ABSTRACT
The molecular composition of extracellular vesicles (EVs) is emerging as a novel biomarker in many areas of research including reproductive health. EVs transport biological molecules such as RNA and protein to facilitate cell-to-cell communication among cells of the male reproductive tract. Human and animal studies have shown that EVs present in seminal plasma or in the male reproductive tract contain important cargo that are important for successful reproductive outcomes. Small non-coding RNAs (sncRNA) have been at the forefront of this research, and as such, they have the potential to serve as novel biomarkers of male infertility diagnosis and reproductive success. This review provides an overview of EV biosynthesis and examines the molecular payloads of seminal plasma EVs on male infertility and reproductive success as well as future research that is warranted to examine how these molecular payloads may be modified by environmental factors.
Introduction
While much is known about male reproductive health, sperm development, and many causes of reproductive disorders, research pertaining to the mechanisms of sperm pathophysiology is still largely unknown. Global increases in male infertility and subfertility are major public health issue that threatens reproductive success. For instance, a recent meta-regression analysis identified a significant decline in sperm concentration (SC) and total sperm count (TSC) by analyzing 42,935 men from various studies which were published between 1973 and 2011. Between these years, among men from 50 western countries, SC and TSC declined 52.4% (−1.4% per year) and 59.3% (−1.6% per year), respectively (Levine et al. Citation2017). More remarkable, are the data from fertile men between 1977 and 2009 in which large decreases were observed in SC (from 83.8 million/ml to 62.0 million/mL, −0.81 per year) and TSC (from 277.4 million to 209.5 million, −0.76 million per year).
In male infertility assessment, semen analyses include parameters such as volume, pH, sperm concentration, total sperm count, morphology, and viability. Although semen analyses have been conducted since the 1930s as a diagnostic for male infertility at the macroscopic and microscopic level, causes of 10–20% of infertility cases are still unknown. Therefore, molecular approaches to semen analyses may provide new biomarkers for the assessment of male infertility and prognostic indicator of couples’ reproductive success.
This review aims to summarize the function of EVs (e.g., exosomes and microvesicles) in sperm maturation and development while in transit through the male reproductive tract. We will first briefly review EV biogenesis, and then review the involvement of EVs in the male reproductive tract, focusing on their molecular cargo as potential novel biomarkers of male fertility and environmental conditions.
Exosomes and microvesicles: brief history and biogenesis
EVs are nanometer-scale lipid bilayer-enveloped vesicles that are secreted from cells into extracellular space and biofluids. While EVs were first described in eukaryotic cells, they have also been identified in prokaryotic cells (Yanez-Mo et al. Citation2015; Yang et al. Citation2017). The size and content of EVs are highly heterogeneous and are dependent on environmental conditions and cell-type. Classification of EVs is now routinely separated into three main subgroups: 1) apoptotic bodies (1 − 5 µm in size); 2) microvesicles (also referred to ectosomes or microparticles; 100–1000 nm); and 3) exosomes (30–100 nm) (Yanez-Mo et al. Citation2015). Given apoptotic bodies are vesicles released during cell death, this review will focus on microvesicles and exosomes.
EVs were first described by Chargaff and West in 1946 as platelet-derived particles in blood serum, later referred to as platelet dust (Chargaff and West Citation1946). In the 1970s, EVs released from the plasma membrane were observed in various cells and body fluids including seminal plasma (Ronquist et al. Citation1978). In 2007, a major breakthrough for the biological function of EVs was reported through an exquisite set of experiments. Valadi et al. detected the presence of mRNA and microRNA (miRNA) in EVs derived from mouse and human cell lines. They also described ‘exosomal shuttle RNA’ in which mouse exosomal RNA was transferred to human mast cells producing new mouse proteins in the recipient cells (Valadi et al. Citation2007). Since this discovery and others of the time (Ratajczak et al. Citation2006; Skog et al. Citation2008; Yanez-Mo et al. Citation2015), EVs have been recognized as dynamic vehicles of intercellular communication. A single cell-type can release different subclasses of EVs. The distinctions between exosomes and microvesicles will be discussed in the following sections.
Exosomes
The nomenclature ‘exosome’ was initially used to describe exfoliated membrane vesicles from two distinct populations of vesicles that ranged from 500 to 1,000 nm and 40 nm in size (Trams et al. Citation1981). Trams et al. also proposed that these vesicles are not mere by-products of cells but likely serve a physiologic function. In 1987, exosomes were further refined to describe smaller vesicles (40–150 nm) that originated through endocytosis of proteins and formation of multivesicular bodies (MVBs) during reticulocyte differentiation (Johnstone et al. Citation1987). Since their discovery, exosomes are shown to be released by several broad classes of cells including but not limited to immune cells, neurons, stem cells, and epithelial cells and are now more narrowly defined by vesicles with a diameter of 30–100 nm (Thery et al. Citation2009; Pegtel and Gould Citation2019). Furthermore, vesicles with characteristic exosomal features have been extracted from various body fluids including semen ()) (Park et al. Citation2011; Aalberts et al. Citation2012).
Figure 1. EV structure and biogenesis. (A) Exosome image cryo-electron microscopy image of a seminal plasma EV isolated by the differential ultracentrifuge. (B) Exosome biogenesis. Proteins released from the trans-Golgi network and RNA molecules destined to the plasma membrane shed as microvesicles. Exosomes originate from Multivesicular bodies (MVBs). Endosomes mature into MVBs and intravaginal budding results in the formation of intraluminal vesicles (ILVs). In some cases, MVB fuse into the plasma membrane and release ILVs to the extracellular region as exosomes. (C) Exosome structure. Exosomes have protein and RNA that play a critical role in intercellular communication. Tetraspanins (CD9, CD63, CD81, CD82) and Heat-shock proteins (HSP70, HSP90) are distinct proteins commonly used for exosome characterization. Signaling proteins (EGFR, PI3K, HIF-1α, β- Catenin) and MVB proteins are sourced from inward budding of ILVs. Exosomes also have some proteins (MHC I) and lipids (cholesterol, sphingolipids, ceramides) from cellular membrane and RNA cargo (mRNA and miRNA) which function as a post-transcriptional alteration in gene expression
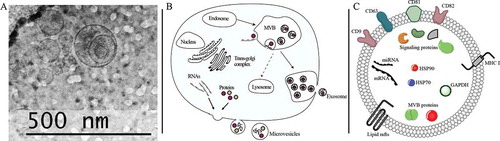
One main distinction between exosome and microvesicles is their biogenesis, which has been extensively reviewed elsewhere (Pegtel and Gould Citation2019). The main route of exosome biogenesis involves the formation of endosomal structures, known as MVBs. First, the inner cell membrane invaginates and buds into the lumen of the MVB to form intraluminal vesicles (ILVs) ()). These ILVs are formed by endosomal sorting complexes required for transport (ESCRT), which are made up of four multiprotein complexes (ESCRT 0, I, II, and III) each owing a distinct role in MVB formation essential to this process (reviewed in Babst Citation2011). The molecular composition of exosomes is formed as a result of the ILVs construction. The MVBs membrane is highly enriched with a class of proteins called tetraspanins (Pols and Klumperman Citation2009). Tetraspanins have a four transmembrane domain that configures a stereotypical tertiary structure and are targeted for the characterization and isolation of exosomes. Four tetraspanins are thought to be involved in exosome formation: CD9, CD63, CD81, and CD82 (Jansen et al. Citation2009; Kosaka et al. Citation2010; Lu et al. Citation2017) ()). Exosomes are highly abundant in membrane proteins, signaling proteins, heat shock proteins, MVB proteins, and proteins involved in vesicle trafficking (Du et al. Citation2016; Bai et al. Citation2018). In the final step of biogenesis, MVBs fuse with the plasma membrane and release ILVs into the extracellular space as exosomes. It must also be mentioned that while the endosomal-directed biogenesis of exosomes is widely accepted as the only biogenesis mechanism, multiple lines of evidence suggest that exosome can also bud from the plasma membrane (reviewed in Pegtel and Gould Citation2019).
Microvesicles
Although exosomes and microvesicles (also referred to ectosomes) are structurally similar, microvesicles differ in their size (100–1000 nm) and biogenesis. While exosomes utilize an endosomal-mediated mechanism, microvesicles are formed from the direct outward budding from the plasma membrane to the extracellular space. Microvesicle biogenesis is the result of two dynamic intracellular processes: phospholipid redistribution and cytoskeletal protein contraction ()). The phospholipids and proteins are not uniformly distributed within the plasma membrane; as a result, they form microdomains. Aminophospholipid translocases are proteins that regulate the asymmetric distribution of the molecules within the membrane. For instance, flippases and floppases enable lipid movement to the inner and outer membrane, respectively, while scramblases allow bidirectional lipid movement (Hugel et al. Citation2005). Finally, phosphatidylserine translocation induces membrane budding and actin–myosin interactions to complete the budding process (Akers et al. Citation2013). Although the molecular content of the microvesicles is dependent upon the cell type of origin, protein content is common to most microvesicles and includes cytoplasmic proteins such as tubulin, cell structure proteins (e.g. actin) as well as signal transduction and transcription proteins (Raposo and Stoorvogel Citation2013). Although the biogenesis of microvesicles is widely accepted to be endosomal-independent, some overlap of the protein machinery used for exosome formation (e.g., ESCRT complexes and TSG101) may also play a role in microvesicle biogenesis (Nabhan et al. Citation2012; Raposo and Stoorvogel Citation2013).
EVs in the male reproductive tract
Sperm acquire their motility and fertilizing ability during epididymal transit; yet studies investigating epididymal functions and how they may relate to male infertility are scarce. EVs in the epididymis (e.g., epididymosomes), prostate (e.g., prostasomes) and other secondary male sex organs are promising research areas to aid in the understanding of sperm physiology and male reproductive health. As this review will discuss further in this section, EVs are important facilitators of sperm development and have implications for reproductive success.
Seminal plasma
One important factor not considered in normal semen analysis is the constituents found in seminal plasma. In humans for example, seminal plasma fluid constitutes >90% of semen and is comprised mostly secretions from accessory sex glands of the male reproductive system (Yuan et al. Citation2009). The pair of seminal vesicles is positioned lateral to the vas deferens and together they produce ~65% of seminal fluid, which is released into the ejaculatory duct. Also, prostatic fluid comprises ~25% of the semen, whereas secretions from the bulbourethral gland and numerous periurethral glands contribute to ~2% of the ejaculated semen. Seminiferous tubules and epididymis secretions including sperm cells and estimated to comprise 8–10% of semen volume (Drabovich et al. Citation2014).
Seminal plasma is a medium used to transit, protect, and modulate sperm function after ejaculation up to fertilization. The composition of seminal plasma contains fructose, inorganic ions, cell-free RNA, and miRNA as well as proteins involved in post-testicular sperm maturation and maintenance (Samanta et al. Citation2018). Slightly alkaline seminal plasma provides a protective milieu for sperm survival in acidic vaginal surroundings. Seminal fluid also facilitates the activation of the endometrial genes and immune cells which is critical for appropriate implantation to ensure pregnancy and healthy offspring (Camargo et al. Citation2018). Furthermore, there is a growing interest in investigating the role of seminal plasma to improve assisted reproduction outcomes. Intercourse during the peri-transfer period of an IVF cycle has been shown to increase pregnancy rates since seminal plasma is identified to promote implantation and embryo development (Tremellen et al. Citation2000). Friedler et al. reported that intravaginal seminal plasma application after transvaginal ovum retrieval reached higher implantation and clinical pregnancy rates (36.9%) when compared to the placebo group (29.1%) (Friedler et al. Citation2013). Similarly, in another prospective randomized control trial of participants undergoing thawed embryo transfer, Tremellen et al. compared the success rate between the women who engaged in vaginal semen exposure and those who abstained. In clinical pregnancy rates, embryo viability after 6–8 weeks was significantly higher in the exposed group, indicating that vaginal semen exposure around the time of embryo transfer enhances early implantation rates and embryo development (Tremellen et al. Citation2000).
Seminal plasma EVs
In addition to the attributes of seminal plasma described above, seminal plasma also contains a heterogeneous population of EVs, which function in sperm development, maturation and may play a key role in early-life development. Seminal plasma has a high concentration of EVs (~1012 particles/ejaculate) released from various epithelial cells in the male reproductive system (Yang et al. Citation2017). The EVs in seminal plasma were initially identified in the late 1970s (Ronquist et al. Citation1978) and were first referred to as prostasomes by Ronquiest and Brody in 1985, describing vesicles secreted from the acinar epithelial cell of the human prostate (Ronquist and Brody Citation1984). Prostasomes represent a heterogeneous population of EVs with a mean diameter of 150 nm (range 30–200) and have similar characteristics of exosomes in terms of their buoyant density, size, and presence of characteristic exosome markers (Ronquist Citation2015).
In addition to the presence of prostasomes, EVs originating from the epididymis (referred to as epididymosomes) have been identified in human seminal fluid. Sperm are initially non-motile when they exit the seminiferous tubules of the testes and undergo maturation process during their passage in the epididymis (which can last up to 14 days). The epididymis is a coiled structure (4–6 m in length), which is positioned posterior to the surface of the testes and bridges the efferent ductus and vas deferens. It is divided into three main regions: proximal caput (head), elongated corpus (body), and distal cauda (tail), where sperm are ultimately stored until ejaculation. The epididymis provides an alkaline intraluminal environment to stimulate sperm motility. The complexity of epididymal intraluminal secretions arises from absorptive activity, secretory activity, and more importantly, bi-directional vesicle trafficking (Hermo et al. Citation2019).
Epididymosomes were first identified from hamster epididymis in the mid-1980s and were found to interact with the sperm plasma membrane in the epididymal lumen (Yanagimachi et al. Citation1985). These vesicles were later described from various species including mice, rats, bulls, rams, and men (Sullivan and Saez Citation2013). Similar to prostasomes, epididymosomes are derived from epithelial cells of the epididymis, are heterogeneous population of vesicles ranging in size from 50 to 250 nm vesicles (Sullivan and Saez Citation2013; Sullivan Citation2015). Epididymosomes have been studied from different segments of the epididymis (caput, corpus, and cauda) and proteins in the epididymal intraluminal compartment have been found to be involved in sperm maturation. When epididymosomes are incubated with spermatozoa in vitro, some epididymosome-associated proteins are transferred into the gamete cell, and researchers found that this process was dependent on temperature, pH, and cations, in particular Zn2+ (Frenette et al. Citation2002). Additionally, localization of transferred proteins was also found to be enriched at the acrosome (Frenette et al. Citation2002). While it is certainly feasible that other secondary male accessory glands contribute EVs to seminal plasma, these are less characterized compared to the aforementioned prostasomes and epididymosomes.
Biological Function of EVs in the male reproductive tract
Animal and in vitro studies
Since spermatozoa are transcriptionally and translationally inert cells lacking motility after spermatogenesis, EVs in the male reproductive tract have been shown to play a pivotal role in post-testicular sperm maturation and fertilization capacity. As early as the early 1990s, the importance of prostasomes was reported with studies showing that inclusion of prostasomes in swim-up media improved the recovery of hyperactive motile sperm (Fabiani et al. Citation1994). In 2003, Palmerini et al. reported that fusion of prostasomes to human spermatozoa increased the sensitivity of sperm to the effect of progesterone on the induction of the acrosome reaction (Palmerini et al. Citation2003). Subsequent work by Park et al. found that prostasomes fusion led to the transfer of calcium signaling tools, such as progesterone receptors and cyclic adenosine diphosphoribose (cADPR), from the prostasomes to the sperm neck enhancing calcium signaling and motility, while the cADPR agonist reduced sperm motility and fertilization rates, indicating that prostasomes enhance calcium signaling in sperm (Park et al. Citation2011). Additionally, utilizing biotin-labeled prostasomes from stallion, prostasomes were found to selectively bind to the sperm head only after initiation of capacitation, suggesting that sperm cells only fuse prostasomes during the final approach to the oocyte (Aalberts et al. Citation2013).
The beneficial effect of prostasomes described above has also been shown in boar seminal plasma EVs. Post-ejaculated sperm incubated with EVs was shown to mediate the transfer of EV-associated proteins and impair capacitation, the latter suggesting a stabilizing role of EVs from potential premature capacitation of sperm (Piehl et al. Citation2013). Moreover, Du et al. reported that seminal plasma EVs had a beneficial effect on sperm characteristics upon long-term liquid storage. Samples stored with a higher concentration of exosomes had increased sperm motility, prolonged survival, enhanced sperm membrane integrity, and higher total antioxidant capacity after thawing the samples while preventing premature capacitation (Du et al. Citation2016).
More recently, research has intensified in the area of epididymosomes in mediating intercellular communication during sperm transit through the epididymis. Given the distinct segments and accompanying microenvironments of the epididymis, it is not surprising that data indicate novel RNA and protein profiles with each segment. For instance, after leaving the testis, sperm interact with epididymal EVs that promote their maturation by facilitating dynamic shifts in miRNA profiles, such that a loss and gain of 113 and 115 miRNAs were observed in mice sperm collected from the caput and cauda epididymal segments, respectively (Nixon et al. Citation2015). Similar to sperm miRNA profiles, subsequent work found distinct miRNA profiles (a total of >350 miRNAs) in mouse epididymosomes isolated at different segments of the epididymis (Reilly et al. Citation2016). This group also provides direct evidence for the transfer of miRNA cargo to spermatozoa. After co-incubation with labeled epididymosomes, 30% of the sperm cells were tagged with a distinct preference for live sperm, in which labeling concentrated in the head and mid-piece. Moreover, sperm incubated with epididymosomes revealed a significant increase in five specific miRNAs (miR-191, miR-375, miR-467a, miR-467d, miR-467e) that were abundantly present in epididymosomes compared to sperm not exposed to epididymosomes (Reilly et al. Citation2016).
Not surprisingly, proteomic studies of mouse epididymosomes also revealed distinct protein profiles in epididymosomes from different segments of the epididymis. A total of 1640 proteins were identified in mouse epididymosomes; however, 146 proteins were differentially accumulated between the caput and corpus epididymosomes, while 344 were differentially accumulated between corpus and cauda epididymosomes. Interestingly, those proteins that were not routinely found in exosomes from other tissue and were specific to epididymosomes were enriched in categories associated with sperm function such a fertilization and binding to the zona pellucida, indicating that protein payloads in epididymosomes have a specific physiological function in post-testicular sperm function (Nixon et al. Citation2019).
A series of studies by Sullivan and colleagues in bovine has shown that epididymosomes can be classified into two distinct populations. The first represents CD-9 positive epididymosomes, which have been shown to preferentially bind and fuse with live spermatozoa, suggesting that only those sperm that will survive the epididymal transit will be targeted for transfer of the transfer of protein and RNA cargo (Caballero et al. Citation2013). The second population of epididymosomes is enriched in epididymal sperm binding protein 1 (ELSPBP1) and display a higher affinity for dead sperm (D’Amours et al. Citation2012). Thus, it has been hypothesized that they may play a role in the protection against the detrimental effects of oxidative stress on bovine sperm (Sullivan Citation2015).
Finally, it is well known that human seminal fluid has immunosuppressive agents to maximize the chance of fertilization (Bromfield Citation2014). Thus, the role of seminal plasma EVs may not be limited to sperm capacitation, motility, and protection against oxidative stress but also immune tolerance. For instance, Bai et al., utilizing porcine endometrial epithelial cells (EECs), showed that treatment of EECs with isolated EVs from the sperm-rich fraction of boar semen resulted in global immune response (Bai et al. Citation2018). RNA profiling of the treated EECs displayed a significant upregulation of gene expression related to immune and inflammatory response, whereas metabolic pathways and T-cell differentiation genes were downregulated. These expression profiles in cell culture were replicated in RNAseq analysis from endometrium cells from naturally mated pig. Collectively, these data in animal models demonstrate that EVs in seminal plasma and/or the male reproductive tract play a supportive role in sperm maturation, vitality, protein and RNA cargo delivery, and immune tolerance in the female reproductive tract.
Human studies
Despite advancing our understanding of the role of EVs on male reproductive health in animal models, research on the function of EVs in human seminal plasma has been limited. However, emerging research supports the potential utility of seminal plasma EVs and their cargo as novel opportunities to detect male infertility and improve reproductive health outcomes. For instance, among a study of oligoasthenozoospermic subfertile men and controls, miRNA profiling of seminal plasma EVs identified 36 differentially expressed miRNAs, which were enriched in numerous biological pathways including Wnt, MAPK, and mTOR signaling (Abu-Halima et al. Citation2016). Similarly, distinct miRNA profiles (60 differentially expressed miRNAs) were found in seminal plasma EVs of secretory or obstructive azoospermic men compared to controls, of which 12 miRNAs, were differentially expressed between secretory and obstructive azoospermic patients (Barceló et al. Citation2018). Interestingly, one miRNA, miR-31-5p, showed promising diagnostic success (AUC > 0.95) in the determination of the cause of the patient azoospermia (secretory vs obstructive) with high sensitivity and specificity (>90% for each).
More recently, distinct proteomic profiles were detected in seminal plasma of infertile men with unilateral varicocele compared to proven fertile donors. Of particular relevance were the findings that differentially expressed proteins were enriched in networks for EV function. It was observed that seminal plasma of varicocele patients, compared to controls, had increased expression of ANXA2, known for its role in membrane trafficking and fusion, and decreased expression of KIF5B, which supports the release of EVs (Panner Selvam et al. Citation2019). In support of findings in animal models discussed above, a recent study also indicates that seminal plasma EVs may improve sperm function. For example, EVs from seminal plasma of normo-, astheno-, and azoospermic patients displayed similar morphology, expression of canonic exosome markers, and proteins involved in sperm maturation and fertilization capacity. Interestingly, seminal plasma EVs from normozoospermic but not from asthenozoospermic males improved sperm motility and initiated capacitation, possibly mediated by the transfer of cysteine-rich secretory protein 1. Importantly, sperm were able to take up exosomes after ejaculation, suggesting that exposing sperm of asthenozoospermic men to normal seminal plasma EVs may improve reproductive success (Murdica et al. Citation2019).
Lastly, seminal plasma EVs have been shown to harbor a diverse set of RNA. RNA-sequencing revealed that in addition to mRNA and miRNA, exosomes contain a variety of other small non-coding RNAs (sncRNA) including tRNA, Y RNA, piwi-RNA, and ribosomal RNA (Vojtech et al. Citation2014). The repertoire of small RNAs isolated from seminal plasma exosomes was found at unexpectedly high concentrations, as data from both blood plasma and cell culture supernatant have shown most extracellular RNAs are independent of exosomes (Vojtech et al. Citation2014). These findings highlight the uniqueness of seminal plasma EV and the potential biological significance of their molecular composition as potential biomarkers for male fertility and reproductive success.
Can environmental conditions affect EV cargo composition?
Due to their role in intercellular communication, the molecular composition of EVs is uniquely suited to be novel biomarkers not only of male fertility and reproductive success but also of environmental factors that are encountered during sensitive periods of development, such as the spermatogenesis and epididymal maturation. While epididymal sperm maturation is directed at the acquisition of fertilization potential, EV cargo payloads may also provide the final opportunity for sperm to ‘epigenetically match’ their current intercellular environment prior to fertilization (Wu et al. Citation2015). These environmental cues generated by cells of the epididymis and other secondary sex organs during ejaculation may play a role in the trajectory of early-life development.
The majority of this line of research stems from mouse studies examining the effects of nutritional manipulation on the acquisition of sperm sncRNA and subsequent early-life development and offspring phenotype. For instance, low-protein diet (LPD) in male mice resulted in corresponding changes in small RNA profiles in epididymosomes and mature sperm (Sharma et al. Citation2016), suggesting the interaction and transfer of EV payloads to sperm during epididymal transit. Interestingly, these LPD-induced changes in small RNA profiles most notably affected the let-7 family of miRNAs and tRNA fragments, which have been reported to be in high concentrations in seminal plasma EV of humans (Vojtech et al. Citation2014). Furthermore, the change in sncRNA profiles induced by LPD affected preimplantation embryo development, such that EV-derived tRNAs isolated from sperm of LPD males and injected into zygotes resulted in delays in embryo development (Sharma et al. Citation2016). Lastly, cauda sperm, but not caput sperm, and their associated sncRNA payloads were necessary for successful embryo implantation, thereby further highlighting the importance of sperm sncRNA acquisition from EVs during epididymal transit (Conine et al. Citation2018).
Most intriguing are the results from Chen and colleagues (Chen et al. Citation2016) who have demonstrated that modifications to RNA nucleotides of sncRNA (e.g., epitranscriptome) may be as important as the transcript itself in affecting early-life development and the inheritance of paternally acquired traits. High-fat diets (HFD) in male mice resulted in changes in sperm 5ʹ tRNA fragment profiles and metabolic disorders in F1 offspring. Sperm tRNA fragments, which were primarily methylated at the 5th-positioned cytosine, recapitulated the metabolic disorders in F1 offspring when injected into normal zygotes. However, synthesized tRNA fragments, which did not contain such modifications, were quickly degraded and were unable to generate metabolic disorders in offspring. While this study does not examine epididymosomal sncRNA, it underscores the need for future EV research to examine the epitranscriptome in concert with transcript abundance.
Future directions
Despite major advances in understanding the molecular and cellular functions in sperm, the diagnosis of male infertility has not changed for 50 years. A major limitation of semen parameters is that they are relatively poor predictors of reproductive success and have a minimal association with fecundability (Jungwirth et al. Citation2012; Buck Louis et al. Citation2014). Accelerating our knowledge of EV molecular payloads in the male reproductive tract may provide a promising biomarker and facilitate our understanding of the pathways involved in the etiology of male factor infertility. Furthermore, compelling animal studies discussed above demonstrate that EV cargo of epididymosomes are responsive to environmental factors and can influence reproductive outcomes. Moving forward, prospective studies are warranted to examine the relationships of EV cargo in human seminal plasma with reproductive outcomes and environmental factors, such as diet, stress, and environmental toxicant exposures. In this manner, EV cargo profiles of seminal plasma may have diagnostic potential to inform clinical care and treatment and provide opportunities for novel therapeutic targets. Lastly, such research will furnish an environmental roadmap to discern which individual exposures, or their mixtures, are driving aberrant profiles of EV cargo that will afford population-wide prevention efforts.
Authors' contributions
Conceived and planned writing: AA and JRP; writing of first draft, gathering, and interpreting cited references: AA; writing of first and second draft, gathering, and interpreting cited references: EH, JRP.
Disclosure statement
The authors report no conflict of interest.
Additional information
Funding
References
- Aalberts M, Sostaric E, Wubbolts R, Wauben MWM, Nolte-’T Hoen ENM, Gadella BM, Stout TAE, Stoorvogel W. 2013. Spermatozoa recruit prostasomes in response to capacitation induction. 1834(11):2326–2335. doi:10.1016/j.bbapap.2012.08.008.
- Aalberts M, van Dissel-emiliani FM, van Adrichem NP, van Wijnen M, Wauben MH, Stout TA, Stoorvogel W. 2012. Identification of distinct populations of prostasomes that differentially express prostate stem cell antigen, annexin A1, and GLIPR2 in humans. Biol Reprod. 86(3):82. doi:10.1095/biolreprod.111.095760.
- Abu-Halima M, Ludwig N, Hart M, Leidinger P, Backes C, Keller A, Hammadeh M, Meese E. 2016. Altered micro-ribonucleic acid expression profiles of extracellular microvesicles in the seminal plasma of patients with oligoasthenozoospermia. Fertil Steril. 106(5):1061–1069.e1063. doi:10.1016/j.fertnstert.2016.06.030.
- Akers JC, Gonda D, Kim R, Carter BS, Chen CC. 2013. Biogenesis of extracellular vesicles (EV): exosomes, microvesicles, retrovirus-like vesicles, and apoptotic bodies. J Neurooncol. 113(1):1–11. doi:10.1007/s11060-013-1084-8.
- Babst M. 2011. MVB vesicle formation: ESCRT-dependent, ESCRT-independent and everything in between. Curr Opin Cell Biol. 23(4):452–457. doi:10.1016/j.ceb.2011.04.008.
- Bai R, Latifi Z, Kusama K, Nakamura K, Shimada M, Imakawa K. 2018. Induction of immune-related gene expression by seminal exosomes in the porcine endometrium. Biochem Biophys Res Commun. 495(1):1094–1101. doi:10.1016/j.bbrc.2017.11.100.
- Barceló M, Mata A, Bassas L, Larriba S, Nicolau M. 2018. Exosomal microRNAs in seminal plasma are markers of the origin of azoospermia and can predict the presence of sperm in testicular tissue. Human Reprod (Oxford, England). 33. doi:10.1093/humrep/dey072.
- Bromfield JJ. 2014. Seminal fluid and reproduction: much more than previously thought. 31(6):627–636.
- Buck Louis GM, Sundaram R, Schisterman EF, Sweeney A, Lynch CD, Kim S, Maisog JM, Gore-Langton R, Eisenberg ML, Chen Z. 2014. Semen quality and time to pregnancy: the longitudinal investigation of fertility and the environment study. Fertil Steril. 101(2):453–462. doi:10.1016/j.fertnstert.2013.10.022.
- Caballero JN, Frenette G, Belleannée C, Sullivan R. 2013. CD9-positive microvesicles mediate the transfer of molecules to bovine spermatozoa during epididymal maturation. PLOS ONE 8(6):e65364.
- Camargo M, Intasqui P, Bertolla RP. 2018. Understanding the seminal plasma proteome and its role in male fertility. Basic Clin Androl. 28:6. doi:10.1186/s12610-018-0071-5.
- Chargaff E, West R. 1946. The biological significance of the thromboplastic protein of blood. J Biol Chem. 166(1):189–197.
- Chen Q, Yan M, Cao Z, Li X, Zhang Y, Shi J, Feng G-H, Peng H, Zhang X, Zhang Y, et al. 2016. Sperm tsRNAs contribute to intergenerational inheritance of an acquired metabolic disorder. Science. 351(6271):397–400. doi:10.1126/science.aad7977.
- Conine CC, Sun F, Song L, Rivera-Pérez JA, Rando OJ. 2018. Small RNAs gained during epididymal transit of sperm are essential for embryonic development in mice. Dev Cell. 46(4):470–480.e473. doi:10.1016/j.devcel.2018.06.024.
- D’Amours O, Frenette G, Bordeleau LJ, Allard N, Leclerc P, Blondin P, Sullivan R. 2012. Epididymosomes transfer epididymal sperm binding protein 1 (ELSPBP1) to dead spermatozoa during epididymal transit in bovine. Biol Reprod. 87(4): 94-94. doi:10.1095/biolreprod.112.100990.
- Drabovich AP, Saraon P, Jarvi K, Diamandis EP. 2014. Seminal plasma as a diagnostic fluid for male reproductive system disorders. Nat Rev Urol. 11(5):278–288. doi:10.1038/nrurol.2014.74.
- Du J, Shen J, Wang Y, Pan C, Pang W, Diao H, Dong W. 2016. Boar seminal plasma exosomes maintain sperm function by infiltrating into the sperm membrane. Oncotarget. 7(37):58832–58847. doi:10.18632/oncotarget.11315.
- Fabiani R, Johnsson L, Lundkvist Ö, Ronquist G. 1994. Enhanced recruitment of motile spermatozoa by prostasome inclusion in swim-up medium. Hum Reprod. 9(8):1485–1489. doi:10.1093/oxfordjournals.humrep.a138735.
- Frenette G, Lessard C, Sullivan R. 2002. Selected proteins of “prostasome-like particles” from epididymal cauda fluid are transferred to epididymal caput spermatozoa in bull. 67(1):308–313. doi:10.1095/biolreprod67.1.308.
- Friedler S, Ben-Ami I, Gidoni Y, Strassburger D, Kasterstein E, Maslansky B, Komarovsy D, Bern O, Ron-El R, Raziel A. 2013. Effect of seminal plasma application to the vaginal vault in in vitro fertilization or intracytoplasmic sperm injection treatment cycles-a double-blind, placebo-controlled, randomized study. J Assist Reprod Genet. 30(7):907–911. doi:10.1007/s10815-013-0033-y.
- Hermo L, Oliveira RL, Smith CE, Au CE, Bergeron JJM. 2019. Dark side of the epididymis: tails of sperm maturation. Andrology. doi:10.1111/andr.12641
- Hugel B, Martinez MC, Kunzelmann C, Freyssinet JM. 2005. Membrane microparticles: two sides of the coin. Physiology (Bethesda). 20:22–27. doi:10.1152/physiol.00029.2004.
- Jansen FH, Krijgsveld J, van Rijswijk A, van den Bemd GJ, van den Berg MS, van Weerden WM, Willemsen R, Dekker LJ, Luider TM, Jenster G. 2009. Exosomal secretion of cytoplasmic prostate cancer xenograft-derived proteins. Mol Cell Proteomics. 8(6):1192–1205. doi:10.1074/mcp.M800443-MCP200.
- Johnstone RM, Adam M, Hammond JR, Orr L, Turbide C. 1987. Vesicle formation during reticulocyte maturation. Association of plasma membrane activities with released vesicles (exosomes). J Biol Chem. 262(19):9412–9420.
- Jungwirth A, Giwercman A, Tournaye H, Diemer T, Kopa Z, Dohle G, Krausz C. 2012. European association of urology guidelines on male fertility: the 2012 update. Eur Urol. 62(2):324–332. doi:10.1016/j.eururo.2012.04.048.
- Kosaka N, Iguchi H, Yoshioka Y, Takeshita F, Matsuki Y, Ochiya T. 2010. Secretory mechanisms and intercellular transfer of microRNAs in living cells. J Biol Chem. 285(23):17442–17452. doi:10.1074/jbc.M110.107821.
- Levine H, Jorgensen N, Martino-Andrade A, Mendiola J, Weksler-Derri D, Mindlis I, Pinotti R, Swan SH. 2017. Temporal trends in sperm count: a systematic review and meta-regression analysis. Hum Reprod Update. 23(6):646–659.
- Lu J, Li J, Liu S, Wang T, Ianni A, Bober E, Braun T, Xiang R, Yue S. 2017. Exosomal tetraspanins mediate cancer metastasis by altering host microenvironment. Oncotarget. 8(37):62803–62815. doi:10.18632/oncotarget.19119.
- Murdica V, Giacomini E, Alteri A, Bartolacci A, Cermisoni GC, Zarovni N, Papaleo E, Montorsi F, Salonia A, Viganò P, et al. 2019. Seminal plasma of men with severe asthenozoospermia contain exosomes that affect spermatozoa motility and capacitation. Fertil Steril. 111(5):897–908.e892. doi:10.1016/j.fertnstert.2019.01.030.
- Nabhan JF, Hu R, Oh RS, Cohen SN, Lu Q. 2012. Formation and release of arrestin domain-containing protein 1-mediated microvesicles (ARMMs) at plasma membrane by recruitment of TSG101 protein. Proc Natl Acad Sci. 109(11):4146–4151. doi:10.1073/pnas.1200448109.
- Nixon B, De Iuliis GN, Hart HM, Zhou W, Mathe A, Bernstein IR, Anderson AL, Stanger SJ, Skerrett-Byrne DA, Jamaluddin MFB, et al. 2019. Proteomic profiling of mouse epididymosomes reveals their contributions to post-testicular sperm maturation. Mol Cell Proteomics. 18(Supplement 1):S91–S108. doi:10.1074/mcp.RA118.000946.
- Nixon B, Stanger SJ, Mihalas BP, Reilly JN, Anderson AL, Tyagi S, Holt JE, McLaughlin EA. 2015. The MicroRNA signature of mouse spermatozoa is substantially modified during epididymal maturation1. Biol Reprod. 93(4): 91-91. doi:10.1095/biolreprod.115.132209.
- Palmerini CA, Saccardi C, Carlini E, Fabiani R, Arienti G. 2003. Fusion of prostasomes to human spermatozoa stimulates the acrosome reaction. Fertil Steril. 80(5):1181–1184. doi:10.1016/s0015-0282(03)02160-5.
- Panner Selvam MK, Agarwal A, Sharma R, Samanta L, Gupta S, Dias TR, Martins AD. 2019. Protein fingerprinting of seminal plasma reveals dysregulation of exosome-associated proteins in infertile men with unilateral varicocele. World J Men’s Health. doi:10.5534/wjmh.180108.
- Park KH, Kim BJ, Kang J, Nam TS, Lim JM, Kim HT, Park JK, Kim YG, Chae SW, Kim UH. 2011. Ca2+ signaling tools acquired from prostasomes are required for progesterone-induced sperm motility. Sci Signal. 4(173):ra31. doi:10.1126/scisignal.2001595.
- Pegtel DM, Gould SJ. 2019. Exosomes. Annu Rev Biochem. 88(1):487–514. doi:10.1146/annurev-biochem-013118-111902.
- Piehl LL, Fischman ML, Hellman U, Cisale H, Miranda PV. 2013. Boar seminal plasma exosomes: effect on sperm function and protein identification by sequencing. Theriogenology. 79(7):1071–1082. doi:10.1016/j.theriogenology.2013.01.028.
- Pols MS, Klumperman J. 2009. Trafficking and function of the tetraspanin CD63. Exp Cell Res. 315(9):1584–1592. doi:10.1016/j.yexcr.2008.09.020.
- Raposo G, Stoorvogel W. 2013. Extracellular vesicles: exosomes, microvesicles, and friends. J Cell Biol. 200(4):373–383. doi:10.1083/jcb.201211138.
- Ratajczak J, Miekus K, Kucia M, Zhang J, Reca R, Dvorak P, Ratajczak MZ. 2006. Embryonic stem cell-derived microvesicles reprogram hematopoietic progenitors: evidence for horizontal transfer of mRNA and protein delivery. Leukemia. 20(5):847–856. doi:10.1038/sj.leu.2404132.
- Reilly J, McLaughlin E, Stanger S, Anderson A, Hutcheon K, Church K, Mihalas B, Tyagi S, Holt J, Eamens A, et al. 2016. Characterisation of mouse epididymosomes reveals a complex profile of microRNAs and a potential mechanism for modification of the sperm epigenome. Sci Rep. 6. doi:10.1038/srep31794.
- Ronquist G. (2015) Prostasomes: Their Characterisation: Implications for Human Reproduction. In: Bronson R. (eds) The Male Role in Pregnancy Loss and Embryo Implantation Failure. Advances in Experimental Medicine and Biology, vol 868. Springer, Cham. https://doi.org/10.1007/978-3-319-18881-2_9
- Ronquist G. 2015. Prostasomes: their characterisation: implications for human reproduction. Springer International Publishing; p. 191–209.
- Ronquist G, Brody I. 1984. The prostasome: its secretion and function in man. Biochimica et Biophysica Acta (BBA). 822(2):203–218. doi:10.1016/0304-4157(85)90008-5.
- Ronquist G, Brody I, Gottfries A, Stegmayr B. 1978. An Mg2+ and Ca2+-stimulated adenosine triphosphatase in human prostatic fluid: part II. Andrologia. 10(6):427–433. doi:10.1111/j.1439-0272.1978.tb03064.x.
- Samanta L, Parida R, Dias TR, Agarwal A. 2018. The enigmatic seminal plasma: a proteomics insight from ejaculation to fertilization. Reprod Biol Endocrinol. 16(1):41. doi:10.1186/s12958-018-0358-6.
- Sharma U, Conine CC, Shea JM, Boskovic A, Derr AG, Bing XY, Belleannee C, Kucukural A, Serra RW, Sun F, et al. 2016. Biogenesis and function of tRNA fragments during sperm maturation and fertilization in mammals. Science. 351(6271):391–396. doi:10.1126/science.aad6780.
- Skog J, Würdinger T, Van Rijn S, Meijer DH, Gainche L, Curry WT, Carter BS, Krichevsky AM, Breakefield XO. 2008. Glioblastoma microvesicles transport RNA and proteins that promote tumour growth and provide diagnostic biomarkers. Nat Cell Biol. 10(12):1470–1476. doi:10.1038/ncb1800.
- Sullivan R. 2015. Epididymosomes: a heterogeneous population of microvesicles with multiple functions in sperm maturation and storage. Asian J Androl. 17(5):726–729.
- Sullivan R, Saez F. 2013. Epididymosomes, prostasomes, and liposomes: their roles in mammalian male reproductive physiology. Reproduction. 146(1):R21–35. doi:10.1530/REP-13-0058.
- Thery C, Ostrowski M, Segura E. 2009. Membrane vesicles as conveyors of immune responses. Nat Rev Immunol. 9(8):581–593. doi:10.1038/nri2567.
- Trams EG, Lauter CJ, Salem N Jr., Heine U. 1981. Exfoliation of membrane ecto-enzymes in the form of micro-vesicles. Biochim Biophys Acta. 645(1):63–70. doi:10.1016/0005-2736(81)90512-5.
- Tremellen KP, Valbuena D, Landeras J, Ballesteros A, Martinez J, Mendoza S, Norman RJ, Robertson SA, Simon C. 2000. The effect of intercourse on pregnancy rates during assisted human reproduction. Hum Reprod. 15(12):2653–2658. doi:10.1093/humrep/15.12.2653.
- Valadi H, Ekstrom K, Bossios A, Sjostrand M, Lee JJ, Lotvall JO. 2007. Exosome-mediated transfer of mRNAs and microRNAs is a novel mechanism of genetic exchange between cells. Nat Cell Biol. 9(6):654–659. doi:10.1038/ncb1596.
- Vojtech L, Woo S, Hughes S, Levy C, Ballweber L, Sauteraud RP, Strobl J, Westerberg K, Gottardo R, Tewari M, et al. 2014. Exosomes in human semen carry a distinctive repertoire of small non-coding RNAs with potential regulatory functions. Nucleic Acids Res. 42(11):7290–7304. doi:10.1093/nar/gku347.
- Wu H, Hauser R, Krawetz SA, Pilsner JR. 2015. Environmental susceptibility of the sperm epigenome during windows of male germ cell development. Curr Environ Health Rep. doi:10.1007/s40572-015-0067-7
- Yanagimachi R, Kamiguchi Y, Mikamo K, Suzuki F, Yanagimachi H. 1985. Maturation of spermatozoa in the epididymis of the Chinese hamster. Am J Anat. 172(4):317–330. doi:10.1002/aja.1001720406.
- Yanez-Mo M, Siljander PR, Andreu Z, Zavec AB, Borras FE, Buzas EI, Buzas K, Casal E, Cappello F, Carvalho J, et al. 2015. Biological properties of extracellular vesicles and their physiological functions. J Extracell Vesicles. 4:27066. doi:10.3402/jev.v4.27066.
- Yang C, Guo WB, Zhang WS, Bian J, Yang JK, Zhou QZ, Chen MK, Peng W, Qi T, Wang CY, et al. 2017. Comprehensive proteomics analysis of exosomes derived from human seminal plasma. Andrology. 5(5):1007–1015. doi:10.1111/andr.12412.
- Yuan A, Farber E, Rapoport A, Tejada D, Deniskin R, Akhmedov N, Farber D. 2009. Transfer of MicroRNAs by embryonic stem cell microvesicles. PloS One. 4:e4722. doi:10.1371/journal.pone.0004722.