ABSTRACT
Obesity, known to cause a systemic elevation in monocyte chemotactic protein-1 (MCP-1), adversely affects normal ovarian function. The aim of this study was to determine whether MCP-1 plays a role in ovarian dysfunction that is related to obesity induced by high-fat (HF) diet intake. Wild type (WT) C57BL/6J mice were fed either normal chow (NC) (Group 1, control group) or HF diet (Group 2). To assess whether MCP-1 is involved in HF-diet-induced ovarian dysfunction, MCP-1 knock-out mice were fed HF diet (Group 3). Body weight, body fat composition, number of oocytes collected following ovarian superovulation with gonadotropins, ovarian macrophage markers and expression of genes important in folliculogenesis and steroidogenesis were quantified in the 3 groups of animals. Animals in Group 2 gained significant body weight and body mass, produced the fewest number of oocytes following superovulation, and had significant alterations in ovarian genes involved in folliculogenesis and steroidogenesis as well as genes involved in inflammation. Although animals in Group 3 had the highest body weight and body fat composition, they produced similar number of oocytes compared to animals in Group 1 but had different ovarian gene expression compared to Group 2. These findings suggest that MCP-1 gene knockout could reverse some of the adverse effects of obesity induced by HF diet intake. Future studies assessing ovarian histology in MCP-1 knock out mouse model will confirm our findings. MCP-1 inhibition could represent a future therapeutic target to protect ovarian health from the adverse effects of HF diet ingestion.
Introduction
More than two-thirds of adult Americans are overweight or obese (Flegal et al. Citation2012; Fryar and Ogden Citation2014). The obesity epidemic, along with the ingestion of Western diet, that contains large amounts of fat, is associated with ovarian dysfunction that leads to anovulation (Grodstein et al. Citation1994), abnormal uterine bleeding, endometrial hyperplasia/cancer, and subfertility (Pasquali et al. Citation2007; Epplein et al. Citation2008). Regardless of anovulation, obesity is associated with ovarian dysfunction such as suboptimal corpus luteum function (Jain et al. Citation2007) and ovarian intrafollicular alterations at steroidogenic, metabolic, and inflammatory pathways (Robker et al. Citation2009). Additionally, it has been shown that obese women have lower ovarian reserve than normal weight women (Buyuk et al. Citation2011), as reflected by their low serum anti-Mullerian hormone (AMH) levels and diminished AMH gene expression in their granulosa cells (Merhi et al. Citation2013).
Obesity is a state of chronic low-grade inflammation (Weyer et al. Citation2001; Fonseca-Alaniz et al. Citation2007), which is thought to adversely impact ovarian function such as oocyte development and ovulation (Robker et al. Citation2009, Citation2011). It has been shown that obesity exerts an inflammatory effect in human granulosa cells and induces changes in the expression of genes involved in cell cycle division and cellular growth (Merhi et al. Citation2015). MCP-1 and its receptor C-C Chemokine receptor type 2 (CCR2) are present in the ovaries (Dahm-Kahler et al. Citation2009) where MCP-1 plays a physiologic role in normal ovulation by attracting monocytes from blood vessels into the ovaries (Dahm-Kahler et al. Citation2009). Human chorionic gonadotropin (hCG) has been shown to induce MCP-1 expression in rat ovaries by 18-fold (Wong et al. Citation2002), indicating that superovulation is associated with MCP-1 production. Systemic serum MCP-1 levels are elevated in obesity (Chatzigeorgiou et al. Citation2013; Gogebakan et al. Citation2015) potentially interfering with normal ovarian function. Although the MCP-1-related inflammatory actions of obesity are well characterized in adipose tissue (Chatzigeorgiou et al. Citation2013; Gogebakan et al. Citation2015), where metabolic signals originating from adipocytes activate MCP-1 related inflammatory responses resulting in macrophage accumulation (Gogebakan et al. Citation2015), their effects in the ovary are not fully elucidated.
Presently, there is a lack of targeted treatment for costly obesity-related ovarian dysfunction (Koning et al. Citation2010). Since weight loss is commonly challenging and often not sustainable (Wadden Citation1993; Stubbs et al. Citation2011), there is a need to understand the mechanisms underlying ovarian dysfunction due to high-fat (HF) diet-induced obesity. We have recently shown that MCP-1 is elevated in the serum and follicular fluid of obese women and is associated with poorer assisted reproductive technology outcomes (Buyuk et al. Citation2017). The objective of this study was to determine, using an MCP-1 knockout (KO) mouse model, whether MCP-1 plays a role in ovarian dysfunction due to HF diet-induced obesity.
Results and discussion
The baseline body weights were similar for mice in each group at 6 weeks of age (). Starting at 7 weeks of age, Group 2 and 3 started to have significantly higher body weight than Group 1 (p < 0.05; ). Interestingly, at 18 weeks of age, Group 3 started to have significantly higher body weight than Group 2 (p < 0.0001) (). Body fat composition of Groups 2 and 3 at 16 weeks of age was significantly higher than that of Group 1 (p < 0.0001) (). In response to superovulation, Group 2 produced significantly fewer oocytes compared to Group 1 (1.8 ± 0.5 vs. 6.7 ± 1.8, respectively; p = 0.03). Interestingly, despite gaining the most body weight and body fat, Group 3 had similar number of oocytes compared to Group 1 (6.2 ± 0.8 vs. 6.7 ± 1.8, respectively; p = 0.8) (). Despite gaining the most body weight, MCP-1 KO mice on HF had similar number of oocytes collected compared to NC group. These results suggest that HF diet-induced obesity causes a suboptimal response to gonadotropin stimulation as reflected by significant reduction in the number of oocytes collected in the HF diet group. Moreover, knocking down MCP-1 could be ovary-protective against the effects of HF diet since MCP-1 KO mice on HF diet had similar number of oocytes when compared to WT mice on NC. Although MCP-1 KO mice had similar weight at the onset of the study and ate the same diet as HF-diet mice, they gained more weight than WT animals. It is possible that these mice may have had higher food intake or had a slower metabolism when compared to WT mice; the mechanism of which remains to be determined. These results suggest that knocking down MCP-1 could be ovary-protective against the effects of HF diet. These results are in accordance with our previous study that showed that elevated serum and follicular fluid MCP-1 levels are associated with poorer assisted reproductive technology outcomes in women undergoing fertility treatments (Buyuk et al. Citation2017).
Figure 1. Body weight and body fat composition.
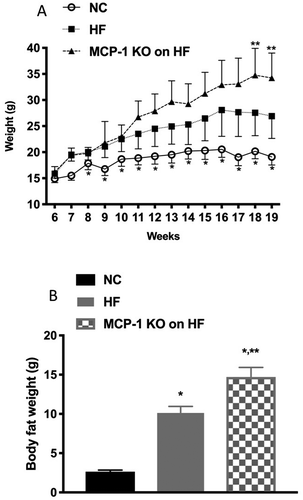
Figure 2. Oocyte collection following superovulation.
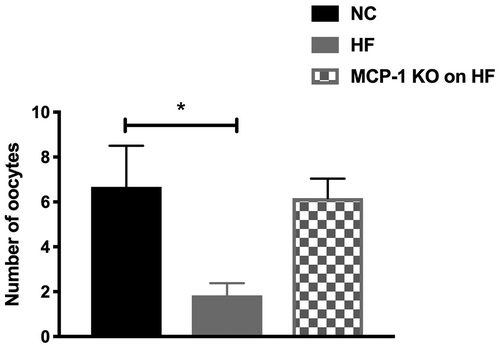
The mechanism by which obesity and HF diet causes ovarian dysfunction is not well understood. Obesity is a state of chronic low-grade inflammation thus elevated inflammatory markers may adversely impact oocyte development resulting in poor ovarian function (Robker et al. Citation2009, Citation2011). We have previously shown that MCP-1 may play a role in the pathogenesis of obesity-induced ovarian dysfunction (Buyuk et al. Citation2017). MCP-1 is physiologically important because it regulates the migration and infiltration of monocytes, basophils, T-lymphocytes, and natural killer cells (Deshmane et al. Citation2009). Since both MCP-1 and its receptor CCR-2, are expressed in the ovaries (Zhou et al. Citation2004), MCP-1 may thus play a role in ovarian dysfunction due to HF diet-induced obesity. The inflammatory milieu created by increased macrophages may interfere with folliculogenesis and steroidogenesis that may lead to decreased number of oocytes collected following superovulation, as seen in WT mice fed HF. To further elucidate the pathogenesis of HF diet and obesity-induced ovarian dysfunction, we examined the expression of ovarian mouse macrophage markers. Mouse macrophage marker F4/80 (universal macrophage marker) and Cd11c (marker for pro-inflammatory M1 macrophages) mRNA expression levels were significantly higher in Group 2 (p = 0.03) and Group 3 (p = 0.004), compared to Group 1 (). Compared to Group 2, Group 3 had significantly higher F4/80 mRNA expression levels and significantly lower Cd11c mRNA expression levels (p < 0.05) (). Cd206 mRNA expression (marker for anti-inflammatory M2 macrophages) showed no significant difference among all groups (). These findings suggest that the proinflammatory macrophage markers are increased in the ovaries following HF diet-induced obesity. However, the fact that the MCP-1 KO mice on HF had similar number of oocytes collected as the WT mice on NC despite expression of increased macrophage markers in their ovaries suggests that this may not be the only mechanism for the decreased response to superovulation. We speculate that MCP-1 has functions, other than macrophage recruitment, that may be detrimental to ovarian function.
Figure 3. Whole ovary mRNA quantification for macrophage markers.
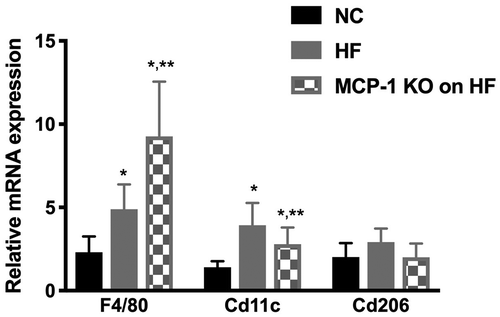
We also found that Fshr and B-fgf mRNA levels were significantly higher in Group 3 compared to Group 1 (p < 0.05) (). There was no difference in mRNA levels in the remaining genes (Foxl2, Gdf-9, Bmp-15, Amh, Amhr, Gja1, Lhcgr, and Ereg (p > 0.05) among all groups (data not shown). Star mRNA expression levels were significantly lower in Group 2 compared to Group 1 (p < 0.05) (). There was no difference in mRNA expression levels of Scc, Cyp17a1, Cyp19a1, or Hsd3b1 among all 3 groups of mice (p > 0.05) (data not shown). In order to determine whether MCP-1 alters the effect of HF diet on the response to superovulation, we compared mRNA levels for genes involved in folliculogenesis and steroidogenesis within the same group of mice before superovulation and after superovulation. As seen in , in Group 1, compared to mice that did not receive superovulation, there was no change in response to superovulation for genes of early folliculogenesis such as Gdf-9, Bmp-15, Amh, Amhr, and Foxl2. Group 2 had significantly lower expression of Gdf-9 mRNA levels (p = 0.03) and higher expression of Foxl2 mRNA (p = 0.03) in response to superovulation but no change in Bmp-15, Amh, or Amhr mRNA levels (p > 0.05). Group 3 had significantly lower mRNA expression levels of Bmp-15 mRNA (p = 0.02) in response to superovulation, but no change in Gdf-9, Amh, Amhr, and Foxl2 mRNA (p > 0.05). As seen in , in Group 1, Gja1 (p = 0.02), Fshr (p = 0.01), and B-fgf (p = 0.02) mRNA expression levels were significantly higher following superovulation. In Group 2, there was no change in mRNA expression levels of Gja1, Fshr, and B-fgf in response to superovulation (p > 0.05). In Group 3, Gja1 mRNA expression levels were significantly higher following superovulation (p = 0.02), but there was no change in Fshr and B-fgf mRNA expression levels. Lhcgr and Ereg mRNA expression levels were significantly higher following superovulation in Group 1 (p = 0.04 and p = 0.01, respectively), Group 2 (p = 0.01 and p = 0.01, respectively), and Group 3 (p = 0.03 and p = 0.03, respectively) (data not shown). As seen in , Group 1 had significantly higher mRNA expression levels of Star (p = 0.01), Scc (p = 0.01), Cyp19a1 (p = 0.01), Hsd3b1 (p = 0.04), and Cyp17a1 (p = 0.01) following superovulation. Group 2 had higher mRNA expression levels of Star (p = 0.01) and Cyp19a1 (p = 0.01) but no change in Scc, Hsd3b1, or Cyp17a1 (p > 0.05). Group 3 had significantly higher mRNA expression levels of Star (p = 0.03) and Scc (p = 0.03) following superovulation, but did not show a change in Cyp19a1, Hsd3b1, or Cyp17a1 following superovulation. In mice fed a HF diet, consistent with a lower number of oocytes produced following superovulation, we observed a decrease in ovarian expression of genes important in late folliculogenesis and steroidogenesis. More importantly, our results showed that the expression of genes (folliculogenesis; Gja1, Fshr, B-fgf; and steroidogenesis; Scc, Hsd3b1, Cyp17a1) did not increase following superovulation, unlike what was observed in mice on NC. Collectively these findings demonstrate that HF diet induced-obesity interferes with the expression of genes important in late folliculogenesis and steroidogenesis, which may explain the low number of oocytes collected from HF fed mice. These results may also suggest that HF diet induced-obesity might impair ovarian function, independent of the hypothalamic pituitary axis, as evidenced by decreased number of oocytes following superovulation of WT mice on HF compared to WT mice on NC.
Figure 4. Whole ovary mRNA quantification for genes involved in folliculogenesis and steroidogenesis.
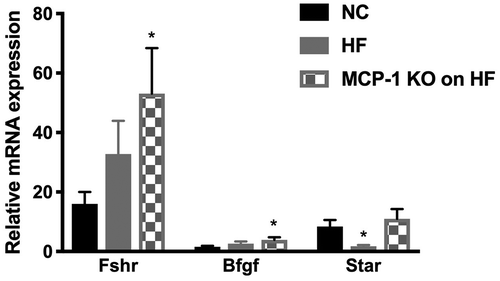
Figure 5. Whole ovary mRNA quantification for genes involved in folliculogenesis and steroidogenesis before and after superovulation.
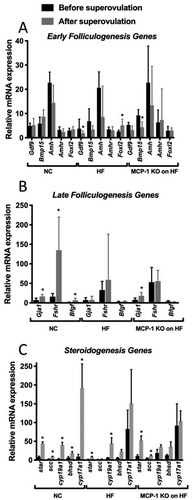
In summary, in this study we used MCP-1 KO mouse model to explore the role of MCP-1 on ovarian dysfunction due to HF diet-induced obesity. Our results showed that animals on HF diet gained significant body weight and body mass, produced the fewest number of oocytes following superovulation, and had alterations in ovarian genes involved in folliculogenesis and steroidogenesis as well as genes involved in inflammation. Although MCP-1 KO mice on HF diet had the highest body weight and body fat composition, they produced similar number of oocytes compared to animals in the control group and had partial ‘normalization’ of ovarian gene expression. In conclusion, our study showed that HF diet-induced obesity has adverse effects on ovarian function. This ovarian dysfunction seems to be associated with increased expression of common and pro-inflammatory macrophage markers in the ovary. Moreover, HF diet-induced obesity seems to be associated with a blunt response of genes responsible for folliculogenesis and steroidogenesis to ovarian superovulation. Finally, knocking out MCP-1 partially reversed the deleterious effects of HF diet-induced obesity on ovarian function. Whether MCP-1 inhibition represents a future therapeutic target to improve ovarian health in obese women who ingest large amount of HF diet remains to be determined.
Materials and methods
Experimental animals and diet groups
Female C57BL/6J wild type (WT) and C57BL/6J MCP-1 knockout (KO) mice were obtained from Jackson Laboratory (Bar Harbor, ME) at 5 weeks of age. They were housed five animals per cage and maintained on a 14-h light, 10-h dark cycle with ad libitum access to chow and water. After one week of acclimation (at 6 weeks of age), WT mice were given either a normal chow (NC) diet (PicoLab® Mouse Diet #5058, LabDiet, St. Louis, MO; 9% fat, 20% protein, 53% carbohydrate, 3.59 kcal/g) (n = 12, control groups; Group 1) or high-fat (HF) diet (#F3282, Bioserve, Frenchtown, NJ; 36% fat, 20.5% protein, 35.7% carbohydrate, 5.49 kcal/g) (n = 12) for 13 weeks (Group 2). To determine whether HF diet adversely impacts ovarian function via MCP-1, MCP-1 KO mice (n = 12) were placed on HF diet for 13 weeks (Group 3). Since MCP-1 KO mice on NC are fertile (Lu et al. Citation1998) we did not include a group of MCP-1 KO mice on NC. Thirteen weeks of feeding was chosen because it was shown that mice develop significant obesity (van der Heijden et al. Citation2015) and metabolic changes (Jiang et al. Citation2005) after 12 weeks of HF diet feeding.
Body weight and body composition
For each mouse, body weight was measured once weekly at 9 AM starting from 6th week of age until sacrifice. The amount of body fat and lean mass were determined at 16 weeks of age using an Echo Magnetic Resonance Imaging (EchoMRI) spectroscopy instrument (Echo Medical Systems, Houston, Texas) (Taicher et al. Citation2003).
Oophorectomy for RT-PCR and oocyte collection following superovulation
After 13 weeks of exposure to the corresponding diet (i.e. 19 weeks of age), half of the mice in each group (n = 6) were sacrificed without superovulation and the other half of mice were superovulated with gonadotropins before sacrifice (n = 6). Superovulation was performed by the administration of intra-peritoneal injection of pregnant mare’s serum gonadotropin (PMSG) (Sigma-Aldrich, St. Louis, MO), followed by hCG (National Hormone & Peptide Program, Torrance, CA) 46 h later. The dosages of PMSG and hCG used were calculated according to body weight (0.25 IU/g) as previously reported (Tortoriello et al. Citation2004) in order to avoid any dilution effect. Twelve to 14 h after the hCG injection, mice were sacrificed by cervical dislocation and their ovaries were immediately harvested. Their oviducts were removed and manually dissected for oocyte collection under a stage microscope by a trained embryologist who was blinded to groups.
RNA extraction, reverse transcription, and real-time polymerase chain reaction (RT-PCR)
At the time of sacrifice, the ovaries were snap frozen in liquid nitrogen and stored in −80°C. Frozen ovaries were homogenized, and RNA extraction was performed using Trizol reagent (Invitrogen, Carlsbad, CA), chloroform extraction, and Qiagen’s RNeasy mini kit (Waltham, MA) according to manufacturer’s instructions. Total RNA concentration was determined using ND-2000 spectrophotometer. RNA quality was confirmed by electrophoresis and optical density (OD) 260/280 > 1.8. First-strand cDNA was synthesized from total RNA using reverse transcriptase (Invitrogen, Carlsbad, CA). mRNA levels were quantified using FastStart SYBR Green Master (Roche, Indianapolis, IN) and ABI Prism 7700 (Applied Biosystems, Carlsbad, CA). Primers were synthesized by Fisher (Pittsburgh, PA) and are shown in . The following genes were assessed:
Table 1. Primer sequences used for RT-PCR.
1) Genes for macrophage markers: F4/80 (Emr1/Egf-like module-containing mucin-like hormone receptor-like 1), Cd206 (Mrc1/Mannose receptor C type 1), Cd11c (Itgax/Integrin, alpha X [complement component 3 receptor 4 subunit]).
2) Genes involved in early folliculogenesis: Gdf9 (growth differentiation factor 9), Bmp15 (bone morphogenetic protein 15), Amh (anti-Mullerian hormone), Amhr (amh receptor), Foxl2 (Forkhead box L2), Bfgf (basic fibroblast growth factor).
3) Genes involved in late folliculogenesis: Gja1 (Gap junction protein alpha 1), Fshr (Follicle stimulating hormone receptor), Lhcgr (Luteinizing hormone/choriogonadotropin receptor), and Ereg (Epiregulin).
4) Genes involved in steroidogenesis: Star (steroidogenic acute regulatory protein), Scc (side chain cleavage enzyme), Cyp19a1 (aromatase), Hsd3b1 (3 beta-hydroxysteroid dehydrogenase), Cyp17a1 (17 alpha-hydroxylase).
Statistical analysis
Statistical analyses were performed using STATA software version 13.1 (StataCorp). For normally distributed data, Student t-test and analysis of variance (ANOVA) with Bonferroni post hoc were used. For non-normally distributed data, Mann-Whitney U and Kruskal-Wallis tests were used. The mRNA expression levels for each gene were calculated relative to the housekeeping gene GAPDH using the 2−ΔΔCT method. Data were reported as mean ± standard error of the mean (SEM) and p-value<0.05 was considered statistically significant.
Ethics approval
All protocols were conducted in accordance with the National Institutes of Health guide for the care and use of laboratory animals and were approved by the Institutional Animal Care and Use Committee of Albert Einstein College of Medicine.
Authors contribution
Designed the study, performed experiments, and wrote major parts of the manuscript, Figures and Tables: O.A, K.T, Z.M, E.B, L.W Helped with study design, performed some of the experiments, and wrote parts of the manuscript: Y.S, X.Q.D, D.S.B, S.J Helped with study design, supervised all the experiments, provided feedback on the progress of the study, and wrote parts of the manuscript: M.J.C.
Supplemental_Table_1.docx
Download MS Word (14.2 KB)Disclosure statement
The authors declare that they have no conflict of interest.
Supplementary material
Supplemental data for this article can be accessed here.
Additional information
Funding
References
- Buyuk E, Asemota OA, Merhi Z, Charron MJ, Berger DS, Zapantis A, Jindal SK. 2017. Serum and follicular fluid monocyte chemotactic protein-1 levels are elevated in obese women and are associated with poorer clinical pregnancy rate after in vitro fertilization: a pilot study. Fertil Steril. 107(3):632–640. doi:10.1016/j.fertnstert.2016.12.023.
- Buyuk E, Seifer DB, Illions E, Grazi RV, Lieman H. 2011. Elevated body mass index is associated with lower serum anti-mullerian hormone levels in infertile women with diminished ovarian reserve but not with normal ovarian reserve. Fertil Steril. 95(7):2364–2368. doi:10.1016/j.fertnstert.2011.03.081.
- Chatzigeorgiou A, Kandaraki E, Piperi C, Livadas S, Papavassiliou AG, Koutsilieris M, Papalois A, Diamanti-Kandarakis E. 2013. Dietary glycotoxins affect scavenger receptor expression and the hormonal profile of female rats. J Endocrinol. 218(3):331–337. doi:10.1530/JOE-13-0175.
- Dahm-Kahler P, Ghahremani M, Lind AK, Sundfeldt K, Brannstrom M. 2009. Monocyte chemotactic protein-1 (MCP-1), its receptor, and macrophages in the perifollicular stroma during the human ovulatory process. Fertil Steril. 91(1):231–239. doi:10.1016/j.fertnstert.2007.07.1330.
- Deshmane SL, Kremlev S, Amini S, Sawaya BE. 2009. Monocyte chemoattractant protein-1 (MCP-1): an overview. J Interferon Cytokine Res. 29(6):313–326. doi:10.1089/jir.2008.0027.
- Epplein M, Reed SD, Voigt LF, Newton KM, Holt VL, Weiss NS. 2008. Risk of complex and atypical endometrial hyperplasia in relation to anthropometric measures and reproductive history. Am J Epidemiol. 168(6):563–570. doi:10.1093/aje/kwn168.
- Flegal KM, Carroll MD, Kit BK, Ogden CL. 2012. Prevalence of obesity and trends in the distribution of body mass index among US adults, 1999-2010. JAMA. 307(5):491–497. doi:10.1001/jama.2012.39.
- Fonseca-Alaniz MH, Takada J, Alonso-Vale MI, Lima FB. 2007. Adipose tissue as an endocrine organ: from theory to practice. J Pediatr. 83(5 Suppl):S192–203. doi:10.1590/S0021-75572007000700011.
- Fryar CDCM, Ogden CL 2014. Prevalence of overweight, obesity, and extreme obesity among adults: United States, 1960–1962 through 2011–2012. National Center for Health Statistics. http://wwwcdcgov/nchs/data/hestat/obesity_adult_11_12/obesity_adult_11_12pdf.
- Gogebakan O, Osterhoff MA, Schuler R, Pivovarova O, Kruse M, Seltmann AC, Mosig AS, Rudovich N, Nauck M, Pfeiffer AF. 2015. GIP increases adipose tissue expression and blood levels of MCP-1 in humans and links high energy diets to inflammation: a randomised trial. Diabetologia. 58(8):1759–1768. doi:10.1007/s00125-015-3618-4.
- Grodstein F, Goldman MB, Cramer DW. 1994. Body mass index and ovulatory infertility. Epidemiology. 5(2):247–250. doi:10.1097/00001648-199403000-00016.
- Jain A, Polotsky AJ, Rochester D, Berga SL, Loucks T, Zeitlian G, Gibbs K, Polotsky HN, Feng S, Isaac B, et al. 2007. Pulsatile luteinizing hormone amplitude and progesterone metabolite excretion are reduced in obese women. J Clin Endocrinol Metab. 92(7):2468–2473. doi:10.1210/jc.2006-2274.
- Jiang T, Wang Z, Proctor G, Moskowitz S, Liebman SE, Rogers T, Lucia MS, Li J, Levi M. 2005. Diet-induced obesity in C57BL/6J mice causes increased renal lipid accumulation and glomerulosclerosis via a sterol regulatory element-binding protein-1c-dependent pathway. J Biol Chem. 280(37):32317–32325. doi:10.1074/jbc.M500801200.
- Koning AM, Kuchenbecker WK, Groen H, Hoek A, Land JA, Khan KS, Mol BW. 2010. Economic consequences of overweight and obesity in infertility: a framework for evaluating the costs and outcomes of fertility care. Human Reprod Update. 16(3):246–254. doi:10.1093/humupd/dmp053.
- Lu B, Rutledge BJ, Gu L, Fiorillo J, Lukacs NW, Kunkel SL, North R, Gerard C, Rollins BJ. 1998. Abnormalities in monocyte recruitment and cytokine expression in monocyte chemoattractant protein 1-deficient mice. J Exp Med. 187(4):601–608. doi:10.1084/jem.187.4.601.
- Merhi Z, Buyuk E, Berger DS, Zapantis A, Israel DD, Chua S Jr., Jindal S. 2013. Leptin suppresses anti-Mullerian hormone gene expression through the JAK2/STAT3 pathway in luteinized granulosa cells of women undergoing IVF. Hum Reprod. 28(6):1661–1669. doi:10.1093/humrep/det072.
- Merhi Z, Polotsky AJ, Bradford AP, Buyuk E, Chosich J, Phang T, Jindal S, Santoro N. 2015. Adiposity alters genes important in inflammation and cell cycle division in human cumulus granulosa cell. Reprod Sci. 22(10):1220–1228. doi:10.1177/1933719115572484.
- Pasquali R, Patton L, Gambineri A. 2007. Obesity and infertility. Curr Opin Endocrinol Diabetes Obes. 14(6):482–487. doi:10.1097/MED.0b013e3282f1d6cb.
- Robker RL, Akison LK, Bennett BD, Thrupp PN, Chura LR, Russell DL, Lane M, Norman RJ. 2009. Obese women exhibit differences in ovarian metabolites, hormones, and gene expression compared with moderate-weight women. J Clin Endocrinol Metab. 94(5):1533–1540. doi:10.1210/jc.2008-2648.
- Robker RL, Wu LL, Yang X. 2011. Inflammatory pathways linking obesity and ovarian dysfunction. J Reprod Immunol. 88(2):142–148. doi:10.1016/j.jri.2011.01.008.
- Stubbs J, Whybrow S, Teixeira P, Blundell J, Lawton C, Westenhoefer J, Engel D, Shepherd R, McConnon A, Gilbert P, et al. 2011. Problems in identifying predictors and correlates of weight loss and maintenance: implications for weight control therapies based on behaviour change. Obes Rev. 12(9):688–708. doi:10.1111/j.1467-789X.2011.00883.x.
- Taicher GZ, Tinsley FC, Reiderman A, Heiman ML. 2003. Quantitative magnetic resonance (QMR) method for bone and whole-body-composition analysis. Anal Bioanal Chem. 377(6):990–1002. doi:10.1007/s00216-003-2224-3.
- Tortoriello DV, McMinn J, Chua SC. 2004. Dietary-induced obesity and hypothalamic infertility in female DBA/2J mice. Endocrinology. 145(3):1238–1247. doi:10.1210/en.2003-1406.
- van der Heijden RA, Sheedfar F, Morrison MC, Hommelberg PP, Kor D, Kloosterhuis NJ, Gruben N, Youssef SA, de Bruin A, Hofker MH, et al. 2015. High-fat diet induced obesity primes inflammation in adipose tissue prior to liver in C57BL/6j mice. Aging. 7(4):256–268. doi:10.18632/aging.100738.
- Wadden TA. 1993. Treatment of obesity by moderate and severe caloric restriction. Results of clinical research trials. Ann Intern Med. 119(7 Pt 2):688–693. doi:10.7326/0003-4819-119-7_Part_2-199310011-00012.
- Weyer C, Funahashi T, Tanaka S, Hotta K, Matsuzawa Y, Pratley RE, Tataranni PA. 2001. Hypoadiponectinemia in obesity and type 2 diabetes: close association with insulin resistance and hyperinsulinemia. J Clin Endocrinol Metab. 86(5):1930–1935. doi:10.1210/jcem.86.5.7463.
- Wong KH, Negishi H, Adashi EY. 2002. Expression, hormonal regulation, and cyclic variation of chemokines in the rat ovary: key determinants of the intraovarian residence of representatives of the white blood cell series. Endocrinology. 143(3):784–791. doi:10.1210/endo.143.3.8699.
- Zhou C, Borillo J, Wu J, Torres L, Lou YH. 2004. Ovarian expression of chemokines and their receptors. J Reprod Immunol. 63(1):1–9. doi:10.1016/j.jri.2004.03.002.