ABSTRACT
In this study, we aimed to explore the potential differences in proteomic profiles from the testicular tissue of azoospermatic men with impaired spermatogenesis and normal spermatogenesis. Isobaric tags for relative and absolute quantitation (iTRAQ) labeled technology and LC-MS/MS technology were used to identify differentially expressed proteins. Potential functions of differentially expressed proteins were predicted using gene ontology (GO) and the Kyoto encyclopedia of genes and genomes (KEGG). Immunohistochemistry (IHC) and western blot (WB) were used to verify the differentially expressed proteins. A protein–protein interaction (PPI) network was built to outline the regulatory network of differentially expressed proteins. A total of 3,945 proteins were identified in men with normal and impaired spermatogenesis. Of these, 116 proteins were differentially expressed in men with impaired spermatogenesis: 39 were upregulated and 77 were downregulated. Furthermore, we found that these differentially expressed proteins were mainly involved in the cellular component, which may be mainly associated with the spliceosome, ribosome, and thyroid hormone synthesis signaling pathways. The spliceosome- and ribosome-associated proteins YBX1, FBL, and HNRNPU were downregulated. And the proteomic profile of testicular tissue in men with impaired spermatogenesis is different from that of men with normal spermatogenesis. For this reason, differentially expressed proteins such as YBX1, FBL and HNRNPU might be involved in the pathology of spermatogenesis dysfunction.
Abbreviations: iTRAQ: Isobaric tags for relative and absolute quantitation;GO: Gene ontology; KEGG: Kyoto encyclopedia of genes and genomes; IHC: Immunohistochemistry; WB: Western blot; PPI: Protein–protein interaction; ICSI: Intracytoplasmic sperm injection; BP: Biological process; CC: Cellular components; MF: Molecular function; snoRNA: Small nucleolar RNA; snRNA: Small nuclear RNA; LC-MS/MS: Liquid chromatography and MS/MS analysis; BSA: Bovine serum albumin; SD: Spermatogenic dysfunction; micro-TESE: Testicular microscopic sperm extraction
KEYWORDS:
Introduction
Approximately 15% of couples are infertile, and >60% of infertility in these couples has a male factor component. Defects in spermatogenesis function are the most common factors in male infertility (Esteves et al. Citation2011; Habib et al. Citation2018). Azoospermia and oligozoospermia are severe conditions of male infertility.
The testis, a sperm-producing tissue, comprises germ cells and various somatic cells including Sertoli cells, myoid cells, Leydig cells, and macrophages (Vloeberghs et al. Citation2015). Sperm develop in the seminiferous tubules of the testis, undergo complex processes, and eventually form mature male gametes. The main processes of spermatogenesis are as follows: proliferation of spermatogonia; differentiation of spermatogonia into spermatocytes via mitosis; meiosis of spermatocytes producing spermatozoa; maturation and release of sperm (Nishimura and L’Hernault Citation2017; Swerdloff Citation2017). Spermatogenesis is tightly regulated, and it can be regulated and interrupted at any stage of sperm development. It has been reported that many nanoparticles are harmful to spermatogenesis (Yang et al. Citation2020). Various factors contribute to spermatogenesis dysfunction including obesity, diabetes, environmental chemicals such as bisphenol A, genetic factors such as Y-chromosome microdeletions, and epigenetic factors such as methylation and miRNA (Neto et al. Citation2016; Pini et al. Citation2020). Although scientific advances provide some explanation for the molecular mechanisms of spermatogenesis, the etiology and molecular mechanisms of spermatogenesis dysfunction still have to be elucidated. In addition, although patients with azoospermia and oligospermia can choose to use assisted reproductive treatments such as intracytoplasmic sperm injection (ICSI), a routine treatment for male infertility caused by nonobstructive azoospermia, some patients do not benefit from these treatments (Vloeberghs et al. Citation2015). Therefore, the analysis of spermatogenesis-related molecules from multiple perspectives will help to deepen our understanding of male infertility and predict patients who may benefit from ICSI treatment.
Proteomics is a useful tool for studying and understanding spermatogenesis disorders. The identification, quantitation, and pairwise comparison of proteins in specific tissues and cells can help in the screening of clinically significant biomarkers. For example, sperm protein expression profiles in patients with decreasing sperm levels have been identified using proteomics (Thacker et al. Citation2011; Hashemitabar et al. Citation2015). Zhao et al. identified differences between the protein expression profiles of sperms with reduced motility and normal sperms (Zhao et al. Citation2007). The differential abundance of sperm phosphoproteins in two human sperm populations with high and/or low mobility was determined by phosphoproteomic analysis (Martin-Hidalgo et al. Citation2020). Presently, there are few studies on proteomic analyses of patients with spermatogenesis dysfunction. A previous study analyzed the testicular protein expression profiles of patients with nonobstructive azoospermia and revealed 520 azoospermia-associated proteins (Alikhani et al. Citation2017). In the present study, we compared the protein expression profiles of men with normal and impaired spermatogenesis by isobaric tags using relative and absolute quantization (iTRAQ) labeled technology and LC-MS/MS technology. Our aim is to determine testicular proteins related to spermatogenesis dysfunction. Our research has potential clinical value for finding biomarkers and developing infertility treatment strategies for patients with impaired spermatogenesis.
Results
Total proteins expressed in men with normal and impaired spermatogenesis
A total of 11 men with normal spermatogenesis and 11 men with impaired spermatogenesis were included in the analyses. The sperm acquisition rate of the SD group was lower than that of the NC group (SD = 11/11 × 100 = 100%, NC = 6/11 × 100 = 54.55%). These patients had been diagnosed with infertility for >1 year ().
Table 1. Testis parameters from patients in spermatogenic dysfunction group and normal group
iTRAQ was used to assess proteomic profiles of testicular tissues in men with normal and impaired spermatogenesis. iTRAQ and LC-MS/MS results revealed two peaks of the isoelectric points, 5–7 and 8.5–9.5, for all the identified peptides (). Among these peptides, most peptides were distributed in the range of 10 to 70 molecular weights (). The largest proportion of peptides matching the unique proteins was 2 peptides, followed by 1, 3, 4, 5, and 6 peptides (). The length of identified peptides was primarily 7–15 amino acids, with the longest length in 10 (). The sequence coverage of most peptides ranged from 0–5% followed by 5–10%. There were 179 protein sequences that reached 50–100% sequence coverage ().
Figure 1. Identification and analysis of the testicular tissue proteome. Distribution of the isoelectric point (A), protein mass (B), number of peptides matched to proteins (C), peptide length (D), and protein sequence coverage (E) identified by iTRAQ and LC-MS/MS. The iTRAQ and LC-MS/MS results revealed two peaks of the isoelectric points, 5–7 and 8.5–9.5, respectively, for all identified peptides. Also, most peptides were distributed within a molecular weight range of 10–70. The largest proportion of peptides matching the unique proteins was 2 peptides followed by 1, 3, 4, 5, and 6 peptides. The length of identified peptides was primarily 7–15 amino acids, and there were 179 protein sequences that reached 50–100% sequence coverage
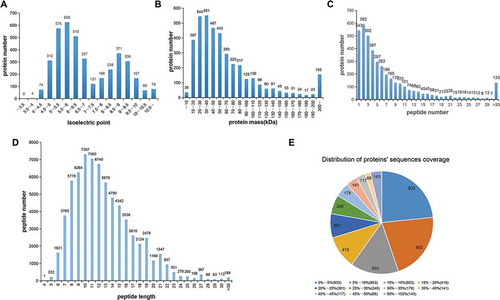
Hierarchical clustering and functional classification of differentially expressed proteins in men with normal and impaired spermatogenesis
Heatmap analysis revealed that protein sequences were differentially expressed in men with impaired spermatogenesis compared (SD) with men in the NC group (). A total of 3,945 proteins were identified in men with normal and impaired spermatogenesis (Supplementary Table 1). Out of these proteins, 116 were differentially expressed in men from the SD group. Among them, 39 and 77 proteins were significantly upregulated and downregulated, respectively (Supplementary Table 2). GO analysis showed that these differentially expressed proteins were mainly enriched in terms of biological processes (BP) (e.g., cellular process, metabolic process, and biological process), in terms of cellular components (CC) (e.g., cells, cell parts, and organelles) and in terms of molecular function (MF) (e.g., binding and catalytic activity) (). KEGG analysis revealed that the differentially expressed proteins were enriched in spliceosome, ribosome and thyroid hormone synthesis pathways ().
Figure 2. Heatmap (A), GO (B), and KEGG analyses (C) showing the expression profile and possible function of differentially expressed proteins. Heatmap analysis revealed that the sequence of proteins was differentially expressed in men with impaired spermatogenesis compared (SD) to men in the NC group. Also, these differentially expressed proteins were mainly involved in the cellular component, which could be mainly associated with the spliceosome, ribosome, and thyroid hormone synthesis signaling pathways. NC1, NC2: two men with normal spermatogenesis function; SD1, SD2: two men with spermatogenic dysfunction
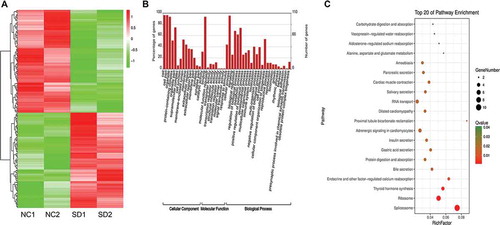
Potential functions of differentially expressed proteins
Combined with the results of LC-MS/MS analysis, we selected proteins with significant differences in differential expression. IHC and WB were performed to verify the change in protein expressions. The relative expression of YBX1, FBL, and HNRNPU were determined (). YBX1, FBL, and HNRNPU were downregulated in the testicular tissue of men from the SD group when compared with men from the NC group, which was confirmed by WB (). IHC also showed that the relative expressions of YBX1, FBL, and HNRNPU proteins were downregulated when compared to those of men from the NC group ().
Table 2. Information of the differential expressed protein verified by IHC and western blot
Figure 3. Expression of YBX1, FBL, and HNRNPU in men with normal spermatogenesis (NC) and men with spermatogenic dysfunction (SD) verified by WB (A) and IHC (B). These three proteins – YBX1, FBL, and HNRNPU – were downregulated in the testicular tissue of men from the SD group when compared to men from the NC group. *P < 0.05, 100x, and 200x represent micrograph magnifications of 100 × and 200 ×
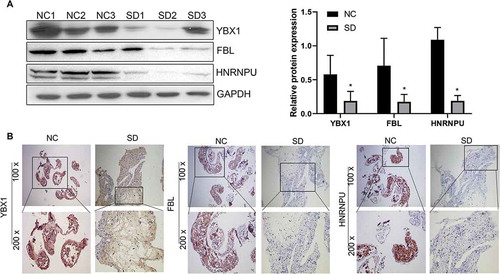
Network analysis of YBX1, FBL, and HNRNPU proteins
Combined with protein sequencing data, a PPI network was constructed to outline the regulatory network of YBX1, FBL and HNRNPU proteins. As shown in , YBX1 and HNRNPU proteins are connected to several proteins including SRSF10, etc. Based on the network analysis, we determined that there was also an internal link among YBX1, HNRNPU and FBL in the PPI network ().
Figure 4. Protein–protein interaction networks of YBX1, FBL, and HNRNPU. The YBX1 and HNRNPU proteins were linked to several proteins including SRSF10. Based on the network analysis, we found that there was an internal link between YBX1, HNRNPU, and FBL in the PPI network. Green represents YBX1, FBL, and HNRNPU proteins; pink represents proteins that can interact with differential proteins YBX1, FBL, and HNRNPU
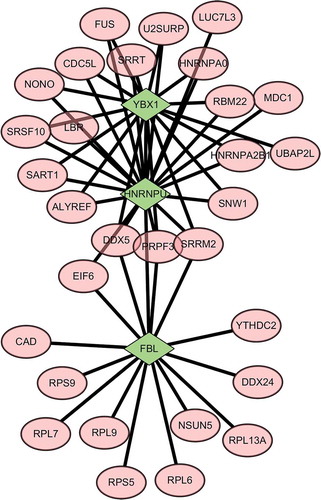
Discussion
In this study, we identified proteins that were differentially expressed in men with impaired spermatogenesis and those with normal spermatogenesis. Bioinformatic analyses revealed that these differentially expressed proteins were mainly enriched in terms of BP (e.g., cellular processes, metabolic processes, and biological processes), in terms of CC (e.g., cells, cell parts, and organelles) and in terms of MF (e.g., binding and catalytic activity), which potentially involved in pathways related to spliceosomes, ribosomes, and thyroid hormone synthesis. Moreover, we verified that the expression of YBX1, FBL and HNRNPU proteins was downregulated in men with impaired spermatogenesis.
Presently, little is known about testicular tissue protein expression profiles in patients with sperm dysfunction and infertility. Previous studies found that patients with non-structural azoospermia have abnormal expression of some related pathways, especially those with Sertoli-cell-only syndrome when compared to patients with infertile structural azoospermia. These pathways include those related to metabolic processes, steroid biosynthetic processes, spliceosomes, and ribosomes (Alikhani et al. Citation2017). Jodar studied semen proteomics and male infertility through comparative proteomics to identify the specific biological pathways affected in infertile patients. For example, the disturbance in energy generation mainly by the citrate cycle and also in the regulation of apoptosis in asthenozoospermic patients was observed (Jodar et al. Citation2016). In the present study, abnormalities in the metabolic process, spliceosome, and ribosome pathways were observed in the testicular tissue of patients with impaired spermatogenesis.
Among these biological processes, hormone and metabolic processes play an important role in spermatogenesis. Thyroid abnormalities (hyperthyroidism or hypothyroidism) can affect testicle size and the proliferation and differentiation of testicular cells such as Sertoli, Leydig, and germ cells, thereby affecting spermatogenesis and fertility (Wajner et al. Citation2009; La Vignera et al. Citation2017; Hernandez Citation2018). In fact, oligozoospermia and teratozoospermia are often observed in patients with thyroid abnormalities (Abalovich et al. Citation1999; La Vignera and Vita Citation2018), indicating that proper thyroid levels in the testes are essential for normal testicular spermatogenesis and maintenance of reproductive function. In the testis, metabolic abnormalities are related to the suppression of spermatogenesis. For example, the abnormal activity of metabolic enzymes was observed in chemically-induced spermatogenesis-inhibited rats (Ravikumar and Srikumar Citation2005). As a physical support for germ cells, testosterone metabolism levels in Sertoli cells affect sperm development (Parapanov et al. Citation2008; Alves et al. Citation2013; Tourmente and Roldan Citation2013). In shrews (Mammalia, Soricidae), the cycle length of spermatogenesis is positively correlated with basal metabolic rate. In our GO and KEGG analyses, the enrichment of differentially expressed proteins in metabolic processes and the thyroid hormone synthesis pathway, respectively, suggests their potential involvement in the regulation of spermatogenesis.
The spliceosome is a type of macromolecular complex that regulates RNA–RNA binding and mediates the processing of precursor mRNA into mRNA. SAP155, an essential component of the spliceosome, is expressed in the mouse testes (Eto et al. Citation2010). Major spliceosome defects affect testicular spermatogonia differentiation and inhibit sperm development and maturation; these issues are linked with the development of nonobstructive azoospermia (Wu et al. Citation2016). In our KEGG analysis, the enrichment of differentially expressed proteins in the spliceosome pathway suggests that the spliceosome might be involved in spermatogonia.
YBX1 is a DNA and RNA binding protein involved in the regulation of transcription, pre-mRNA splicing, and mRNA stability and translation (Lyabin et al. Citation2014). In the PPI regulatory network of YBX1, FBL, and HNRNPU proteins, HNRNPU is linked to SRSF10, a member of the same family of spliceosome U5 small nuclear RNA (snRNA), and the RNA binding protein YBX1. Although YBX1 function has still not been completely elucidated, existing research suggests that HNRNPU and YBX1 are present in the c-Myc mRNA complex and play a role in mRNA stabilization. HNRNPU and YBX1 were identified as factors involved in promoting c-Myc mRNA stabilization via the CRD (Weidensdorfer et al. Citation2009). Besides, Du showed that linc02042 regulated c-Myc mRNA stability in an YBX1-dependent mechanism (Du et al. Citation2020). In addition, YBX1 potentially regulates tumor invasion (Gupta et al. Citation2019), and YBX1 is reported to be involved in regulating cell proliferation, differentiation, and survival (Wolffe Citation1994; Bhullar and Sollars Citation2011). In mice, YBX1 expression in the testis was found to be specifically in the cells on the basement membrane of the seminiferous tubules, which co-localized with undifferentiated spermatogonia (Phillips and Orwig Citation2010). Also, WB and IHC detected downregulation of YBX1 in the testicular tissues of patients with spermatogenesis disorders, suggesting that it may function in the regulation of spermatogenesis (Alikhani et al. Citation2017).
Furthermore, HNRNPU protein is an abundant nucleoplasmic phosphoprotein (Kiledjian et al. Citation1992). It has been reported that cytoplasmic HNRNPU plays a role in the stability control of mRNA (Yugami et al. Citation2020) and the nuclear matrix protein HNRNPU was complexed in vivo with the glucocorticoid receptor (Eggert et al. Citation1997). Fackelmayer et al. believed that HNRNPU might play a role in the organization of chromosomal DNA in addition to its suggested role in hnRNA metabolism (Fackelmayer et al. Citation1994). A global analysis of HNRNPU-dependent splicing by RNA-seq coupled with bioinformatic analysis of associated splicing signals concluded that splicing site selection occurs through modulating the core splicing machinery. HNRNPU has also been identified as a potent regulator of nuclear ribonucleoprotein particles in several gene expression pathways (Xiao et al. Citation2012). We verified the differential expression of a spliceosome protein HNRNPU through WB and IHC, and determined that it was downregulated in the testicular tissues of patients with spermatogenesis disorders, which was like previously found to play an important role in spermatogenesis by affecting the regulation of apoptosis, death, and growth of spermatogenic cells (Li et al. Citation2012).
FBL is a 2′-O-RNA methyltransferase located in the dense fibrillar component of the nucleolus. It is homologous to yeast NOP1, a protein essential for ribosome synthesis. And it associates with U3 snoRNA which is involved in regulating pre-RNA splicing (Tollervey et al. Citation1993). Some of the mechanisms of action of ribosome and spliceosome are similar (Francisco-Velilla et al. Citation2016). The interaction of ribosome and spliceosome pathway-associated proteins has been suggested to exert gene regulatory functions and to regulate spermatogenesis (Francisco-Velilla et al. Citation2016; Mageeney and Ware Citation2019). Ribosome-related pathways include ribosomal components as well as non-ribosomal proteins, ribosomal proteins, and small nucleolar RNA (snoRNA). The abnormal expression of ribosome pathway-related proteins in patients with spermatogenesis impairment supports the involvement of the ribosome pathway in the regulation of spermatogenesis. We specifically verified the downregulation of FBL expression in the testes of men with impaired spermatogenesis. Hassouni believed that targeting key protein rRNA methyltransferase FBL revealed great potential, because of its pivotal role in ribosome biogenesis (Hassouni et al. Citation2019). Also, Gentric found that FBL17 is a regulator of DNA-damage response (Gentric et al. Citation2020). WB and IHC expressed the downregulation of FBL in patients with impaired spermatogenesis in our study, implying that abnormal RNA splicing of key proteins may be involved in sperm differentiation and maturation in the testes of these patients.
Likewise, we found an internal connection between YBX1, FBL, and HNRNPU in a PPI network. This interaction indicates that YBX1, FBL, and HNRNPU could play key roles in spermatogenesis dysfunction; moreover, they may be components of the complex interconnected regulatory network that affects this dysfunction. Despite the data presented above, small sample size is still a limitation of this study. Therefore, further investigation will be required to collect more samples to further study the testicular tissue of males with impaired spermatogenesis and normal spermatogenesis.
In summary, our study found that the protein expression profile of men with impaired spermatogenesis is different from that of men with normal spermatogenesis. Specifically, we verified that ribosome- and spliceosome-associated proteins, YBX1, HNRNPU, and FBL, are downregulated in the testicular tissue of men with impaired spermatogenesis. Our results provide evidence for the mechanism and etiology of spermatogenesis and human infertility. Proteomic studies will help explore the therapies for male infertility and discover potential clinically significant biomarkers.
Materials and methods
Sample collection and iTRAQ labeling
Fresh testicular tissues were obtained from men with azoospermia who underwent testicular sperm aspiration or testicular microscopic sperm extraction (micro-TESE) at the Reproductive Medical Center, Guangdong Women and Children Hospital. The testis function was classified according to testis biopsy and laboratory investigation. All tissues were clinical residual specimens. The study was approved by the ethical committee of Guangdong Women and Children Hospital [202,001,002], and consent was obtained from all participants.
Testicular tissues from the testicular tissues of two men in spermatogenic dysfunction (SD1 and SD2) and normal control (NC1 and NC2) group were collected. They were lyzed using RIPA reagent (Beyotime, Shanghai, China) and quantified using Bradford kit (Beyotime). Proteins (300 μg) were isolated, alkylated, and digested with trypsin (Promega, Madison, WI). The digested peptides were dried and reconstituted using 50 μL triethyl ammonium bicarbonate (TEAB, 0.5 M). The peptides were labeled with iTRAQ reagents (AB Sciex, CA, USA) according to the manufacturer’s recommendations. Proteins in the NC group were labeled with 114 iTRAQ reagents, whereas those in the SD group were labeled with 116 iTRAQ reagents. The iTRAQ-labeled samples were mixed and dried by vacuum concentrator. The basic clinical parameters of participants are listed in . And the outline of experimental design is presented in Supplementary Figure 1.
Two-dimensional liquid chromatography and MS/MS analysis (LC-MS/MS)
Two-dimensional liquid chromatography was performed. Briefly, the iTRAQ-labeled peptides were fractionated using a strong cation exchange column on a high-performance liquid chromatography system (Dionex, Sunnyvale, CA). Fractionated peptides were separated by a linear gradient elution [formed by phase A (20 mM HCOONH4, pH = 10) and phase B (20 mM HCOONH4, 80% acetonitrile (ACN), pH = 10)] at a flow rate of 0.2 mL/min. The separation gradient was set as follows: 5% B, 0–5 min; 5 to 10% B, 5–10 min; 10 to 40% B, 10–60 min; 40 to 95% B, 60–65 min; 95% B, 65–75 min; 95 to 5% B, 75–85 min. A total of 20 fractions were collected and 100-μL fractions were collected every minute. The UV detector was set at 214/280 nm. The peptides were vacuum-dried and then eluted using buffer A (0.1% formic acid) for 65 min to buffer B (80% ACN containing 0.1% formic acid) at a rate of 300 nl/min. LC-MS/MS analysis was performed on a Q Exactive system (Thermo Scientific) in the information-dependent acquisition mode. The mass spectrometry parameters were as follows: 350–1800 m/z for scan range; 70,000 for resolution; 100 ms for maximum injection time per mass spectrum. Tandem mass spectra were recorded at a resolution of 17,500. A total of 20 strongest precursors per MS cycle were selected for fragment separation. The maximum injection time was 60 ms.
LC-MS/MS analysis
RAW files obtained from LC-MS/MS were converted to MGF format using Proteome Discoverer 1.4 (Version 1.4.0.288, Thermo Fisher). ProteinPilot™ Software 4.5 (version 1656, AB Sciex) was used for protein identification and annotation and quantification of MGF format files. The relative expression of proteins in the SD group was normalized to the NC (118:114 or 121:114, 118:116, or 121:116). Proteins were considered to be differentially expressed when the following criteria were fulfilled: Unused (ProtScore) > 1.30 (95.0%), CV ≤ 0.5, AVG ≥ 1.5 or AVG ≤ 0.67, T.TEST < 0.05, Peptides (95%) ≥ 2, and FDR < 0.01 (Tollervey et al. Citation1993).
To explore the potential functions of differentially expressed proteins and the pathways these proteins might be involved in, heatmap, gene ontology (GO), Kyoto encyclopedia of genes and genomes (KEGG) analyses were performed using R package (https://cran.r-project.org/web/packages/pheatmap/). When FDR was ≤0.001, GO terms and KEGG pathways were significantly enriched. A protein–protein interaction network was constructed using String and visualized using Cytoscape.
Immunohistochemistry (IHC)
Testicular puncture biopsies were paraffin embedded. To prepare slides, 3-μm paraffin-embedded tissue sections were cut. The slides were deparaffinized, rehydrated, subjected to microwaves for antigen retrieval, and inactivated by endogenous peroxidase. Thereafter, the sections were incubated in 5% bovine serum albumin (BSA) followed by primary antibodies against fibrillarin FBL (sc-166,001; dilution 1:1000; Santa Cruz), YBX1 (20,339-1-AP; dilution 1:500; Proteintech), and HNRNPU antibodies (sc-32,315; dilution 1:500; Santa Cruz) overnight at 4°C. After incubation with horseradish peroxidase-conjugated secondary antibodies, the sections were determined by 3,3′-diaminobenzidine and photographed. Views obtained from five fields of each picture were selected for the quantification of relative protein expression.
Western blot
Protein concentrations were quantified using a Bradford kit (Beyotime, Shanghai, China). A total of 50 μg protein was loaded and separated by 10% SDS–PAGE gels for each sample. The gels were subsequently transferred to PVDF membranes (Millipore, MA, USA), blocked with 5% BSA and then incubated in FBL (sc-166,001; dilution 1:1000; Santa Cruz), YBX1 (20,339-1-AP; dilution 1:1000; Proteintech), HNRNPU (sc-32,315; dilution 1:1000; Santa Cruz), and GAPDH antibodies (10,494-1-AP; dilution 1:5000; Proteintech) overnight at 4°C. On the next day, the membranes were incubated in horseradish peroxidase-conjugated secondary antibodies (Jackson ImmunoResearch, West Grove, PA, USA). Protein expression was detected using an ECL Western blotting kit (Amercontrol Biosciences, London, UK) and normalized to that of GAPDH. The relative protein expression was calculated based on three independent experiments.
Statistical analysis
All values are presented as mean ± SD. Comparisons between spermatogenic dysfunction and normal control groups were conducted using Student’s t-test. Data analyses were performed using SPSS 22.0 software (IBM, Armonk, NY, USA) and P < 0.05 was considered statistically significant.
Ethics approval
The present study protocol was reviewed and approved by the ethical committee of Guangdong Women and Children Hospital [202,001,002].
Informed consent
Informed consent was submitted by all subjects when they were enrolled.
Authors’ contributions
Conceptualization, methodology, and critically revising the manuscript: YZ. Performing the experiments, analyzing the data and drafting the manuscript: JL, YZ. Participating in study design, studying implementation and revising the manuscript: WZ, LC, SY, PD, YW, XY, XZ. All authors read and approved the final manuscript.
Supplemental Material
Download Zip (2.1 MB)Availability of data and materials
The datasets used and/or analyzed during the current study are available from the corresponding author on reasonable request.
Disclosure statement
The authors declare that they have no competing interests.
Supplementary material
Supplemental data for this article can be accessed here.
Additional information
Funding
References
- Abalovich M, Levalle O, Hermes R, Scaglia H, Aranda C, Zylbersztein C, Oneto A, Aquilano D, Gutierrez S. 1999. Hypothalamic-pituitary-testicular axis and seminal parameters in hyperthyroid males. Thyroid. 9(9):857–863. doi:10.1089/thy.1999.9.857.
- Alikhani M, Mirzaei M, Sabbaghian M, Parsamatin P, Karamzadeh R, Adib S, Sodeifi N, Gilani MAS, Zabet-Moghaddam M, Parker L. 2017. Quantitative proteomic analysis of human testis reveals system-wide molecular and cellular pathways associated with non-obstructive azoospermia. J Proteomics. 162:141–154. doi:10.1016/j.jprot.2017.02.007.
- Alves MG, Rato L, Carvalho RA, Moreira PI, Socorro S, Oliveira PF. 2013. Hormonal control of Sertoli cell metabolism regulates spermatogenesis. Cell Mol Life Sci. 70(5):777–793.
- Bhullar J, Sollars VE. 2011. YBX1 expression and function in early hematopoiesis and leukemic cells. Immunogenetics. 63(6):337–350. doi:10.1007/s00251-011-0517-9.
- Du J, Zhang G, Qiu H, Yu H, Yuan W. 2020. A novel positive feedback loop of linc02042 and c-Myc mediated by YBX1 promotes tumorigenesis and metastasis in esophageal squamous cell carcinoma. Cancer Cell Int. 20:75. doi: 10.1186/s12935-020-1154-x.
- Esteves SC, Miyaoka R, Agarwal A. 2011. An update on the clinical assessment of the infertile male. Clinics. 66(4):691–700. doi:10.1590/S1807-59322011000400026.
- Eto K, Sonoda Y, Jin Y, Abe S-I. 2010. Phosphorylated SAP155, the spliceosomal component, is localized to chromatin in postnatal mouse testes. Biochem Biophys Res Commun. 393(4):577–581. doi:10.1016/j.bbrc.2010.02.014.
- Eggert M, Michel J, Schneider S, Bornfleth H, Baniahmad A, Fackelmayer FO, Schmidt S, Renkawitz R. 1997. The glucocorticoid receptor is associated with the RNA-binding nuclear matrix protein hnRNPU. J Biol Chem. 272(45):28471–28478. doi: 10.1074/jbc.272.45.28471.
- Francisco-Velilla R, Fernandez-Chamorro J, Ramajo J, Martinez-Salas E. 2016. The RNA-binding protein Gemin5 binds directly to the ribosome and regulates global translation. Nucleic Acids Res. 44(17):8335–8351. doi:10.1093/nar/gkw702.
- Fackelmayer FO, Dahm K, Renz A, Ramsperger U, Richter A. 1994. Nucleic-acid-binding properties of hnRNP-U/SAF-A, a nuclear-matrix protein which binds DNA and RNA in vivo and in vitro. Eur J Biochem. 221(2): 749–757. doi: 10.1111/j.1432-1033.1994.tb18788.x.
- Gupta MK, Polisetty RV, Sharma R, Ganesh RA, Gowda H, Purohit AK, Ankathi P, Prasad K, Mariswamappa K, Lakshmikantha A, Uppin MS, Sundaram C, Gautam P, Sirdeshmukh R. 2019. Altered transcriptional regulatory proteins in glioblastoma and YBX1 as a potential regulator of tumor invasion. Sci Rep. 9(1):10986. doi: 10.1038/s41598-019-47360-9.
- Gentric N, Masoud K, Journot RP, Cognat V, Chabouté M-E, Noir S, Genschik P. 2020. The F-box-like protein FBL17 is a regulator of DNA-damage response and co-localizes with RETINOBLASTOMA RELATED 1 at DNA lesion sites. Plant Physiol. 183(3):1295–1305. doi: 10.1104/pp.20.00188.
- Habib S, Elbakry M, Sakran M, Toson E, El-Baz R, Elafify ME. 2018. The impact of heavy metals in impairment of spermatogenesis and sperm density in the human. J Biochem Tech. 9(4):1–8.
- Hashemitabar M, Sabbagh S, Orazizadeh M, Ghadiri A, Bahmanzadeh M. 2015. A proteomic analysis on human sperm tail: comparison between normozoospermia and asthenozoospermia. J Assist Reprod Gen. 32(6):853–863. doi:10.1007/s10815-015-0465-7.
- Hernandez A. 2018. Thyroid hormone role and economy in the developing testis. Vitam Horm. 106:473–500.
- Hassouni BE, Sarkisjan D, Vos JC, Giovannetti E, Peters GJ. 2019. Targeting the ribosome biogenesis key molecule fibrillarin to avoid chemoresistance. Curr Med Chem. 26(33):6020–6032. doi: 10.2174/0929867326666181203133332.
- Jodar M, Soler-Ventura A, Oliva R, Molecular Biology of Reproduction and Development Research Group. 2016. Semen proteomics and male infertility. J Proteomics. 162: 125–134. doi: 10.1016/j.jprot.2016.08.018.
- J-A M, Koskenniemi JJ, Virtanen HE, Toppari J. 2018. Testis development. Endocr Rev. 40(4):857–905.
- Kiledjian M, Dreyfuss G. 1992. Primary structure and binding activity of the hnRNP U protein: binding RNA through RGG box. EMBO J. 11(7):2655–2664.
- La Vignera S, Vita R. 2018. Thyroid dysfunction and semen quality. Int J Immunopathol Pharmacol. 32:2058738418775241.
- La Vignera S, Vita R, Condorelli RA, Mongioì LM, Presti S, Benvenga S, Calogero AE. 2017. Impact of thyroid disease on testicular function. Endocrine. 58(3):397–407.
- Li J, Guo W, Li F, He J, Yu Q, Wu X, Li J, Mao X. 2012. HnRNPL as a key factor in spermatogenesis: lesson from functional proteomic studies of azoospermia patients with sertoli cell only syndrome. J Proteomics. 75(10):2879–2891. doi:10.1016/j.jprot.2011.12.040.
- Lyabin DN, Eliseeva IA, Ovchinnikov LP. 2014. YB-1 protein: functions and regulation. Wiley Interdiscip Rev RNA. 5(1),95–110. doi: 10.1002/wrna.1200.
- Mageeney CM, Ware VC. 2019. Specialized eRpL22 paralogue-specific ribosomes regulate specific mRNA translation in spermatogenesis in Drosophila melanogaster. Mol Biol Cell. 30(17):2240–2253. doi:10.1091/mbc.E19-02-0086.
- Martin-Hidalgo D, Serrano R, Zaragoza C, Garcia-Marin LJ, Bragado MJ. 2020. Human sperm phosphoproteome reveals differential phosphoprotein signatures that regulate human sperm motility. J Proteomics. 215:103654. doi:10.1016/j.jprot.2020.103654.
- Neto FTL, Bach PV, Najari BB, Li PS, Goldstein M. 2016. Spermatogenesis in humans and its affecting factors. Semin Cell Dev Biol. 59:10–26. doi:10.1016/j.semcdb.2016.04.009.
- Nishimura H, L’Hernault SW. 2017. Spermatogenesis. Curr Biol. 27(18):R988–R994. doi:10.1016/j.cub.2017.07.067.
- Parapanov R, Nusslé S, Hausser J, Vogel P. 2008. Relationships of basal metabolic rate, relative testis size and cycle length of spermatogenesis in shrews (Mammalia, Soricidae). Reprod Fertil Dev. 20(3):431–439. doi:10.1071/RD07207.
- Phillips BT, Orwig KE. 2010. Regulation of the RNA binding protein YBX1 in mouse undifferentiated spermatogonia. Biol Reprod. (Suppl_1).
- Pini T, Parks J, Russ J, Dzieciatkowska M, Hansen KC, Schoolcraft WB, Katz-Jaffe M. 2020. Obesity significantly alters the human sperm proteome, with potential implications for fertility. J Assist Reprod Genet. 37(4):777–787. doi:10.1007/s10815-020-01707-8.
- Phillips BT, Orwig KE. 2010. Regulation of the RNA binding protein YBX1 in mouse undifferentiated spermatogonia. Biol Rep. (Suppl_1): Suppl_1. doi: 10.1093/biolreprod/83.s1.407.
- Ravikumar S, Srikumar K. 2005. Metabolic dysregulation and inhibition of spermatogenesis by gibberellic acid in rat testicular cells. J Environ Biol. 26(3):567–569.
- Swerdloff RS. 2017. Male reproductive physiology. Nihon Hinyokika Gakkai Zasshi. 81(20):1980–1981.
- Thacker S, Yadav SP, Sharma RK, Kashou A, Willard B, Zhang D, Agarwal A. 2011. Evaluation of sperm proteins in infertile men: a proteomic approach. Fertil Steril. 95(8):2745–2748. doi:10.1016/j.fertnstert.2011.03.112.
- Tollervey D, Lehtonen H, Jansen R, Kern H, Hurt EC. 1993. Temperature-sensitive mutations demonstrate roles for yeast fibrillarin in pre-rRNA processing, pre-rRNA methylation, and ribosome assembly. Cell. 72(3):443–457. doi:10.1016/0092-8674(93)90120-F.
- Tourmente M, Roldan ER. 2013. Metabolic rate limits the effect of sperm competition on mammalian spermatogenesis. Plos One. 8(9):e76510. doi:10.1371/journal.pone.0076510.
- Vloeberghs V, Verheyen G, Haentjens P, Goossens A, Polyzos N, Tournaye H. 2015. How Successful Is TESE-ICSI in Couples with Non-obstructive Azoospermia? Hum Reprod. 30(8):1790–1796.
- Weidensdorfer D, Stöhr N, Baude A, Lederer M, Köhn M, Schierhorn A, Buchmeier S, Wahle E, Hüttelmaier S. 2009. Control of c-myc mRNA stability by IGF2BP1-associated cytoplasmic RNPs. RNA. 15(1):104–115. doi: 10.1261/rna.1175909.
- Wajner SM, Wagner MS, Maia AL. 2009. Clinical implications of altered thyroid status in male testicular function. Arq Bras Endocrinol Metabol. 53(8):976–982. doi:10.1590/S0004-27302009000800011.
- Wolffe AP. 1994. Structural and functional properties of the evolutionarily ancient Y‐box family of nucleic acid binding proteins. Bioessays. 16(4):245–251. doi:10.1002/bies.950160407.
- Wu H, Sun L, Wen Y, Liu Y, Yu J, Mao F, Wang Y, Tong C, Guo X, Hu Z. 2016. Major spliceosome defects cause male infertility and are associated with nonobstructive azoospermia in humans. P Natl Acad Sci. 113(15):4134–4139. doi:10.1073/pnas.1513682113.
- Yugami M, Okano H, Nakanishi A, Yano M. 2020. Analysis of the nucleocytoplasmic shuttling RNA-binding protein HNRNPU using optimized HITS-CLIP method. PLoS ONE. 15(4):e0231450. doi: 10.1371/journal.pone.0231450.
- Yang Q, Li F, Miao Y, Luo X, Dai S, Liu J, Niu W, Sun Y. 2020. CdSe/ZnS quantum dots induced spermatogenesis dysfunction via autophagy activation. J Hazard Mater. 398:122327. doi:10.1016/j.jhazmat.2020.122327.
- Xiao R, Tang P, Yang B, Huang J, Zhou Y, Shao C, Li H, Sun H, Zhang Y, Fu X-D. 2012. Nuclear matrix factor hnRNP U/SAF-A exerts a global control of alternative splicing by regulating U2 snRNP maturation. Mol Cell. 45(5):656–668. doi: 10.1016/j.molcel.2012.01.009.
- Zhao C, Huo R, Wang F-Q, Lin M, Zhou Z-M, Sha J-H. 2007. Identification of several proteins involved in regulation of sperm motility by proteomic analysis. Fertil Steril. 87(2):436–438. doi:10.1016/j.fertnstert.2006.06.057.