.