ABSTRACT
One of the major causes of defective sperm function is oxidative stress, which limits the fertilizing potential of these cells as the result of collateral damage to proteins and lipids in the sperm plasma membrane. On this point, a derangement of both generation and neutralization of reactive oxygen species (ROS) is a recognized cause of male infertility. Antioxidant protection in sperm has been widely investigated, as well as the sperm composition of fatty acids (FA), which represents the preferred substrate for ROS, most frequently linked to the disease-related infertility. Isoprostanes are compounds derived from free radical-mediated oxidation of FAs. As such, they are considered an index of lipid oxidative damage and lipid mediators. This article discusses the role of isoprostanes as relevant factors both to sperm FA composition and sperm membrane integrity. Additionally, isoprostane’s influence on sperm quality is reviewed. With reference to male reproductive dysfunction, increasing evidence indicates isoprostanes, detectable in biological fluids or sperm membrane, as the specific index of 1) exposure to chemical etiological agents, 2) oxidative damage, 3) reduced antioxidant response, and 4) sperm immaturity.
Abbreviations
OS: oxidative stress; ROS: reactive oxygen species; PUFAs: polyunsaturated fatty acids; ARA: arachidonic acid, F2-IsoPs; F2-isoprostanes, PLA2: phospholipase A2; NADPH: nicotinamide adenine dinucleotide phosphate; IVF: in vitro fertilization
Introduction
Male infertility is related to a wide variety of etiological factors ranging from genetics to infections. Oxidative stress (OS) is a condition in which basal-free radical levels are transiently or chronically enhanced and affect both physiological cellular metabolism and cellular constituents (Lushchak Citation2014). OS represents one of the main causes of infertility, considering that a large number of infertile males display abnormal-free radical (reactive oxygen species, ROS) levels in semen (Agarwal et al. Citation2014). When present at physiological levels, ROS are not harmful, and their presence is essential for sperm fertilizing capacity (Aitken Citation2017). High levels of ROS provide a common underlying etiology of male infertility and recurrent pregnancy loss (Bisht et al. Citation2017). The measurement of oxidative damage to biomolecules may represent a new tool to evaluate the impact of OS on sperm fertilization potential.
There is a growing interest in the new markers that could be used in infertility diagnosis, also in combination to the evaluation of sperm concentration, morphology, and motility. For example, the enzymes related to ROS scavenging/decomposition seem appropriate candidates as new indices of human fertility (Agarwal et al. Citation2005). Recently, Rubio-Riquelme and colleagues have also suggested that catalase, the enzyme able to decompose hydrogen peroxide, one of the ROS related to OS, could play an important role in male fertility and become a good target for male infertility diagnosis and monitoring (Rubio-Riquelme et al. Citation2020).
In particular, ROS can cause lipid peroxidation in cell membranes. Aldehydes, such as malondialdehyde and 4-hydroxynonenenal, conjugated diene compounds and isoprostanoids (a class of prostanoid isomers) are the secondary products of the oxidative degradation of lipids (lipid peroxidation) and they have been investigated as a seminal marker (Collodel et al. Citation2015; Moazamian et al. Citation2015).
It is known that isoprostanoids derive from free radical-induced peroxidation of polyunsaturated fatty acids (PUFAs) esterified in membrane phospholipids. Isoprostanoids derived from different PUFAs, including arachidonic acid (ARA), eicosapentaenoic acid, docosahexanenoic acid and adrenic acid have been identified (Vigor et al. Citation2014). The relevance of isoprostanoids in indicating human disease status related to OS has been largely demonstrated (Milne et al. Citation2015; Galano et al. Citation2017; Signorini et al. Citation2018).
In male infertility, the evidence for OS and F2-isoprostanes (F2-IsoPs), isoprostanoids, which are stereoisomers of PGF2α generated by the free radical-induced peroxidation of ARA, is here reviewed. The present review examines the evidence of a specific link between isoprostanes and male infertility, to indicate a new and additional point of investigation in the pathogenesis of male infertility.
Isoprostanes are an index of oxidative stress
In pathophysiological processes, OS is considered as the result of the imbalance between oxidants (i.e., ROS) and antioxidants leading to the molecular damage of proteins, lipids, and nucleic acids (Phaniendra et al. Citation2015; Sies et al. Citation2017; Gutteridge and Halliwell Citation2018; ).
Table 1. Oxidative damages to the molecular targets of reactive oxygen species.
Among the biological targets for ROS, PUFAs are particularly susceptible to oxidation due to the presence of double bonds in their structure. Thus, lipid peroxidation is the commonly used definition to indicate oxidative damage to PUFAs (Halliwell and Gutteridge Citation1984) and can be described as the process by which oxidants, such as free radicals, attack lipids containing carbon-carbon double bond(s).
Isoprostanoids, which are prostaglandin isomers, are end-products of PUFA lipid peroxidation. Unlike enzymatically produced PUFA metabolites (i.e., prostaglandins), isoprostanes are formed in situ, within the membrane, and released through hydrolysis via phospholipase A2 (PLA2, Morrow et al. Citation1992). Through the interaction of radical species, PUFAs generate non-enzymatic-oxygenated metabolites, which are classified according to the chemical structure. Accordingly, F2-IsoPs are compounds (four regioisomer classes, 64 isomeric compounds) structurally similar to F2α-prostaglandins and originate from the ARA peroxidation (Milne et al. Citation2015, , ).
Figure 1. Chemical structures of arachidonic acid and F2-isoprostane series. Isoprostanes are named based upon the structure of the functional groups on the cyclopentane ring in a manner analogous to that of prostaglandins. F2-isoprostanes (F2-IsoPs) are F2-prostaglandin-like compounds formed from the free radical-catalyzed peroxidation of arachidonic acid (ARA). Based on the mechanism of formation, four F2-IsoP series are generated. The four F2-IsoP series are named (5, 8, 12, and 15 series) according to the carbon number on which the side chain hydroxyl group is attached, carboxyl carbon being 1. In the figure, chemical structure of ARA and F2-IsoP series are shown.
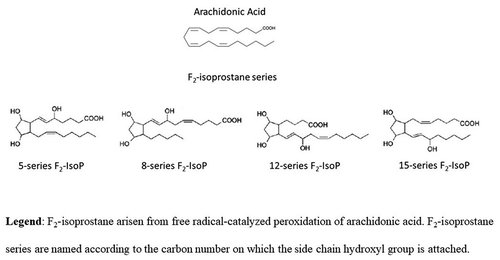
Figure 2. Synthesis of F2-isoprostanes from arachidonic acid esterified in membrane phospholipids. In contrast to cyclooxygenase-derived prostaglandin that are generated from free arachidonic acid (ARA), F2-isoprostanes (F2-IsoPs) are initially formed from the oxidation (free-radical-induced peroxidation) of ARA esterified in membrane phospholipids (in the figure, membrane phospholipid bilayer with esterified ARA and F2-IsoPs are shown). F2-IsoPs can then be released from the phospholipid backbone as free fatty acids (free F2-IsoPs in the figure) by phospholipase action (Phospholipase A2 activity, in the figure).
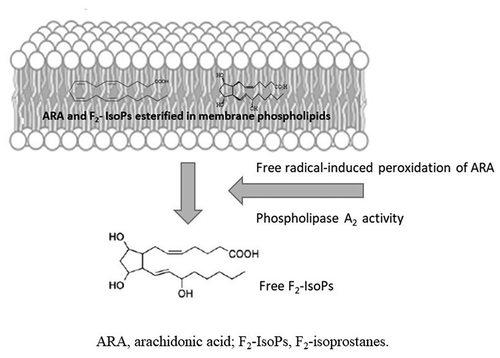
The oxidative modification of ARA would not undergo an enzymatic action by cyclooxygenase, as for the generation of inflammatory mediators (Morrow et al. Citation1992, Citation1996; Morrow and Roberts Citation1997; Praticò Citation1999; Basu Citation2007). Therefore, the consequences of F2-IsoP formation depend on the balance between two phenomena: 1) the production of F2-IsoPs esterified to FAs, and 2) the release of F2-IsoPs into biological fluids in free form (Morrow et al. Citation1992; Morrow and Roberts Citation1997).
F2-IsoPs are advantageous over other markers of lipid peroxidation, such as lipid hydroperoxides and aldehydes, due to their in vivo and in vitro stability. Consequently, F2-IsoPs can be easily detected both in plasma and urine (Roberts and Morrow Citation2002; Fam and Morrow Citation2003; Basu Citation2004), and the assessment of F2-IsoP levels in biological fluids allows to accurately ascertain lipid oxidative damages, both in vivo and ex vivo conditions (Morrow and Roberts Citation1997). Elevated plasma and/or urinary levels of F2-IsoPs have been found in many human diseases, related to different apparatuses and etiological causes, as well as in many models of experimental medicine (Milne et al. Citation2005, Citation2015). Additionally, according to the pathway of F2-IsoP formation esterified F2-IsoPs can be determined in the cell membranes of biological tissue (liver, myocardium, lung, brain, kidney, muscle, and testicular tissues). Thus, F2-IsoPs are currently considered as the best available markers of lipid peroxidation and can be used to evaluate oxidative status in many human pathologies (Milne et al. Citation2015). With regard to the evaluation of isoprostanes to assess the involvement of lipid peroxidation in male infertility, it has been reported that F2-IsoPs are detectable both in sperm membrane and seminal plasma (Collodel et al. Citation2018, Citation2020b; Moretti et al. Citation2019; ).
Figure 3. F2-IsoP formation is valuable in both spermatozoa and seminal plasma. Upon semen fractionation (by centrifugation) into its seminal plasma and spermatozoa, F2-isoprostanes (F2-IsoPs) levels were determined, as free F2-IsoPs and esterified F2-IsoPs, respectively. According to the mechanism shown in and the text, in seminal plasma free F2-IsoPs can be released from the phospholipid backbone of sperm (or other different cell) membrane; in spermatozoa, F2-IsoPs can be detected as still esterified to phospholipid.
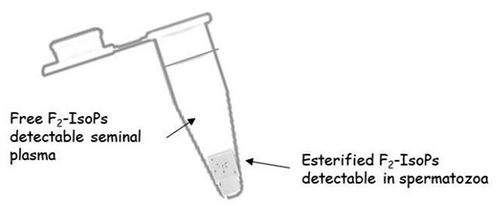
The relevance of F2-IsoPs is not limited to their reliability in assessing the extent of OS, but it is also linked to their biological activity, being mediators of oxidative damage (Milne et al. Citation2015). In particular, it has been shown that 8-iso-PGF2α (also known as 15-F2 t-IsoP; Taber et al. Citation1997), the most represented isomer for F2-IsoP measurement (Milne et al. Citation2015), has a vasoconstrictive action that is exercised on smooth muscle cells by the binding to specific receptors, distinct from the thromboxane ones (Takahashi et al. Citation1992; Comporti et al. Citation2008). In addition, it appears that F2-IsoPs released during oxidative damage induce endothelial cell alterations by stimulating the expression of the gene coding for endothelin-1 (Yura et al. Citation1999).
A report of the nationwide Biomarkers of Oxidative Stress Study shows that the measurement of isoprostanes is one of the potential candidates for general markers of oxidative stress (Kadiiska et al. Citation2005). Currently, a variety of analytical approaches have been developed for the quantification of F2-IsoPs. Mainly, F2-IsoPs are tested by liquid chromatography-tandem mass spectrometry (LC-MS/MS; Zhang et al. Citation2010; Casetta et al. Citation2012; Medina et al. Citation2012), gas chromatography/negative-ion chemical ionization tandem mass spectrometry (GC/NICI-MS/MS; Morrow and Roberts Citation1991; Nourooz-Zadeh Citation1999; Liu et al. Citation2009), radioimmunoassay (Basu Citation1998) and ELISA (Sasaki et al. Citation2002). A protocol has been optimized and validated to provide the best sensitivity and selectivity for the quantification of F2-IsoPs from a variety of biological sources (Liu et al. Citation2009). Immunoassays have helped in expanding the research in this area because of their low cost and relative ease of use (Milne et al. Citation2007).
ROS and oxidative stress have relevance to sperm quality
The balance between pro-oxidant and antioxidant levels is very essential for regulating spermatogenesis, testis function and sperm maturation. Minimal quantities of ROS are free to act modulating physiological functions of spermatozoa, such as capacitation, acrosomal reaction and the fusion process to oocytes (Baskaran et al. Citation2020). Seminal leukocytes and morphologically abnormal spermatozoa have been indicated as the main sources of ROS in seminal fluid (Chatterjee and Talwar Citation2019). Leukocytes activated in response to various stimuli, including inflammation and infection (Pasqualotto et al. Citation2000), can produce quantities of ROS 1000 times greater than the inactivated ones (Plante et al. Citation1994; de Lamirande and Gangnon Citation1995). In turn, spermatozoa can generate ROS through: i) nicotinamide adenine dinucleotide-phosphate (NADPH) oxidase system in sperm plasma membrane and ii) the activity of the oxide reductase NADH-dependent in mitochondria, that has been indicated as the main source of ROS in sperm with regard to infertile men (Amaral et al. Citation2013). Overproduction of ROS and oxidative damage to the sperm cells have been acknowledged as the leading causes of reduced fertility potential in males (El-Tohamy Citation2012). Elevated levels of ROS are detected in up to 30–80% of men with male infertility (Agarwal and Allamaneni Citation2011).
ROS production changes in spermatozoa at different stages of maturation and during sperm migration from the seminiferous tubules to the epididymis, mature spermatozoa may be damaged in case of ROS production in immature sperm (Gil-Guzman et al. Citation2001). It is known that an excess residual cytoplasm in sperm contains elevated levels of cytoplasm enzymes, producing pathological amounts of ROS. High levels of ROS may later result in OS. Excess residual cytoplasm may be associated with sperm immaturity and it affects sperm parameters, leading to male infertility (Gomez et al. Citation1996).
Increased levels of ROS have been correlated with a reduction in sperm motility (Agarwal and Saleh Citation2002; Desai et al. Citation2009). Pathological levels of ROS are known to cause apoptosis of spermatozoa, resulting in cell death and reduced sperm count (Agarwal et al. Citation2014). Nevertheless, sperm membrane fluidity, flexibility, fusion, fission, and curvature largely depend on the FA composition of phospholipids (Hishikawa et al. Citation2014). Thus, the lipid peroxidation process triggered by OS is able to affect sperm function, modifying plasma membrane fluidity and lipid integrity (Collodel et al. Citation2020a). Notably, an increase in ROS has been associated with male infertility diseases related to inflammation conditions including varicocele, tobacco usage, alcohol, obesity/metabolic syndrome, leukocytospermia, sexually transmitted diseases, bacterial prostatitis and viral infections (Agarwal et al. Citation2018).
Sperm oxidative damage should be avoided and/or rescued by the activity of antioxidant enzymes (Aitken et al. Citation2016); on this point, the ability of spermatozoa to defend themselves from ROS attack is also supported by glucose-6-phosphate dehydrogenase and NADPH activity (Urner and Sakkas Citation2005). Additionally, the antioxidant defenses of seminal plasma (such as superoxide dismutase, glutathione peroxidase and catalase, carotene α-tocopherol, and transferrin and ceruloplasmin) protect spermatozoa from ROS attack (Agarwal and Sekhon Citation2011). In this regard, ROS levels in sperm of infertile groups are associated with lower amounts of chain-breaking antioxidants in seminal plasma (Lewis et al. Citation1995).
Isoprostanes in male reproduction
The last decade has shown a growing interest in the role of isoprostanes in diseases related to male infertility, in addition to all the pathological conditions in which the relevance of isoprostanes has been already demonstrated (Milne et al. Citation2015). In particular, investigations in the isoprostane field could be useful to identify novel markers for the evaluation of i) infertility pathogenesis, ii) semen quality, and iii) new therapeutic/nutraceutical/antioxidant approaches to infertility management. By considering the role of isoprostanes as markers, the relevance of the methodology, as previously described and used for the quantification of isoprostanes, should be taken into account, in order to obtain the most accurate detection (Milne et al. Citation2007).
Increased levels of urinary 8-iso-PGF2α (8-isoprostane or 15-F2 t isoprostane) were considered as a marker of oxidative stress in leukocytospermia and oligozoospermia conditions, both associated with urogenital inflammation (Kullisaar et al. Citation2013; Punab et al. Citation2013). In a recent paper (Rosen et al. Citation2019), the levels of urinary 8-isoprostane-PGF2α were measured in men of couples attending fertility treatment and no significant associations were observed between marker and reproductive outcomes. Interestingly, in urine samples from couples undergoing in vitro fertilization (IVF) the relationship between urinary 15-F2 t isoprostane and phthalates has been studied; a positive association between the investigated parameters (isoprostanes and phthalates) was observed in males only, indicating a sex-specific behavior of these compounds. (Wu et al. Citation2017).
Concerning the role of isoprostanes as markers of OS in seminal plasma, Moreno-Fernandez et al. (Citation2019) have recently demonstrated a decrease in 15-F2 t-isoprostane and a reduced DNA oxidative damage in germ cells of rats fed with fermented goat or cow milk-based diets, suggesting that both products may have an anti-inflammatory activity, preventing injuries to testis germinal epithelium. In boars, 8-isoprostane was used as a marker of OS in dialyzed semen treated with α-tocopherol prior to storage and compared to control sample (Zakošek Pipan et al. Citation2017). In humans, although the number of papers available is small (), the seminal F2-IsoP levels seem to be relevant in the evaluation of OS in seminal fluid. Khosrowbeygi and Zarghami (Citation2007) reported that free 8-iso-PGF2α was significantly higher in infertile patients. Sperm motility and morphology were negatively correlated with free 8-iso-PGF2α and positively with catalase.
Table 2. Current relevant findings on isoprostanes in male infertility.
According to WHO (Citation2010), leukocytospermia is a condition defined as the presence of more than 1 million peroxidase-positive leukocytes per ml in an ejaculate. Moreover, it has been reported that sexual intercourse with a male partner having leukocytospermia, a pathology characterized by OS revealed by the 8-isoprostane level, increases the OS level in the woman interfering with fertilization (Mändar et al. Citation2013). In addition, seminal 8-isoprostane levels were able to discriminate between mild OS, beneficial for sperm telomere length maintenance, and severe OS that induces the shortening of telomeres (Mishra et al. Citation2016). Eight-isoprostane and its antioxidant activity were also listed as paramount factors in influencing semen parameters, sperm DNA integrity and pregnancy rate in a cohort of infertile patients after exercise training (Hajizadeh Maleki and Tartibian Citation2018). Moreover, seminal F2-IsoP levels were confirmed to be a reliable indicator of seminal OS in oligoasthenozoospermic infertile men treated with Coenzyme Q10 supplementation (Nadjarzadeh et al. Citation2014).
It is well known that varicocele is a common risk factor for male fertility and OS may be associated with this condition (Agarwal et al. Citation2018). On this point, Collodel et al. (Citation2018) demonstrated that seminal F2-IsoP levels are a sensitive marker in infertile varicocele patients. In this research, they reported that the level of isoprostanes positively correlated with sperm immaturity, a sperm pathology frequently increased in individuals affected by varicocele. In the same study, immunofluorescence analysis using an 8-iso-PGF2α antibody was performed in spermatozoa of patients with different seminal conditions. In fertile men, the signal was almost absent, in patients with idiopathic infertility the label was absent in about 65% of spermatozoa and when present, the signal was weakly localized in the neck region and sometimes along the tail (). In spermatozoa from infertile patients affected by varicocele, the staining of 8-iso-PGF2α was present in almost 68% of spermatozoa appearing clearly localized in the cytoplasm surrounding the head and coiled tails of immature sperm (). These data suggest that a correct FA integrity is needed for sperm maturation.
Figure 4. Immunofluorescence staining of spermatozoa using an anti8-iso-PGF2α polyclonal antibody and 4,6‐Diamidino‐2‐phenylindole (DAPI) solution. (A) sperm from an idiopathic infertile patient show a weak label of anti8-iso-PGF2α in the sperm tail, the signal appears more evident at the level of the mitochondrial sheath. The nuclei are staining with DAPI. In (B), showing spermatozoa from an infertile varicocele patient, the anti8-iso-PGF2α staining is located in the tail and in presence of cytoplasmic droplets (arrow). The nuclei stained with DAPI are altered. In (C), spermatozoa of a globozoospermic patient are shown; the nuclei appear perfectly round as evidentiated by DAPI, the anti8-iso-PGF2α fluorescent label is strongly evident in the tails, sometimes coiled, and in the abundant cytoplasmic residues surrounding the round heads. A, B: bar 5 µm, C: bar 7 µm.
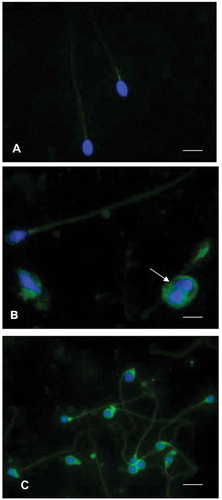
For the first time, F2-IsoPs were investigated in a case of globozoospermia, a rare genetic sperm defect. Globozoospermia results in primary infertility and low fertilization rate following intracytoplasmic sperm injection due to a reduced ability of globozoospermic sperm to activate the oocyte. This phenotype is observed in less than 0.1% of infertile men and characterized by round-headed sperm lacking the acrosome and by immature chromatin (Moretti et al. Citation2005; Dam et al. Citation2007). The F2-IsoP levels were increased both in semen and sperm pellet of a globozoospermic patient versus fertile subjects; the immunolocalization of F2-IsoPs showed a strong label around the sperm head and in the cytoplasmic residue frequently present in this region (). These data indicated that an increased susceptibility to non-enzymatic ARA oxidation could be, at least in part, responsible for fertilization failure in intracytoplasmic sperm injection (Moretti et al. Citation2019).
In the study on the function of isoprostanes in male infertility, PLA2, an enzyme involved in the release of free isoprostanes and eicosanoid production, plays an important role. PLA2 is expressed in different areas of human spermatozoa. Spermatozoa from asthenozoospermic patients showed a reduction in total specific activity and enzyme distributions compared to those in spermatozoa from normozoospermic men indicating that PLA2 could be a potential marker of asthenozoospermia (Anfuso et al. Citation2015). Moreover, Murase et al. (Citation2016) detected that endogenous sPLA2-X, which is highly expressed in spermatozoa, improves sperm fertility.
Collodel et al. (Citation2020b) showed the interplay among cytosolic PLA2 (cPLA2), F2-IsoPs and sperm characteristics in infertile men with leukocytospermia, idiopathic infertility and varicocele, pathologies in which OS may be involved. In this research, F2‐IsoP and cPLA2 levels were positively correlated in the semen of the whole infertile population, in addition, seminal F2‐IsoPs were increased in varicocele and leukocytospermia groups. These results seem to confirm that ARA oxidation/metabolism influences the different male reproductive pathological conditions. Isoprostanes appear to be promising markers of OS and male infertility. They should be added to those already routinely used in the evaluation of semen quality, for the assessment of i) biological mechanism involved in male reproductive dysfunction ii) sperm immaturity and iii) new therapeutic/nutraceutical/antioxidant approaches to infertility management.
Authors’ contributions
Contributing to the writing of the manuscript and drawing of review figures and table: CS, GC, EM; carried out the collection of relevant data and literature: CS, GC; conceived the draft, edited the manuscript, revised the manuscript: CS, GC, EM. All authors have read and approved the final version of the manuscript and agreed with the order of presentation of the authors.
Disclosure statement
No potential conflict of interest was reported by the authors.
Additional information
Funding
References
- Agarwal A, Allamaneni SSR. 2011. Free radicals and male reproduction. J Indian Med Assoc. 109(3):184–187.
- Agarwal A, Gupta S, Sharma RK. 2005. Role of oxidative stress in female reproduction. Reprod Biol Endocrinol. 3:28.
- Agarwal A, Rana M, Qiu E, AlBunni H, Bui AD, Henkel R. 2018. Role of oxidative stress, infection and inflammation in male infertility. Andrologia. 50(11):e13126.
- Agarwal A, Saleh RA. 2002. Role of oxidants in male infertility: rationale, significance, and treatment. Urol Clin North Am. 29(4):817–827.
- Agarwal A, Sekhon LH. 2011. Oxidative stress and antioxidants for idiopathic oligoasthenoteratospermia: is it justified? Indian J Urol. 27(1):74–85.
- Agarwal A, Virk G, Ong C, Du Plessis SS. 2014. Effect of oxidative stress on male reproduction. World J Mens Health. 32:1–17.
- Aitken RJ. 2017. Reactive oxygen species as mediators of sperm capacitation and pathological damage. Mol Reprod Dev. 84(10):1039–1052.
- Aitken RJ, Gibb Z, Baker MA, Drevet J, Gharagozloo P. 2016. Causes and consequences of oxidative stress in spermatozoa. Reprod Fertil Dev. 28(1–2):1–10.
- Amaral A, Lourenço B, Marques M, Ramalho-Santos J. 2013. Mitochondria functionality and sperm quality. Reproduction. 146(5):R163–74.
- Anfuso CD, Olivieri M, Bellanca S, Salmeri M, Motta C, Scalia M, Satriano C, La Vignera S, Burrello N, Caporarello N, et al. 2015. Asthenozoospermia and membrane remodeling enzymes: a new role for phospholipase A2. Andrology. 3(6):1173–1182.
- Baskaran S, Finelli R, Agarwal A, Henkel R. 2020. Reactive oxygen species in male reproduction: a boon or a bane? Andrologia. 2020:e13577.
- Basu S. 1998. Radioimmunoassay of 8-iso-prostaglandin F2alpha: an index for oxidative injury via free radical catalysed lipid peroxidation. Prostaglandins Leukot Essent Fatty Acids. 58(4):319‐325.
- Basu S. 2004. Isoprostanes: novel bioactive products of lipid peroxidation. Free Radic Res. 38:105–122.
- Basu S. 2007. Novel cyclooxygenase-catalyzed bioactive prostaglandin F2alpha from physiology to new principles in inflammation. Med Res Rev. 27(4):435–468.
- Bisht S, Faiq M, Tolahunase M, Dada R. 2017. Oxidative stress and male infertility. Nat Rev Urol. 14(8):470–485.
- Casetta B, Longini M, Proietti F, Perrone S, Buonocore G. 2012. Development of a fast and simple LC-MS/MS method for measuring the F2-isoprostanes in newborns. J Matern Fetal Neonatal Med. 114–11814(8):470–485.
- Chatterjee S, Talwar P. 2019. Role of measurement of reactive oxygen species in semen sample of patients with male factor infertility and treatment with antioxidants in patients with high ROS levels. Int J Contemp Med Res. 6(2):B1–B5.
- Collodel G, Castellini C, Chung-Yung Lee J, Signorini C. 2020a. Relevance of fatty acids to sperm maturation and quality. Oxid Med Cell Longev. 7038124:2020.
- Collodel G, Castellini C, Iacoponi F, Noto D, Signorini C. 2020b. Cytosolic phospholipase A2 and F2-isoprostanes are involved in semen quality and human infertility-a study on leucocytospermia, varicocele and idiopathic infertility. Andrologia. 52(2):e13465.
- Collodel G, Moretti E, Longini M, Pascarelli NA, Signorini C. 2018. Increased F2-isoprostane levels in semen and immunolocalization of the 8-iso prostaglandin F2α in spermatozoa from infertile patients with varicocele. Oxid Med Cell Longev. 7508014:2018.
- Collodel G, Moretti E, Micheli L, Menchiari A, Moltoni L, Cerretani D. 2015. Semen characteristics and malondialdehyde levels in men with different reproductive problems. Andrology. 3:280–286.
- Comporti M, Signorini C, Arezzini B, Vecchio D, Monaco B, Gardi C. 2008. F2-isoprostanes are not just markers of oxidative stress. Free Radic Biol Med. 44(3):247–256.
- Dam AHDM, Feenstra I, Westphal JR, Ramos L, van Golde RJT, Kremer JAM. 2007. Globozoospermia revisited. Hum Reprod Update. 13(1):63–75.
- de Lamirande E, Gangnon C. 1995. Capacitation-associated production of superoxide anion by human spermatozoa. Free Radical Biol Med. 18:487–495.
- Desai N, Sharma R, Makker K, Sabanegh E, Agarwal A. 2009. Physiologic and pathologic levels of reactive oxygen species in neat semen of infertile men. Fertil Steril. 92:1626–1631.
- El-Tohamy MM. 2012. The mechanisms by which oxidative stress and free radical damage produces male infertility. Life Sci J. 9:674–688.
- Fam SS, Morrow JD. 2003. The isoprostanes: unique products of arachidonic acid oxidation-A review. Curr Med Chem. 10(17):1723–1740.
- Galano JM, Lee YY, Oger G, Vigor C, Vercauteren J, Durand T, Glera M, Lee JC. 2017. Isoprostanes, neuroprostanes and phytoprostanes: an overview of 25years of research in chemistry and biology. Prog Lipid Res. 68:83–108.
- Gil-Guzman E, Ollero M, Lopez MC, Sharma RK, Alvarez JG, Thomas AJ Jr, Agarwal A. 2001. Differential production of reactive oxygen species by subsets of human spermatozoa at different stages of maturation. Hum Reprod. 16:1922–1930.
- Gomez E, Buckingham DW, Brindle J, Lanzafame F, Irvine DS, Aitken RJ. 1996. Development of an image analysis system to monitor the retention of residual cytoplasm by human spermatozoa: correlation with biochemical markers of the cytoplasmic space, oxidative stress, and sperm function. J Androl. 17:276–287.
- Gutteridge JMC, Halliwell B. 2018. Mini-Review: oxidative stress, redox stress or redox success? Biochem Biophys Res Commun. 502(2):183–186.
- Hajizadeh Maleki B, Tartibian B. 2018. Resistance exercise modulates male factor infertility through anti-inflammatory and antioxidative mechanisms in infertile men: a RCT. Life Sci. 203:150–160.
- Halliwell B, Gutteridge JM. 1984. Oxygen toxicity, oxygen radicals, transition metals and disease. Biochem J. 219(1):1–14.
- Hishikawa D, Hashidate T, Shimizu T, Shindou H. 2014. Diversity and function of membrane glycerophospholipids generated by the remodeling pathway in mammalian cells. J Lipid Res. 55(5):799–807.
- Kadiiska MB, Gladen BC, Baird DD, Germolec D, Graham LB, Parker CE, Nyska A, Wachsman JT, Ames BN, Basu S, et al. 2005. Biomarkers of oxidative stress study II: are oxidation products of lipids, proteins, and DNA markers of CCl4 poisoning? Free Radic Biol Med. 38(6):698–710.
- Khosrowbeygi A, Zarghami N. 2007. Levels of oxidative stress biomarkers in seminal plasma and their relationship with seminal parameters. BMC Clin Pathol. 7:6.
- Kullisaar T, Türk S, Kilk K, Ausmees K, Punab M, Mändar R. 2013. Increased levels of hydrogen peroxide and nitric oxide in male partners of infertile couples. Andrology. 1(6):850–858.
- Lewis SE, Boyle PM, McKinney KA, Young IS, Thompson W. 1995. Total antioxidant capacity of seminal plasma is different in fertile and infertile men. Fertil Steril. 64(4):868–870.
- Liu W, Morrow JD, Yin H. 2009. Quantification of F2-isoprostanes as a reliable index of oxidative stress in vivo using gas chromatography-mass spectrometry (GC-MS) method. Free Radic Biol Med. 47(8):1101‐1107.
- Lushchak VI. 2014. Free radicals, reactive oxygen species, oxidative stress and its classification. Chem Biol Interact. 224:164–175.
- Mändar R, Kullisaar T, Borovkova N, Punab M. 2013. Sexual intercourse with leukocytospermic men may be a possible booster of oxidative stress in female partners of infertile couples. Andrology. 1(3):464–468.
- Medina S, Domínguez-Perles R, Gil JI, Ferreres F, García-Viguera C, Martínez-Sanz JM, Gil-Izquierdo A. 2012. A ultra-pressure liquid chromatography/triple quadrupole tandem mass spectrometry method for the analysis of 13 eicosanoids in human urine and quantitative 24 hour values in healthy volunteers in a controlled constant diet. Rapid Commun Mass Spectrom. 26(10):1249‐1257.
- Milne GL, Dai Q, Roberts LJ 2nd. 2015. The isoprostane—25 years later. Biochim Biophys Acta. 1851:433–445.
- Milne GL, Musiek ES, Morrow JD. 2005. F2-isoprostanes as markers of oxidative stress in vivo: an overview. Biomarkers. 10(Suppl 1):S10–23.
- Milne GL, Yin H, Brooks JD, Sanchez S, Jackson Roberts L 2nd, Morrow JD. 2007. Quantification of F2-isoprostanes in biological fluids and tissues as a measure of oxidant stress. Methods Enzymol. 433:113–126.
- Mishra S, Kumar R, Malhotra N, Singh N, Dada R. 2016. Mild oxidative stress is beneficial for sperm telomere length maintenance. World J Methodol. 6(2):163–170.
- Moazamian R, Polhemus A, Connaughton H, Fraser B, Whiting S, Gharagozloo P, Aitken RJ. 2015. Oxidative stress and human spermatozoa: diagnostic and functional significance of aldehydes generated as a result of lipid peroxidation. Mol Hum Reprod. 21:502–515.
- Moreno-Fernandez J, Alférez MJM, López-Aliaga I, Diaz-Castro J. 2019. Protective effects of fermented goat milk on genomic stability, oxidative stress and inflammatory signalling in testis during anaemia recovery. Sci Rep. 9(1):2232.
- Moretti E, Collodel G, Salvatici MC, Belmonte G, Signorini C. 2019. New insights into sperm with total globozoospermia: increased fatty acid oxidation and centrin1 alteration. Syst Biol Reprod Med. 65(5):390–399.
- Moretti E, Collodel G, Scapigliati G, Cosci I, Sartini B, Baccetti B. 2005. ’Round Head’ sperm defect. Ultrastructural and meiotic segregation study. J Submicrosc Cytol Pathol. 37:297–303.
- Morrow JD, Awad JA, Boss HJ, Blair IA, Roberts LJ 2nd. 1992. Non-cyclooxygenase-derived prostanoids (F2-isoprostanes) are formed in situ on phospholipids. Proc Natl Acad Sci U S A. 89(22):10721–10725.
- Morrow JD, Awad JA, Wu A, Zackert WE, Daniel VC, Roberts LJ 2nd. 1996. Nonenzymatic free radical-catalyzed generation of thromboxane-like compounds (Isothromboxanes) in vivo. J Biol Chem. 271(38):23185–23190.
- Morrow JD, Roberts LJ 2nd. 1991. Quantification of noncyclooxygenase derived prostanoids as a marker of oxidative stress. Free Radic Biol Med. 10(3–4):195–200.
- Morrow JD, Roberts LJ. 1997. The isoprostanes: unique bioactive products of lipid peroxidation. Prog Lipid Res. 36(1):1–21.
- Murase R, Sato H, Yamamoto K, Ushida A, Nishito Y, Ikeda K, Kobayashi T, Yamamoto T, Taketomi Y, Murakami M. 2016. Group X secreted phospholipase A2 releases ω3 polyunsaturated fatty acids, suppresses colitis, and promotes sperm fertility. J Biol Chem. 291(13):6895–6911.
- Nadjarzadeh A, Shidfar F, Amirjannati N, Vafa MR, Motevalian SA, Gohari MR, Nazeri Kakhki SA, Akhondi MM, Sadeghi MR. 2014. Effect of coenzyme Q10 supplementation on antioxidant enzymes activity and oxidative stress of seminal plasma: a double-blind randomised clinical trial. Andrologia. 46(2):177–183.
- Nourooz-Zadeh J. 1999. Gas chromatography-mass spectrometry assay for measurement of plasma isoprostanes. Methods Enzymol. 300:13–17.
- Pasqualotto FF, Sharma RK, Potts JM, Nelson DR, Thomas AJ, Agarwal A. 2000. Seminal oxidative stress in patients with chronic prostatitis. Urology. 55(6):881–885.
- Phaniendra A, Jestadi DB, Periyasamy L. 2015. Free radicals: properties, sources, targets, and their implication in various diseases. Indian J Clin Biochem. 30(1):11–26.
- Plante M, de Lamirande E, Gagnon C. 1994. Reactive oxygen species released by activated neutrophils, but not by deficient spermatozoa, are sufficient to affect normal sperm motility. Fertil Steril. 62:387–393.
- Praticò D. 1999. F(2)-isoprostanes: sensitive and specific non-invasive indices of lipid peroxidation in vivo. Atherosclerosis. 147(1):1–10.
- Punab M, Kullisaar T, Mändar R. 2013. Male infertility workup needs additional testing of expressed prostatic secretion and/or post-massage urine. PLoS One. 8(12):e82776.
- Roberts LJ 2nd, Morrow JD. 2002. Products of the isoprostane pathway: unique bioactive compounds and markers of lipid peroxidation. Cell Mol Life Sci. 59(5):808–820.
- Rosen EM, Mínguez-Alarcón L, Meeker JD, Williams PL, Milne GL, Hauser R, Ferguson KK, EARTH Study Team. 2019. Urinary oxidative stress biomarker levels and reproductive outcomes among couples undergoing fertility treatments. Hum Reprod. 34(12):2399–2409.
- Rubio-Riquelme N, Huerta-Retamal N, Gómez-Torres MJ, Martínez-Espinosa RM. 2020. Catalase as a molecular target for male infertility diagnosis and monitoring: an overview. Antioxidants (Basel). 9(1):pii: E78.
- Sasaki DM, Yuan Y, Gikas K, Kanai K, Taber D, Morrow JD, Roberts LJ 2nd, Callewaert DM. 2002. Enzyme immunoassays for 15-F2T isoprostane-M, an urinary biomarker for oxidant stress. Adv Exp Med Biol. 507:537‐541.
- Sies H, Berndt C, Dean PJ. 2017. Oxidative stress. Annu Rev Biochem. 86:715–748.
- Signorini C, De Felice C, Galano JM, Oger C, Leoncini S, Cortelazzo A, Ciccoli L, Durand T, Hayek J, Chung-Yung Lee J. 2018. Isoprostanoids in clinical and experimental neurological disease models. Antioxidants (Basel). 7:7.
- Taber DF, Morrow JD, Roberts LJ 2nd. 1997. A nomenclature system for the isoprostanes. Prostaglandins. 53(2):63–67.
- Takahashi K, Nammour TM, Fukunaga M, Ebert J, Morrow JD, Roberts LJ 2nd, Hoover RL, Badr KF. 1992. Glomerular actions of a free radical-generated novel prostaglandin, 8-epi-prostaglandin F2 alpha, in the rat. Evidence for interaction with thromboxane A2 receptors. J Clin Invest. 90(1):136–141.
- Urner F, Sakkas D. 2005. Involvement of the pentose phosphate pathway and redox regulation in fertilization in the mouse. Mol Reprod Dev. 70(4):494–503.
- Vigor C, Bertrand-Michel J, Pinot E, Oger C, Vercauteren J, Le Faouder P, Galano JM, Chung-Yung Lee J, Durand T. 2014. Non-enzymatic lipid oxidation products in biological systems: assessment of the metabolites from polyunsaturated fatty acids. J Chromatogr B Analyt Technol Biomed Life Sci. 964:65–78.
- World Health Organization. 2010. WHO laboratory manual for the examination and processing of human semen, 5th ed. Geneva, Switzerland: WHO Press.
- Wu H, Olmsted A, Cantonwine DE, Shahsavari S, Rahil T, Sites C, Pilsner JR. 2017. Urinary phthalate and phthalate alternative metabolites and isoprostane among couples undergoing fertility treatment. Environ Res. 153:1–7.
- Yura T, Fukunaga M, Khan R, Nassar GN, Badr KF, Montero A. 1999. Free-radical-generated F2-isoprostane stimulates cell proliferation and endothelin-1 expression on endothelial cells. Kidney Int. 56(2):471–478.
- Zakošek Pipan M, Mrkun J, Nemec Svete A, Zrimšek P. 2017. Improvement of liquid stored boar semen quality by removing low molecular weight proteins and supplementation with α-tocopherol. Anim Reprod Sci. 186:52–61.
- Zhang H, Il’yasova D, Sztaray J, Young SP, Wang F, Millington DS. 2010. Quantification of the oxidative damage biomarker 2,3-dinor-8-isoprostaglandin-F(2alpha) in human urine using liquid chromatography-tandem mass spectrometry. Anal Biochem. 399(2):302–304.