ABSTRACT
The prevalence of infertility is increasing and worrisome. About 10 to 30% of infertility is classified as idiopathic or unexplained infertility (UI).TGF-β is multifunctional and immunoregulatry cytokine which regulates both implantation and adhesion of trophoblasts to the extracellular matrix during pregnancy. The aim of the current study was to investigate the association between two polymorphisms rs1800470 (C29T) and rs1800471 (G74C) of the TGF-β1 gene in Iranian patients with unexplained infertility. A total of 250 UI patients and 484 healthy individuals with no history of infertility were included in the study. The amplification and sequencing of target DNA fragments were done using PCR and automated sequencing methods, respectively. The effects of these polymorphisms on both TGF-β1 structure and function of mRNA and protein were analyzed using new in-silico tools. The frequency distribution of the alleles, genotypes, and haplotypes of both rs1800470 and rs1800471 polymorphisms had a statistically significant difference between subjects and controls. CC genotype of TGF-β1 rs1800470 (29C→T) increase the risk of UI in male UI patients. Moreover, C alleles of TGF-β1 rs1800471 was associated with increased risk of UI in female UI patients. Couples, subgroup analysis revealed a significant association between TGF-β1 polymorphisms (rs1800470, rs1800471) and the risk of UI in male, female, and all UI patients. The frequency of TG and CG haplotypes were statistically different in both UI and healthy subjects group (P < 0.05). RS1800471 polymorphisms changed the secondary structure of TGF-β1 mRNA and resulted in the removal of one mRNA arm and creation of two new arms. Taken together, the results of the current study suggest that TGF-β1 functional polymorphisms may play an important role in the susceptibility to UI in Iranian population. According to in silico analysis, polymorphisms in TGF-β1 can reduce mRNA half-life and, therefore, reduced TGF-β1 expression.
Introduction
The fertility of a couple is characterized by their capability to obtain a successful pregnancy. In 2010, the prevalence of 60-month infertility was 1.5% in Europe (Boivin et al. Citation2007). Thus far, the major causes of infertility have been divided into three main categories with variable prevalence (Roupa et al. Citation2009; Parsanezhad et al. Citation2016; Punab et al. Citation2016): male causes (25 to 39 %), female causes (33 to 41 %), and mixed causes (9 to 39 %). Various factors are involved in idiopathic infertility, including obstructions that restrict the delivery of sperm, anti-sperm antibodies, low or abnormal sperm function, hormone imbalances, infection, varicocele, or tumors (Krausz Citation2011). Female infertility is mainly due to tubal infertility, ovulation disorders (hypothalamic dysfunction, polycystic ovary syndrome, and premature ovarian insufficiency), uterine and cervical causes (polyps, cervical stenosis, and tumors), and/or endometriosis (Dor et al. Citation1977; Poppe and Velkeniers Citation2004). Based on the previous studies, an etiologic evaluation of 8–20% is considered as ‘unexplained or idiopathic infertility’ (Gelbaya et al. Citation2014). Immune infertility is considered as one of the major factors in couples with idiopathic infertility (Carp et al. Citation2012). Uterine immune modulation is essential in protecting the fetus in its semiallogenic state. It has been reported that an imbalance between prorejective and antirejective cytokines has a negative effect on implantation (Wilczyński Citation2005). Among the antirejective cytokines which are effective in a successful pregnancy is TGF-β1 (Schäfer-Somi Citation2003). TGF-β1 is a pleotropic cytokine which is mainly synthesized and released by regulatory T cells (Li Citation2014). This cytokine is a potent immune regulator and is engaged in the embryo’s implantation process and the binding of trophoblast cells to the extracellular matrix (Li Citation2014). It also stimulates the production of proteolytic enzymes which facilitate the penetration of the trophoblast into the prepared endometrium (Poniatowski et al. Citation2015). Semen contains a large amounts of active and inactive forms of TGF-β1, which regulate the production of inflammatory cytokines and chemokines involved in the implantation process (Robertson et al. Citation2002). The acidic milieu of mother’s reproductive system can be an activation factor for TGF-β1 (Guzeloglu-Kayisli et al. Citation2009). This cytokine is involved in immune deviation from Th1 to Th2 and plays an essential role in maintaining pregnancy (Qiu et al. Citation2016). The human TGF-β1 gene, which is located on chromosome 19q13 (19q13.2–19q13.1), encodes the secreted form of the TGF-beta (Massague Citation1990). This gene spans 23.5 kb and contains seven exons and six introns (Cebinelli et al. Citation2016). Considering the significant role of TGF- β in successful pregnancy, various single nucleotide polymorphisms (SNPs) in the exon 1 and exon 2 of the human TGF-β1 gene have been reported (Cebinelli et al. Citation2016). These SNPs are suggested to be related to the basal levels of this cytokine (Cebinelli et al. Citation2016). The +869 C > T SNP (rs1800470) polymorphism, also recognized as c.+29C>T and Pro10Leu, and the +915 G > C SNP (rs1800471) polymorphism, also recognized as c.+74 G > C and Arg25Pro, are found in the signal peptide sequence of TGF-β1 gene and affect the serum concentration of this cytokine (Khani et al. Citation2015).
To the best of our knowledge, no study has so far investigated the association between TGF-β1 rs1800470 and rs1800471 polymorphisms and the risk of unexplained infertility. Therefore, we aimed to elucidate whether there is an association between TGF-β1 polymorphisms and the risk of UI in Iranian population.
Results
Patients and control subjects
In this case-control study, participants were comprised of 250 infertile subjects (178 men and 72 women, mean age of 35.8 ± 4.5 and 31.9 ± 4.9, respectively) and 484 healthy controls (306 men and 178 women, mean age of 39 ± 3 and 32 ± 3.7, respectively). A total of 72 infertile couples and 74 healthy couples were among the participants. There were significant differences between UI and control groups based on sex and age ().
Table 1. Demographic information of patients and healthy participants.
SNP identification
Sequencing of exon 1 and exon 2 of TGFβ1 gene in 250 UI patients and 484 healthy participants detected four different base changes, which schematically illustrated in ( and ). These base changes included two SNP at the signal peptide of exon 1 (29 C→T and 74 G→C) and two uncommon polymorphisms of rs199758510 and rs201567874 in exon1 and exon 2, respectively. Each of these two uncommon SNPs had low allele frequencies; therefore, only the results of SNPs at the signal peptide of exon 1 (rs1800470 and rs1800471) are reported.
Genotype and allele frequencies of TGF-β1 rs1800470 (29 C→T)
The genotype and allele frequencies of TGF-β1 rs1800470 (29 C→T(gene polymorphism between the patients and the healthy participants are shown in -. Alleles C and T for rs1800470 (29 C→T(SNP were identified in 53.7% and 47.3% of male UI patients, respectively, approximately equal to allele frequencies of control group (51% and 49%, respectively) (p = 0.15, OR = 1.2, 95% CI: 0.93 to 1.57); . On the other hand, the frequencies of C and T Alleles in female UI patients and female control were 51.4% and 48.6% as well as 49.1% and 50.9%, respectively (p = 0.65, OR = 1.09, 95% CI: 0.74 to 1.61); . Overall, there was no significant differences in allelic frequency of rs1800470 (29 C→T(polymorphism between All UI patients and healthy control group; . Furthermore, no significant difference was found in CC and CT genotypes frequency between male UI patients and male control groups (p = 0.177, OR = 1.41, 95% CI: 85 to 2.33, p = 0.55, OR = 0.87, 95% CI: 0.55 to 1.37, respectively). The CT genotype was shown in 42.1% of male UI patients and 50% of healthy participants, and it was identified as the most common genotype. The genotypes frequency of CC and CT did not showed a significant differences between All UI patients and healthy control groups (p = 0.34, OR = 1.34, 95% CI: 0.88–2.02, and p = 0.64, OR = 0.91, 95% CI: 0.63–1.33, respectively); . Moreover, the distribution of genotype and allele frequencies of TGF-β1 rs1800470 (29 C→T(between male UI and female UI patients missed the statistically significant threshold; .
Table 2. Allele and genotype frequencies of TGF-β1 gene SNPs in male UI patients and male control participants.
Table 3. Allele and genotype frequencies of TGF-β1 gene SNPs in female UI patients and control female subjects.
Table 4. Allele and genotype frequencies of TGF-β1 gene SNPs in all UI patients and all control individuals.
Table 5. Allele and genotype frequencies of TGF-β1 gene SNPs in male and female UI patients.
Genotype and allele frequencies of TGF-β1 rs1800470 in couples
Separate analysis of the genotype and allele frequencies of TGF-β1 rs1800470 gene polymorphism between the patients and the healthy individuals in 72 infertile and 74 healthy couples are shown in -. The frequencies of CC genotype in male UI patients compared to that of healthy control groups, was observed to be statistically significant (p = 0.018, OR = 2.42, 95% CI: 1.16 to 5.2); . In addition, the frequency of CC genotype in All UI patients was marginally different compared to healthy subjects (p = 0.08, OR = 1.93, 95% CI: 1.02 to 3.67). Neither allele nor genotype frequencies of TGF-β1 rs1800470 (29 C→T(in female UI patients reached statically significant threshold; . Finally, There was a significant difference in the frequencies of C Alleles for rs1800470 SNP in All UI patients as compared to the control group (p = 0.03, OR = 1.42, 95% CI: 1.03 to 1.97); .
Table 6. Allele and genotype frequencies of TGF-β1 gene SNPs in male UI patients and control individuals between couples.
Table 7. Allele and genotype frequencies of TGF-β1 gene SNPs in female UI patients and control individuals between couples.
Table 8. Allele and genotype frequencies of TGF-β1 gene SNPs in All UI patients and control individuals between couples.
Table 9. Allele and genotype frequencies of TGF-β1 gene SNPs in male and female UI patients between couples.
Genotype and allele frequencies of TGF-β1 rs1800471 (74 G→C)
The genotype and allele frequencies of TGF-β1 rs1800471 (74 G→C) gene polymorphism between the patients and the healthy participants are shown in , , and . The G and C variants of TGF-β1 rs1800471 (74 G→C) were equally distributed among male UI patients and healthy participants (92.1% vs. 93.8.8% respectively for G allele; and 7.9% vs. 6.2% respectively for C allele) and therefore no significant difference was found between the two groups (p = 0.32, OR = 1.28, 95% CI: 0.77 to 2.14); . In comparison between female UI and control groups, C alleles was associated with increased risk of UI (p = 0.02, OR = 2.44, 95% CI: 1.14 to 5.21); . The GG genotype was the most common genotype in both All UI patients and controls (86.4% vs. 89%, respectively). On the contrary, the CC genotype had the lowest distribution in both All UI patients and healthy groups (3.2% vs. 0%, respectively); there was a significant difference in the genotype frequency of CC in All UI patients compared to healthy subjects (p = 0.028, OR = 33.8, 95% CI: 1.94 to 589.1); . The frequencies of C allele for rs1800471 (74 G→C) SNP was 8.4% in All UI patients and 5.5% in healthy subjects, and it was marginally associated with increased risk of UI in All UI patients (p = 0.06, OR = 1.49, 95% CI: 0.98 to 2.27); . The GC genotype was identified in 10.4% of All UI patients and 11% of healthy participants; and the genotype frequency difference was not observed to be statistically significant (p = 0.93, OR = 0.97, 95% CI: 0.59 to 1.60); . Considering the subgroup analysis in male UI and female UI patients, no significant difference was observed in the alleles and genotypes frequencies of TGF-β1 rs1800471 (74 G→C) SNP; .
Genotype and allele frequencies of TGF-β1 rs1800471 in couples
Analysis of the genotype and allele frequencies of TGF-β1 rs1800471 (74 G→C) gene polymorphism between patients and healthy participants in couples are shown in -. CC genotype had the lowest frequency and there was no significant difference in the genotype frequency of CC in male, female, and all UI patients compared to control groups (p = 0.46, OR = 3.36, 95% CI: 0.13 to 84.1, p = 0.12, OR = 10.44, 95% CI: 0.55 to 197.7, p = 0.06, OR = 14.9, 95% CI: 0.83 to 268.1) –. The frequencies of genotypes GC and CC for rs1800471 (74 G→C) SNP were 8.5% and 1.2% in male UI patients and 1.4% and 0% in healthy subjects, respectively; . The C allele of rs1800471 (74 G→C) SNP was associated with increased risk of the UI in female groups (p = 0.007, OR = 7.86, 95% CI: 1.75 to 35.2); . While the frequencies of GC genotype in male and female UI patients were not statistically significant, the frequencies of GC genotype showed significant differences in All UI patients as compared to the healthy control group (p = 0.04, OR = 4.60, 95% CI: 1.27 to 16.68); . Moreover, there was a significant difference in the frequencies of C alleles for rs1800471 (74 G→C) SNP in all UI patients compared to the control group (p = 0.001, OR = 6.1, 95% CI: 2.05 to 17.74); .
Haplotype frequencies
Four different haplotypes were made by two 29 C→T and 74 G→C polymorphisms (). The most common haplotypes were CG and TG with the frequency of 38.5% and 49.4% in All UI patients. As well as, CG and TG with the frequency of 40.2% and 49.3% were the most frequent haplotypes in couples with UI. The frequencies of TG and CG haplotypes were significantly different in both male UI and all UI patients as compared with control groups (P < 0.05). Except for the CG haplotype, there was a significant difference in haplotypes frequencies between UI and control couples. Moreover, the frequencies of TC and CC haplotypes in male UI patients, and CC haplotype in both female and all UI patients were significantly different compared with those of healthy group (P < 0.0001). As a matter of fact, none of these haplotypes were seen in healthy control couples. Overall, the frequencies of haplotypes in male and all UI patients, as compared with control groups, showed significant differences ().
Table 10. Comparison of haplotype frequency distributions between UI patients and controls.
In silico results
The effect of TGF-β1 gene polymorphisms on the structure and function of Protein
By examining the secondary structure of the TGF-β1 signal peptide protein using the PSIPRED server, the presence of proline at either positions 10 or 25 shortens the structure of the helix α (). Based on the information obtained from the SNAP2 tool, both P10L and R25P polymorphisms can cause a disruption of protein function (respectively, score: 4 expected accuracy: 53%, and score: 5 expected accuracy: 75%). Furthermore, the data of SUSPECT tool also indicated the role of both polymorphisms P10 L (score: 38) and R25P (score: 68) in unstable protein secondary structure (Score: neutral (0) -Disease associated (100)).
The evaluation of Ramachandran plot using DISCOVERY-STUDIO-VISUALIZER software shows that the proline at position 25 changes the α-helix structure (; lower left quadrant) of the TGF-β1 peptide signal by placing in the β sheet regions (; upper left-hand quadrant). Furthermore, the evaluation of protein hydrophobicity suggests that both P10 L and R25P polymorphisms increase protein hydrophobicity. Thus, the displacements of R25P and P10 L, increase hydrophobicity at specific positions from −1.2 to −0.7 and from 2.8 to 3.8, respectively ().
The effect of TGF-β1 gene polymorphisms on the structure and function of mRNA
The effect of polymorphisms on the structure and function of mRNA was investigated using the RNAsnp Web Server and the results indicated that, compared to P10 L, R25P polymorphism has a more destructive effect on mRNA (P value: 0.1315, and P value: 0.312 respectively, P > 0.2 is significant); . Moreover, evaluation of the secondary structure of TGF-β1 mRNA indicated that, while P10 L polymorphism does not affect the secondary structure of this molecule, R25P polymorphism led to a severe and destructive change in the secondary structure of mRNA (). One of the mRNA arms removed and also the structure of the other arm severely disrupted and two new loops added to the molecule ().
Discussion
TGF-β is a multifunctional cytokine that plays an essential role in various biological processes including bone formation, cell replication, migration, differentiation, healing, apoptosis, immune system regulation, and angiogenesis (Krstic and Santibanez Citation2014; Poniatowski et al. Citation2015). This cytokine belongs to a TGF-superfamily that contains more than 30 forms of cytokines such as bone morphogenetic protein (BMP), growth differentiation factor (GDF), activins, inhibins, anti-Müllerian hormone (AMH), and TGF-β (Kingsley Citation1994). Three homologous isoforms of TGF-β have been identified in mammals (TGF-β1, TGF-β2, and TGF-β3), of which TGF-β1 that is the most abundant isoform which is ubiquitously expressed (Lichtman et al. Citation2016). Various functions of TGF-β have been elucidated in ovarian, embryo implantation, gonad and secondary sex organ development, placental development, immune regulation of pregnancy, and spermatogenesis (Yoshimura and Muto Citation2010; Morikawa et al. Citation2016). Additionally, TGF-β exists in semen and is generated by the decidua and embryo; hence, it may have a potential role in generating and maintaining an immunotolerant milieu for the conceptus (Ni and Li Citation2017).
The expression of TGF-β seems to be diminished in a number of reproductive disorders, including recurrent spontaneous abortion, unexplained infertility, pre-eclampsia, endometriosis, breast cancer, prostate cancer, and penile fibrosis (Kajdaniuk et al. Citation2013). Evidently, TGF-β is implicated in Treg cell development, and delivery of exogenous male TGF-β via coitus significantly increased vaginal Treg cell numbers (Robertson et al. Citation2009). Previously, the low expression of TGF- β and pregnancy failure were shown (Gorivodsky et al. Citation1999). Moreover, decreased TGF-β and increased IFN-γ and IL-2 serum concentration in UI patients have been reported by Jasper et al (Jasper et al. Citation2006).
The regulation of TGF- β1 synthesis happens at four major levels: transcriptional, translational, secretion, and post translational modiftiction to an active form in the extracellular setting (Meng et al. Citation2016). In the present study, we highlighted the effect of genetic variations on TGF- β1 expression. Single nucleotide polymorphisms (SNPs) are the frequent type of polymorphisms and are mainly detected in regions flanking protein-coding genes that are fundamental for gene expression but can occur in other parts of the gene. (Sachidanandam et al. Citation2001). SNPs can affect messenger RNA (mRNA) stability, gene expression, microRNA target sequence, alternative splicing, transportation of protein to endoplasmic reticulum (ER) through signal peptides, or changing protein function via amino acid alterations (Cargill et al. Citation1999).
TGF-β1 functional polymorphisms have been reported to be associated with a functional impact on TGF-1 production. Although genotype frequency of CC and CT genotype of TGF-β1 rs1800470 (29 C→T (in male, female and All UI patients as compared with control groups missed statistically significant threshold, our results showed CC genotype was associated with increased risk of UI in male UI patients (p = 0.018, OR = 2.42, 95% CI: 1.16 to 5.2). There was also a trend to increase the OR for CC genotype of TGF-β1 rs1800471 (74 G→C), as compared with healthy participants in all UI patients, indicating that patients homozygous for the C-allele may have the greatest risk for UI. Furthermore, C alleles were associated with increased risk of UI in female UI patients (p = 0.02, OR = 2.44, 95% CI: 1.14 to 5.21). Couples, subgroup analysis revealed a significant association between TGF-β1 polymorphisms (rs1800470, rs1800471) and the risk of UI in male, female, and all UI patients. Our results revealed that the CC genotype of TGF-β1 rs1800470 (29 C→T(increased the risk of UI in male UI patients. In All UI couples, allele frequencies of TGF-β1 rs1800471 (74 G→C) polymorphism, C alleles were associated with increased the risk of UI. Furthermore, the C allele was associated with increased risk of disease in female UI patients. In haplotype analysis, the frequencies of haplotypes in male and all UI patients showed significant differences as compared with control groups. The most common haplotypes were CG and TG with the frequency of 38.5% and 49.4% in All UI patients. As well as, CG and TG with the frequency of 40.2% and 49.3% were the most frequent haplotypes in couples with UI. Additionally, in silico analysis revealed that the presence of proline at either positions 10 or 25 shortens the structure of the α helix and both P10 L and R25P polymorphisms can disrupt of protein function.
Proline at position 25 changes the α- helix structure of the TGF-β1 signal peptide to a β sheets. Based on our research, P10 L polymorphism had almost no effect on the secondary structure of the related mRNA. Nonetheless, the R25P polymorphism drastically changed the secondary structure of mRNA and resulted in the removal of one mRNA arm and creation of two new arms. These changes can reduce mRNA half-life and, therefore, reduce TGF-β1 levels. By analyzing the secondary structure of the TGF-β1 signal peptide, it was determined that the presence of proline at both position 10 h and 25 led to its shortened α-helix. In addition, it increased the hydrophobicity of the signal peptide of TGF-β1 and, thus, negatively affected the transportation of the protein through endoplasmic reticulum. In comparison, in silico analysis of TGF-B1 gene promoter variant (c.–832 G > A) using TFSEARCH predicted the modified binding of two transcription factors, v‐Myb and MZF1, in the mutated promoter sequence in the Otosclerosis patients (Priyadarshi et al. Citation2013). Considering this observation, and the results of our study, functional analysis is necessary to evaluate the consequence of TGFB1 gene polymorphisms on the mRNA and protein expression, since mutation or truncation in the promoter region results in impaired protein synthesis (Chatterjee and Pal Citation2009; Daoud et al. Citation2009).
To the best of our knowledge, this is the first study to determine the contribution of TGF-β1 polymorphisms in UI. After a comprehensive search in electronic databases, no similar study in which association of TGF-β1 polymorphisms with UI was identified. Therefore, we compared the findings of the current study with the results of other reproductive and immunological disorders.
In the previous studies, the association of TGF-β1 rs1800470 (29 C→T(with different types of cancers, including oral, cardiac, esophagus, breast, nasopharynx, and lung cancer have been demonstrated (Cebinelli et al. Citation2016). Moreover, TGF-β1 rs1800471 (74 G→C) has been associated with coronary heart disease, recurrent pregnancy loss, IgA nephropathy, and chronic kidney disease (Cebinelli et al. Citation2016). On one hand, the study by Magdoud et al. patients with recurrent abortions, reported that a significant difference in the genotype frequency of RS1800470 SNP between patients and control group. CC genotype was associated with an increased risk of abortion and also there was a trend to increase the OR for CC genotype in the presence of CT genotype (Magdoud et al. Citation2013). However, the study of Amani et al failed to find an association between TGF-β1 29 C→T polymorphism and the risk of recurrent abortions (Amani et al. Citation2005). On the other hand, the study of Dunning and colleagues on women with breast cancer revealed that 29 C→T polymorphism in the peptide signal of TGF-β1 significantly increased the risk of breast cancer (Dunning et al. Citation2003). In the study of Khani et al TGF-b1 gene 788 G→C polymorphism was reported as an important factor in mediating the casual pathway of preeclampsia. In this study, at positions 74 G→C and 29 T→C the genotypes and allele frequencies revealed no significant differences between preeclampsia patients and healthy controls. However, in the position 788 G→C both genotypes and allele frequencies were significantly different between preeclampsia patients and healthy subjects (Khani et al. Citation2015).
Interestingly, these missense polymorphisms of TGF- β1 (rs1800470, rs1800471) are found in the signal peptide sequence of TGF-β1 gene and affect the serum concentration of TGF- β. Signal peptides contain three regions: N-terminal region with a positive charge, a central hydrophobic part and a polar C-terminal domain (Randall and Hardy Citation1989). The rs1800470 SNP (29 C→T) is situated in the hydrophobic region, and T allele encodes leucine and C allele encodes proline as an apolar amino acid at position 10. The rs1800471 SNP (74 G→C) at amino acid position 25 is a substitution of a large polar amino acid (allele G encodes arginine) with a small apolar proline (allele C encodes proline). This amino acid is placed adjacent to the cleavage site of pro- TGF-β, which gives rise to Latency-Associated Peptide (LAP) and mature form of TGF-β (Cambien et al. Citation1996). It has been shown that in the rs1800470 (29 C→T) SNP, C allele, as compared with T allele, potentially increases TGF- β1 secretion (Dunning et al. Citation2003). The study of Dunning and colleagues demonstrated that substitution of the proline with the lUI patients compared to control groupsucien at position 10 resulted in 2.8-fold increase in secretion of TGF- β1. These studies highlighted the fact that the allele encoding proline10 is related with increased of TGF- β1 secretion and with increased risk of breast cancer (Dunning et al. Citation2003). Furthermore, it has been found that in patients with c.+29 CC genotype, the serum concentration of TGF- β1 was higher than those with the c.+29 TT or c.+29 CT genotype. Finally individuals with the c.+74 G allele had higher serum level of TGF-β1 in comparison with the c.+74 C allele (Taubenschuß et al. Citation2013; Cao et al. Citation2014).
Some limitations of the current study should be noted, first, unexplained infertility is regarded as a polygenic disorder, and consequently it is expected that several gene loci are likely interacting in the pathogenesis of unexplained infertility. Second, while the sample size was large enough to find an association between TGF-β polymorphism and unexplained infertility, for establishing prognostic implications, more samples are needed. Third, we tested only the rs1800470 and rs1800471 SNPs from the promoter region of TGF-β. Nevertheless. It is possible that these SNPs, which are in strong linkage disequilibrium with other TGF-β polymorphisms, are associated with other SNPs associated with the same effect. Finally, other sites in promoter region of TGF-β were also found to be associated with UI susceptibility, such as rs2317130, rs11466316 and rs11466315 (Martelossi et al. Citation2016), we didn’t evaluate the association of other sites in TGF-β promoter with UI.
Considering the results of the present study, rs1800471 (74 G→C) and rs1800470 (29 C→T) were found to be associated with the risk of UI in Iranian population. We found a significant difference in genotype frequencies of c.+74 CC between all UI patients and healthy individuals indicating that patients with homozygous for the C-allele may have an enhanced risk for UI. It appears that the adverse effects of rs1800471 (74 G→C) and rs1800470 (29 C→T) polymorphisms were related to their impact on the secondary structure of the signal peptide, secondary structure of the mRNA, and consequent instability of the mRNA.
Materials and methods
Patients and control subjects
The patients were recruited from the Health Research Center Fertility and Infertility of Shahid Beheshti University of Medical Sciences, Royan Research Infertility Center and Laleh Hospital Infertility Center in Iran. 250 UI patients (178 male and 72 female) and 484 healthy subjects (306 male and 178 female) as the control group was enrolled in the study. The mean age of UI patients and healthy subject was 33.5 ± 2.5 and 35.5 ± 4.5, respectively. Healthy and fertile people who had children and had no history of UI were selected as the control group. The diagnosis of UI were made by gynecologists, obstetricians, urologists, and internal medicine specialists (Traven et al. Citation2017). The inclusion criteria for infertile females including, normal results of ovulation and normal luteal phase, normal results of fallopian tube, normal results of sex hormone analysis and normal results in semen analysis of their partners. People with anatomical, physiological, hormonal, infectious, chromosomal, genetic, and autoimmune disorders, as well as those with a history of the using a particular drug were excluded from the study. Next, written informed consent was taken from both patients and healthy participants. All participants in the study were administered in agreement with the ethical standards of the Ethics Committee at Shahid Beheshti University of Medical Sciences and with the revised Helsinki Declaration in 2000. During clinical diagnosis, about 10 ml blood samples were collected in EDTA-coated tubes from healthy controls and UI patients.
DNA extraction and sequencing of TGFβ1 gene
Blood sample was obtained from patients and healthy participants and DNA was extracted using salting out DNA extraction method (Nasiri et al. Citation2005). For evaluation of possible new SNPs in exon 1 and exon 2 of TGFβ1 gene (), direct sequencing PCR method applied. Briefly, PCR reaction was conducted in the final volume of 25 μl, containing 1 μl DNA (0.1–0.3 mg/μl), 0.8 μl of each Primer (10 pM), 12 μl 2x PCR MASTERMIX, and 1 μl Taq polymerase (TaKaRa Shuzo, Otsu, Japan). PCR thermocycler conditions were as follows: First, Denaturation at 95°C (3 min), then 35 cycles of Denaturation at 95°C (30 s), 63°C (45 s), 75°C (55 s), and the final extension at 72°C for 7 min. Next, PCR product was electrophoresed on 1.5% agarose gel with TAE Buffer for amplification validation (Supplementary Figure 1). In the next step, the gel placed on a UV transilluminator and an image for documentation and interpretation taken (Supplementary Figure 1). Forward and reverse primers sequence for exon1 and exon 2 of TGFβ1 gene were as follow: Forward, 5ʹ-GCTCCGGTTCTGCACTCT-3˴ and reverse, 5΄-CAGCCGACCCACAGCCACC-3΄; Forward, 5ʹ-GGTATCAGAGACTGACTCCA-3ʹ and reverse 5ʹ-ATCTAGGTGGACCTTGTAAC-3ʹ; respectively. The PCR product size for exon 1 and exon 2 was 383 bp and 494 bp, respectively. The amplified PCR products were cleaned up applying Exo SAP-IT enzymatic solution (Usb Corp., USA) and then sequenced via Sanger sequencing (Macrogene Co. Korea).
In-silico analysis
DNA and protein sequence of TGF-β1 gene were extracted from NCBI. Since the Protein Data Bank (PDB) file for pre- and pro-TGF-β1 was not found in any database, the prediction of the protein structure was conducted using the I-TASSER (Roy et al. Citation2010) and PHYRE2 (Kelley et al. Citation2015) online tools. For evaluation of 3D protein structures, DS-VISUALIZER software was used, and Ramachandrian plots were drawn using this software. Secondary protein structures were predicted using the PSIPRED server (McGuffin et al. Citation2000). In order to determine the effects of RS1800470 and RS1800471 polymorphisms on protein structure, SNAP2 (Bromberg and Rost Citation2007), SUSPECT (Yates et al. Citation2014), and SNPeffect4 (Yates et al. Citation2014) servers were used. Besides, to determine the effect of polymorphisms on the structure and stability of mRNA, RNAsnp server (Sabarinathan et al. Citation2013) were applied.
Statistical analysis
Allele frequency for each polymorphic allele was determined using direct allele counting method. Differences in the allele and genotype frequencies between case and healthy groups were assessed by Pearson’s Chi-square test (χ2 test). In addition, two-tailed t-test was used for assessing age between case and control groups. Furthermore, odds ratios (ORs) as the effect size with 95% confidence intervals (95% CI) were calculated. The control group was evaluated for Hardy–Weinberg Equilibrium (HWE) in each locus using SHEsis online tool, Chi-square test.
The PowerMarker V3.25 software was used for analyzing haplotype frequencies for unrelated populations [39]. In order to controlling the false discovery rate (FDR) and adjusting multiple comparisons, Benjamini and Hochberg method was used. All of statistical analyzes were done using SPSS software (version 22.0). P-value less than 0.05 was considered as statistically significant. For electro-photogram wavelengths evaluation of each sequenced sample, the Finch TV software version 1.4 was used (). Finally, the alignment and comparison of the sequenced samples with reference to the sequence of TGFβ1 gene was conducted using Codon Code Aligner software version 5.
Figure 1. Electropherogram wavelengths of sequenced samples. Analysis of the wavelengths of sequenced samples obtained from amplification of a fragment of exon 1 and exon 2 of TGF-β1 gene with a length of 494 bP and 383 bp, contain two important and two uncommon polymorphisms: A. RS1800470 polymorphism (left) and RS1800471 polymorphism (right) in exon 1; B. Rs199758510 in exon 1, C. Rs201567874 in exon 2. (arrow indicates polymorphic area).
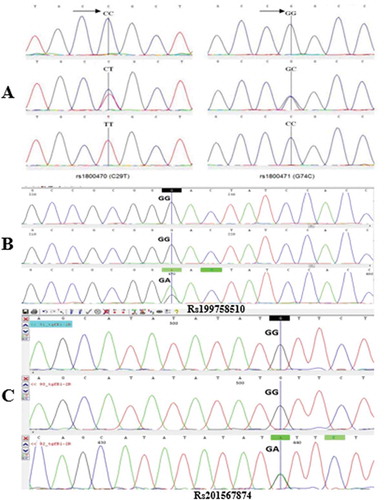
Figure 2. Structure of TGF-β1 Gene and schematic illustration of positions of detected SNPs. Exon 1 encodes the 5ʹUTR that encompasses the signal peptide (SP), Promoter region 2, and fragment of the mature protein. The SNPs c.+29 C > T and c.+74 G > C are located in signal peptide sequence. The SNPs rs199758510 and rs201567874 are located in exon 1 and exon 2, respectively. Each of these SNPs was seen only in one case. The end of exon 5 to exon 6 and exon 7 encodes the mature TGF-β1, and the exon 2, exon 3, exon 4, and part of exon 5 encode the LAP. LAP: latency-associated peptide; UTR: Untranslated Region; TGF-β1: Transforming growth factor beta 1.
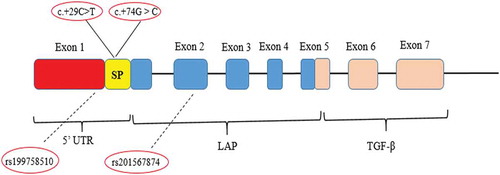
Figure 3. The effect of polymorphisms on the secondary structure of TGF-β1 signal peptide. Evaluating the secondary structure of a signal peptide that contains P10 L and R25P polymorphisms by PSIPRED online tool shows that the presence of proline amino acids at both sites causes a perturbation in the α helix structure. (C: Coil; H: Helix).
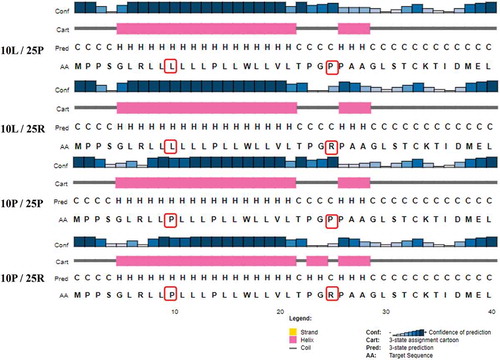
Figure 4. Ramachandran plot represents the effects of P10 L and R25P polymorphisms on protein structure. Evaluating Ramahandranian plot by DISCOVERY-STUDIO-VISUALIZER software shows that the presence of proline amino acid at position 25 (A and C) impairs the formation of α -helix structure. Actually, the prolin at position 25 by placing in the β sheet regions (upper left-hand quadrant) changes the helix α structure of (lower left quadrant) the TGF-β1 peptide signal.
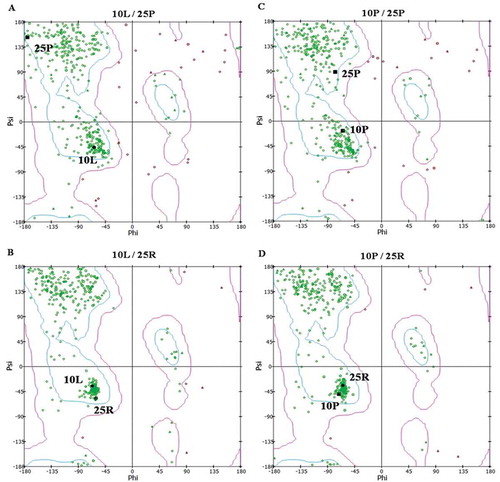
Figure 5. Protein hydrophobicity in the presence of various polymorphism compounds. Evaluation of protein hydrophobicity suggests that both polymorphisms increase protein hydrophobicity. Thus, the displacements of R25P and P10 L, increase hydrophobicity at specific positions from −1.2 to −0.7 and from 2.8 to 3.8, respectively. R25P: Arg25Pro; P10 L: Pro10 Leu.
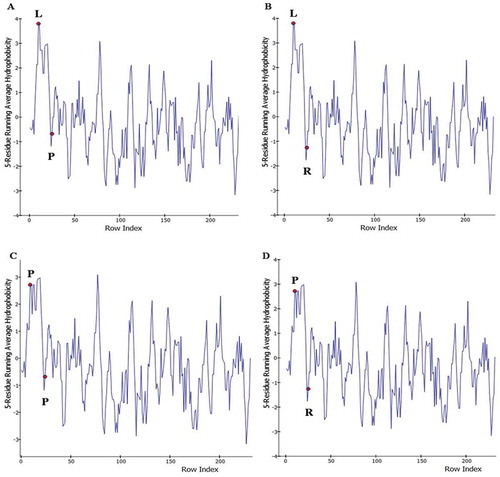
Figure 6. The secondary structure of the mRNA is predicted by the RNAsnp online tool. The wild form A) and the mutant form (B) deleted and added loops represented with the red and the green arrow, respectively. Analysis of R25P displacement’s effect on the secondary structure of mRNA predicted by the RNAsnp server: R25P polymorphism results in a severe and destructive change in the secondary structure of the mRNA. The wild form (A) and the mutant form (B) – removed loops and added loops are respectively specified with red and green arrows (P-value = 0.3126 – with the explanation that P > 0.2 is significant).
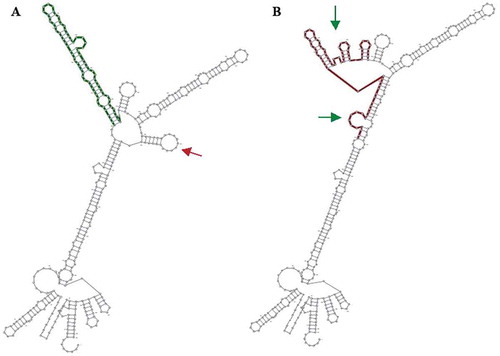
Ethics approval
This study has been approved by ethic committee of Shiraz University of Medical Sciences.
Authors’ contribution
Execution, analysis, and interpretation of data: F-M, S-M; Manuscript writing and analysis: R-R; Clinical consultant: AM, RT; Participation in study design, manuscript drafting, and critical discussion: D-A.
Figure_1.tif
Download TIFF Image (2.8 MB)2020_90.r1_Supplementary_Fig_1_caption.docx
Download MS Word (15.4 KB)Acknowledgments
Shahid Beheshti University of Medical Sciences (grant number 94-03-41-1663) funded this study.
Disclosure statement
The authors declare that they have no conflict of interest.
Supplementary material
Supplemental data for this article can be accessed here.
Data availability statement
The data that support the findings of this study are available on request from the corresponding author
Additional information
Funding
References
- Amani D, Dehaghani AS, Zolghadri J, Ravangard F, Niikawa N, Yoshiura KI, Ghaderi A. 2005. Lack of association between the TGF-β1 gene polymorphisms and recurrent spontaneous abortion. J Reprod Immunol. 68(1–2):91–103.
- Boivin J, Bunting L, Collins JA, Nygren KG. 2007. International estimates of infertility prevalence and treatment-seeking: potential need and demand for infertility medical care. Hum Reprod. 22(6):1506–1512. doi:10.1093/humrep/dem046.
- Bromberg Y, Rost B. 2007. SNAP: predict effect of non-synonymous polymorphisms on function. Nucleic Acids Res. 35(11):3823–3835. doi:10.1093/nar/gkm238.
- Cambien F, Ricard S, Troesch A, Mallet C, Generenaz L, Evans A, Arveiler D, Luc G, Ruidavets JB, Poirier O. 1996. Polymorphisms of the transforming growth factor-beta 1 gene in relation to myocardial infarction and blood pressure. The Etude Cas-Temoin de l’Infarctus du Myocarde (ECTIM) Study. Hypertension. 28(5):881–887. eng. doi:10.1161/01.HYP.28.5.881.
- Cao H, Zhou Q, Lan R, Røe OD, Chen X, Chen Y, Wang D. 2014. A functional polymorphism C-509T in TGFβ-1 promoter contributes to susceptibility and prognosis of lone atrial fibrillation in Chinese population. PLoS One. 9(11):e112912. doi:10.1371/journal.pone.0112912.
- Cargill M, Altshuler D, Ireland J, Sklar P, Ardlie K, Patil N, Shaw N, Lane CR, Lim EP, Kalyanaraman N, et al. 1999. Characterization of single-nucleotide polymorphisms in coding regions of human genes. Nat Genet. 22(3):231–238. eng. doi:10.1038/10290.
- Carp HJ, Selmi C, Shoenfeld Y. 2012. The autoimmune bases of infertility and pregnancy loss. J Autoimmun. 38(2–3):J266–J274. doi:10.1016/j.jaut.2011.11.016.
- Cebinelli GCM, Trugilo KP, Garcia SB, de Oliveira KB. 2016. TGF-β1 functional polymorphisms: a review. Eur Cytokine Netw. 27(4):81–89. doi:10.1684/ecn.2016.0382.
- Chatterjee S, Pal JK. 2009. Role of 5′‐and 3′‐untranslated regions of mRNAs in human diseases. Biol Cell. 101(5):251–262. doi:10.1042/BC20080104.
- Daoud H, Bonnet-Brilhault F, Védrine S, Demattéi M-V, Vourc’h P, Bayou N, Andres CR, Barthélémy C, Laumonnier F, Briault S. 2009. Autism and nonsyndromic mental retardation associated with a de novo mutation in the NLGN4X gene promoter causing an increased expression level. Biol Psychiatry. 66(10):906–910. doi:10.1016/j.biopsych.2009.05.008.
- Dor J, Homburg R, Rabau E. 1977. An evaluation of etiologic factors and therapy in 665 infertile couples. Fertil Steril. 28(7):718–722. doi:10.1016/S0015-0282(16)42671-3.
- Dunning AM, Ellis PD, McBride S, Kirschenlohr HL, Healey CS, Kemp PR, Luben RN, Chang-Claude J, Mannermaa A, Kataja V. 2003. A transforming growth factorβ1 signal peptide variant increases secretion in vitro and is associated with increased incidence of invasive breast cancer. Cancer Res. 63(10):2610–2615.
- Gelbaya TA, Potdar N, Jeve YB, Nardo LG. 2014. Definition and epidemiology of unexplained infertility. Obstet Gynecol Surv. 69(2):109–115. doi:10.1097/OGX.0000000000000043.
- Gorivodsky M, Torchinsky A, Zemliak I, Savion S, Fein A, Toder V. 1999. TGF beta 2 mRNA expression and pregnancy failure in mice. Am J Reprod Immunol. 42(2):124–133. eng.
- Guzeloglu-Kayisli, O., Kayisli, U.A. and Taylor, H.S., 2009. The Role of Growth Factors and Cytokines during Implantation: Endocrine and Paracrine Interactions. Semin Reprod Med. 27(1):062–079
- Jasper MJ, Tremellen KP, Robertson SA. 2006. Primary unexplained infertility is associated with reduced expression of the T-regulatory cell transcription factor Foxp3 in endometrial tissue. Mol Hum Reprod. 12(5):301–308. eng. doi:10.1093/molehr/gal032.
- Kajdaniuk D, Marek B, Borgiel-Marek H, Kos-Kudla B. 2013. Transforming growth factor beta1 (TGFbeta1) in physiology and pathology. Endokrynol Pol. 64(5):384–396. eng. doi:10.5603/EP.2013.0022.
- Kelley LA, Mezulis S, Yates CM, Wass MN, Sternberg MJ. 2015. The Phyre2 web portal for protein modeling, prediction and analysis. Nat Protoc. 10(6):845. doi:10.1038/nprot.2015.053.
- Khani M, Amani D, Taheripanah R, Sanadgol N, Feizollahzadeh S, Rahmani Z. 2015. Transforming growth factor beta-1 (TGF-β1) gene single nucleotide polymorphisms (SNPs) and susceptibility to pre-eclampsia in Iranian women: A case–control study. Pregn Hyper. 5(4):267–272. doi:10.1016/j.preghy.2015.01.002.
- Kingsley DM. 1994. The TGF-beta superfamily: new members, new receptors, and new genetic tests of function in different organisms. Genes Dev. 8(2):133–146. doi:10.1101/gad.8.2.133.
- Krausz C. 2011. Male infertility: pathogenesis and clinical diagnosis. Best Pract Res Clin Endocrinol Metab. 25(2):271–285. doi:10.1016/j.beem.2010.08.006.
- Krstic J, Santibanez JF. 2014. Transforming growth factor-beta and matrix metalloproteinases: functional interactions in tumor stroma-infiltrating myeloid cells. The Scientific World Journal. 20(3):514–528.
- Li Q. 2014. Transforming growth factor β signaling in uterine development and function. J Anim Sci Biotechnol. 5(1):52. doi:10.1186/2049-1891-5-52.
- Lichtman MK, Otero‐Vinas M, Falanga V. 2016. Transforming growth factor beta (TGF‐β) isoforms in wound healing and fibrosis. Wound Repair Regen. 24(2):215–222. doi:10.1111/wrr.12398.
- Magdoud K, Granados Herbepin V, Messaoudi S, Hizem S, Bouafia N, Almawi W, Mahjoub T, Touraine R. 2013. Genetic variation in TGFB1 gene and risk of idiopathic recurrent pregnancy loss. Mol Hum Reprod. 19(7):438–443. doi:10.1093/molehr/gat012.
- Martelossi GC, Paiva KT, Badaró SG, de Oliveira Brajão K. 2016. TGF-β1 functional polymorphisms: a review. Eur Cytokine Netw. 27(4):81–89.
- Massague J. 1990. The transforming growth factor-beta family. Annu Rev Cell Biol. 6(1):597–641. doi:10.1146/annurev.cb.06.110190.003121.
- McGuffin LJ, Bryson K, Jones DT. 2000. The PSIPRED protein structure prediction server. Bioinformatics. 16(4):404–405. doi:10.1093/bioinformatics/16.4.404.
- Meng XM, Nikolic-Paterson DJ, Lan HY. 2016. TGF-beta: the master regulator of fibrosis. Nat Rev Nephrol. 12(6):325–338. eng. doi:10.1038/nrneph.2016.48.
- Morikawa M, Derynck R, Miyazono K. 2016. TGF-beta and the TGF-beta family: context-dependent roles in cell and tissue physiology. Cold Spring Harb Perspect Biol. 8(5). eng. doi:10.1101/cshperspect.a021873.
- Nasiri H, Forouzandeh M, Rasaee M, Rahbarizadeh F. 2005. Modified salting‐out method: high‐yield, high‐quality genomic DNA extraction from whole blood using laundry detergent. J Clin Lab Anal. 19(6):229–232. doi:10.1002/jcla.20083.
- Ni N, Li Q. 2017. TGFbeta superfamily signaling and uterine decidualization. Reproductive Biolol and Endocrin. 15(1):84.
- Parsanezhad ME, Jahromi BN, Zare N, Keramati P, Khalili A, Parsa-Nezhad M. 2016. Epidemiology and etiology of infertility in Iran, systematic review and meta-analysis. Journal of Womens Health, Issues Care. 2(6):2–12.
- Poniatowski ŁA, Wojdasiewicz P, Gasik R, Szukiewicz D. 2015. Transforming growth factor Beta family: insight into the role of growth factors in regulation of fracture healing biology and potential clinical applications. Mediators Inflamm. 12(3):262–279.
- Poppe K, Velkeniers B. 2004. Female infertility and the thyroid. Best Pract Res Clin Endocrinol Metab. 18(2):153–165. doi:10.1016/j.beem.2004.03.004.
- Priyadarshi S, Ray CS, Panda KC, Desai A, Nayak SR, Biswal NC, Ramchander PV. 2013. Genetic association and gene expression profiles of TGFB1 and the contribution of TGFB1 to otosclerosis susceptibility. J Bone Miner Res. 28(12):2490–2497. doi:10.1002/jbmr.1991.
- Punab M, Poolamets O, Paju P, Vihljajev V, Pomm K, Ladva R, Korrovits P, Laan M. 2016. Causes of male infertility: a 9-year prospective monocentre study on 1737 patients with reduced total sperm counts. Hum Reprod. 32(1):18–31. doi:10.1093/humrep/dew284.
- Qiu T, Teng Y, Wang Y, Xu L. 2016. Adoptive transfer of transforming growth factor-β1-induced CD4+ CD25+ regulatory T cells prevents immune response-mediated spontaneous abortion. Reprod Fertil Dev. 28(11):1788–1797. doi:10.1071/RD14503.
- Randall LL, Hardy SJ. 1989. Unity in function in the absence of consensus in sequence: role of leader peptides in export. Science. 243(4895):1156–1159. eng. doi:10.1126/science.2646712.
- Robertson SA, Guerin LR, Moldenhauer LM, Hayball JD. 2009. Activating T regulatory cells for tolerance in early pregnancy - the contribution of seminal fluid. J Reprod Immunol. 83(1–2):109–116. eng. doi:10.1016/j.jri.2009.08.003.
- Robertson SA, Ingman WV, O’Leary S, Sharkey DJ, Tremellen KP. 2002. Transforming growth factor β—a mediator of immune deviation in seminal plasma. J Reprod Immunol. 57(1–2):109–128. doi:10.1016/S0165-0378(02)00015-3.
- Roupa Z, Polikandrioti M, Sotiropoulou P, Faros E, Koulouri A, Wozniak G, Gourni M. 2009. Causes of infertility in women at reproductive age. Health Sci J. 3:2.
- Roy A, Kucukural A, Zhang Y. 2010. I-TASSER: a unified platform for automated protein structure and function prediction. Nat Protoc. 5(4):725. doi:10.1038/nprot.2010.5.
- Sabarinathan R, Tafer H, Seemann SE, Hofacker IL, Stadler PF, Gorodkin J. 2013. The RNAsnp web server: predicting SNP effects on local RNA secondary structure. Nucleic Acids Res. 41(W1):W475–W479. doi:10.1093/nar/gkt291.
- Sachidanandam R, Weissman D, Schmidt SC, Kakol JM, Stein LD, Marth G, Sherry S, Mullikin JC, Mortimore BJ, Willey DL, et al. 2001. A map of human genome sequence variation containing 1.42 million single nucleotide polymorphisms. Nature. 409(6822):928–933. eng.
- Schäfer-Somi S. 2003. Cytokines during early pregnancy of mammals: a review. Anim Reprod Sci. 75(1–2):73–94. doi:10.1016/S0378-4320(02)00222-1.
- Taubenschuß E, Marton E, Mogg M, Frech B, Ehart L, Muin D, Schreiber M. 2013. The L10P polymorphism and serum levels of transforming growth factor β1 in human breast cancer. Int J Mol Sci. 14(8):15376–15385. doi:10.3390/ijms140815376.
- The role of growth factors and cytokines during implantation: endocrine and paracrine interactions. Semin Reprod Med. 2009. NIH Public Access.
- Traven E, Ogrinc A, Kunej T. 2017. Initiative for standardization of reporting genetics of male infertility. Syst Biol Reprod Med. 63(1):58–66.
- Wilczyński JR. 2005. Th1/Th2 cytokines balance—yin and yang of reproductive immunology. Eur J Obstetr Gynecol Rep Biol. 122(2):136–143. doi:10.1016/j.ejogrb.2005.03.008.
- Yates CM, Filippis I, Kelley LA, Sternberg MJ. 2014. SuSPect: enhanced prediction of single amino acid variant (SAV) phenotype using network features. J Mol Biol. 426(14):2692–2701. doi:10.1016/j.jmb.2014.04.026.
- Yoshimura A, Muto G. 2010. TGF-β function in immune suppression. Negative Co-Receptors and Ligands. Springer. 12(6):127–147.