ABSTRACT
The present study was conducted to analyze the mRNA expression of the BMP/SMAD signaling and steroidogenesis associated genes in the granulosa cells (GCs) of newly developed Booroola homozygous carrier GMM (FecBBB) and non-carrier GMM (FecB++) ewes through qRT-PCR. Results showed that the expression of BMP2 (P < 0.05) and BMP6 (P < 0.01) was significantly higher in the GCs of the homozygous carrier GMM (FecBBB) than the non-carrier GMM (FecB++) ewes, while the expression of BMP4 was significantly higher (P < 0.001) in the GCs of non-carrier GMM (FecB++) than the homozygous carrier GMM (FecBBB). In comparison, the expression of TGFβR1, BMPR1A, BMPR1B, and BMPRII was not significantly different between GCs of the homozygous carrier GMM (FecBBB) and non-carrier GMM (FecB++) ewes. Similarly, the expression of SMAD1, SMAD2, SMAD3, SMAD4, and SMAD5 was not significantly different between GCs of homozygous carrier GMM (FecBBB) and non-carrier GMM (FecB++). Further, expression of the INHIBIN, P4R, CYP11A1, and 3βHSD1 genes were not significantly different among the GCs of homozygous carrier GMM (FecBBB) and non-carrier GMM (FecB++), while the expression of StAR was significantly higher (P < 0.01) in the GCs of homozygous carrier GMM (FecBBB) than that of GCs of non-carrier GMM (FecB++) ewes. It is concluded that the FecB mutation significantly up-regulates the expression of BMP2, BMP6, and StAR genes and down-regulate the expression of BMP2 in granulosa cells of newly developed GMM ewes.
Abbreviations
BMP: Bone morphogenetic protein; TGFβ: Transforming growth factor-beta; SMAD: Fusion of Caenorhabditis elegans Sma genes and the Drosophila Mad, Mothers against decapentaplegic; GCs: Granulosa cells; GMM: Garole x Malpura x Malpura; FecB: Booroola fecundity; BMPR: Bone morphogenetic protein receptor; CYP11A1: Cytochrome P450 family 11 subfamily A member 1; StAR (Steroidogenic acute regulatory protein); 3βHSD1: 3 Beta-hydroxysteroid dehydrogenase; IGF: Insulin-like growth factor; ActR2: Activin receptor 2; ACVR1: Activin A receptor, type I; ACVR2: Activin A receptor, type II; ACVRL1: Activin A receptor like type 1; ACVR1B: Activin A receptor type 1B; ACVR1C: Activin A receptor type 1C; RFLP-PCR: Restriction fragment length polymorphism-polymerase chain reaction; qRT-PCR: Quantitative real-time PCR; ANOVA: Analysis of variance; P4R: Progesterone receptor: AMH: Anti mullerian hormone; RT-PCR: Reverse transcriptase-polymerase chain reaction; ER: Estrogen receptor; FSHR: Follicle stimulating hormone receptor.
Introduction
The reproductive efficiency of Indian sheep breeds is poor because they exist in adverse climatic conditions and hence they encountered insufficient feed, fodder, and nutrients. Reproductive efficiency of sheep is directly associated with the fecundity of an individual. The fecundity of an individual is governed by ovarian folliculogenesis, which is highly regulated process manifested by a precise proliferation and differentiation of the somatic cells (granulosa cells and theca cells) and the germ cells (oocytes). This process depends on the complex action and interactions between the locally produce hormones and the growth factors including the insulin/IGF, inhibin/activin, and bone morphogenetic proteins (BMPs) systems (Fabre et al. Citation2006).
About 15 members (BMP1–BMP15) of the BMP family have been identified in mammalian species (Wang et al. Citation2014). These BMP members belong to the transforming growth factor-β (TGFβ) superfamily. Several members of the BMP family (BMP2, BMP4, BMP6, BMP7, and BMP15) act as an autocrine/paracrine regulators of the ovarian follicular development and ovulation through BMP/SMAD signaling pathway (Galloway et al. Citation2000; Juengel et al. Citation2006). Hence, BMP molecules have a multitude of cellular functions including osteogenesis, cell differentiation, anterior/posterior axis specification, growth, and development (Wang et al. Citation2014).
There are about eight members of the SMAD family identified in mammals (Sekelsky et al. Citation1995). Based on their function, the SMADs are classified as receptor-activated SMADs (SMAD1, -2, -3, -5, and -8), common-partner SMAD (SMAD4), and inhibitory SMADs (SMAD6 and SMAD7). SMAD2 and -3 are activated by TGFβ, growth and differentiation factors (GDFs) and activin molecules, whereas SMAD1, -5 and -8 are activated by BMP ligands. SMAD6 and -7 are the inhibitory SMADs that interfere with SMAD-receptor and SMAD-SMAD interactions (Massagué Citation2000; Kaivo-oja et al. Citation2006). They function as intracellular transcription factors that mediate the signaling of TGFβ superfamily which is comprised of more than 40 members, including TGFβs, activins, inhibins, GDFs, BMPs, glial-derived neurotrophic factors (GDNFs), nodal, lefty, and anti-Müllerian hormones (AMH) (Kaivo-oja et al. Citation2006; Wang et al. Citation2014; Yadin et al. Citation2016).
It is well known that the BMP factors (ligands) act through the two sub-types of receptors with serine/threonine kinase activity. Seven type I receptors and five type II serine/threonine kinase receptors have been reported (Attisano and Wrana Citation1996). Activin receptor-like kinase 1 (Alk1 or ACVRL1), Alk2 (ACVR1), Alk3 (BMPR1A), Alk4 (ACVR1B), Alk5 (TGFβR1), Alk6 (BMPR1B), and Alk7 (ACVR1C) act as type 1 receptors, whereas BMP receptor 2 (BMPRII), Activin receptor 2 (ActR2), Activin receptor 2A/Activin receptor 2B (ActR2A/2B), and TGFβ receptor 2 (TGFβRII) are the type 2 receptors (Kaivo-oja et al. Citation2006; Loomans and Andl Citation2016). Out of seven type 1 receptors, three receptors bind and interact with BMP ligands; BMPR1A, BMPR1B, and ActR1A (Horbelt et al. Citation2012). Out of five type 2 receptors, three receptors are known to interact with BMP ligands; BMPRII, ActR2A, and ActR2B (Wang et al. Citation2014). These BMP factors interact with hetero-tetrameric complexes of type 1 and type 2 receptors. Type 2 receptor phosphorylates the type 1 receptor. Once phosphorylated, the type 1 receptor phosphorylates one of the receptor-regulated intracellular signaling SMAD proteins (either SMAD1, -5 and -8). Hence, the phosphorylated BMP receptor-regulated SMADs hetero-dimerizes with common SMAD4 and translocate into the nucleus, where these hetero-complexes act as a transcriptional activator to regulate the expression of the target genes (Massagué Citation1998, Citation2000; Souza et al. Citation2004). Interaction of the BMP, TGFβ, and GDF ligands to their respective receptors have been mentioned in -.
Figure 1. Interaction of the BMP, TGFβ and GDF ligands to their respective receptors. (A) BMPs signaling via the SMAD protein dependent pathway in granulosa cells. BMP ligands initiate the signal transduction cascade by binding to type 1 receptor (BMPR1A/BMPR1B) and type II receptor (BMPRII) serine/threonine kinase and forming a heterotetrameric complex. The constitutively active type II receptor then transphosphorylates the type I receptor, and the type I receptor phosphorylates the Receptor-SMADs (either SMAD1/5/8). Phosphorylated SMAD1/5/8 associates with the co-SMAD (SMAD4), and the complex translocates into the nucleus where it further associates with co-activators or co-repressor to regulate the gene expression. (B) TGFβ signaling via the SMAD protein dependent pathway in granulosa cells. TGFβ1 ligand binding initiate the signal transduction cascade by binding to type 2 receptor (TGFβRII) and type 1 receptor (TGFβR1) and forming a heterotetrameric complex. The active type II receptor then transphosphorylates the type I receptor, and the type I receptor phosphorylates the receptor-SMADs (either SMAD2/3). Phosphorylated SMAD2/3 associates with the co-SMAD (SMAD4), and the complex translocates into the nucleus for regulate the expression of the target gene. (C) Growth and differentiation factor (GDF) signaling via the SMAD protein dependent pathway in granulosa cells. GDF9 ligand binding initiate the signal transduction cascade by binding to type 2 receptor (BMPRII) and type 1 receptor (TGFβR1) and forming a heterotetrameric complex. The active type II receptor then transphosphorylates the type I receptor, and the type I receptor phosphorylates the receptor-SMADs (either SMAD2/3). Phosphorylated SMAD2/3 associates with the co-SMAD (SMAD4), and the complex translocates into the nucleus where it bind to the regulatory sequences and regulate the expression of the target gene.
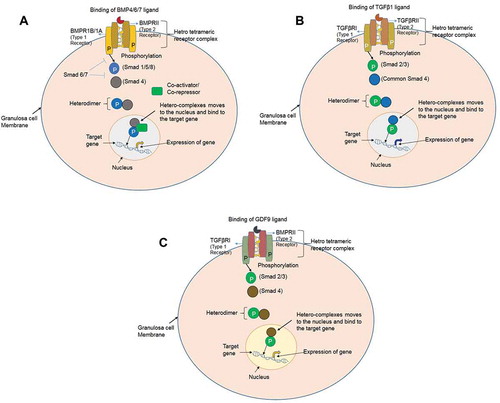
It is well known that the type 1 receptor (TGFβRI) and the type 2 receptor (BMPRII) are the downstream of the GDF9 ligand on the cell surface (Vitt et al. Citation2002; Mazerbourg et al. Citation2004). GDF9 ligand binds to BMPRII and interacts with downstream TGFβRI (Gilchrist et al. Citation2008). After binding of GDF9 to BMPRII and TGFβRI, this ligand-receptor activation allows the downstream phosphorylation and activation of the SMAD proteins (Castro et al. Citation2015). Thus, GDF9 specifically activates the SMAD2 and SMAD3 signaling which in turn forms a complex with common SMAD4. This complex translocates into the nucleus and regulates the expression of the target gene (Gilchrist et al. Citation2008). Thus, GDF9 is essential for early follicular development and ovulation through its direct action on the granulosa cells allowing their proliferation and differentiation (Otsuka et al. Citation2011). In comparison, the TGFβ ligand binds to the extracellular domain of the TGFβRI, which in turn activates the receptor and allows it to bind with other receptors (TGFβRII) on the cell surface. Hence, these three proteins form a complex and trigger signal transduction by activating the signaling molecules (SMAD2 and SMAD3) through the TGFβ signaling pathway (Source: Genetic Home Reference: https://ghr.nlm.nih.gov/gene/TGFβR1; https://omim.org/entry/190181; Franzen et al. Citation1993). The role of TGFβ1 has been implicated in ovarian folliculogenesis, steroidogenesis, and proliferation of the granulosa cells (Rosairo et al. Citation2008). In summary, the molecules of BMP/SMAD pathway, GDF9, and TGFβ1 interact with each other and play an important role in the regulation of ovulation rate and folliculogenesis in prolific ewes.
It is well known that the high prolificacy of Australian Booroola Merino sheep is associated with Booroola fecundity (FecB) gene. The high prolificacy of Booroola Merino is due to the effect of FecB mutation (p.Q249R; nucleotide change from A to G at 746 bp) in a highly conserved intracellular serine/threonine kinase-signaling domain of BMPR1B, which is expressed in the granulosa cells and the ovary (Mulsant et al. Citation2001; Souza et al. Citation2001; Wilson et al. Citation2001). The FecB mutation has been identified in several sheep breeds viz. Kendrapada, Bonpala, Nilagiri and Chhotanagpuri sheep from India (Kumar et al. Citation2008; Roy et al. Citation2011; Sudhakar et al. Citation2014; Oraon et al. Citation2016); Javanese from Indonesia (Davis et al. Citation2002); Hu, Small tail Han and Bayanbulak sheep from China (Davis et al. Citation2006; Chu et al. Citation2007; Zuo et al. Citation2013); and Kalehkoohi sheep from Iran (Mahdavi et al. Citation2014). High prolificacy of Indian Garole sheep is due to the effect of FecB mutation (Davis et al. Citation2002; Kumar et al. Citation2006), although, FecG mutation was identified in Garole sheep (Polley et al. Citation2010), but the Galway mutation (FecXG) was absent (Kumar et al. Citation2008aa).
The most prominent physiological effects of the FecB mutation are on the number of ovulatory follicles, ovulation rate, and the litter size. The ovulatory follicles mature and ovulate at significantly smaller diameters and have fewer GCs in the homozygous FecB carrier ewes than the non-carrier ewes (Driancourt et al. Citation1985; McNatty et al. Citation1985, Citation1986; Souza et al. Citation1994). Further, Booroola ewes homozygous (FecBBB) for a mutation in the BMPR1B gene exhibit a higher ovulation rate, have larger diameter oocytes at earlier stages of follicular development (i.e., Type 3) and small follicles at ovulation than the wild type sheep (FecB++) (Reader et al. Citation2012). How the FecB mutation regulates the number of follicles in Booroola homozygous ewes? Whether FecB mutation acts at the level of an ovary or pituitary. It is still elusive.
It is known that BMPR1B is likely to be partially inactivated due to FecB mutation which leads to an advanced differentiation of GCs and maturation of the ovulatory follicles. Therefore, FecB mutation probably affects the folliculogenesis through an impairment of the BMPR1B receptor activity (Mulsant et al. Citation2001. It was also advocated that the FecB mutation acts at the level of the ovary and modulates gonadotrophin responsiveness (Campbell et al. Citation2003, Citation2006). Subsequently, Feary et al. (Citation2007) argued that the mutated BMPR1B receptor is likely to be less responsive to BMP ligands, and thus FecB mutation decreases the signaling ability of the BMPR1B receptor. Although, the exact genetic mechanism of high-prolificacy of sheep is still unknown (Tang et al. Citation2019). It is also unclear how FecB mutation affects the BMPR1B receptor activity and causes alteration in the BMP/SMAD signaling, hormone secretion, and regulation of folliculogenesis?
The effect of FecB mutation on ovulation rate, litter size, body weight, ewe’s productivity efficiency, and semen production characteristics have been analyzed in Indian Garole and GM sheep (Kumar et al. Citation2006, Citation2007, Citation2008, Citation2012; Maurya et al. Citation2008). However, the information on the expression of the BMP/TGFβ/SMAD signaling molecules, hormone, hormone receptor, and steroidogenic-associated genes in GCs of newly developed prolific GMM ewes is lacking. Therefore, the aims of the present study were undertaken to analyze the mRNA expression profile of BMP/SMAD signaling pathway and other ovarian associated genes in GCs of the homozygous carrier (FecBBB) and non-carrier (FecB++) GMM ewes with respect to the Malpura ewe as a base breed.
Results
The aim of the present study was conducted to analyze the mRNA expression profile of the BMP/TGFβ/SMAD signaling molecules, hormone, hormone receptor, steroidogenic and other ovarian associated genes in GCs of the homozygous carrier (FecBBB), non-carrier GMM (FecB++) and Malpura (FecB++) ewes.
FecB genotyping and oestrus synchronization
Twenty-eight ewes (19 GMM and 9 Malpura) were genotyped for the presence of FecB mutation using forced-RFLP-PCR technique. Nine and 10 GMM ewes were found as the homozygous carrier (FecBBB) and non-carrier (FecB++), respectively. All Malpura ewes (as a base breed) were found as non-carrier for the FecB mutation. Oestrus of all the ewes was synchronized by progesterone sponges. The results showed that the proportion of ewes that exhibited oestrus did not differ significantly between FecB genotypes (data not shown). Most of the ewes exhibited oestrus (83.3-100%) within 48 h after sponge removal; however, an oestrus response was observed early in homozygous carrier GMM (50.0%) as compared to non-carrier GMM ewes (28.6%).
Expression of BMP/SMAD signaling and other genes in cultured GCs through RT-PCR
It is well known that the BMP factors and receptors are mainly expressed in the GCs and the oocytes of mammalian species. The GCs from GMM and Malpura ewes were cultured and the morphological features of GCs have appeared as epithelial-like cells (). In the present study, BMP/GDF factors (BMP2, BMP4, BMP6, and GDF9), BMP receptors (BMPR1A, BMPR1B, and BMPRII), TGFβR1, and intracellular transducers (SMAD1, SMAD2, SMAD3, SMAD4, and SMAD5) were expressed in GCs of GMM (FecBBB and FecB++) and Malpura (FecB++) ewes (). Further, hormone and hormone receptor (INHIBIN and P4R) and steroidogenic associated genes (StAR, CYP11A1, and 3βHSD1) was also expressed in the GCs of homozygous carrier GMM (FecBBB), non-carrier GMM (FecB++), and non-carrier Malpura (FecB++) ewes. Results showed that all genes were expressed in cultured GCs of the GMM and Malpura ewes.
Figure 2. Aspiration and culture of the granulosa cells from antral follicle of sheep. Antral follicles (~3.5mm) were punctured by 26G needle (Becton Dickenson) and the follicular fluid was aspirated for culturing of the sheep granulosa cells. The morphological features of the granulosa cells of non-carrier Malpura (FecB++) (X), homozygous carrier GMM (FecBBB) (Y), and non-carrier GMM (FecB++) (Z) shown in the culture of McCoy’s 5A medium. All granulosa cells were healthy and appeared like an epithelial cells.

Figure 3. Expression of the BMP/SMAD family of genes in sheep granulosa cells by reverse transcription-polymerase chain reaction (RT-PCR). Representative agarose gel photograph showing the amplification of BMP factors/receptors and SMAD signaling genes. M: 50bp DNA ladder (Fermentas), Lane 1: RPL19, Lane 2: SMAD1, Lane 3: SMAD 3, Lane 4: SMAD 4, Lane 5: SMAD 5, Lane 6: SMAD 8, Lane 7: ACVR2, Lane 8: CYP11A1, Lane 9: AMH, Lane 10: BMPR1B, Lane 11: BMP15.
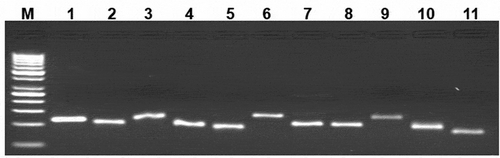
Quantitative expression of BMP/SMAD signaling and other genes in cultured GCs through qRT-PCR
Relative abundances of BMP/GDF factors (BMP2, BMP4, BMP6, and GDF9), BMP receptors (BMPR1A, BMPR1B, and BMPRII), TGFβR1, intracellular transducers (SMAD1, SMAD2, SMAD3, SMAD4, and SMAD5), hormone and hormone receptor (INHIBIN and P4R), and steroidogenic associated genes (StAR, CYP11A1, and 3βHSD1) were analyzed in GCs of homozygous carrier GMM (FecBBB), non-carrier GMM (FecB++), and non-carrier Malpura (FecB++) through qRT-PCR. Results showed that the expression of BMP2 (P < 0.05) and BMP6 (P < 0.01) was significantly higher in GCs of homozygous carrier GMM (FecBBB) than the non-carrier GMM ewes (FecB++), while the expression of BMP4 was significantly higher (P < 0.001) in the GCs of non-carrier GMM (FecB++) than the homozygous carrier GMM ewes (FecBBB) (). The expression of GDF9 was slightly increased in GCs of non-carrier GMM (FecB++) as compared to homozygous carrier GMM ewes (FecBBB), but the values were non-significant.
Figure 4. Quantitative expression of the BMP factors/receptors in the granulosa cells of different FecB genotypic groups of ewes. Relative abundance (RFU, relative fold unit) of the BMP factors and BMP receptors shown in granulosa cells of homozygous carrier GMM (FecBBB), non-carrier GMM (FecB++), and non-carrier Malpura (FecB++) ewes with reference to RPL19 as housekeeping gene (internal control). Pairwise significant difference between means of different FecB groups was considered from P value lower than 0.05 to 0.001. Straight lines between two values with asterisks above them depict the significant difference as *P < 0.05, **P < 0.01, ***P < 0.001.
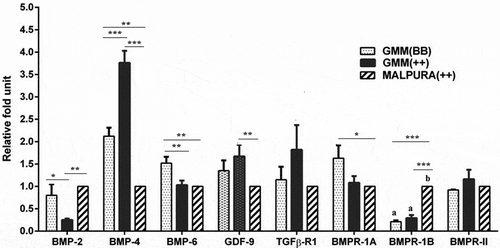
In the present study, the expression of BMP/TGFβ receptor was analyzed in the GCs of both FecB genotypic groups of GMM ewes. It was noted that the expression of TGFβR1, BMPR1B, and BMPRII was little increased (P > 0.05) in the GCs of non-carrier GMM (FecB++) as compared to the homozygous carrier GMM ewes (FecBBB), while the expression of BMPR1A was slightly increased (P > 0.05) in GCs of homozygous carrier GMM (FecBBB) than that of non-carrier GMM ewes (FecB++). It was found that the expression of BMP/TGFβ receptor molecule was not significantly different between the GCs of homozygous and non-carrier GMM ewes ().
Expression of the intracellular transducer was studied in GCs of the homozygous carrier and non-carrier GMM ewes. The expression of SMAD1 and SMAD5 was slightly higher (P > 0.05) in GCs of homozygous carrier GMM than that of non-carrier GMM ewes (). In comparison, the expression of SMAD2 (P > 0.05), SMAD3 (P > 0.05), and SMAD4 (P > 0.05) was slightly advanced in the GCs of non-carrier GMM (FecB++) than the homozygous carrier GMM (FecBBB). It was noted that the expression of the intracellular transducers was not significantly different between the GCs of both FecB genotypic groups of GMM ewes ().
Figure 5. Quantitative expression of the SMAD signaling genes in the granulosa cells of different FecB genotypic groups of ewes. The relative abundance (RFU, relative fold unit) of the SMAD signaling genes shown in the granulosa cells of homozygous carrier GMM (FecBBB), non-carrier GMM (FecB++) and non-carrier Malpura (FecB++) ewes with reference to RPL19 as housekeeping gene (internal control). Pairwise significant difference between means of FecB groups was considered from P value lower than 0.05 to 0.001. No significant difference between means of gene expression data (RFU) of different FecB groups was recorded.
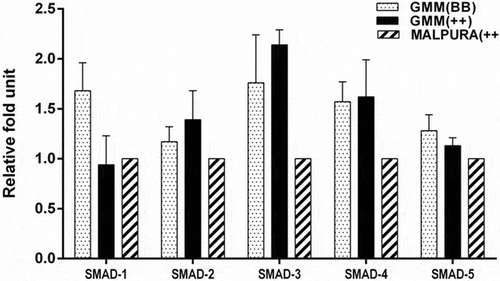
Further, the expression of INHIBIN and P4R was analyzed in GCs of both FecB groups of GMM ewes. The expression of INHIBIN (P > 0.05) and P4R (P > 0.05) was slightly increased in the GCs of homozygous carrier GMM (FecBBB) than the non-carrier GMM (FecB++) ewes, but the values were not significantly different among the groups (). Similarly, the expression of CYP11A1 and 3βHSD1 was slightly increased in the GCs of homozygous carrier GMM, but the values did not differ significantly (P > 0.05) between both FecB genotypes of GMM ewes. Interestingly, the expression of StAR was significantly higher (P < 0.01) in the GCs of homozygous carrier GMM (FecBBB) as compared to that of non-carrier GMM ewes (FecB++). It was also noted that the expression of FSHR, estrogen receptor (ER), and anti Müllerian hormone (AMH) genes in the GCs of GMM and Malpura ewes were poorly detected on the agarose gel (therefore the data was not studied).
Figure 6. Quantitative expression of the hormone, hormone receptor and steroidogenic associated genes in the granulosa cells of different FecB genotypic groups of ewes. Relative abundance of the hormone, hormone receptor and steroidogenic associated genes in GCs of homozygous carrier GMM (FecBBB), non-carrier GMM (FecB++) and non-carrier Malpura (FecB++) ewes with reference to RPL19 as housekeeping gene (internal control). Pairwise significant difference between means was considered from P value lower than 0.05 to 0.001. Straight lines between two values with asterisks above them depict the significant difference as *P < 0.05, **P < 0.01, ***P < 0.001.
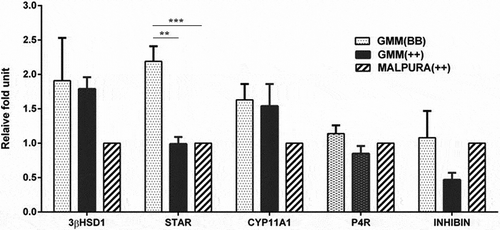
This, study on the in vitro culture suggested that the FecB mutation slightly attenuate the activities of type I and/or type II receptors (TGFβR1, BMPR1B, and BMPRII) in GCs of the homozygous carrier GMM ewes (FecBBB). This supports the view that the FecB mutation significantly enhances the expression of certain BMP ligand molecules (BMP2 and BMP6) and steroidogenic associated gene (StAR) in the GCs of homozygous GMM ewes.
Discussion
The effects of Booroola mutation on the body weight, litter size, ovulation rate, and other production traits have been well documented in prolific Garole and GM sheep (Kolte et al. Citation2005; Kumar et al. Citation2006, Citation2007, Citation2008). However, very limited information is available on the expression of BMP/TGFβRI/SMAD signaling molecules, hormone, hormone receptor, and steroidogenic associated genes in newly developed prolific GMM ewes (Bahire et al. Citation2019). Therefore, the present study was conducted to analyze the expression of the BMP/SMAD and other ovarian associated genes in the GCs of newly developed FecB homozygous carrier and non-carrier of GMM ewes.
It is well known that the members of the TGFβ superfamily play an important role in the proliferation and differentiation of the somatic cells (GCs and theca cells) and germ cell (oocyte) and also provide intercellular communication between the GCs and the oocytes. Several BMP factors play an important role in the ovarian follicular development of mammalian species. Some BMP factors (viz. BMP2) induce bone and cartilage formation in mammals (Chen et al. Citation2004). While BMP4 regulates the formation of teeth, limbs, and bone from mesoderm, it also plays an important role in fracture repair, epidermis formation, dorsal-ventral axis formation, and ovarian follicle development. Further, BMP4, in conjunction with BMP7, also regulate an early ovarian follicle development and primordial-to-primary follicle transition (Nilsson and Skinner Citation2003). In a similar fashion, BMP6 plays a role in homeostatis, bone and cartilage formation, but also enhanced the growth of secondary follicles in goat (Frota et al. Citation2013).
In the present study, the expression of BMP/GDF factors, BMP receptors, intracellular transducers, hormone, hormone receptor, and steroidogenic-associated genes was found in the GCs of GMM (FecBBB and FecB++) and Malpura (FecB++) ewes. It is well known that the GCs secrete several steroidogenic enzymes, signaling factors, and steroid hormones during steroidogenesis. Hence, it is concluded that mRNA transcripts for all the genes under this study were expressed in the GCs of GMM and Malpura ewes.
Effects of FecB mutation on the expression of BMP/GDF factors and receptors
The BMPR1B, BMP15, and GDF9 are the hyper prolificacy-associated genes that affect the BMP signaling in sheep (Drouilhet et al. Citation2013). BMPR1B is expressed in the oocytes and granulosa cells from the primary stage to the late stage of the antral follicle. A previous in vitro culture study suggested that the BMP4 and GDF5 ligands bind to the BMPR1B/BMPRII receptors and initiate the BMP signaling pathway in GCs of sheep (Mulsant et al. Citation2001), but mutation in the BMPR1B gene has a strong inhibitory effect on the binding of GDF5 and BMP4 ligands to the BMPR1B and BMPRII receptors; and hence BMP pathway seems to be disrupted in Booroola homozygous ewes. It has been also suggested that the BMPR1B protein is likely to be partially inactivated due to the single point mutation (FecB) in the intercellular kinase signaling domain of BMPR1B (Q249R); which leads to an alteration in the BMP pathway and consequently improved the ovulation rate and litter size of Booroola homozygous carrier ewes (Mulsant et al. Citation2001; Fabre et al. Citation2006).
In the present study, expression of the BMP/SMAD signaling and other ovarian associated genes was analyzed in GCs of the homozygous GMM, non-carrier GMM, and non-carrier Malpura ewes (–). The mRNA expression profile of the BMP2 (P < 0.05) and BMP6 (P < 0.01) was significantly higher in GCs of homozygous carrier GMM as compared to the non-carrier GMM ewes, while the expression of BMP4 (P < 0.001) was higher in the GCs of non-carrier GMM than that of homozygous carrier GMM ewes. In the contrary, the expression of BMP2 and BMP6 was not found to be significantly different in the antral follicles of high and low fecundity Hu sheep (Xu et al. Citation2010). Similarly, the results are in contrast with the findings of previous study that the expression of BMP4 was more abundant in the antral follicle of high fecundity than that of low fecundity Hu sheep (Xu et al. Citation2010). In a similar way, BMP4 expression was higher in the ovaries of homozygous carrier Garole than the non-carrier Decaani ewes (Goyal et al. Citation2017).
It is well known that the GDF9 is an oocyte-derived growth factor, which is an essential molecule for ovarian follicular development and normal ovulation and/or corpus luteum formation in sheep (Juengel et al. Citation2002). GDF9 acts as a ligand molecule for the BMPRII and TGFβRI receptors of BMP/TGFβ signaling pathway (Vitt et al. Citation2002; Mazerbourg et al. Citation2004) (). In the present study, the expression of the GDF9 gene showed no-significant (P > 0.05) difference between non-carrier GMM and homozygous carrier GMM ewes. In contrary, GDF9 expression was higher in the antral follicle and the ovary of high fecundity sheep than in that of low fecundity sheep (Xu et al. Citation2010; Tang et al. Citation2019). In a similar way, Crawford et al. (Citation2011) also found higher expression of the GDF9 in the GCs of homozygous ewes as compared to wild-type ewes. Further, Goyal et al. (Citation2017) showed the variable expression of GDF9 in the Graffian follicles of carrier Garole and non-carrier Deccani ewes, while the values were significantly higher in the ovaries of non-carrier Deccani (FecB++) than that of carrier Garole ewes (FecBBB).
Figure 7. Schematic representation of the BMP factors/receptors, SMAD signaling molecules, and steroidogenesis associated genes in granulosa cells. Binding and interactions of the ligand molecules viz. BMP2/4/6/7, GDF9 and TGFβ1 to their respective receptors molecules BMPRII/TGFβRII. Phosphorylation of the receptor regulated SMAD proteins (either SMAD1/-2/-3/-5/-8) and their association with common SMAD4. Entry of the hetero-complexes into nucleus and acting as transcription activators for expression of the target genes of a particular pathway. Secretion of the progesterone and inhibin after activation through their receptors. Depiction of the expression of the steroidogenesis associated genes in the granulosa cells.
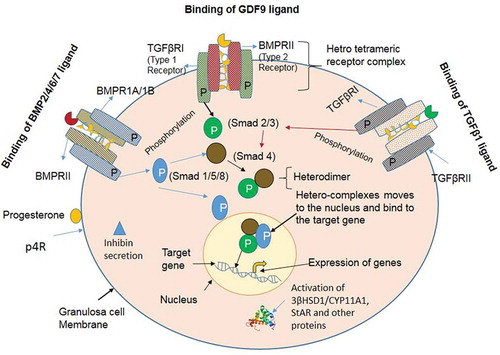
On one hand, it is well known that sheep homozygous for a GDF9 gene mutation are anovulatory, sterile and have a streak ovary, whereas heterozygous sheep have higher ovulation rates than the wild-type sheep (Hanrahan et al. Citation2004). Mutation in the GDF9 gene increases the ovulation rate in heterozygous individuals, but causes infertility in homozygous individuals (Nicol et al. Citation2009). On the other hand, Vage et al. (Citation2013) showed the polymorphism (c.1111G>A) in the GDF9 gene of Norwegian white sheep, increases the ovulation rate in homozygous ewes. Thus, it is concluded that the protein composition and the precise expression of the GDF9 are very crucial for normal ovulation and folliculogenesis in sheep.
Hence, the BMP/GDF factors may play an important role in the process of normal ovarian folliculogenesis; however, the variable expression of BMP factors in GMM ewes may be due to the effect of tissue type, follicle size, breed, age, background genotype, and other environmental factors. Further, in vitro culture condition may also alter the gene expression profile and the results cannot exactly mimic in vivo conditions. Therefore, the previous results of the in vitro study suggested that the FecB mutation may alter the interactions of the signaling molecules of BMP pathway as well as the secretion of the gonadotrophin hormone, which could not be exactly replicated as in vivo conditions (Fabre et al. Citation2003; Young et al. Citation2008).
Effects of FecB mutation on the expression of BMP/TGFβ receptors
It is well established that a single point mutation in the BMPRIB receptor (A746G) is associated with the hyper-prolificacy of the Booroola Merino and the Garole ewes (Mulsant et al. Citation2001; Souza et al. Citation2001; Wilson et al. Citation2001; Kumar et al. Citation2006). It has been found that BMPR1B mutation causes partial inactivation of the protein, therefore, BMPRIB might not have sufficient receptor activity for binding of its ligand molecules for proper BMP-signaling pathway (Mulsant et al. Citation2001). In the present study, the expression of BMPR1B and BMPRII were not-significantly higher (P > 0.05) in the GCs of non-carrier GMM than that of homozygous carrier GMM ewes. In other studies, no significant difference was reported in the expression of BMPR1B gene among three FecB genotypes (FecBBB, FecBB+, FecB++) of small tailed Han sheep (Tang et al. Citation2019). While in contrast, other workers have found the expression of BMPRIB and BMPRII higher in antral follicles of the high fecundity sheep than that of low fecundity sheep (Xu et al. Citation2010). Similarly, Regan et al. (Citation2015) also demonstrated the up-regulation of the BMPR1B transcripts in GCs of homozygous Booroola ewes. Similar observations were also reported in the antral follicles of the homozygous Garole ewes (Goyal et al. Citation2017). It is inferred from the above discussion that the FecB mutation in BMPR1B may cause alteration in the binding site of its ligand molecules; therefore it might not be sufficiently potentiated to interact with BMPRII protein in close proximity; hence BMPRII is possibly not enough to phosphorylate the BMPR1B. Therefore, BMP signaling cascade would be disrupted in homozygous carrier ewes; henceforth it may cause higher ovulation rate in homozygous carrier ewes by an unknown mechanism.
In the present study, expression of the TGFβRI was not-significantly increased (P > 0.05) in the GCs of non-carrier GMM as compared to carrier GMM ewes. While the study of Xu et al. (Citation2010) suggested that the expression of TGFβRI was higher in the antral follicles of high fecundity than that of low fecundity ewes. Similarly, TGFβRI expression was also higher in the GCs and antral follicle of the homozygous Booroola ewes, respectively (Regan et al. Citation2015; Goyal et al. Citation2017). In the present study, higher expression of the TGFβRI in non-carrier ewes may be directly correlated with the slightly up-regulation of the SMAD2 and SMAD3 expression, which is downstream of the TGFβ pathway.
Expression of the BMPR1A transcript was slightly higher (P > 0.05) in the GCs of the homozygous GMM as compared to non-carrier GMM ewes. Similar results were also reported by Goyal et al. (Citation2017) when BMPR1A expression was higher in the ovaries of highly prolific Garole than that of non-prolific Deccani ewes, but the expression of BMPR1A was not-significant in the Graffian follicles of both the genotypes of GMM ewes. Although in support, the expression of BMPR1A did not differ significantly in the antral follicles of high and low fecundity Hu ewes (Xu et al. Citation2010). Henceforth, it may be suggested that after partial inactivation of the BMPR1B receptor due to FecB mutation, the BMPR1A (type 1 receptor) might be activated and could maintain the BMP signaling cascade in the GCs of homozygous ewes. This may be reconciled as both type 1 and type 2 receptors play a cooperative role in initiating the proper BMP signaling pathway in granulosa cells. Due to the change in amino acid residues of BMPR1B and the variable expressions of the BMP/TGFβ receptor molecules, the BMP signaling cascade might be partially altered by an unknown mechanism. Hence, it may be possible that the change in the amino acid composition and the expression profile of certain BMP receptors could influence the ovulation rate and folliculogenesis in prolific ewes.
Effects of FecB mutation on the expression of SMAD signaling molecules
In the present study, expression of the SMAD1, SMAD2, SMAD3, SMAD4, and SMAD5 genes were studied in GCs of the GMM and Malpura ewes. The expression of SMAD1 and SMAD5 was slightly enhanced (P > 0.05) in the GCs of homozygous GMM ewes, but the expression of SMAD2, SMAD3, and SMAD4 did not-significantly increase (P > 0.05) in non-carrier GMM ewes (). It is well known that the SMADs are an intracellular transducer of the BMP signaling pathway, which is crucial for normal reproduction of sheep and other mammals. These intracellular signaling molecules play an important role in initiating the BMP signaling pathway in the sheep ovary. It was fascinating to note that the expression of SMAD2, SMAD3, and SMAD4 was slightly lower in the GCs of homozygous GMM ewes than that of non-carrier GMM ewes. It may be due to the effect of FecB mutation that this mutation may alter the BMPR1B protein receptor activity and the receptor may not have enough activity to phosphorylate any receptor-regulated SMAD protein and subsequently interacts with common SMAD4. Conclusively, BMP/SMAD signaling pathway might be disrupted in homozygous GMM ewes.
Xu et al. (Citation2010) found higher expression of SMAD4 in antral follicles of high fertility sheep than that of low fertility ewes, but the expression of SMAD1 and SMAD5 was not significantly different among high and low fertility Hu ewes. This might be attributed to the fact that SMAD4 is a common factor, which is an essential transducer for both TGFβ/activin and BMP signaling pathways. It has been found that the SMAD1 and SMAD5 knockout mice have an embryonic lethality (Chang et al. Citation1999; Tremblay et al. Citation2001), hence these intracellular transducers have a pivotal role in the normal reproduction of an animal. Present results suggested that the expression of the SMAD genes in GCs could not be affected by the presence of FecB mutation except slight variation in the gene expression. These variations may be attributed due to breed type, age of animals, cell culture conditions, and other unknown factors.
Effect of FecB mutation on the expression of inhibin, hormone receptor, and steroidogenic associated genes
Inhibin is a glycoprotein hormone which plays an important role in the negative feedback regulation of the FSH. The role of progesterone receptor is to activate the steroid hormone progesterone, therefore, it acted as a positive regulator of the progesterone secretion. Steroidogenesis associated genes such as StAR are indispensable for the synthesis of steroids. It is primarily present in steroid-producing cells, including theca cells and luteal cells in the ovary, Leydig cells in the testis and in the adrenal cortex. The other steroidogenesis associated gene is P450scc, which is a member of the cytochrome P450 superfamily of enzymes (family 11, subfamily A, polypeptide 1). This enzyme is encoded by the Cyp11A1 gene. The role of the P450scc enzyme is to catalyze the conversion of cholesterol to pregnenolone. This is the first reaction in the process of steroidogenesis that specializes in the production of various steroid hormones (Hanukoglu Citation1992). 3βHSD1 enzyme catalyzes the biosynthesis of the steroid progesterone from pregnenolone, 17α-hydroxyprogesterone from 17α-hydroxypregnenolone, and androstenedione from dehydroepiandrosterone (DHEA) in the adrenal gland. It has an essential role in the biosynthesis of all classes of hormonal steroids, namely progesterone, glucocorticoids, mineralocorticoids, androgens, and estrogens (Lachance et al. Citation1992).
The effect of FecB mutation on the gonadotrophin hormone secretion has been well studied in Booroola Merino and FecB introgressed progenies (Xia et al. Citation2003; Campbell et al. Citation2003, Citation2006). It has been reported that plasma FSH concentration was higher in New Zealand Booroola homozygous ewes than that of non-carrier ewes (McNatty et al. Citation1991, Citation1993). It has been suggested that the FecB mutation probably inhibits the progesterone secretion from the granulosa cells and increases the FSH concentration in FecB carrier ewes (Mulsant et al. Citation2001). In vitro culture indicates that the primary pituitary cell cultures of Booroola ewes secreted less FSH than the wild-type ewes in presence of BMP2, BMP4, and BMP6 (Young et al. Citation2008). It may be possible that the FecB mutation may alter the interaction of the BMP molecules with other factors regulating FSH secretion in vivo, which is not exactly the same as in vitro conditions (Young et al. Citation2008).
In the present study, expression of the INHIBIN, P4R, StAR, CYP11A1, and 3βHSD1 was slightly increased in the GCs of homozygous than the non-carrier GMM ewes. The results are in accordance with the in vitro study of Campbell et al. (Citation2003) that the estradiol and inhibin A secretion was four- to fivefold greater (P < 0.001) from the granulosa cells of homozygous (FecBBB) than the non-carrier (FecB++) ewes. Similar observations were also recorded by Gonzalez-Bulnes et al. (Citation2004) that the estradiol and inhibin A secretion was lower in older non-carrier ewes during the early luteal phase (P = 0.08 and P < 0.05, respectively), but, the secretion of inhibin A was inversely related to the secretion of FSH in aged sheep of both the genotypes (P < 0.0001). The present study suggested that the homozygous GMM ewes have more INHIBIN expression as compared to non-carrier GMM ewes, which may be directly correlated with the high secretion of inhibin in homozygous carrier ewes.
A study of Souza et al. (Citation1997) found no differences in the concentration of FSH, progesterone, inhibin A, estradiol, and androstenedione secretion during the follicular or luteal phase between FecB carrier and non-carrier ewes. It has been documented that the FecB mutation impaired the GCs response to the stimulatory action of the BMP4 on antimullerian hormone (AMH) production, indicating a crucial role of BMPR1B on AMH regulation in prolific Booroola ewes (Estienne et al. Citation2015). It is concluded from the above discussion that the FecB mutation did not have any prominent effect on the hormone secretion in the prolific GMM ewes.
The expression of StAR was significantly higher (P < 0.01) in GCs of the homozygous than the non-carrier GMM ewes, but the expression of CYP11A1 (P > 0.01) and 3βHSD1 (P > 0.01) was not significantly different among both genotypes of GMM ewes (). Although, none of reports on the expression of steroidogenic associated genes in GCs with respect to the FecB gene are available, a discussion on the expression of steroidogenic genes is impossible. Hence, an up-regulation of the StAR in the GCs of homozygous GMM ewes is an interesting feature as it has not been reported in any prolific ewes. It is suggested that the up-regulation of the StAR gene in the GCs of homozygous ewes by unknown mechanism may provide insight into the regulation of follicle development and ovulation in the homozygous carrier ewes.
In summary the present study, mRNA expression of the BMP/TGFβ/SMAD signaling, hormone, hormone receptor, and steroidogenic associated genes was analyzed in the GCs of homozygous carrier GMM, non-carrier GMM, and non-carrier Malpura ewes. The FecB mutation significantly increased the expression of BMP2 (P < 0.05) and BMP6 (P < 0.01), but reduced the expression of BMP4 in the GCs of homozygous carrier GMM (FecBBB) ewes. Whereas, the expression of GDF9 did not differ significantly between both FecB groups of the GMM ewes. Expression of the BMP/TGFβ receptor molecules (BMPR1A, BMPR1B, BMPRII, and TGFBR1), SMAD signaling molecules (SMAD1, SMAD2, SMAD3, SMAD4, and SMAD5), hormone and hormone receptor (INHIBIN and P4R), and steroidogenic associated genes (CYP11A1 and 3βHSD1) was not significantly different between the homozygous carrier GMM (FecBBB) and non-carrier GMM (FecB++) ewes. Interestingly, StAR expression was found significantly higher (P < 0.01) in homozygous carrier GMM (FecBBB) than the non-carrier GMM (FecB++) ewes. It is suggested that the expression of BMP factors/receptors is not consistent between the FecB carrier and non-carrier ewes. Therefore, the variable expression of BMP factors/receptors and other ovarian-associated genes may be due to the effect of tissue type, follicle type, breed, genetic background, cell culture conditions, and other unknown environmental factors. It is concluded that BMP factors or receptors/SMAD signaling molecules play a critical role in the regulation of the ovulation rate in prolific sheep by an unknown mechanism.
Materials and methods
Location of the study and animal management
The study was conducted at ICAR-Central Sheep and Wool Research Institute (CSWRI), Avikanagar, Rajasthan. The climate of this location is typically hot and semi-arid with yearly minimum and maximum temperature ranges from 4°C to 46°C, respectively. The FecB introgression program was implemented at CSWRI, Avikanagar with the aim to introduce the FecB gene from prolific Garole sheep into non-prolific Malpura sheep and produced the F1 Garole x Malpura (GM) as half-bred sheep. Inter se mating was performed to generate the homozygous GM individuals. Further, GM rams (FecB homozygous carrier) was then backcrossed to Malpura ewes to produce the GM x Malpura (GMM: 25% Garole and 75% Malpura inheritance). A newly developed prolific GMM sheep is a carrier of FecB allele and most of the individuals are capable to produce the multiple births (ICAR-CSWRI Annual Report 2016–17). Majority of GMM individuals are homozygous and heterozygous carriers (FecBBB and FecBB+) for the FecB mutation. Several GMM sheep were transferred to Sheep Breeding Farm (Sector 9) of Animal Physiology and Bio-Chemistry Division, ICAR-CSWRI Avikanagar. Finally, 28 ewes were selected based on their body weight, age, litter records and litter sizes with respect to their reproductive performance. Reproductive performances of the ewes used in the experiments were normal and free from any debility and diseases. All animals were grazed daily for 8-10 h on native Cenchrus ciliaris pastures interspersed with natural grasses, shrubs and, forbs. Animals were housed in chain-link fence enclosure having asbestos sheet roof open from all the sides.
FecB genotyping using forced RFLP-PCR
Approximately 2 mL of venous blood was collected from GMM ewes in anticoagulant-coated vacutainer tubes (Becton Dickinson). DNA was extracted from blood using the QIAamp DNA Blood Mini Kit (QIAGEN). FecB genotyping of GMM ewes was carried out by forced RFLP-PCR technique as described earlier (Wilson et al. Citation2001; Kumar et al. Citation2006).
Oestrus synchronization
A total of 28 GMM and Malpura ewes of 3–6 years of age were selected for oestrus synchronization. Nine homozygous carrier GMM (FecBBB), 10 non-carrier GMM (FecB++) and 9 non-carrier Malpura (FecB++) ewes were synchronized with progesterone impregnated sponges (Avikesil-S@350mg per ewe) as per the protocol followed at Avikanagar (De et al. Citation2015). Progesterone sponges were inserted into the vagina for 12 days in a major breeding season (July-August). Sponges were removed on day 12 and oestrus response of each ewe was recorded. To culture the granulosa cells, ovariectomy was performed to retrieve the single ovary from each synchronized animal during the follicular phase of the oestrus cycle. All procedures were conducted as per the guidelines of the Institute Animal Ethics Committee.
Isolation and culture of GCs
The GCs were isolated from antral follicles of the ovary as per the method described by Campbell et al. (Citation1996) with minor modifications. Antral follicles (~3.5mm) were punctured by 26G needle (Becton Dickenson) and the follicular fluid was aspirated gently and transferred into 1.5 ml Eppendorf tube containing McCoy’s 5A media (Sigma). Filtered media was added to the above fluid to make the volume of 1ml and the tube was stood for 10 min at 37°C to settle down the theca cells. The supernatant was gently aspirated in a new 1.5 mL Eppendorf tube and the content was briefly centrifuged at 300 g for 7 min. The pellet of GCs was then suspended and cultured in 500 µL McCoy’s 5A media containing NaHCO3 2.2g/L and HEPES 4.76g/L supplemented with 2mM L-glutamine, 0.1% BSA, 2 μg/mL insulin, 1 μg/mL FSH, 20 ng/mL IGF-I, 0.02 IU/mL LH, 0.8 μg/mL selenium with 5% (v/v) FBS (all purchased from Sigma) in 4-well plate. After 6–7 days of primary culture, GCs were trypsinized by 0.25% Trypsin-EDTA (Sigma) and sub-cultured in 24-well plate. The GCs were cultured in McCoy’s 5A media at 37°C in 5% CO2 for further study.
RNA isolation and preparation of cDNA from GCs
The GCs were trypsinized and counted by hemocytometer. The viability of GCs was checked through a 0.4% trypan blue method. About 1.0 × 105 GCs from homozygous carrier GMM (FecBBB), non-carrier GMM (FecB++), and non-carrier Malpura (FecB++) ewes were taken for RNA isolation. Total RNA was isolated using an RNAqueous Micro kit (Ambion, Austin, TX, USA). RNA was treated with DNaseI at 37°C for 20 min and the enzyme was inactivated by DNase Inactivation Reagent (Ambion). The cDNA was synthesized using RevertAid first-strand cDNA synthesis kit as per the manufacturer’s guidelines. The final concentration of RNA was adjusted to 100 ng/per sample for cDNA synthesis. The quality of cDNA was ascertained through PCR using GAPDH (glyceraldehyde 3-phosphate dehydrogenase) as a house-keeping gene.
Designing and standardization of the primers
Primers were designed using internet-based Primer3 software (Untergasser et al. Citation2012) and the properties of each primer were checked through ‘PCR Primer Stats’ using Sequence Manipulation Suite (www.bioinformatics.org/sms2). The sequences of the primers and probable PCR product size are given in . Initially, all primers were standardized on cDNA of sheep ovary.
Table 1. List of the primer sequences and their expected product size (base pair).
Quantitative expression of BMP/TGFβ/smad signaling molecules and other ovarian associated genes in GCs
Expression of the BMP/GDF factors (BMP2, BMP4, BMP6, and GDF9), BMP receptors (BMPR1A, BMPR1B, and BMPRII), TGFβ receptor (TGFβR1), intracellular transducers (SMAD1, SMAD2, SMAD3, SMAD4, and SMAD5), hormone and hormone receptor (INHIBIN and P4R (progesterone receptor), and steroidogenic pathway-associated genes i.e., StAR (steroidogenic acute regulatory protein, CYP11A1 (cytochrome P450 family 11 subfamily A member 1) and 3βHSD1 (3 beta-hydroxysteroid dehydrogenase) was studied in GCs of GMM and Malpura ewes through reverse transcription-polymerase chain reaction (RT-PCR). The PCR reaction components for RT-PCR were as follows: 1X PCR buffer with NH4 (SO4)2, 1.5 mM MgCl2, 200 µM dNTPs mix, 200 nM each primer, 1.66U Taq DNA polymerase (Fermentas, USA), and 2 µL cDNA. PCR amplification conditions for all the genes were as follows: initial denaturation at 95°C for 3 min, followed by 35 cycles of denaturation at 95°C for 25 s, annealing 56-60°C for 25 s, extension at 72°C for 35 s and a final extension at 72°C for 5 min.
Finally, all the genes were analyzed in GCs of homozygous carrier GMM (FecBBB) (n = 2), non-carrier GMM (FecB++) (n = 2) and non-carrier Malpura (FecB++) (n = 2) through quantitative real-time PCR (qRT-PCR). qRT-PCR was performed on CFX96TM Real-Time System (Bio-Rad) using SYBR green (double-stranded DNA specific fluorescent dye) i.e., Maxima SYBR green/ROX qPCR Master Mix (2X) (Thermo Scientific). The reaction mixture was prepared in triplicates for the target and the housekeeping RPL19 (60S ribosomal protein L19) genes. RPL19 was used as a housekeeping gene because its expression was higher than that of GAPDH in sheep ovary. Each reaction mixture contained 2.0 µL c-DNA (i.e., 200ng per reaction). 0.2 µL of each forward and reverse primer (10 µM), 5 µL of 2X SYBR green master mixture (containing dNTPs, Taq DNA Polymerase, MgCl2, and buffer) and 2.8 µL Milli Q water to make the final volume of 10 µL. Amplification reaction conditions were as follows: initial denaturation at 95°C for 3 min, followed by 45 cycles of denaturation at 95°C for the 20 s, annealing at 55-58°C for 20 s and extension at 72°C for 25 s. A melting curve was performed from 65°C to 95°C with 0.5°C increment for 0.05 min for all the genes. Fold difference in mRNA abundance for the target genes was calculated by using equation 2−∆∆Ct, assuming the amplification efficiency of two (Livak and Schmittgen Citation2001).
Statistical analysis
Relative mRNA abundance of the genes in the GCs of homozygous carrier GMM (FecBBB), non-carrier GMM (FecB++), and non-carrier Malpura (FecB++) ewes were expressed as relative fold unit (RFU). Expression of each gene was calculated using ΔCt method (Target gene Ct – RPL19 housekeeping gene Ct) for all individual samples, where Ct is the PCR cycle number at which the fluorescence signal generated within a reaction crosses an arbitrary threshold. Differences in the gene expression among the groups were estimated using 2−∆∆Ct method of Livak and Schmittgen (Citation2001). Quantitative real-time PCR data on different gene transcripts were analyzed by one way ANOVA and post ANOVA pairwise comparison by Fisher’s LSD in SYSTAT 12.0 program.
Authors’ contributions
Contributed to the molecular work and generated real-time PCR data: SK, PKR; statistically analyzed the research data: VK; was involved in the culture of granulosa cells: BJ; carried out estrus synchronization and laparoscopic collection of the ovary: SVB, DK; planned the overall experiment, experimental design, and manuscript preparation: SK. All authors read and approved the final manuscript.
Acknowledgments
Authors are highly thankful to the Director, ICAR-CSWRI Avikanagar for providing the necessary facilities for carrying out this research work.
Disclosure statement
No potential conflict of interest was reported by the authors.
Additional information
Funding
References
- Attisano L, Wrana JL. 1996. Signal transduction by members of the transforming growth factor-β superfamily. Cytokine Growth Factor Rev. 7:327–339.
- Bahire SV, Rajput PK, Kumar V, Kumar D, Kataria M, Kumar S. 2019. Quantitative expression of mRNA encoding BMP/SMAD signaling genes in the ovaries of Booroola carrier and non‐carrier GMM sheep. Reprod Dom Animals. 00.1-9. doi:10.1111/rda.13535.
- Campbell BK, Baird DT, Souza CJH, Webb R. 2003. The FecB (Booroola) gene acts at the ovary: in vivo evidence. Reprod. 126(1):101–111.
- Campbell BK, Scaramuzzi R, Webb R. 1996. Induction and maintenance of oestradiol and immuno-reactive inhibin production with FSH by ovine granulosa cells cultured in serum-free media. J Reprod Fertil. 106:7–16.
- Campbell BK, Souza CJH, Skinner AJ, Webb R, Baird DT. 2006. Enhanced response of granulosa and theca cells from sheep carriers of the FecB mutation in vitro to gonadotropins and bone morphogenic protein-2, −4, and −6. Endocrinol. 147:1608–1620.
- Castro F, Cruz M, Leal C. 2015. Role of growth differentiation factor 9 and bone morphogenetic protein 15 in ovarian function and their importance in mammalian female fertility -a review. Asian Aust J Anim Sci. 29(8):1065–1074.
- Chang H, Huylebroeck D, Verschueren K, Guo Q, Matzuk MM, Zwijsen A. 1999. Smad5 knockout mice die at mid-gestation due to multiple embryonic and extraembryonic defects. Dev. 126:1631–1642.
- Chen D, Zhao M, Mundy GR. 2004. Bone morphogenetic proteins. Growth Factors. 22(4):233–241.
- Chu MX, Liu ZH, Jiao CL, He YQ, Fang L, Ye SC, Chen GH, Yang JY. 2007. Mutations in BMPR-IB and BMP-15 genes are associated with litter size in small tailed Han sheep (Ovis areas). J Anim Sci. 85(3):598–603.
- Crawford JL, Heath DA, Reader KL, Quirke LD, Hudson NL, Juengel JL, McNatty KP. 2011. Oocytes in sheep homozygous for a mutation in bone morphogenetic protein receptor 1B express lower mRNA levels of bone morphogenetic protein 15 but not growth differentiation factor 9. Reprod. 142:53–61.
- Davis GH, Balakrishnan L, Ross IK, Wilson T, Galloway SM, Lumsden BM, Hanrahan JP, Mullen M, Mao XZ, Wang GL, et al. 2006. Investigation of the Booroola (FecB) and Inverdale (FecXI) mutations in 21 prolific breeds and strains of sheep sampled in 13 countries. Anim Reprod Sci. 92:87–96.
- Davis GH, Galloway SM, Ross IK, Gregan MS, Ward J, Nimbkar BV, Ghalsasi PM, Nimbkar C, Gray GD, Subandryo II, et al. 2002. DNA test in prolific sheep from eight countries provide new evidence on origin of the Booroola (FecB) mutation. Biol Reprod. 66:1869–1874.
- De K, Kumar D, Sethi D, Gulyani R, Naqvi SMK. 2015. Estrus synchronization and fixed-time artificial insemination in sheep under field conditions of a semi-arid tropical region. Trop Anim Health Prod. 47(2):469–472.
- Driancourt MA, Cahill LP, Bindon BM. 1985. Ovarian follicular populations and preovulatory enlargement in Booroola and control Merino ewes. J Reprod Fertil. 73:93–107.
- Drouilhet L, Mansanet C, Sarry J, Tabet K, Bardou P, Woloszyn F, Lluch J, Harichaux G, Viguié C, Monniaux D, et al. 2013. The highly prolific phenotype of Lacaune sheep is associated with an ectopic expression of the B4GALNT2 gene within the ovary. PLoS Genet. 9(4):1003809.
- Estienne A, Pierre A, Di Clemente N, Picard JY, Jarrier P, Mansanet C, Monniaux D, Fabre S. 2015. Anti-Müllerian hormone regulation by the bone morphogenetic proteins in the sheep ovary: deciphering a direct regulatory pathway. Endocrinol. 156(1):301–313. doi:10.1210/en.2014-1551.
- Fabre S, Pierre A, Mulsant P, Bodin L, Pasquale ED, Persani L, Monget P, Monniaux D. 2006. Regulation of ovulation rate in mammals: contribution of sheep genetic models. Reprod Biol Endocrinol. 4:20.
- Fabre S, Pierre A, Pisselet C, Mulsant P, Lecerf F, Pohl J, Monget P, Monniaux D. 2003. The Booroola mutation in sheep is associated with an alteration of the bone morphogenetic protein receptor-IB functionality. J Endocrinol. 177:435–444.
- Feary ES, Juengel JL, Smith P, French MC, O’Connell AR, Lawrence SB, Galloway SM, Davis GH, McNatty KP. 2007. Patterns of expression of messenger RNAs encoding GDF9, BMP15, TGFβR1, BMPR1B, and BMPR2 during follicular development and characterization of ovarian follicular populations in ewes carrying the Woodlands FecX2W mutation. Biol Reprod. 77:990–998.
- Franzen P, Ten Dijke P, Ichijo H, Yamashita H, Schulz P, Heldin CH, Miyazono K. 1993. Cloning of a TGF beta type I receptor that forms a heteromeric complex with the TGF beta type II receptor. Cell. 75(4):681–692.
- Frota IM, Leitao CC, Costa JJ, Van Den Hurk R, Saraiva MV, Figueiredo JR, Silva JR. 2013. Levels of BMP-6 mRNA in goat ovarian follicles and in vitro effects on BMP-6 on secondary follicle development. Zygotes. 21(3):270–278.
- Galloway SM, McNatty KP, Cambridge LM, Laitinen MP, Juengel JL, Jokiranta TS, McLaren RJ, Luiro K, Dodds KG, Montgomery GW, et al. 2000. Mutations in an oocyte-derived growth factor gene (BMP15) cause increased ovulation rate and infertility in a dosage-sensitive manner. Nat Genet. 25:279–283.
- Gilchrist R, Lane M, Thompson J. 2008. Oocyte-secreted factors: regulators of cumulus cell function and oocyte quality. Hum Reprod Update. 14(2):159–177.
- Gonzalez-Bulnes A, Souza CJ, Campbell BK, Baird DT. 2004. Effect of ageing on hormone secretion and follicular dynamics in sheep with and without the Booroola gene. Endocrinol. 145(6):2858–2864.
- Goyal S, Aggarwal J, Dubey PK, Mishra BP, Ghalsasi P, Nimbkar C, Joshi BK, Kataria RS. 2017. Expression analysis of genes associated with prolificacy in FecB carrier and non-carrier Indian sheep. Anim Biotech. 28(3):1–8.
- Hanrahan JP, Gregan SM, Mulsant P, Mullen M, Davis GH, Powell R, Galloway SM. 2004. Mutations in the genes for oocyte-derived growth factors GDF9 and BMP15 are associated with both increased ovulation rate and sterility in Cambridge and Belclare sheep (Ovis aries). Biol Reprod. 70:900–909.
- Hanukoglu I. 1992. Steroidogenic enzymes: structure, function, and role in regulation of steroid hormone biosynthesis. J. Steroid Biochem Mol Biol. 43(8):779–804.
- Horbelt D, Denkis A, Knaus P. 2012. A portrait of transforming growth factor β superfamily signaling: background matters. Int J Biochem Cell Biol. 44(3):469e474.
- Juengel JL, Hudson NL, Heath DA, Smith P, Reader KL, Lawrence SB, O’Connell AR, Laitinen MP, Cranfield M, Groome NP, et al. 2002. Growth differentiation factor 9 and bone morphogenetic protein 15 are essential for ovarian follicular development in sheep. Biol Reprod. 67:1777–1789.
- Juengel JL, Reader KL, Bibby AH, Lun S, Ross I, Haydon LJ, McNatty KP. 2006. The role of bone morphogenetic proteins 2, 4, 6 and 7 during ovarian follicular development in sheep: contrast to rat. Reprod. 131:501–513.
- Kaivo-oja N, Jeffery LA, Ritvos O, Mottershead DG. 2006. Smad signaling in the ovary. Reprod Biol Endocr. 4:21. doi:10.1186/1477-7827-4-21.
- Kolte AP, Mishra AK, Kumar S, Arora AL, Singh VK. 2005. A study on effect of carrying FecB gene on body weight in Garole and Malpura sheep. Asian-Aust J Anim Sci. 18:1379–1382.
- Kumar D, Joshi A, Naqvi SMK, Kumar S, Mishra AK, Maurya VP, Arora AL, Mittal JP, Singh VK. 2007. Sperm motion characteristics of Garole × Malpura sheep evolved in a semi-arid tropical environment through introgression of FecB gene. Anim Reprod Sci. 100:51–60.
- Kumar D, Naqvi SMK, Kumar S. 2012. Sperm motion characteristic of FecBBB and FecBB+ Garole X Malpura rams during non-breeding season under hot semi-arid environment. Livest Sci. 150:337–341.
- Kumar S, Kolte AP, Mishra AK, Arora AL, Singh VK. 2006. Identification of FecB mutation in Garole x Malpura sheep and its effect on litter size. Small Rumin Res. 64:305–310.
- Kumar S, Mishra AK, Kolte AP, Arora AL, Singh D, Singh VK. 2008. Effects of the Booroola (FecB) genotypes on growth performance, ewe’s productivity efficiency and litter size in Garole × Malpura sheep. Anim Reprod Sci. 105:319–331.
- Kumar S, Mishra AK, Kolte AP, Dash SK, Karim SA. 2008a. Screening for Booroola (FecB) and Galway (FecXG) mutations in Indian sheep. Small Rum Res. 80:57–61.
- Lachance Y, Luu-The V, Labrie C, Simard J, Dumont M, de Launoit Y, Guérin S, Leblanc G, Labrie F. 1992. Characterization of human 3 beta-hydroxysteroid dehydrogenase/delta 5-delta 4-isomerase gene and its expression in mammalian cells. J Biol Chem. 267(5):3551.
- Livak KJ, Schmittgen TD. 2001. Analysis of relative gene expression data using real-time quantitative PCR and the 2(-Delta Delta C (T)) method. Methods. 25(4):402–408.
- Loomans HA, Andl CD. 2016. Activin receptor-like kinases: a diverse family playing an important role in cancer. Am J Cancer Res. 6(11):2431–2447.
- Mahdavi M, Nanekarani S, Hosseini SD. 2014. Mutation in BMPR-IB gene is associated with litter size in Iranian Kalehkoohi sheep. Anim Reprod Sci. 147(3–4):93–98.
- Massagué J. 1998. TGF-beta signal transduction. Ann Rev Biochem. 67:753–791.
- Massagué J. 2000. How cells read TGF-beta signals. Nat Rev Mol Cell Biol. 1:169–178.
- Maurya VP, Naqvi SMK, Kumar S, Kumar D, Joshi A, Gulyani R. 2008. Comparative assessment of sexual behaviour and ovulation rate in prolific and non-prolific sheep reared under semi-arid tropical climate. Indian J Anim Sci. 78(8):805–807.
- Mazerbourg S, Klein C, Roh J, Kaivo-Oja N, Mottershead DG, Korchynskyi O, Ritvos O, Hsueh AJ. 2004. Growth differentiation factor-9 signaling is mediated by the Type I receptor, activin receptor-like kinase 5. Mol Endocrinol. 18:653–665.
- McNatty KP, Henderson KM, Lun S, Heath DA, Ball K, Hudson NL, Fannin J, Gibb M, Kieboom LE, Smith P. 1985. Ovarian activity in Booroola x Romney ewe which have a major gene influencing their ovulation rate. J Reprod Fertil. 1985(73):109–120.
- McNatty KP, Hudson NL, Lun S, Heath DA, Shaw L, Condell LA, Phillips DJ, Clarke IJ. 1993. Gonadotrophin-releasing hormone and the control of ovulation rate by the FecB gene in Booroola ewes. J Reprod Fertil. 98:97–105.
- McNatty KP, Hudson NL, Shaw L, Condell LA, Ball K, Seah SL, Clarke IJ. 1991. GnRH-induced gonadotrophin secretion in ovariectomized Booroola ewes that are homozygous carriers or non-carriers of the FecB gene. J Reprod Fertil. 91:583–592.
- McNatty KP, Lun S, Heath DA, Ball K, Smith P, Hudson NL, McDiarmid J, Gibb M, Henderson KM. 1986. Differences in ovarian activity between Booroola Merino ewes which were homozygous, heterozygous and non-carriers of a major gene influencing their ovulation rate. J Reprod Fertil. 77:193–205.
- Mulsant P, Lecerf F, Fabre S, Schibler L, Monget P, Lanneluc I, Pisselet C, Riquet J, Monniaux D, Callebaut I, et al. 2001. Mutation in the bone morphogenetic protein receptor-IB is associated with increased ovulation rate in Booroola ewes. Proc Natl Acad Sci USA. 98:5104–5109.
- Nicol L, Bishop SC, Pong-Wong R, Bendixen C, Holm LE, Rhind SM, McNeilly SA. 2009. Homozygosity for a single base-pair mutation in the oocyte-specific GDF9 gene results in sterility in Thoka sheep. Reprod. 138(6):921–933.
- Nilsson EE, Skinner MK. 2003. Bone morphogenetic protein-4 acts as an ovarian follicle survival factor and promotes primordial follicle development. Biol Reprod. 69(4):1265–1272.
- Oraon T, Singh DK, Ghosh M, Kullu SS, Kumar R, Singh LB. 2016. Allelic and genotypic frequencies in polymorphic Booroola fecundity gene and their association with multiple births and postnatal growth in Chhotanagpuri sheep. Vet World. 9(11):1294–1299.
- Otsuka F, McTavish K, Shimasaki S. 2011. Integral role of GDF-9 and BMP-15 in ovarian function. Mol Reprod Dev. 78(1):9–21.
- Polley S, De S, Brahma B, Mukherjee A, Vinesh PB, Batabyal S, Arora JS, Pan S, Samanta AK, Datta TK, et al. 2010. Polymorphism of BMPR-1B, BMP-15 and GDF-9 fecundity genes in prolific Garole sheep. Trop Anim Health Prod. 42:985–993.
- Reader KL, Haydon LJ, Littlejohn RP, Juengel JL, McNatty KP. 2012. Booroola BMPR1B mutation alters early follicular development and oocyte ultrastructure in sheep. Reprod Fertil Dev. 24(2):353–361.
- Regan SL, McFarlane JR, O’Shea T, Andronicos N, Arfuso F, Dharmarajan A, Almahbobi G. 2015. Flow cytometric analysis of FSHR, BMRR1B, LHR and apoptosis in granulosa cells and ovulation rate in merino sheep. Reprod. 150:151–163.
- Rosairo D, Kuyznierewicz I, Findlay J, Drummond A. 2008. Transforming growth factor beta: its role in ovarian follicle development. Reprod. 136(6):799–809.
- Roy J, De S, Polley S, Goswami SL. 2011. Polymorphism of fecundity genes (FecB, FecX, and FecG) in the Indian Bonpala sheep. Anim Biotech. 22(3):151–162.
- Sekelsky JJ, Newfeld SJ, Raftery LA, Chartoff EH, Gelbart WM. 1995. Genetic characterization and cloning of mothers against dpp, a gene required for decapentaplegic function in Drosophila melanogaster. Genet. 139(3):1347–1358.
- Souza CJH, Campbell BK, Webb R, Baird DT. 1997. Secretion of inhibin A and follicular dynamics throughout the estrus cycle in the sheep with or without the Booroola gene (FecB). Endocrinol. 138:5333–5340.
- Souza CJH, Gonzalez-Bulnes A, Campbell BK, McNeilly AS, Baird DT. 2004. Mechanisms of action of the principal prolific genes and their application to sheep production. Reprod Fertil Dev. 16:395–401.
- Souza CJH, MacDougall C, Campbell BK, McNeilly AS, Baird DT. 2001. The Booroola (FecB) is associated with a mutation in the bone morphogenetic protein receptor type 1b (BMPR1B) gene. J Endocrinol. 169:R1–R6.
- Souza CJH, Moraes JCF, Chagas LM. 1994. Effect of the Booroola gene on time of ovulation and ovulatory dynamics. Anim Reprod Sci. 37:7–13.
- Sudhakar S, Rajendran R, Rahumathulla PS. 2014. Detection of Booroola (FecB) mutation in Indian sheep-Nilagiri. Small Rum Res. 113(1):55–57.
- Tang J, Hu W, Chen S, Di R, Liu Q, Wang X, He X, Gan S, Zhang X, Zhang J, et al. 2019. The genetic mechanism of high prolificacy in small tail Han sheep by comparative proteomics of ovaries in the follicular and luteal stages. J Proteomics. 27:103394.
- Tremblay KD, Dunn NR, Robertson EJ. 2001. Mouse embryos lacking Smad1 signals display defects in extra-embryonic tissues and germ cell formation. Dev. 128:3609–3621.
- Untergasser A, Cutcutache I, Koressaar T, Ye J, Faircloth BC, Remm M, Rozen SG. 2012. Primers were designed using internet-based Primer3 software. Nucleic Acids Res. 40:15.
- Vage DI, Husdal M, Kent MP, Klemetsdal G, Boman IA. 2013. A missense mutation in growth differentiation factor 9 (GDF9) is strongly associated with litter size in sheep. BMC Genet. 14:1.
- Vitt UA, Mazerbourg S, Klein C, Hsueh AJW. 2002. Bone morphogenetic protein receptor Type II is a receptor for growth differentiation factor-9. Biol Reprod. 67:473–480.
- Wang RN, Green J, Wang Z, Deng Y, Qiao M, Peabody M, Zhang Q, Ye J, Yan Z, Denduluri S, et al. 2014. Bone morphogenetic protein (BMP) signaling in development and human diseases. Genes Dis. 1(1):87–105.
- Wilson T, Wu XY, Juengel JL, Ross IK, Lumsden JM, Lord EA, Dodds KG, Walling GA, McEwan JC, O’Connell AR, et al. 2001. Highly prolific Booroola sheep have a mutation in the intracellular kinase domain of bone morphogenetic protein 1B receptor (ALK-6) that is expressed in both oocytes and granulosa cells. Biol Reprod. 64:1225–1235.
- Xia Y, O’Shea T, Murison R, McFarlane JR. 2003. Concentrations of progesterone, follistatin, and follicle stimulating hormone in peripheral plasma across the estrus cycle and pregnancy in merino ewes that are homozygous or non-carriers of the Booroola gene. Biol Reprod. 69:1079–1084.
- Xu YF, Li EL, Han YD, Chen L, Xie ZA. 2010. Differential expression of mRNAs encoding BMP/Smad pathway molecules in antral follicles of high- and low-fecundity Hu sheep. Anim Reprod Sci. 120:47–55.
- Yadin D, Knaus P, Mueller TD. 2016. Structural insights into BMP receptors: specificity, activation and inhibition. Cytokine Growth Factor Rev. 27:13–34.
- Young JM, Juengel JL, Dodds KG, Laird M, Dearden PK, McNeilly AS, McNatty KP, Wilson T. 2008. The activin receptor-like kinase 6 Booroola mutation enhances suppressive effects of bone morphogenetic protein 2 (BMP2), BMP4, BMP6 and growth and differentiation factor-9 on FSH release from ovine primary pituitary cell cultures. J Endocrinol. 196(2):251–261.
- Zuo B, Qian H, Wang Z, Wang X, Nisa N, Bayier A, Ying S, Hu X, Gong C, Guo Z, et al. 2013. A study on BMPR-IB genes of Bayanbulak sheep. Asian-Aust J Anim Sci. 26(1):36–42.