ABSTRACT
Bacterial contamination in swine semen affects the quality and longevity of sperm and consequently fertility is reduced. Antibiotics have been used to prevent bacterial growth, but the frequency of bacterial resistance to various antibiotics are increasing. Silver nanoparticles (AgNPs) of 10–20 nm in size have shown a biocide effect in bacteria and fungi microorganisms without toxicity to certain mammalian cells. The goal of this study was to analyze both, antimicrobial activity against Staphylococcus aureus and toxicity in swine sperms after 10–20 nm AgNPs treatment. S. aureus proliferation decreased when concentrations from 0.4 to 10 mM AgNPs were assayed. Also, sperm viability measured by mitochondrial metabolism after AgNPs treatment up to a concentration of 10 mM, was viable. In addition, viability determined by membrane integrity of sperms showed that AgNPs treatment up to a concentration of 10 mM was safe. Sperm morphology was evaluated by automated quantification of proximal and distal drops and whiptails. Data indicated that AgNPs treatment up to a concentration of 4 mM were harmless. Finally, sperm capacitation and acrosome reactions were determined by (chlortetracycline) CTC assay. Data showed that no changes in sperm capacitation were observed when sperms were treated with 2 mM of AgNPs, but data showed increased calcium mobilization when treated with 10 mM AgNPs, which suggested sperm capacitation. Finally, there were no significant changes encountered on sperm acrosome reaction for any of the treatments after AgNPs treatment. Taken together, these results show the potential of AgNPs as an alternative to conventional antimicrobial agents that are currently used in extenders to preserve semen required for storage.
Abbreviations
AgNPs: silver nanoparticles; AMK: amikacin; AMP: adenosine monophosphate; AR: acrosome reaction; C: capacitation; CF: cefallotin; CFU: colony-forming unit; CTC: chlortetracycline; CXM: cefuroxime; DMSO: dimethyl sulfoxide; NC: non-capacitation; NOM: Norma Oficial Mexicana; PBS: phosphate buffered saline; RLUs: relative light units; ROS: reactive oxygen species; SQS: Seminal Quality System
KEYWORDS:
Introduction
Bacterial contamination in semen samples employed for assisted reproduction methods facilitates sperm agglutination, low sperm motility, and changes in seminal pH (Althouse Citation2008; Althouse et al. Citation2008). In some cases, bacterial contamination leads to diseases in the female that will undergo assisted reproduction (Pineda and Santander Citation2007). Bacterial contamination occurs when the sample is exposed to the environment, either by bacteria found in the foreskin of the animal or by mishandling the sample during acquisition or use. (Bennemann et al. Citation2018). In consequence, the quality and longevity of the sperm is affected and fertility is reduced. Commercial extenders of semen contain a variety of antibiotics to prevent bacterial growth (Althouse Citation2008). Some pathogenic microorganisms, such as Escherichia coli, Sthaphylococcus epidermidis, Klebsiella spp., Pseudomona aeruginosa and Sthaphylococcus aureus are common contaminants of swine semen (Pineda and Santander Citation2007). Due to the increasing frequency of bacterial resistance to various antibiotics, alternatives to the use of these substances have been proposed (Dakal et al. Citation2016). Several authors have demonstrated the biocide effect of AgNPs, interact with pathogenic microorganisms through a series of mechanisms that are not yet fully understood (Lara et al. Citation2011; Acosta-Torres et al. Citation2012; Ortiz-Gil et al. Citation2015; Zhang et al. Citation2016; Dakal et al. Citation2016). We have previously reported that 10–20 nm spherical AgNPs, obtained by reduction of Ag+ ions, exert a biocide action on different microorganisms, including S. aureus obtained from humans (Acosta-Torres et al. Citation2012; Ortiz-Gil et al. Citation2015). Moreover, other authors have analyzed AgNP antimicrobial effect in many pathogenic microorganisms (Arzate et al. Citation2017). In fact, previously proposed AgNP antimicrobial mechanisms include: a) direct bacterium/AgNP interaction, which causes the disruption of the electron transfer mechanism in the membrane; b) penetration of AgNP into the microbial cell membrane, as well as the production of secondary metabolites such as reactive oxygen species, and c) production of heavy metal ions, which cause damage to the microorganism and prevents its growth (Acosta-Torres et al. Citation2012; Dakal et al. Citation2016). It has been demonstrated that AgNP exert an antimicrobial and antifungal effect without toxic effects on human hosts (Acosta-Torres et al. Citation2012; Nuñez-Anita et al. Citation2014). Nanoparticles for biological systems require toxicity evaluations on in vivo and in vitro models. The cytocompatibility of AgNP has been previously analyzed in mammalian cells of different cell types, such as fibroblasts and lymphocytes, in which no toxicity was observed (Acosta-Torres et al. Citation2012); whereas in cell lines HepG2 and HeLa, low cytotoxicity was observed (Zhang et al. Citation2016). We and other authors have proposed that AgNPs toxicity in mammalian cells depends on different factors: size (nanoparticles smaller than 10 nm are more toxic); shape (amorphous shapes are more toxic than spherical); dose (higher doses are more toxic); route of administration (systemic and inhaled routes are associated with greater toxicity compared to other routes); form of administration (suspended nanoparticles are usually more toxic than AgNP coatings), with toxicity depending on the cell type diverse (Acosta-Torres et al. Citation2012; Nuñez-Anita et al. Citation2014; Abdulkareem and Hatim Citation2015; Salomoni et al. Citation2017).
AgNP toxicity has been poorly studied in germ cells such as sperm. It has been reported that AgNPs are toxic for mouse and rat sperm (Braydich-Stolle et al. Citation2005, Citation2010; Gromadzka-Ostrowska et al. Citation2012; Asare et al. Citation2012; Yoisungnern et al. Citation2015; Lafuente et al. Citation2016; Gromadzka-). Conversely, AgNPs showed no toxicity in human sperm (Moretti et al. Citation2013; Wang et al. Citation2017) (). Current literature, suggests that this is the first time in which the effect of a10–20 nm AgNPs biocide has been studied on swine sperms. In addition, it is the first time that toxicity of nanoparticles and their influence in the function of swine sperm has been evaluated.
Table 1. AgNP toxicity in some sperm species.
Results and discussion
The broad antimicrobial effects of silver are well known. In fact, silver has been used in different fields of medicine for many years (Burdușel et al. Citation2018; Tarannum and Divya Citation2019). Recently, silver has been employed as part of different biomaterials in the form of nanoparticles: AgNP gels, AgNP coatings, AgNP in aqueous solution and many others (Acosta-Torres et al. Citation2012; López-Pérez et al. Citation2017; Burdușel et al. Citation2018; Tarannum and Divya Citation2019). Dakal et al. (Citation2016), reviewed several AgNP antimicrobial mechanisms, such as, adhesion to microbial cells, penetration inside cells, ROS and free radical generation, and modulation of microbial signal transduction pathways. In this context, it is known that silver antimicrobial effects are dependent on superficial contact. Other authors described that AgNP can penetrate the cells, then; silver ions can inhibit enzymatic systems in the respiratory chain and alter DNA synthesis, with some preference over bacterial cells (Brett Citation2006). However, the resistance of some pathogens such as S. aureus to silver was reported by Li et al. (Citation1997), who proposed that plasmids within pathogens and pathogens’ own DNA provide the means to achieve silver resistance through beta-lactamases. In addition, E. coli mutant strains deficient in some types of porins were 4 to 8-fold more resistant to silver nanoparticles compared to the wild-type strain. This is an indication that porins may play an important function related to antibacterial effects of silver nanoparticles (Duran et al. Citation2016). These data suggest that certain bacteria may have resistance to AgNPs.
The synthesis, morphology and size of AgNPs used in this work have been previously reported in Acosta-Torres et al. (Citation2012). shows the calibration curve of commercial AgNPs to determine experimental concentrations, as well as characteristic spectra at 420 nm. In this work, we showed that 10–20 nm AgNPs do not induce AgNP resistance in our samples. Antimicrobial activities of AgNPs on S. aureus obtained from semen were evaluated using the following AgNP concentrations: 0.4, 1, 2, 4 or 10 mM. In the control condition (in the absence of AgNPs), bacterial cells grow normally, but all treatments with AgNPs or antibiotics, reduce S. aureus growth (). When AgNPs vs antibiotic treatments are compared,, their behavior was similar, remaining at a concentration of approximately 1.5 × 106 CFU/ml. Our results showed that in the S. aureus strain obtained from swine semen, proliferation decreased similarly in both cases, i.e., antibiotics or 10–20 nm AgNPs. This indicates that S. aureus is highly susceptible to AgNPs. Wong and Liu (Citation2010), proposed two possible mechanisms of AgNP toxicity in bacteria. One is that AgNP provide a large surface for contact with bacteria, which may allow the particles to attach to the cell membrane and easily penetrate into the bacteria. Another possibility is that silver nanoparticles or silver ions interfere with the respiratory chain in bacterial mitochondria, resulting in cell death.
Figure 1. AgNP concentration by optical density. A) Calibration curve to determine AgNP concentrations from commercial AgNPs (SIGMA-Aldrich). B) Spectra of AgNPs obtained from Ag+ reduction at 420 nm.
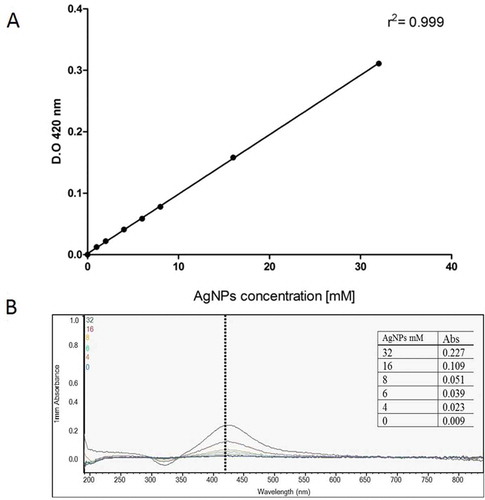
Figure 2. S. aureus growth assessed by the BactiterGlo test. Bacterial viability is proportional to relative light units (RLUs). Antibiotics were used as controls, amikacin (AMK) [30 μg/ml], cefallotin (CF) [30 μg/ml], and cefuroxime (CXM) [30 μg/ml]. Bacteria were incubated for 24 hours at 37°C after treatments and evaluated. Different letters above the bars represent significant differences.
![Figure 2. S. aureus growth assessed by the BactiterGlo test. Bacterial viability is proportional to relative light units (RLUs). Antibiotics were used as controls, amikacin (AMK) [30 μg/ml], cefallotin (CF) [30 μg/ml], and cefuroxime (CXM) [30 μg/ml]. Bacteria were incubated for 24 hours at 37°C after treatments and evaluated. Different letters above the bars represent significant differences.](/cms/asset/a1deca93-4b9b-4d43-b17c-7cebe5c04476/iaan_a_1754962_f0002_b.gif)
In another group of experiments, motility, viability, sperm morphology, concentration and calcium mobilization patterns employing fluorescent chlortetracycline calcium-binding, known as CTC assays, were analyzed in swine sperms to determine AgNPs toxicity, given the absence of data in the literature for this species. Sperm motility showed a slight tendency to increase when sperms were treated with AgNPs, however, no statistically significant differences were observed (). Sperm viability measured by metabolic activity showed no changes at all doses analyzed (). Data suggest biocompatibility of AgNP with swine sperm. To corroborate these data, sperm viability was measured with an automatized Seminal Quality System (SQS). Results showed viability of AgNPs at all concentrations tested (,) showed representative photographs.
Table 2. Motility of sperms after AgNPs treatment.
Figure 3. Sperm viability. A) Sperm viability measured by MTT assay. The percentage of sperm viability was determined by MTT assay after AgNPs treatment. B) Sperm viability measured by SQS system after AgNPs treatment. The percentage of sperm viability was determined using the sperm classification software of the SQS equipment after AgNPs treatments. C) Representative microphotographs of sperm according to treatment conditions captured in SQS system. In both experiments, sperms were treated as follows: a) control; b) vehicle control; c) 2 mM AgNPs; d) 4 mM AgNPs, and e) 10 mM AgNPs, followed by incubation for 1 h. No significant differences were observed. N = 8.
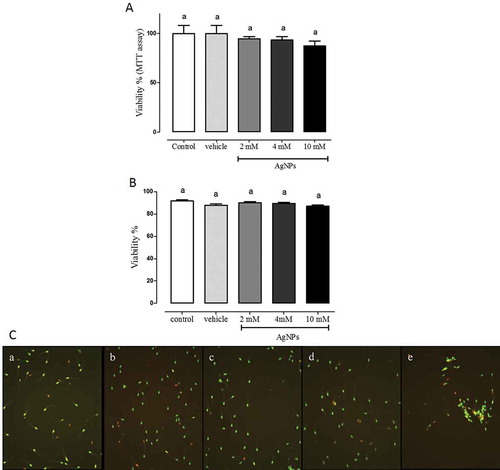
Sperm concentration was measured with an automatized SQS system. The same concentration of sperms were used in each experiment, showing that AgNPs apparently decreased sperm concentration only after 10 mM AgNPs treatment (). Also, it is important to note that, sperm aggregation was observeded at 10 mM AgNPs (). These data correlate with apparent decreased concentration measured with 10 mM AgNPs, indicating the possibility that sperm clusters were not properly counted by the software program. However, additional experiments will be necessary to corroborate this finding. Morphology changes were determined by automated counting using SQS system, such as, proximal drops, distal drops, and whip tails. The lack of changes in sperm morphology at 2 or 4 mM of AgNPs treatment, but, contrasted treatment with 10 mM AgNPs that increased whip tails ().
Figure 4. Sperm concentration analysis. Sperm concentration was determined using the sperm classification software of the SQS equipment. Sperm was treated according to these experimental groups: control, vehicle, 2, 4 or 10 mM followed by incubation for 1 h. Different letters above the bars represent significant differences. N = 8.
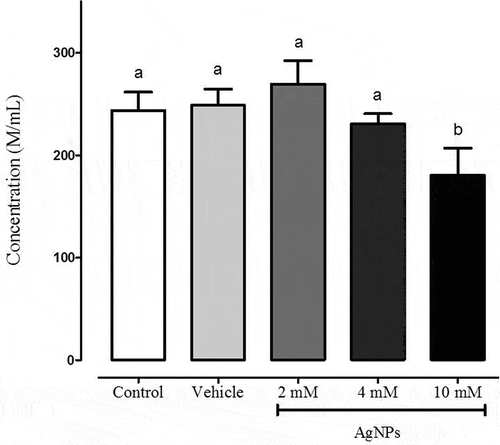
Figure 5. Sperm morphology. The percentage of Aa) proximal drops, Bb) distal drops and Cc) whip tails was determined after 1 hour of treatment with AgNP at the following concentrations: control, vehicle, 2, 4 or 10 mM. Representative photographs were included. Different letters above the bars represent significant differences. N = 8.
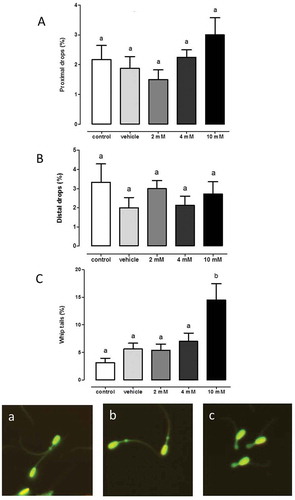
Sperm capacitation was analyzed by chlortetracycline assay (CTC assay) in sperm exposed to 2 or 10 mM AgNP. Fluorescence patterns were analyzed as previously described and classified as follows: capacitation (C), non-capacitation (NC) and acrosome reaction (AR). No significant changes were observed with 2 mM AgNPs compared with untreated sperms. But, capacitated sperms significantly increased when sperm was treated with 10 mM of AgNPs. Moreover, at both concentrations non-capacitated sperms decreased significantly compared to the control group ().
Figure 6. Calcium mobilization patterns through CTC assay. CTC assay of sperm samples exposed to different AgNP concentrations: control, 2 mM or 10 mM. Fluorescence patterns were analyzed under fluorescence confocal microscopy. Groups were classified as: capacitation (C), non-capacitation (NC) and acrosome reaction (AR). Different letters above bars represent significant differences with respect to the control. B) Representative photographs of CTC assay, Confocal Microscopy.
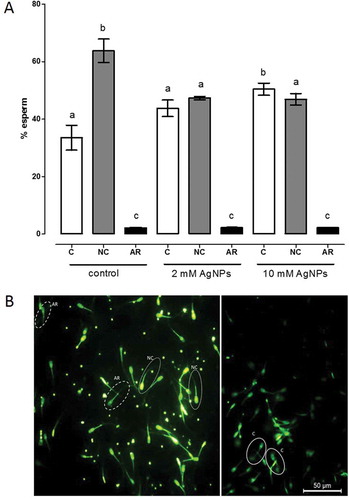
In vivo, after ejaculation, sperm cells go through several essential physiological changes during their time in female reproductive tract to reach and penetrate the oocyte membrane. The first change in this cascade is capacitation. The primarily event in capacitation is cholesterol efflux leading to the elevation of intracellular calcium and bicarbonate activating adenylyl cyclase to produce cyclic AMP, which activates a protein kinase to indirectly phosphorylate tyrosine residues on certain proteins. Whereas, in vitro, components such as serum albumin, calcium and bicarbonate, play important regulatory roles in promoting capacitation. In addition, changes in temperature or other components can induce calcium mobilization. For example, in vitro, changes in the porcine sperm membrane have been previously reported as ‘capacitation like changes’ which can appear in absence of any special media to induce sperm capacitation (Conejo-Nava et al. Citation2009). It is known that AgNPs can induce ROS production (Dakal et al. Citation2016). On one hand some reactive oxidants at low concentrations can show a positive effect and act selectively. On the other hand, limited amounts of reactive oxygen species (ROS) can also interfere physiologically in the regulation of sperm functions (Sanocka and Kurpisz Citation2004). In fact, low amounts of free radicals in human semen enhance the ability of spermatozoa to bind to the zona pellucida. In addition, the incubation of sperm cells with low concentrations of hydrogen peroxide was found to stimulate sperm capacitation, hyperactivation, acrosome reaction and oocyte fusion (Sanocka and Kurpisz Citation2004; Mayorga-Torres et al. Citation2015). However, additional studies are required to fully elucidate the role and effect of AgNPs on porcine sperm capacitation. In this context, nanoparticles are being explored extensively with regard to their toxicological effects, as well as possible risks to animal and human health (Nuñez-Anita et al. Citation2014).
The extensive use of new antimicrobial nanoparticles and the diversity of applications need to be thoroughly studied to determine their cytotoxicity and implications on cell function according to the systems in which these nanoparticles will be employed. The size of the nanoparticles allows them to enter into living microorganisms and cells, causing several cellular changes, necessitating the importance of understanding their toxicity. Doses of 0.4 mM to 4 mM of AgNPs were sufficinent to inhibit S. aureus growth, without toxic effects on swine sperm. But a dose up to 10 mM caused changes in sperm morphology and calcium mobilization. Taken together these data suggest a potent antimicrobial effect of AgNPs against S. aureus without toxic effects on swine sperms can be achieved at these lower concentrations.
Materials
Strains: Sthaphylococcus aureus obtained from swine semen was isolated and identified, according to established standard protocols.
Silver nanoparticles: AgNPs from a 20 mg/ml stock solution in water (spherical and 10–20 nm size) were employed. The obtention method of AgNP was previously reported (Acosta-Torres et al. Citation2012). AgNPs were maintained in distilled water and tested at different concentrations. A calibration curve was carried out using commercial AgNPs (730,785, Sigma-Aldrich, St. Louis, MO). A linear regression analysis was performed and yielded a value r2 = 0.99.
Antibiotics: Antibiotics used as controls were: Amikacin (AMK) [30 μg/ml], Cefallotin (CF) [30 μg/ml], and Cefuroxime (CXM) [30 μg/ml] (PiSA farmacéutica, México), which are common antibiotics against S.aureus.
All chemicals were of the highest grade of purity commercially available.
Sampling: Eight clinically healthy swine males in reproductive stage (between 2–4 years of age), Pietrain, Landrace and Yorkshire breeds were used to obtain semen samples. Pigs were kept under temperature conditions (25 + 1°C) at 50% humidity on individual stockyards and permitted ad libitum access to food and purified water.
All procedures were performed using an artificial vagina, which involved a conical thin latex sleeve ending in a sterile collection tube, without external lubricant. The procedure of sampling collection was through manual stimulation, also known as manual ejaculation. Animals were previously trained. All efforts were made to minimize discomfort.
Only samples with up to 70–80% of visual microscopic viability were included in the experiments. Determination of motility was evaluated by the same experimenter.
Methods
Evaluation of S. aureus growth assessed by the BactiterGlo test
Tubes containing Luria Bertani medium and isolated bacteria were incubated under continuous shaking at 180 rpm for 2 h. At 37°C, an initial inoculum reached an absorbance between 0.08–0.1 at a wavelength of 625 nm, equivalent to 0.5 on the McFarland scale corresponding to 1.5 × 108 CFU/ml. From there, a 1:100 dilution of the inoculum was selected for the proliferation assay, i.e., 1.5 × 106 CFU/ml (Mirzajani et al. Citation2011).
From this solution (1.5 x 106/ml of bacteria) 500 μl were placed in Eppendorf tubes and AgNPs in different concentrations were added: 0.4, 1, 2, 4 or 10 mM. Subsequently, tubes were kept under continuous stirring at 180 rpm during 22 h, 37°C. After this incubation period, BactiterGlo reagent (PROMEGA, Madison WI, USA) was added in a 1:1 vol/vol proportion, and after 5 minutes of orbital shaking, the luciferase reading was recorded in the Varioskan LUX equipment (Thermo Scientific, USA). The light emitted was registered as relative light units (RLUs).
Sperm viability assay
Cell viability was determined by 3-(4, 5-dimethylthiazol-2-yl)-2, 5-diphenyltetrazolium bromide (MTT) assay. The MTT assay is a calorimetric assay to determine viable cells through the assessment of mitochondrial metabolic activity. From each animal analyzed, 100 µl of ejaculated semen were placed by quadruplicate in 99 well plates at room temperature (approximate 24–26°C). Next, AgNPs were added according to the experimental groups: control, vehicle (water), 2, 4 or 10 mM of AgNPs. Immediately, 20 μl of MTT (5 mg/ml in PBS) were added to each well, and cells were incubated for 1 h at 37°C. The supernatants were removed by centrifugation at 2500 rpm during 5 min in a centrifuge with a 96-well plate rotor (Universal 320 centrifuge, Hettich). Then, 200 μl of dimethyl sulfoxide (DMSO) were added, and the absorbance (595 nm) was determined with a microplate reader (Bio-Rad, Hercules, CA, USA). Cell viability was expressed as the percentage of change over the control group and calculated using the formula: (mean OD of treated cells/mean OD of untreated control cells)×100.
Sperm motility
The assessment of sperm motility was made within a couple of minutes from semen collection and measured in phase contrast microscope coupled to a camera. A small drop of semen was placed on a slide and examined directly using the 10 X lens at 25°C. Samples before treatment showed 80 ± 2% of motility. Immediately, 500 µl of semen without dilution was placed in 1.5 ml tubes according to the experimental groups: control, vehicle (water), 2, 4 or 10 mM of AgNPs. After 1 h incubation at 25°C, motility was analyzed.
Visual assessment of sperm motility included total sperm motility (percentage of sperm that exhibit motility of any form). Rapid and slow progressive motility was calculated by the speed at which sperm moved with flagellar movement in a given volume as a percentage (range 0%-100%). Moreover, for evidence of mass motility was scored from no swirling wave to rapid swirling wave on a 1–5 scale. All experiments were made by the same experimenter with extensive experience. The experimenter estimated the proportion of sperm that were not moving forward and categorized the sample with respect to the controls.
Analysis of the integrity of the sperm membrane (viability), concentration and morphology
In 1.5 ml Eppendorf tubes, a sample (100 μl) of fresh semen previously diluted 1:10 was deposited with the Androhep® EnduraGuardTM diluent and neomycin sulfate, prepared according to the manufacturer’s instructions. Immediately, AgNPs were added at concentrations of 0, 2, 4 or 10 mM. Incubation was carried out for 1 hour at room temperature between 25 and 27°C. Next, each sample was gently agitated by manual inversion of the tube. A 20 μl aliquot was placed in the tube provided in the commercial kit (Swine Sperm Viability and Morphology kit, Zoitech Lab, Madrid, Spain) and mixed carefully until the suspension turned into a red/orange color, which indicated the end of the staining process. Then, a 2 μl drop of the cell suspension was placed inside the counting chamber of the SQS device (Zoitech Lab, Madrid, Spain); manufacturer’s instructions were followed to perform an automated analysis of the samples. Photographs and data were visualized, using the SQS software. Data such as % of viability, concentration, % of proximal drops, % of distal drops and % of whip tails were obtained directly from SQS software.
Analysis of sperm capacitation and acrosome reaction
A volume of 0.5 ml of semen was placed in Eppendorf tubes and centrifuged at 2000 rpm for 10 minutes. The sperm button was suspended in 100 μl of fixing buffer (0.2% glutaraldehyde, 0.5 M Tris; final pH 7.4). According to the procedure by Fraser and Herod (Citation1990), the sperm was stained with chlortetracycline (CTC, Sigma Aldrich) (stock solution, 0.0020 gr of CTC diluted in 3 ml buffer (20 mM Tris, NaCl 130 mM, 5 mM L-cisteína pH 7.8). Mixing a suspension of 5 μl of fixed spermatozoa with 5 μl of CTC on a glass slide was then incubated at room temperature during 60 seconds. Subsequently, a drop of 1.4-Diaza-bicyclo (2.2.2) octane (0.22 M, DABCO, Sigma Aldrich) in 90% glycerol was added to preserve fluorescence. Incubation took place for 24 h at room temperature in the dark. Sperm smears were analyzed under fluorescence confocal microscopy and photographed (FluoView FV1000 Confocal Microscope, Olympus). The results are described as distinctive staining patterns as follows: Non-capacitated spermatozoa showed uniform fluorescence in the head and intact acrosome, whereas capacitated spermatozoa presented fluorescence concentrated in the acrosomal region, non-fluorescent band in the post acrosomal region, and intact acrosome. Sperm with acrosome reaction, presented no fluorescence in the head but a thin band in the equatorial region. Counts of a minimum of 200 sperm cells per preparation were carried out.
Statistical analysis
Statistical analysis: Data represents the results of at least four independent experiments by quadruplicate. The differences between the experimental groups were analyzed with one-way ANOVA and Tukey’s post hoc test. Differences with a value of P ≤ 0.05 were considered statistically significant. The GraphPad Prism V5 software was employed.
Ethics and animal care
All of the procedures followed the Animal Care and Use Program (NIH, USA) and Norma official Mexicana NOM-066-ZOO-1999, which include protocols of handling, care, use and welfare of swine and were approved by the Institutional Research Ethics Committee at IIBQ-UMSNH (Protocol #IIQB-CIBE-05-2017).
Author contributions
Data acquisition, and data interpretation: FP-D, LSA-T, PNS-D, EG-C; reagents: LSA-T, IBO-Z; samples collection and seminal attributes examination: IAT-T, IBO-Z; critical analysis, data interpretation, and critical revision of the article for intellectual content: LSA-T, REN–A; conception and design of the work, data analysis, interpretation, drafting of the article: FP-D, REN-A.
Acknowledgments
We are grateful for the technical support and SQS system provided by Servicios de Occidente S.A de C.V., Av. Atotonilco No. 4584-A Col. Benito Juárez, Jalisco, México and ZoitechLab. S.L., Calle Calibre 121, Polígono Industrial P-29, Collado-Villalba, Madrid, España. We thank CIC-UMSNH founding (2018-2019), which made part of this research possible. We thank Proteomic and Cellular Bioengineering Unit, INFR-2015-01 255010 (CONACyT).
Disclosure statement
The authors declare that they have no conflict of interests regarding this study.
Additional information
Funding
References
- Abdulkareem M, Hatim N. 2015. Evaluation the biological effect of adding aluminum oxide, silver nanoparticles into microwave treated PMMA powder. IJERSTE. 4:172–178.
- Acosta-Torres LS, Mendieta I, Nunez-Anita RE, Cajero-Juarez M, Castano VM. 2012. Cytocompatible antifungal acrylic resin containing silver nanoparticles for dentures. Int J Nanomed. 7:4777–4786. doi:10.2147/IJN.S32391.
- Althouse GC. 2008. Sanitary procedures for the production of extended semen. Reprod Dom Anim. 43:374–378. doi:10.1111/j.1439-0531.2008.01187.x.
- Althouse GC, Pierdon MS, Lu KG. 2008. Thermotemporal dynamics of contaminant bacteria and antimicrobials in extended porcine semen. Theriogenol. 70:1317–1323. doi:10.1016/j.theriogenology.2008.07.010.
- Arzate QC, Sánchez RB, Infante RR. 2017. Toxicity effects in pathogen microorganisms exposed to silver nanoparticles. Nanosci Nanotechnol Lett. 9:165–173. doi:10.1166/nnl.2017.2275.
- Asare N, Instanes C, Sandberg WJ, Refsnes M, Schwarze P, Kruszewski M, Brunborg G. 2012. Cytotoxic and genotoxic effects of silver nanoparticles in testicular cells. Toxicology. 291:65–72. doi:10.1016/j.tox.2011.10.022.
- Bennemann PE, Machado SA, Girardini LK, Sonálio K, Tonin AA. 2018. Bacterial contaminants and antimicrobial susceptibility profile of boar semen in Southern Brazil Studs. Rev MVZ Cordoba. 23(2):6637–6648.
- Braydich-Stolle L, Hussain S, Schlager JJ, Hofmann MC. 2005. In vitro cytotoxicity of nanoparticles in mammalian germline stem cells. Toxicol Sci. 88:412–419. doi:10.1093/toxsci/kfi256.
- Braydich-Stolle LK, Lucas B, Schrand A, Murdock RC, Lee T, Schlager JJ, Hussain SM, Hofmann MC. 2010. Silver nanoparticles disrupt GDNF/Fyn kinase signaling in spermatogonial stem cells. Toxicol Sci. 116:577–589. doi:10.1093/toxsci/kfq148.
- Brett DW. 2006. A discussion of silver as an antimicrobial agent: alleviating the confusion. Ostomy Wound Manage. 52:34–41.
- Burdușel AC, Gherasim O, Grumezescu AM, Mogoantă L, Ficai A, Andronescu E. 2018. Biomedical applications of silver nanoparticles: an up-to-date overview. Nanomaterials. 8(9):681. doi:10.3390/nano8090681.
- Conejo-Nava J, Fierro R, Gutiérrez CG, Betancourt M. 2009. Membrane status and in vitro capacitation of porcine sperm preserved in long-term extender at 16°C. Arch Androl. 49(4):287–295. doi:10.1080/01485010390204931.
- Dakal TC, Kumar A, Majumdar RS, Yadav V. 2016. Mechanistic basis of antimicrobial actions of silver nanoparticles. Front Microbiol. 7:1831. doi:10.3389/fmicb.2016.01831.
- Duran N, Duran M, de Jesus MB, Seabra AB, Favaro WJ, Nakazato G. 2016. Silver nanoparticles: A new view on mechanistic aspects on antimicrobial activity. Nanomedicine: NBM. 12:789–799. doi:10.1016/j.nano.2015.11.016.
- Fraser LR, Herod JE. 1990. Expression of capacitation-dependent changes in chlortetracycline fluorescence patterns in mouse spermatozoa requires a suitable glycolysable substrate. J Reprod Fertil. 88:611–621. doi:10.1530/jrf.0.0880611.
- Gromadzka-Ostrowska J, Dziendzikowska K, Lankoff A, Dobrzynska M, Instanes C, Brunborg G, Gajowik A, Radzikowska J, Wojewodzka M, Kruszewski M. 2012. Silver nanoparticles effects on epididymal sperm in rats. Toxicol Lett. 15:251–258. doi:10.1016/j.toxlet.2012.08.028.
- Lafuente D, Garcia T, Blanco J, Sanchez DJ, Sirvent JJ, Domingo JL, Gomez M. 2016. Effects of oral exposure to silver nanoparticles on the sperm of rats. Reprod Toxicol. 60:133–139. doi:10.1016/j.reprotox.2016.02.007.
- Lara HH, Garza-Treviño EN, Ixtepan-Turrent L, Singh DK. 2011. Silver nanoparticles are broad-spectrum bactericidal and virucidal compounds. J Nanobiotechnology. 9:2–9. doi:10.1186/1477-3155-9-30.
- Li XZ, NIKAIDO H, Williams KE. 1997. Silver-resistant mutants of escherichia coli display active efflux of Ag1 and are deficient in porins. J Bacteriol. 179:6127–6132. doi:10.1128/JB.179.19.6127-6132.1997.
- López-Pérez R, Acosta-Torres LS, Serrano-Díaz P, Avilés-López YS, Toscano-Torres IA, Olivo-Zepeda IB, Pérez-Duran F, Núñez-Anita RE. 2017. Silver nanoparticles prevent bacterial growth without toxic effects on swine semen. AICA. 10:34–40.
- Mayorga-Torres BJM, Camargo M, Cadavid ÁP, Cardona-Maya WD. 2015. Estrés oxidativo: ¿un estado celular defectuoso para la función espermática? Rev. Chil Obstet Ginecol. 80:486–492. doi:10.4067/S0717-75262015000600009.
- Mirzajani F, Ghassempour A, Aliahmadi A, Esmaeili MA. 2011. Antibacterial effect of silver nanoparticles on Staphylococcus aureus. Res Microbiol. 162:542–549. doi:10.1016/j.resmic.2011.04.009.
- Moretti E, Terzuoli G, Renieri T, Iacoponi F, Castellini C, Giordano C, Collodel G. 2013. In vitro effect of gold and silver nanoparticles on human spermatozoa. Andrología. 45:392–396. doi:10.1111/and.12028.
- Nuñez-Anita RE, Acosta-Torres LS, Vilar-Pineda J, Martinez-Espinosa JC, de la Fuente-hernandez J, Castano VM. 2014. Toxicology of antimicrobial nanoparticles for prosthetic devices. Int J Nanomed. 9:3999–4006. doi:10.2147/IJN.S63064.
- Ortiz-Gil M, Nuñez-Anita RE, Arenas-Arrocena MC, Martínez-Alvarado O, Emiliano-Ramírez J, De la Fuente-hernández J, A-tL S. 2015. Silver nanoparticles for the inhibition of Staphylococcus aureus. Entreciencias. 3:133–142.
- Pineda Y, Santander J. 2007. Evaluación de la flora bacteriana del semen de verracos en granjas porcinas de Venezuela. Zootecnia trop. 25:173–177.
- Salomoni R, Leo P, Montemor AF, Rinaldi BG, Rodrigues M. 2017. Antibacterial effect of silver nanoparticles in Pseudomonas aeruginosa. Nanotechnol Sci Appl. 10:115–121. doi:10.2147/NSA.S133415.
- Sanocka D, Kurpisz M. 2004. Reactive oxygen species and sperm cells. Reprod Biol Endocrinol. 2:12. doi:10.1186/1477-7827-2-12.
- Tarannum N, Divya GYK. 2019. Facile green synthesis and applications of silver nanoparticles: A state-of-the-art review. RSC Adv. 9(60):34926–34948. doi:10.1039/C9RA04164H.
- Wang E, Huang Y, Du Q, Sun Y. 2017. Silver nanoparticle induced toxicity to human sperm by increasing ROS (reactive oxygen species) production and DNA damage. Environ Toxicol Pharmacol. 52:193–199. doi:10.1016/j.etap.2017.04.010.
- Wong KKY, Liu X. 2010. Silver nanoparticles—the real “silver bullet” in clinical medicine? MedChemComm. 1:125. doi:10.1039/c0md00069h.
- Yoisungnern T, Choi YJ, Han JW, Kang MH, Das J, Gurunathan S, Kwon DN, Cho SG, Park C, Chang WK, et al. 2015. Internalization of silver nanoparticles into mouse spermatozoa results in poor fertilization and compromised embryo development. Sci Rep. 8(5):11170. doi:10.1038/srep11170
- Zhang XF, Liu ZG, Shen W, Gurunathan S. 2016. Silver nanoparticles: synthesis, characterization, properties, applications, and therapeutic approaches. Int J Mol Sci. 17(9):1534. doi:10.3390/ijms17091534.