ABSTRACT
Oxidative stress from the trophoblasts is one of the possible pathological mechanisms of Preeclampsia (PE). This study aimed at exploring the potential effects of astaxanthin (ATX) on oxidative stress damaged placental trophoblast cell line HTR-8/SVneo. Oxidative stress-induced damaged through H2O2 treatment was checked by MTS CellTiter 96® cell viability, 2ʹ,7ʹ-dichlorofluorescein diacetate (DCFH-DA) induced fluorescence, the level of the intracellular malondialdehyde (MDA), and the detection of glutathione peroxidase (GSH-Px), superoxide dismutase (SOD) and catalase (CAT). Different concentrations of ATX were applied, and then the proliferation rate, apoptotic percentage, cell cycle distribution, invasion test and relative biological function of the rescued cells were followed. We provide evidence that ATX had an anti-oxidative effect against oxidative stress induced by H2O2 on the trophoblast cell line and had beneficial role in promoting cell proliferation, inhibiting cell apoptosis, and inducing cell invasion.
Abbreviations: UV: ultraviolet; DCFH-DA: 2’,7’-dichlorofluorescein diacetate; EVT: extravillous trophoblast; MMPs: matrix metalloproteinases; IUGR: intrauterine growth restriction.
Introduction
Successful embryo implantation highly depends on close cooperation of blastocyst invasiveness and endometrial receptivity (Kong et al. Citation2019; Chiarello et al. Citation2020). These two aspects are related to cell differentiation/developmental status and are precisely controlled by various factors, highlighted by trophoblast invasion, a necessary process for placenta implantation (Chiarello et al. Citation2020). An hypoxic environment is a prerequisite for completing trophoblast invasion (Lu et al. Citation2018; Chiarello et al. Citation2020). Due to changes in hormone metabolism and low intrauterine inflammation caused by early embryo implantation, embryo metabolism and/or embryo surroundings could generate compensatory reactive oxygen species (ROS). On one hand, ROS can alter most of the cellular molecules and could also retard or induce a developmental block. On the other hand, moderate levels of ROS can be beneficial to reduce inflammation, wound healing, and tissue repair processes (Lu et al. Citation2018; Kong et al. Citation2019; Chiarello et al. Citation2020). However, excessive ROS production is reported to have negative impacts on placental formation and embryo development. High ROS levels could lead to reduced bioavailability of nitric oxide, impaired endothelium-dependent vasodilation, and hence promote vasoconstriction (Aouache et al. Citation2018). Minimizing oxidative stress is a complex problem Several different compensatory pathways targeting ROS in embryos, including exogenous: non-enzymatic antioxidants, e.g., hypotaurine, and endogenous, e.g., superoxide dismutase, which could immediately inhibit ROS. However, the concentration of these pro-oxidants is hard to ascertain and is difficult to maintain in embryos. Thus, limiting oxidative stress during embryo culture is still of great importance.
The HTR-8 cell line is derived from normal human chorionic villus tissue and could be cultured and identified by histological culture methods. It has an invasive phenotype of extravillous trophoblast cell line. In order to achieve longer survival and increased the stability, the pS3-neo plasmid of the SV40 region upstream gene and the neomycin phosphotransferase gene were inserted into the HTR-8 cell line to establish the immortalized cell line HTR-8/SVneo (Abou-Kheir et al. Citation2017) that has been used in research about trophoblast-related diseases. The HTR-8/SVneo cells not only maintain the biological characteristics and phenotype like the original cells, but and high purity cultures are easy to prepare. HTR8/SVneo cells exposed to hypoxia/reoxygenation (H/R) revealed that oxidative stress from the trophoblasts is one of the possible pathological mechanisms of PE (Chen et al. Citation2016; Rao et al. Citation2019).
Astaxanthin (ATX) is a natural ketone carotenoid, which is enriched in marine carapace. The most important character of ATX is the powerful antioxidant effect which could reduce active oxygen species 40 times more than that of β-carotene and 1,000 times that of vitamin E (Chen et al. Citation2015; Vahidinia et al. Citation2017). It also has multiple roles in ultraviolet (UV) protection, pigmentation reduction, immune response control, regulation of reproductive capacity, stress tolerance, anti-inflammatory and DNA damage repair processes (Wang et al. Citation2019). In this study, the in vitro oxidative stress cell model using human placental trophoblast cell line HTR-8/SVneo was established to investigate the preventive effect and biological function of ATX on oxidative stress injury in placental trophoblast cells.
Results
Response of ATX to H2O2
Optimal H2O2 concentrations were determined based on viability following 50, 100, 200, 400, or 800 μM H2O2 treatment. The MTS assay confirmed that 200 μM H2O2 was appropriate for modeling oxidative stress (Figure S1). Low concentration of H2O2 (50 μM) was not enough to inhibit cell proliferation. After treatment with100 μM and 200 μM H2O2for 2 h, no significant change was noticed in cell viability, while after 6 h culture, relative cell viability decreased to 71.1%. When the concentration exceeded 200 μM (400 μM and 800 μM) cytotoxicity increased significantly and the cell survival rate decreased to nearly zero. In the subsequent experiments, 200 μM H2O2 was selected for 6 h to establish the ROS model.
To investigate the antioxidant impact of ATX at different concentrations on the ROS cell model, cell viability, the fluorescence of 2ʹ,7ʹ-dichlorofluorescein diacetate (DCFH-DA), the level of intracellular malondialdehyde (MDA), glutathione peroxidase (GSH-Px), superoxide dismutase SOD activity and the enzymatic activities of catalase (CAT) were determined. After ATX treatment, cell viability gradually increased compared with the control group, especially when exposed in 10 μM ATX. Interestingly, cell survival remained unchanged. A significant rise (P < 0.01) in cell viability was shown between 10 μM ATX group and 0 μM ATX group (). The fluorescence of DCFH-DA and concentration of MDA, decreased significantly (P < 0.01) in the 10 μM ATX-treated group, compared with that in 0 μM ATX group (). The main defense of cells against ROS is provided by SOD and CAT, which convert superoxide radicals to H2O2 first, and then to molecular oxygen and water. Other enzymes, such as GSH-Px, use a thiol reducing power of GSH to reduce oxidized lipids and protein. All rose significantly (P < 0.01) in 10 μM ATX-treated group in comparison with that in the 0 μM ATX group, even nearly close to the control group (none H2O2 treatment) (). The antioxidant effects of ATX were dose dependent, and 10 μm ATX appeared optimal ().
Figure 1. Determination of the optimal concentrations of ATX. To investigate the optimal concentrations of ATX applied to the ROS model of HTR-8/SVneo cells, (a) the cell viability, (b) the level of DCFH-DA, (c) MDA content, (d) SOD activity, (e) GSH-Px content, and (f) the enzymatic activities of CAT were determined. Compared with control group: ** P < 0.01; *** P < 0.001. Compared with H2O2 group: # P < 0.05; ## P < 0.01; ### P < 0.001. DCFH-DA, 2ʹ,7ʹ-dichlorofluorescein diacetate; MDA, malondialdehyde; GSH-Px, glutathione peroxidase; SOD, superoxide dismutase; CAT, catalase
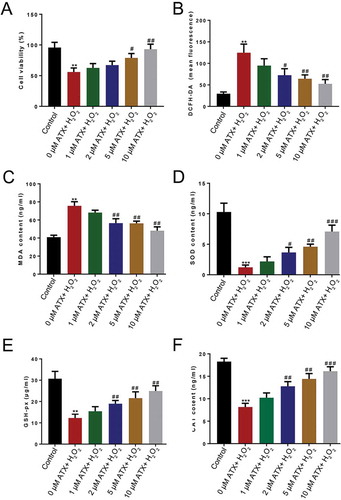
ATX promotes the proliferation of HTR-8/SVneo cells
EDU immunofluorescence staining of HTR-8/SVneo cells after the series treatments suggested the ATX maintains cell viability. Compared with the control group (no treatment), the EDU-positive cells of H2O2-treated groups significantly decreased. However, upon supplementation with extra 10 μM ATX, the number of EdU-positive cells was rescued (, Figure S2). Surprisingly, ATX did affect the cell cycle distribution of HTR-8/SVneo cells. The percentage of S phase cell is typically used as an indicator of the state of cell proliferation. It was higher in 10 μM ATX group (22.7%) than that in both the control group (20.3%) and the H2O2-treated group (16.0%) (), Figure S3).
Figure 2. EdU staining of HTR-8/SVneo cells. EdU staining was used to observe cell proliferation (original magnification ×10). The number of EdU-positive cells was recorded. The proliferation rate of H2O2-treated cell is sharply decreased, while ATX could attenuate this effect. Red for Edu; blue for DAPI; dark reddish purple for merge, scale bar is 100 μm
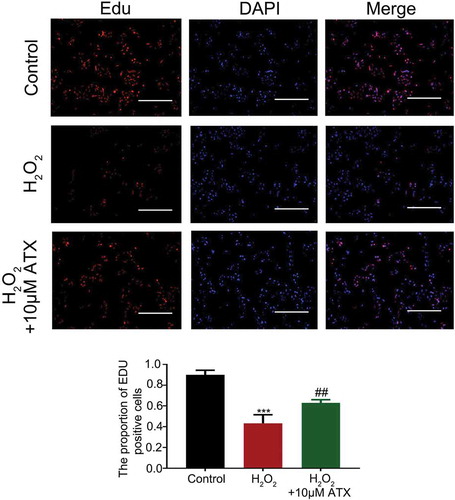
Figure 3. The cell cycle distribution and apoptosis percentage of HTR-8/SVneo cells.(A) HTR-8/SVneo cells were treated differently for 24 h, then analyzed by flow cytometry for cell cycle distribution. Cell cycle distributions after H2O2 and ATX treatment are shown. Blue for cells in 2 n; green for cells in 2 n–4 n; pink for 4 n. (B) The apoptosis percentage of cells was determined by flow cytometry after Annexin V/PI staining. *P < 0.05 and **P < 0.01 were considered statistically significant
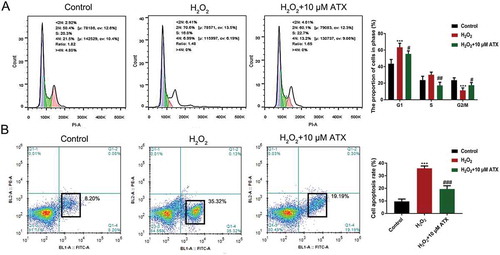
ATX inhibits HTR-8/SVneo cells apoptosis and induces cell invasion
Annexin V+/PI+ cells are characterized as late apoptotic cells and Annexin V-/PI+ cells as early apoptotic cells. After ATX treatment, the number of Annexin V-/PI+ cells (19.19%) were much lower than that in the H2O2 group (35.32%). ATX inhibited early HTR-8/SVneo cell apoptosis (), Figure S4). In addition, the transwell migration assay showed that H2O2 treatment reduced the number of migrated cells, which was reversed by ATX administration (), Figure S5). Cell invasion of the lower chamber was counted after crystal violet staining. The number was enhanced significantly after ATX supplementation, compared with that of the H2O2 group (), Figure S6). These results confirmed the role of ATX in inhibiting apoptosis and inducing cell invasion.
Figure 4. The apoptosis percentage of cells and cell invasion situation. (A) The migrated cells were detected by crystal violet staining and the numbers were counted under microscope (original magnification ×10). (B) The invaded cells were detected by crystal violet staining and the numbers were counted under microscope (original magnification ×10) **P < 0.01; ***P < 0.001. Compared with H2O2 group: #P < 0.05; ##P < 0.01; ###P < 0.001
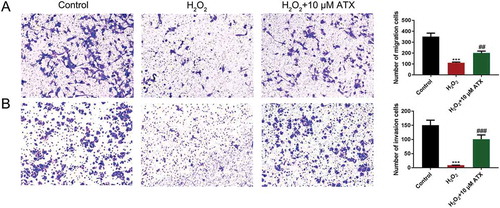
ATX treatment rescues cell invasion of HTR-8/SVneo cells
The levels and activities of several MMPs (especially MMP2 and MMP9) were demonstrated to relate to trophoblast invasion. Therefore, we examined their expression by qRT-PCR and Western blotting. The results showed that both MMP2 and MMP9 decreased (P < 0.01) in the H2O2 group at the RNA and protein levels. This effect was reversed and showed a 1.5-fold (P < 0.05) increase after ATX treatment. Similar changes of activin A, NRF2 were also observed (P < 0.05). Smad3 was phosphorolated and levels elevated (P < 0.05) in response to oxidative after ATX intervention ().
Figure 5. The effect of ATX treatment on HTR-8/SVneo cells function exposed to oxidative stress. (A) Real-time PCR was used to measure the expression of MMP-2, MMP-9, NRF2 and Smad3. (B) Western blot analysis was applied to assess the expression of MMP-2, MMP-9, NRF2, p-Smad3 and Smad3. Compared with control group: **P < 0.01; ***P < 0.001. Compared with H2O2 group: #P < 0.05; ##P < 0.01. MMP-2: matrix metalloproteinase-2; MMP-9: matrix metalloproteinase-9; NRF2: nuclear factor erythroid 2-related factor 2; p-Smad3: phosphor-mothers against decapentaplegic homolog 3; Smad3: mothers against decapentaplegic homolog 3
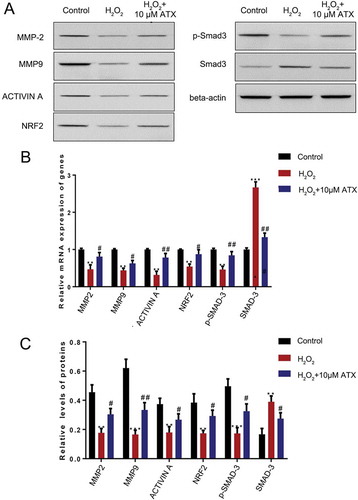
Discussion
Embryo attachment occurs in a harmonious state between the mother and the fetus playing an active invasive role (Dai et al. Citation2016). Under physiological conditions, blastocyst implantation during th early pregnancy phase is accompanied by invasion of extravillous trophoblast (EVT) cells into the decidua, forming a continuous EVT shell at the maternal-placental interface (Chiarello et al. Citation2020). The EVT cells then become vascular trophoblast cells and transform the helical arteries into dilated blood vessels which are derived from a muscle layer (Taysi et al. Citation2019). These processes can limit blood flow and oxygenation as the placenta develops (Taysi et al. Citation2019; Chiarello et al. Citation2020). This process finally leads to reduced oxygen tension in the cavity, which is necessary for normal embryo development and to prevent free radical damage (Taysi et al. Citation2019). High oxidative stress is thought to be involved in diverse pathological conditions. Our results showed that after treatment with 200 μM H2O2, ROS (Mittler Citation2017) mimicked the oxidative damage, offering a promising cell model for PE studies.
In addition to anchoring the placenta to the uterus, trophoblast cells can also infiltrate as part of the transformation of the spiral artery to form a complete uterus-placental blood circulatory system. This ensures that the fetus has sufficient nutrients and oxygen supply. In comparison, iinsufficient or excessive invasion could lead to obstetric diseases, such as PE (Abou-Kheir et al. Citation2017; Chiarello et al. Citation2020). Therefore, maintaining a homeostatic trophoblast cell state is another aspect of normal pregnancy and fetal growth (Abou-Kheir et al. Citation2017). Invasion firstly requires the adhesion of the trophoblast cells to the endometrial stroma, followed by infiltration of tumor cells (Dai et al. Citation2016). Adhesion requires the attachment of extracellular matrix (ECM) to integrin, which requires the degradation of ECM by matrix metalloproteinases (MMPs) (Chen and Khalil Citation2017). MMP-2 mediates vascular responses by promoting the production of an anti-angiogenic peptide (endopeptidase 1) through the division of peptides associated with the vascular calcitonin gene (Espino et al. Citation2017). MMP-9 levels rise during normal pregnancies and those complicated by preeclampsia. Our results showed that both were elevated after ATX supplementation, suggesting that ATX promotes expression to recover the adhesion function of trophoblast. In addition to MMPs, the expression of activin A, a member of the TGF- beta family, in placenta and serum of patients with pre-eclampsia, is significantly higher than those of normal pregnancy, always at 2.52 ng/mL (1.24 multiples of the median (MoM)) (Spencer et al. Citation2008). This is consistent with the view that it may be involved in pre-eclampsia caused by dystrophic cell dysfunction (Muttukrishna et al. Citation1997). Activin A also produced comparable increases in human trophoblast cell invasion via SMAD2/3-SMAD4-dependent N-cadherin upregulation (Li et al. Citation2014). In our study, both RNA and protein level of activin A were elevated. Although many aspects about the activin A in the pathological mechanism of PE remain ambiguous, ATX certainly had an up-regulatory effect of activin A. It is reasonable that we hypothesis that ATX may play a preventive role in the occurrence of PE by acting on activin A molecule. Oxidative stress is related to a number of placental pathologies including spontaneous miscarriage, gestational diabetes, and intrauterine growth restriction (IUGR) (Mannaerts et al. Citation2018). In our study, the antioxidant effect of ATX was shown to be sufficient to promote the proliferation of HTR-8/SVneo cells, inhibiting cell apoptosis and inducing cell invasion after ROS damage through its antioxidant and anti-inflammatory effects and these rescue functions were concentration dependent. Under physiological conditions, ROS can induce the expression of various antioxidant-related genes through feedback to the activation of NRF2 (nuclear transcription factor) (Loboda et al. Citation2016). Our results revealed that the expression of NRF2 decreased after the ROS damage H2O2 brought, and the expression was rescued by stimulation by ATX. But the molecule mechanism of how ATX affect NRF2 again remains to be clarified. We confirmed that the oxidative stress of trophoblast cells induced by H2O2 could affect the normal HTR-8/SVneo cell proliferation and invasion, and the beneficial role of ATX on ROS damaged cells. However, whether ATX could also improve the pathological conditions in humans remains unknown. The application of ATX has a series of limitations. These include that only Haematococcus pluvialis and P. carotenatin ATX were approved for human dietary supplementation at 12 to 24 mg/day and 6 mg/day, respectively, for 30 days or less (Tapeinos and Pandit Citation2016). The use of ATX in different animal models was necessary to confirm the antioxidant capacity of ATX. Nevertheless, the antioxidant effect of ATX can protect HTR-8/SVneo cells from ROS, characterized by promoting the proliferation of HTR-8/SVneo cells, inhibiting cell apoptosis and inducing cell invasion, might have a potential to prevent the occurrence of PE and other relative diseases resulted by oxidative stress.
Materials and methods
Cell line and cell culture
Human placental trophoblast cell line HTR-8/SVneo was purchased from Shanghai Academy of Sciences Cell Bank (Shanghai. China). High-glucose DMEM containing 10% FBS (Gibco; Thermo Fisher Scientific, Inc., Waltham, MA, USA), and penicillin/streptomycin (Lonza, Castleford, UK) was used for cell culture in a humidified 5% CO2 37°C incubator. Cells were cultured to 90% confluence and then Trypsin-EDTA (Thermo fisher scientific, Waltham, MA, USA) was used for passaging cells, which were split 1:3 into fresh T75 flasks. Medium was changed every other day, and cells in logarithmic growth phase were taken for experiments.
Establishment and identification of oxidant stress model in vitro
HTR-8/SVneo cells were then plated in a 96-well plate with 10,000 cells/well when grown to 70–80% confluence, three replicates for each group. After 24 h culture, the cells were treated with H2O2 (Sigma Aldrich, MO, USA) with a gradient concentration of 0, 50, 100, 200, 400, or 800 μM, 200 μL per well. After culture for 2, 6, 12, 24 hours, cell viability was monitored by MTS assay. Briefly, 0.5 mg/mL of the MTS dye (Promega, Madison, WI, USA) was added per well for 4 h. Performance was in strict accordance with reagent instructions.
ATX incubation
After treatment with H2O2, AHTR-8/SVneo cells were transferred to a 96-well plate with a concentration of 5,000 cells/well with three replicates for each group. After 24 hours’ culture, ATX (Sigma Aldrich, MO, USA) dissolved in polyethylene glycol 400-N, N-dimethylacetamide (PEG400) (Sigma Aldrich, MO, USA) with a 50:50 v/v ratio and were used with a concentration gradient of (0, 1, 2, 5, 10 μM, respectively) was used to rescue the H2O2 cells, and then were incubated at 37°C with 5% CO2 for 6 h. Following steps for H2O2 intervention were performed as above.
Evaluations of the intracellular MDA, GSH-Px, SOD and CAT
After ATX and H2O2 treatments, cells were harvested at 1 × 107/mL in DMEM, and then 10 mM DCFH-DA was added at 37°C for 30 min in dark. Cells were washed thrice using serum-free media, and then flow cytometry was performed to quantify fluorescence with 488 and 525 nm excitation and emission wavelengths. To determine the intracellular MDA level, GSH-Px content, SOD activity and the enzymatic activities of CAT, all were measured by kits (Nan Jiancheng Bioengineering Institute, Nanjing, China, Molecular Technology, Tokyo, Japan).
Evaluation of the cell proliferative ability
EdU Assay provides a sensitive and robust method to detect and quantify cell proliferation in living mammalian cells using flow cytometry or fluorescence microscopy. For EdU assays, medium was changed by medium supplemented with 0.1% EdU buffer A and culture for 2 h. Cells were then collected, spun for 5 min at 350 ×g, washed in 1× PBS, ph 7.4, and then fixed by 4% paraformaldehyde/PBS for 10 min. After fixation, cells were washed 3 times by PBS again, then permeabilized for 10 min with 0.5% Triton X-100, washed again, resuspended for 10 min in 1× Apollo staining solution. Cells were then washed thrice using 0.5% Triton X-100 before resuspension in PBS as described in the Cell-Light EdU ApolloR 594 Kit (RiboBio, Guangzhou, China). Anti-fluorescence quench sealing liquid was then used for cell mounting prior to inverted fluorescence microscope (Bx51.Olympus Corporation, Shinjuku, Japan) analysis.
Apoptosis/necrosis assay (Annexin V/PI assay)
A FITC-annexin V PI kit was used to assess apoptosis based on provided directions. Briefly, 4.5 × 105 cells were seeded to 6-well plate until confluent, ATX and H2O2 were added as appropriate. Cells were incubated for an additional 24 h, prior to staining for 15 min with FITC-annexin V and PI (BD, Rhode Island, USA) to stain apoptotic and necrotic cells, respectively. A FACS Calibur flow cytometer was then used to analyze cells, cells were Annexin V+ being apoptotic, and those that were Annexin V+ PI+ being necrotic.
Cell-Cycle analysis
Flow cytometry PI staining was used for detecting cell cycle of three groups of cells, and the protective mechanism of ATX on ROS-related DNA damage was verified. Measurement was conducted following the instructions of the ACPA Elisa kit (MyBioSource, San Diego, USA) instructions.
Transwell assays
Transwell chambers (the aperture in the bottom membrane was 8 µm) (Corning, NY, USA) coated with Matrigel (BD Biosciences, Oxford, UK) were used for the determination of invasive ability. Totally, 10,000 cells were seeded and incubated with serum-free DMEM medium for 12 h at 37°C with 5% CO2. Then, the medium of the lower chamber was DMEM medium containing 10% FBS. After 24 h, a swab was used to remove the non-invading cells and remaining cells were fixed with methyl alcohol followed by 0.1% crystal violet staining. Migration/invasion were then assessed under microscopy (x 200).
Invasion assay
Invasion assay was performed as described before [9]. Briefly, growth factor reduced matrigel matrix (1 μg/mL; BD Biosciences, #356,230) was added to the upper chamber of 8 µm filter pore size transwell insert (Greiner Bio, #662,638). 1: 1 ratio of DMEM and Ham’s F12 medium (300 µL/well) supplemented with 1% FBS was added to the lower chamber containing transwell inserts. HTR-8/SVneo cells (0.1 × 106 cells/150 µL) suspended in the same medium were seeded in the upper chamber of the transwell. After 24 h of incubation at 37°C under humidified atmosphere, medium from lower chamber was aspirated and the excess of cells and Matrigel on the top of membrane of the transwell inserts were removed using moist cotton swab. Cells from the lower side of the membrane were fixed by chilled methanol for 7–10 min at 4°C, followed by crystal violet (Biotium Inc., Hayward, CA, USA) staining and visualized for counting using the microscopy.
qRT-PCR
Total RNA was extracted according to the reagent instruction of Trizol regent (Invitrogen, Waltham, MA, USA). Qualitative analysis was performed by formaldehyde denaturing gel electrophoresis, and quantitative analysis was performed by ultraviolet spectrophotometer. A total of 1 μg total RNA was used to design MMP-2, MMP-9, activin A, NRF2, p-Smad3, Smad3 and internal reference β-actin primers, which were synthesized by Takara (). A Prime Script TM reverse transcription reagent kit (Takara Bio, Shiga, Japan) was used for reverse transcription. The target genes and the reference genes were amplified by fluorescent quantitative PCR. The PCR reaction solution was 50 μL, including 25 μL of 10× PCR buffer, 2 μL of PCR forward primer, 2 μL PCR reverse primer, 1 μL of ROX Reference Dye (50×), 4 μL of cDNA, and 16 μL of sterile distilled water. Reaction conditions: successive denaturation at 94°C for 5 min, at 94°C for 30 sec, at 58°C for 45 sec, and at 72°C for 30 sec, for 40 cycles, followed by extending at 72°C for 10 min. Three replicate wells were set for all reactions. The expression of each gene was analyzed. The relative difference in the expression of the target gene was calculated by 2−ΔΔCt. ΔCt = Ct target gene – Ct reference gene; ΔΔCt = Δt treatment group – ΔCt control group.
Table 1. Oligonucleotide primers used in real-time PCR
Western blotting
Cell lysate protein was quantified by a BCA kit (Thermo fisher scientific, Waltham, MA, USA), samples were treated with β-mercaptothion and then heated for 3 min in 95°C water bath. Then, 25 μg samples with 4× laemmlii buffer were load on 10% SDS-PAGE and then transfer to PVDF membrane. Blots were blocked with 5% BSA for 1 h at room temperature. Then, incubated with primary antibodies overnight at 4°C: rabbit anti-Matrix metalloproteinase-2 (MMP2) (1:1000, Abcam, Cambridge, UK), rabbit anti-MMP9 (1:1000, GeneTex, Irvine, CA, USA), mouse anti-activin A (1:1000, Abcam, Cambridge, UK), rabbit anti-NRF2 (1:500, Abcam, Cambridge, UK), rabbit anti-p-Smad3 (1:1000, Cell Signaling Technology, MA, USA), rabbit anti-Smad3 (1:1000, Cell Signaling Technology), and rabbit anti-β-actin (1:4000, CST). The next day, rinse the membrane thrice with TBST, each time for 5 min. Goat Anti-Mouse Anti-Rabbit IgG/IgM H&L (HRP polymer) and Goat Anti-Rabbit IgG+ IgM H&L (HRP polymer) (Abcam, Cambridge, UK) were respectively added according to the primary antibody for 1 h at room temperature. ECL chemiluminescence reagent and Gel Doc EZ Imager were used to develop images. The gray value of the target band was analyzed by Image J.
Statistical analysis
Experiments were conducted in technical triplicates of at least biological quadruplicates. All statistical calculations were performed using Prism 5 software (GraphPad Software, La Jolla, CA, USA). Data are presented as means ± standard error of the mean. Data were compared via one-way ANOVAs with Dunnett’s multiple comparison test. P < 0.05 was the significance threshold.
Author contributions
Designed experiments: FJY, ZYJ; carried out experiments: JY, XYP; analyzed experimental results: WXH, GYW; wrote the manuscript: FJY; approved the manuscript: ZYJ. All authors read and approved the final manuscript.
Ethics approval
N/A; Informed consent statement N/A.
Supplemental Material
Download Zip (5.7 MB)Disclosure statement
The authors declare no conflict of interest, financial or otherwise.
Supplementary material
Supplemental data for this article can be accessed here.
Data availability statement
All data generated or analyzed during this study are included in this published article.
References
- Abou-Kheir W, Barrak J, Hadadeh O, Daoud G. 2017. HTR-8/SVneo cell line contains a mixed population of cells. Placenta. 50:1–7. eng. DOI: 10.1016/j.placenta.2016.12.007.
- Aouache R, Biquard L, Vaiman D, Miralles F. 2018. Oxidative stress in preeclampsia and placental diseases. Int J Mol Sci. 19(5). eng. DOI: 10.3390/ijms19051496.
- Chen J, Khalil RA. 2017. Matrix metalloproteinases in normal pregnancy and preeclampsia. Prog Mol Biol Transl Sci. 148:87–165. eng.
- Chen Y, Zhang Y, Deng Q, Shan N, Peng W, Luo X, Zhang H, Baker PN, Tong C, Qi H. 2016. Wnt5a inhibited human trophoblast cell line HTR8/SVneo invasion: implications for early placentation and preeclampsia. J Maternal-fetal Neonatal Med. 29(21):3532–3538. eng. DOI: 10.3109/14767058.2016.1138102.
- Chen YY, Lee PC, Wu YL, Liu LY. 2015. In vivo effects of free form astaxanthin powder on anti-oxidation and lipid metabolism with high-cholesterol diet. PloS One. 10(8):e0134733. eng. DOI: 10.1371/journal.pone.0134733.
- Chiarello DI, Abad C, Rojas D, Toledo F, Vazquez CM, Mate A, Sobrevia L, Marin R. 2020. Oxidative stress: normal pregnancy versus preeclampsia. Biochim Et Biophys Acta Mol Basis Dis. 1866(2):165354. eng. DOI: 10.1016/j.bbadis.2018.12.005.
- Dai ZY, Liu D, Li R, Wang Y, Zhang J, Liu J, Zhou R, Zeng G. 2016. [Association between gestational weight gain per trimester/total gestational weight gain and gestational diabetes mellitus]. Zhonghua Liu Xing Bing Xue Za Zhi = Zhonghua Liuxingbingxue Zazhi. 37(10):1336–1340. chi.
- Espino YSS, Flores-Pliego A, Espejel-Nunez A, Medina-Bastidas D, Vadillo-Ortega F, Zaga-Clavellina V, Estrada-Gutierrez G. 2017. New insights into the role of matrix metalloproteinases in preeclampsia. Int J Mol Sci. 18(7):1448. eng
- Huang BK, Langford TF, Sikes HD. 2016. Using sensors and generators of H2O2 to elucidate the toxicity mechanism of piperlongumine and phenethyl Isothiocyanate. Antioxid Redox Signal. 24(16):924–938. eng. DOI: 10.1089/ars.2015.6482.
- Kong S, Zhou C, Bao H, Ni Z, Liu M, He B, Huang L, Sun Y, Wang H, Lu J. 2019. Epigenetic control of embryo-uterine crosstalk at peri-implantation. Cell Mol Life Sci. 76(24):4813–4828. eng.
- Li S, Takahara T, Fujino M, Fukuhara Y, Sugiyama T, Li XK, Takahara S. 2017. Astaxanthin prevents ischemia-reperfusion injury of the steatotic liver in mice. PloS One. 12(11):e0187810. eng. DOI: 10.1371/journal.pone.0187810.
- Li Y, Klausen C, Cheng JC, Zhu H, Leung PC. 2014. Activin A, B, and AB increase human trophoblast cell invasion by up-regulating N-cadherin. J Clin Endocrinol Metab. 99(11):E2216–2225. eng.
- Loboda A, Damulewicz M, Pyza E, Jozkowicz A, Dulak J. 2016. Role of Nrf2/HO-1 system in development, oxidative stress response and diseases: an evolutionarily conserved mechanism. Cell Mol Life Sci. 73(17):3221–3247. eng.
- Lu J, Wang Z, Cao J, Chen Y, Dong Y. 2018. A novel and compact review on the role of oxidative stress in female reproduction. Reprod Biol Endocrinol. 16(1):80. eng.
- Mannaerts D, Faes E, Cos P, Briede JJ, Gyselaers W, Cornette J, Gorbanev Y, Bogaerts A, Spaanderman M, Van Craenenbroeck E. et al. 2018. Oxidative stress in healthy pregnancy and preeclampsia is linked to chronic inflammation, iron status and vascular function. PloS One. 139:e0202919. eng. doi:10.1371/journal.pone.0202919.
- Mittler R. 2017. ROS Are Good. Trends Plant Sci. 22(1):11–19. eng. DOI: 10.1016/j.tplants.2016.08.002.
- Muttukrishna S, Knight PG, Groome NP, Redman CW, Ledger WL. 1997. Activin A and inhibin A as possible endocrine markers for pre-eclampsia. Lancet (London, England). 349(9061):1285–1288. eng. DOI: 10.1016/S0140-6736(96)09264-1.
- Okazaki Y, Okada S, Toyokuni S. 2017. Astaxanthin ameliorates ferric nitrilotriacetate-induced renal oxidative injury in rats. J Clin Biochem Nutr. 61(1):18–24. eng. DOI: 10.3164/jcbn.16-114.
- Rao H, Bai Y, Zhang F, Li Q, Zhuang B, Luo X, Qi H. 2019. The role of SATB1 in HTR8/ SVneo cells and pathological mechanism of preeclampsia. J Maternal-fetal Neonatal Med. 32(12):2069–2078. eng. DOI: 10.1080/14767058.2018.1425387.
- Spencer K, Cowans NJ, Nicolaides KH. 2008. Maternal serum inhibin-A and activin-A levels in the first trimester of pregnancies developing pre-eclampsia. Ultrasound Obstetrics Gynecol. 32(5):622–626. eng. DOI: 10.1002/uog.6212.
- Tapeinos C, Pandit A. 2016. Physical, chemical, and biological structures based on ros-sensitive moieties that are able to respond to oxidative microenvironments. Adv Mater (Deerfield Beach, Fla). 28(27):5553–5585. eng. DOI: 10.1002/adma.201505376.
- Taysi S, Tascan AS, Ugur MG, Demir M. 2019. Radicals, oxidative/nitrosative stress and preeclampsia. Mini Rev Med Chem. 19(3):178–193. eng. DOI: 10.2174/1389557518666181015151350.
- Vahidinia A, Rahbar AR, Shakoori Mahmoodabadi MM. 2017. Effect of astaxanthin, vitamin E, and vitamin C in combination with calorie restriction on sperm quality and quantity in male rats. J Diet Suppl. 14(3):252–263. eng. DOI: 10.1080/19390211.2016.1211783.
- Visioli F, Artaria C. 2017. Astaxanthin in cardiovascular health and disease: mechanisms of action, therapeutic merits, and knowledge gaps. Food Funct. 8(1):39–63. eng. DOI: 10.1039/C6FO01721E.
- Wang XJ, Tian DC, Wang FW, Zhang MH, Fan CD, Chen W, Wang MH, Fu XY, Ma JK. 2019. Astaxanthin inhibits homocysteine induced endothelial cell dysfunction via the regulation of the reactive oxygen species dependent VEGFVEGFR2FAK signaling pathway. Mol Med Rep. 19(6):4753–4760. eng.
- Zhang J, Wang QZ, Zhao SH, Ji X, Qiu J, Wang J, Zhou Y, Cai Q, Zhang J, Gao HQ. 2017. Astaxanthin attenuated pressure overload-induced cardiac dysfunction and myocardial fibrosis: partially by activating SIRT1. Biochim et Biophys Acta Gen Subj. 1861(7):1715–1728. eng.. DOI: 10.1016/j.bbagen.2017.03.007.
- Zhang M, Cui Z, Cui H, Cao Y, Zhong C, Wang Y. 2016. Astaxanthin alleviates cerebral edema by modulating NKCC1 and AQP4 expression after traumatic brain injury in mice. BMC Neurosci. 17(1):60. eng. DOI: 10.1186/s12868-016-0295-2.
- Zuluaga M, Gregnanin G, Cencetti C, Di Meo C, Gueguen V, Letourneur D, Meddahi-Pelle A, Pavon-Djavid G, Matricardi P. 2017. PVA/Dextran hydrogel patches as delivery system of antioxidant astaxanthin: a cardiovascular approach. Biomed Mater (Bristol, England). 13(1):015020. eng. DOI: 10.1088/1748-605X/aa8a86.