ABSTRACT
In the mammalian ovary, <1% of the follicles ovulate, with most undergoing degenerative atresia during ovarian follicular development. Follicular atresia is caused by the apoptosis of granulosa cells (GCs), although the precise underpinning mechanism remains unidentified. MiR-26a regulates various cellular events, including cell division, apoptotic signaling, and cell differentiation, migration, and autophagy. Here, we demonstrated that miR-26a regulated apoptosis in GCs in the mouse ovary through Ezh2, a key regulator of GC viability. We also found that transcription of miR-26a changed in response to an LH antagonist and a GnRH agonist. In addition, miR-26a transcription was downregulated following LH-induced transition of GCs to granulosa-lutein cells (GLCs). Dual-luciferase reporter assays confirmed Ezh2 as a miR-26a target. Exogenous expression in GCs of miR-26a mimics resulted in decreased Ezh2 expression, while miR-26a inhibition in GCs induced the opposite phenotype. Ezh2 silencing additionally reduced the anti-apoptotic effect of miR-26a inhibition in GCs. These data highlight the critical role of miR-26a in targeting Ezh2 and regulating apoptosis in mouse ovarian GCs.
Abbreviations: GC: Granulosa cell; GLCs: Granulosa-lutein cells; LH: Luteinizing hormone; miRNA: MicroRNA; NC: Negative control; Cyt-c: Cytochrome c; GnRH: Gonadotropin releasing hormone; i.p.: intraperitoneal injection; cKO: conditional knock-out; WB: Western blotting; hCG: Human chorionic gonadotropin; NPC: nasopharyngeal carcinoma.
Introduction
Granulosa cells (GCs) originate from the ovarian surface epithelium. The GCs that surround each oocyte have an oligo-clonal origin, and encompass cumulus and mural granulosa cells. The healthy development and function of these cells is key to follicular development and the formation of functional oocytes. Under the action of luteinizing hormone (LH), GCs are transformed into terminally differentiated granular luteal cells (GLCs), with significantly changed cell morphology and function (Carletti et al. Citation2010). In this process, >99% of ovarian follicles degenerate, undergoing atresia. It has been reported that atresia results from GC apoptosis (Manabe et al. Citation2008), although the precise underpinning mechanism remains undefined.
MicroRNAs (miRNAs) are gradually considered essential mediators of cell differentiation, proliferation, and apoptosis (Ademokun and Turner Citation2008; Gangaraju and Lin Citation2009). Bioinformatic analyses emphasizes that a large number of miRNA targets are closely related to ovarian function (Bartel, Citation2004; Ambros Citation2004; Chivukula and Mendell Citation2008; Hashimi et al. Citation2009; Yang et al. Citation2009). For example, Dicer, which controls small interfering RNA and microRNA production, is a critical part of RNA interference. Conditional knockout of Dicer1 in GCs using an anti-Mullein hormone receptor-2 promoter-Cre, alters ovarian function, highlighting the key role of miRNAs in ovary function. A conditional Dicer1 knockout (cKO) in female mice decreases ovulation and enhances GC apoptosis, promoting infertility (Hong et al. Citation2008; Nagaraja et al. Citation2008). The miR-26 family is an essential class of miRNAs discovered in 2001 by Lagos-Quintana et al. (Citation2001) in HeLa cells. It generally comprises miR-26a-1, miR-26a-2 and miR-26b members, which are evolutionarily conserved miRNAs. High miR-26a expression in human ovaries, fallopian tubes, uterus, and cervix has been observed (Nothnick Citation2012). In addition, Carletti and Christenson (Citation2009) observed elevated levels of miR-26a amounts in the ovaries of newborn mice. Studies have confirmed that abnormal expression of miR-26a affects cell proliferation and apoptosis (Lu et al. Citation2011; Lv et al. Citation2013; Chunbo et al. Citation2016). In addition, EZH2, a miR-26a target, represents a protein that affects apoptosis and senescence (Caretti et al. Citation2004; Wong and Tellam Citation2008; Ji et al. Citation2009; Kim et al. Citation2010). It was reported that Ezh2 mRNA levels are decreased in GCs undergoing luteinization after ovulation (Maekawa et al. Citation2016), suggesting EZH2’s contribution to mediating apoptosis in GCs (Carletti et al. Citation2010). However, the role of EZH2 and its regulation by miRNAs have not been fully defined in the ovaries.
Therefore, we hypothesized that miR-26a participates in GC apoptosis. To test the above hypothesis, we investigated whether miR-26a regulates Ezh2 and GC apoptosis in mouse ovaries.
Results and discussion
Effects of LH and miR-26a on apoptosis in GCs
LH induces ovarian GCs to promote ovulation and luteinization, leading to oocyte meiosis and subsequent cumulus expansion. Granulosa cell phenotypes change as early as five to seven hours (h) after the chorionic gonadotropin (hCG) surge begins (Oonk et al. Citation1989; Chaffin and Stouffer Citation2002). Besides affecting differentiation, LH can inhibit GC apoptosis (Robker and Richards Citation1998). Through the assessment of miR-26a expression in GCs following treatment with LH antagonists and GnRH agonists, the transcription of miR-26a was variably regulated by the endocrine hormone LH. In concert, LH antagonists caused follicular atresia and reduced the number of ovulations. GC apoptosis has a known correlation with follicular atresia (Reza et al. Citation2019), which suggests that miR-26a controls apoptosis in LH-related differentiation of GCs. These data and conclusions highlighted a crucial role for miRNA-26a in follicular development and fertility.
In the process of transformation from GCs to GLCs, it is necessary to rely on LH to inhibit apoptosis and form functional luteal cells. Otherwise, follicular atresia occurs. A total of 8 h upon injection, we first assessed the levels of LH in mice assigned to groups 1 (administered ECG; EFs), 2 (administered LH antagonist; LaFs), and 3 (administered gonadotropin releasing hormone agonist; GaFs). LH levels were significantly higher in the GaFs and EFs groups compared with the LaFs group (). At 12h following injection, the level of the cumulus-oocyte complex (COC) in the oviduct of the LaFs group were significantly lower than those of the GaFs and EFs groups (). These results showed that LH antagonists or GnRH agonists alter LH levels in mice, with LH antagonists further causing follicular atresia and reducing the number of ovulations.
Figure 1. Effects of LH antagonist and GnRH agonist. (A) LH levels in the LaFs, EFs, and GaFs groups assessed by chemiluminescence. (B) Amounts of cumulus-oocyte complexes (COCs) within the ovaries in the three groups. Data are mean ± standard deviation (SD) (n = 3). *P < 0.05, ** P < 0.01 (Student’s t-test)
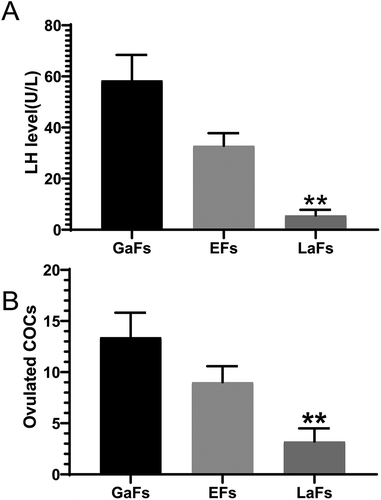
Furthermore, we assessed cellular miRNA expression in these cells through microarray analysis in the follicles of mouse ovaries. The μParaflo TM miRNA microarray assay was used to evaluate the expression profiles of 747 mature miRNAs during mouse follicular atresia. They were composed of two parts: 662 unique mature miRNAs from mouse, human, and pig, (based on Sanger miRBase release 13.0, marked by species) and 85 mouse miRNA candidates predicted in our laboratory (Supplemental File 1). In total, 321, 329, and 415 miRNAs were detected respectively in EFs, LaFs, and GaFs follicles. There are about 200 differentially expressed miRNAs (P < 0.01) identified by Analysis of Variance. 23 miRNAs (signal value >1000 with an EFs/GaFS >2.0 or an EFs/GaFS <0.8) were screened, including miR-26a (). As shown in , there were significant differences among the three groups after injection. Compared with GaFs and EFs groups, the level of miR-26a in LaFs group was significantly higher, which had the opposite result to the change of LH level, indicating that the change of miR-26a had a negative correlation with the level of LH. The levels of miR-26a in GaFs, EFs, and LaFs groups were further confirmed by qRT-PCR. All data were shown in . These results indicated that LH deficiency may lead to increased expression of miR-26a and led to follicular atresia. After the GO and Pathway analysis, 324 significant target genes (Supplemental File 2) were selected with widespread roles in cell processes such as cell proliferation, differentiation, adhesion, apoptosis, and DNA replication (Supplemental File 3). miR-26a was one of the most prevalent miRNAs and involved in apoptosis.
Table 1. Differentially expressed miRNA in three group
Figure 2. miR-26a expression associated with LH levels induces GCS apoptosis. (A) miR-26a expression in the LaFs, EFs, above data suggested that Bax exprand GaFs groups, assessed by microarrays. (B) Relative miR-26a amounts assessed by qRT-PCR. Data are mean ± standard deviation (SD). (n = 3). *P < 0.05, **P < 0.01 (Student’s t-test). (C) GC apoptosis upon exogenous overexpression of miR-26a or Negative control (NC) mimics. qRT-PCR assessment of Bcl-2 (D) and Bax (E) mRNA levels after treatment with miR-26a or NC mimics. (F) GC apoptosis following miR-26a inhibitor administration, as assessed by FACS analysis. (G) Rates of apoptosis in the indicated cells. Data are mean ± standard deviation (SD). *P < 0.05, **P < 0.01 (Student’s t-test)
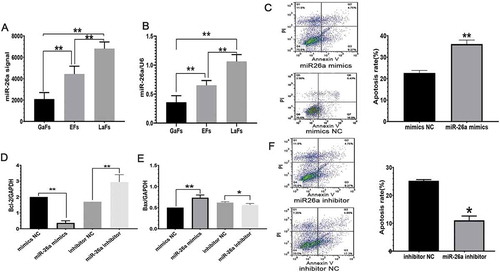
Effects of miR-26a on GC apoptosis
A previous study confirmed that GC apoptosis regulates follicular atresia to examine whether mir26a contributes to apoptosis, exogenous miR-26a and Negative control (NC) mimics were assessed for their effects on apoptotic rates in GCs by PI and annexin V staining. GCs expressing miR-26a mimics had enhanced apoptosis (), confirming its effects on GC viability.
Bcl-2 proteins encompass anti- and pro-apoptotic members. Bcl-2 is anti-apoptotic as it regulates the mitochondrial membrane potential and prevents cytochrome c (Cyt-c) release into the cytoplasm for cell survival maintenance. Bax is a pro-apoptotic molecule that inhibits Bcl-2 to induce Cyt-c release into the cytoplasm and subsequent apoptosis. To further confirm the above data, qRT-PCR was performed for Bcl-2 and Bax mRNA level assessment. The expression of Bcl-2 was remarkably reduced, while Bax was substantially upregulated in cells treated with miR-26a mimics compared with cells administered mimics NC ( and E). Altered Bcl-2 and Bax levels were also found in the miR-26a inhibitor group. Indeed, miR-26a inhibition downregulated Bax and upregulated Bcl-2 compared with the NC group ( and E). In addition, miR-26a’s effects on GC apoptosis were further confirmed as the apoptotic rate was markedly lower after treatment with a miR-26a inhibitor versus the NC group (). The above data suggested that Bax expression is enhanced, while Bcl-2 is suppressed following exogenous treatment of GCs with miR-26a mimics, indicating a role for miR-26a overexpression in GC mediated cell death.
Ezh2 is a miR-26a target
Mutual recognition of miRNAs and target genes is one of the current research hotspots (Marrero Citation2009). EZH2 belongs to the polycomb protein family, and has a major regulatory function in multiple critical cell processes (Sparmann and Van Lohuizen Citation2006).
Cellular gene targets of miR-26a were assessed via TargetScan, miRBase, and miRanda algorithms. Ezh2 was detected as a miR-26a target in all three databases, highlighting it as a potential target. In nasopharyngeal carcinoma (NPC), Ezh2 has been identified as a miR-26a target repressing E-cadherin expression to promote cell metastasis (Alajez et al. Citation2010; Lu et al. Citation2011; Tong et al. Citation2012). We constructed pmirGLO expressing the 3ʹ-UTR of mouse Ezh2 and expressed the construct in HeLa 229 cells with miR-26a and NC mimics, respectively (). Exogenous expression of miR-26a mimics resulted in reduced luciferase activity (). These data confirmed that mouse Ezh2 is a miR-26a target.
miR-26a induces apoptosis through Ezh2 targeting in GCs
To further investigate miR-26a’s effects on Ezh2 expression, in vitro mouse GCs underwent transfection with miR-26a mimics and inhibitors, respectively. Ezh2 expression at the mRNA level was decreased upon transfection with miR-26a mimics, but increased following the expression of miR-26a inhibitors ( A). These findings were confirmed at the protein level by WB analysis ( B). These data suggested that Ezh2 is targeted by miR-26a in mouse ovary GCs.
Ezh2 suppression causes apoptotic in GCs
miRNAs regulate gene expression through translational repression or by promoting mRNA degradation (Edlund et al. Citation2005). miRNAs are ‘multivalent,’ with single miRNAs targeting many cellular genes, and subsequently regulating the expression of diverse proteins. To investigate whether the effects of miR-26a are mediated through Ezh2, gain and loss-of-function studies were performed. Ezh2 was silenced through RNAi ( and ), which further enhanced GC apoptosis () and Bax expression (), while simultaneously suppressing Bcl-2 expression (). Furthermore, Ezh2 silencing suppressed the anti-apoptotic effect of the miR-26a inhibitor (). Mouse GCs expressing Ezh2-specific siRNAs had inhibited Ezh2 expression at both the gene and protein levels. Ezh2 is upregulated and inversely correlates with miR-26a (Lu et al. Citation2011). It forms the catalytic core of PcG complexes, with H3K27me3 as a characteristic feature of facultative heterochromatin. Ezh2 silencing induces senescence in response to DNA damage, causing DNA damage regulated via the ATM-p53-p21 axis (Ito et al. Citation2018). These data reveal miR-26a as a factor that induces GC apoptosis in the ovaries of mice.
Figure 5. EZH2 prevents GC apoptosis and alleviates miR-26a’s effects in GCs. (A) Ezh2-siRNA administration reduces Ezh2 expression at the gene level. (B) Ezh2 silencing at the protein level. (C) Ezh2 silencing reduces Bcl-2 expression. (D) Ezh2 silencing increases Bax expression. (E) Ezh2 silencing enhances apoptosis in GCs. (F) Ezh2 siRNA reduces the impact of miR-26a inhibitor in GCs. Data are mean ± standard deviation (SD) (n = 3). * P < 0.05, **P < 0.01 (Student’s t-test)
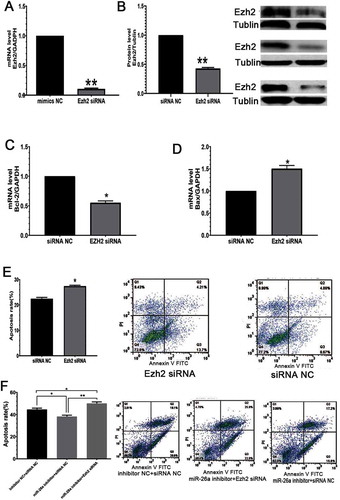
In summary, miR-26a is highly expressed in GCs, inducing apoptosis and Ezh2 was confirmed as a miR-26a target. These data provide new insights into the mechanisms of ovarian follicular atresia. Further studies are required to confirm the functional mechanisms of miR-26a in GCs and the related signaling pathways.
Materials and methods
Animal protocol
Our local ethics committee approved all animal experiments. Female CF1 mice were obtained from the Shanghai SLAC Laboratory for in vivo assessments, the animals underwent intraperitoneal injection (i.p.) at 5 IU of equine chorionic gonadotropin (ECG, FSH analog; ProSpec, Rehovot, Israel, catalog number: HOR-272) to enhance follicular development. A total of 48 h later, the mice were divided into three groups. Mice in Group 1 were administered 0.05 ml of normal saline. Group 2 animals received 0.01 mg of Cetrorelix Acetate (LH antagonist; ProSpec, Rehovot, Israel, catalog number: HOR-277) to decrease LH amounts. Mice in Group 3 were treated with 0.05 mg of Triptorelin (Gonadotropin releasing hormone (GnRH) agonist; ProSpec, Rehovot, Israel, catalog number: HOR-238) to raise the levels of LH. A total of 8 h after injection, LH amounts were assessed. The mice were euthanized, and tissue samples from one ovary were collected after 12 h. Ovaries were transferred into Petri dishes containing PBS, and punctured under a surgical dissecting microscope to obtain mural granulosa cells. In the other ovary, cumulus-oocyte complexes in the oviduct were enumerated to derive the ovulation rate. GCs were divided into ECG follicles (EFs), LH antagonist follicles (LaFs), and GnRH agonist follicles (GaFs), which respectively corresponded to groups 1, 2 and 3. RNA was extracted using miRNeasy Mini Kit (Qiagen, Germany, Catalog number: 217,004), and miRNAs were grouped according to μParaflo miRNA microarrays. In addition, miR-26a was assessed with PrimeScript™ miRNA qPCR Starter Kit (TakaRa, Japan, Catalog number: CY505S) and normalized to U6 expression. Primer sequences are shown in . All protocols were performed according to the manufacturer’s recommendations when commercial kits were employed.
Table 2. Small fragments of RNA synthesized in the present study
Cell culture
To obtain high-quality pre-ovulation granulosa cells, ovarian tissues from 5-week-old mice were cultured in M-199 medium with 0.5 M sucrose for 15 minutes, and washed with the medium. Granulosa cells were obtained by puncturing follicles and rinsing with M-199 medium (Campbell Citation1979). Isolated GCs were seeded into six-well culture plates. In the logarithmic growth phase, ovarian GCs were incubated in DMEM/Nutrient Mixture F-12 (Gibco, USA, Catalog number: 12,400,024) supplemented with 15% FBS (Gibco, USA, Catalog number: 10,010,023) and pen/strep for 3 d at 37°C under normal cell culture conditions. HeLa 229 cells were treated by identical protocols. Upon 24 h of culture, GCs were transfected with the transfection complex for 6 h utilizing Lipofectamine 2000 (Invitrogen, USA, Catalog number: 11,668,027) and further cultured for 48 h. The miR-26a and negative control (NC) mimics, miR-26a and NC inhibitors, and Ezh2-siRNA and scrambled controls were manufactured by GenePharma (Shanghai, China). Oligonucleotides were transfected with Lipofectamine 2000 as per the instructions of the manufacturer.
LH detection
Blood samples were collected from the posterior orbital venous plexus of female CF1 mice, and plasma was separated at 3000 r/min×10 min and stored at −80°C. Plasma LH levels were measured with a mouse LH detection kit (Endocrine Technologies, USA, Catalog number: BR B2003b).
Apoptosis assays
Apoptosis assessment was performed with a commercial Annexin V FITC/PI kit (KeyGEN, Nanjing, China, Catalog number: KGA107) as instructed by the manufacturer. Cells were resuspended in PI/FITC-conjugated annexin V binding buffer. Apoptotic cells were identified by FACS analysis on a Beckman Coulter (Indianapolis, IN).
MicroRNA microarrays
The microRNA microarray Assay was conducted using a service provider (LC, TX, USA). The assay needed 3 to 6 μg RNA. Hybridization was performed overnight on a µParaflo™. Data was analyzed by first subtracting the background and then normalizing the signals by using a LOWESS filter. The differentially expressed miRNAs were identified by Analysis of Variance. The EFs/GaFS value (signal value >1000 with an EFs/GaFS >2.0 or an EFs/GaFS <0.8) was used for screening. After the Gene Ontology (GO) and Pathway analysis, 324 significant target genes (Supplemental File 2) were selected with widespread roles in cell processes such as cell proliferation, differentiation, adhesion, apoptosis, and DNA replication (Supplemental File 3). miR-26a was one of the most prevalent miRNAs and involved in apoptosis.
qRT-PCR analysis
GCs were lysed in TRIzol (Invitrogen, USA, Catalog number: 15,596,026). DNase treatment of the obtained RNA samples was carried out to ensure the absence of DNA traces. Reverse transcription utilized oligo (DT) 18 primers and M-MLV reverse transcriptase. Then, qRT-PCR was performed using SYBR Premix Ex Taq. U6 was assessed as a standard for normalizing miR-26a expression. The 2–ΔΔct method was used to assess gene expression, which was normalized to GAPDH or U6 (n = 3, data: mean ± SE). All assays were performed three or more times in an independent manner.
Bioinformatics
GO analysis was carried out with MAS 3.0 (http://bioinfo.capitalbio.com/mas3/). Predicted miR-26a targets were investigated with TargetScanv5.1 (http://www.targetscan.org/vert_72/) and the Miranda software (http://www.microrna.org/microrna/home.do), as previously described.
Plasmids and reporter assays
The predicted miR-26a-targeted 3ʹUTR binding sites in Ezh2 were subcloned into pmirGLO with SacI and XbaI restriction sites (). Point mutations were introduced into the Ezh2 3´-UTR as controls (TACTTGAA’ to CGAGGACC’; Generay, Shanghai, China). GCs were transfected in 6-well plates, with Firefly and Renilla luciferase activities assessed the following day (Promega, WI, USA). Values are shown as Renilla/Firefly signals.
Table 3. The primers used in this study
WB analysis of EZH2 and α-tubulin
GCs underwent lysis in the RIPA buffer plus protease inhibitors, and protein content was assessed by the Bradford assay. Equal protein concentrations were resolved by SDS–PAGE, and electro-transferred onto nitrocellulose membranes. After blocking, the membranes were incubated with anti-EZH2 antibody (1:1000; Santa Cruz, TX, USA, Catalog number: sc-156,000) or anti-α-tubulin antibody (Santa Cruz, Catalog number: sc-3), and labeled with goat anti-mouse IgG-HRP (1:2000; CST, MA, USA, Catalog number: sc00082). Protein visualization used the Super Signal West Pico system. All assays were repeated three times and quantified with Image J.
Analysis of EZH2’s effects on miR-26a inhibitor-associated alterations of GC apoptosis
GCs underwent transfection with siRNAs targeting Ezh2 and NC siRNAs for 24 h, and silencing was confirmed by qRT-PCR and Western blotting (WB). Apoptotic rates were assessed 48 h post-transfection. The effects of EZH2 on miR-26a inhibitor-associated alterations of GC apoptosis were assessed through co-transfection of GCs with miR-26a inhibitors, siRNA-Ezh2, or NC. Cells were analyzed 48 h post-transfection.
Statistical analysis
Data were expressed as mean ± SD. The Student’s t-test was performed for comparisons, with P < 0.05 indicating statistical significance. Excel and GraphPad Prism 7.0 (GraphPad, San Diego, CA) were utilized for data analysis. Image J was employed for densitometric assessment of immunoblot data. No data, samples or animals were excluded or omitted from reporting.
Ethics approval
The mouse study had approval from the Institutional Animal Care and Use Committee (IACUC) of Taian University (0538 IACUC2018-135), and followed all Regulations for the Administration of Affairs Concerning Experimental Animals approved by the State Council of the People’s Republic of China.
Supplemental Material
Download Zip (3.1 MB)Acknowledgments
We thank Shujing Wu for providing the CF1 lines used in this project. We would like to acknowledge all our colleagues, who generously provided good advices and interesting discussions about our findings.
Disclosure statement
The authors declared no conflicts of interest in this work.
Authors’ contributions: Study conception and design: SWH; performed the experiments: HQ, CZL; data analysis: YXS, WYD; manuscript writing: SWH.
Supplemental Material
Supplemental data for this article can be accessed here.
References
- Ademokun A, Turner M. 2008. Regulation of B-cell differentiation by microRNAs and RNA-binding proteins. Biochem Soc Trans. 36(Pt 6):1191. doi:10.1042/BST0361191.
- Alajez N, Shi W, Hui A, Bruce J, Lenarduzzi M, Ito E, Yue S, O’sullivan B, Liu F. 2010. Enhancer of Zeste homolog 2 (EZH2) is overexpressed in recurrent nasopharyngeal carcinoma and is regulated by miR-26a, miR-101, and miR-98. Cell Death Dis. 1(10):e85. doi:10.1038/cddis.2010.64.
- Ambros V. 2004. The functions of animal microRNAs. Nature. 431(7006):350. doi:10.1038/nature02871.
- Bartel DP. 2004. MicroRNAs: genomics, biogenesis, mechanism, and function. cell. 116(2):281–297. doi:10.1016/S0092-8674(04)00045-5.
- Campbell KL. 1979. Ovarian granulosa cells isolated with EGTA and hypertonic sucrose: cellular integrity and function. Biol Reprod. 4:773–786. doi:10.1095/biolreprod21.4.773.
- Caretti G, Di Padova M, Micales B, Lyons GE, Sartorelli V. 2004. The Polycomb Ezh2 methyltransferase regulates muscle gene expression and skeletal muscle differentiation. Genes Dev. 18(21):2627–2638. doi:10.1101/gad.1241904.
- Carletti M, Christenson L. 2009. MicroRNA in the ovary and female reproductive tract. J Anim Sci. 87(suppl_14):E29–E38. doi:10.2527/jas.2008-1331.
- Carletti MZ, Fiedler SD, Christenson LK. 2010. MicroRNA 21 blocks apoptosis in mouse periovulatory granulosa cells. Biol Reprod. 83(2):286–295. doi:10.1095/biolreprod.109.081448.
- Chaffin CL, Stouffer RL. 2002. Local role of progesterone in the ovary during the periovulatory interval. Rev Endocr Metab Disord. 3(1):65–72. doi:10.1023/A:1012704903128.
- Chivukula RR, Mendell JT. 2008. Circular reasoning: microRNAs and cell-cycle control. Trends Biochem Sci. 33(10):474–481. doi:10.1016/j.tibs.2008.06.008.
- Chunbo ZHUANG,PEI, H Da, L Xu, L Hu. 2016. A double-negative feedback loop between EZH2 and miR-26a regulates tumor cell growth in hepatocellular carcinoma. Int J Oncol. 48(3):1195.
- Edlund S, Lee SY, Grimsby S, Zhang S, Aspenström P, Heldin C-H LM. 2005. Interaction between Smad7 and β-catenin: importance for transforming growth factor β-induced apoptosis. Mol Cell Biol. 25(4):1475–1488. doi:10.1128/MCB.25.4.1475-1488.2005.
- Gangaraju VK, Lin H. 2009. MicroRNAs: key regulators of stem cells. Nat Rev Mol Cell Biol. 10(2):116. doi:10.1038/nrm2621.
- Hashimi ST, Fulcher JA, Chang MH, Gov L, Wang S, Lee B. 2009. MicroRNA profiling identifies miR-34a and miR-21 and their target genes JAG1 and WNT1 in the coordinate regulation of dendritic cell differentiation. Blood. 114(2):404–414. doi:10.1182/blood-2008-09-179150.
- Hong X, Luense LJ, McGinnis LK, Nothnick WB, Christenson LK. 2008. Dicer1 is essential for female fertility and normal development of the female reproductive system. Endocrinology. 149(12):6207–6212. doi:10.1210/en.2008-0294.
- Ito T, Teo YV, Evans SA, Neretti N, Sedivy JM. 2018. Regulation of cellular senescence by polycomb chromatin modifiers through distinct DNA Damage- and histone methylation-dependent pathways. Cell Rep. 22(13):3480–3492. doi:10.1016/j.celrep.2018.03.002.
- Ji J, Shi J, Budhu A, Yu Z, Forgues M, Roessler S, Ambs S, Chen Y, Meltzer PS, Croce CM. 2009. MicroRNA expression, survival, and response to interferon in liver cancer. New England J Med. 361(15):1437–1447. doi:10.1056/NEJMoa0901282.
- Kim H, Huang W, Jiang X, Pennicooke B, Park PJ, Johnson MD. 2010. Integrative genome analysis reveals an oncomir/oncogene cluster regulating glioblastoma survivorship. Pro Nat Acad Sci. 107(5):2183–2188.
- Lagos-Quintana M, Rauhut R, Lendeckel W, Tuschl T. 2001. Identification of novel genes coding for small expressed RNAs. Science. 294(5543):853–858.
- Lu J, He M-L, Wang L, Chen Y, Liu X, Dong Q, Chen Y-C, Peng Y, Yao K-T, Kung H-F. 2011. MiR-26a inhibits cell growth and tumorigenesis of nasopharyngeal carcinoma through repression of EZH2. Cancer Res. 71(1):225–233.
- Lv M, Zhang X, Li M, CHen Q, Ye M, Liang WQ, Ding LB, Cai HD, Da F, Lv Zw. 2013. miR-26a and its target CKS2 modulate cell growth and tumorigenesis of papillary thyroid carcinoma. PLOS ONE. p. 8.
- Maekawa R, Lee L, Okada M, Asada H, Shinagawa M, Tamura I, Sato S, Tamura H, Sugino N. 2016. Changes in gene expression of histone modification enzymes in rat granulosa cells undergoing luteinization during ovulation. J Ovarian Res. 9(1):15.
- Manabe N, Matsuda‐Minehata F, Goto Y, Maeda A, Cheng Y, Nakagawa S, Inoue N, Wongpanit K, Jin H, Gonda H. 2008. Role of cell death ligand and receptor system on regulation of follicular atresia in pig ovaries. Reprod Domestic Ani. 43:268–272.
- Marrero JA. 2009. Modern diagnosis of hepatocellular carcinoma: utilization of liver biopsy and genomic markers. J Hepatol. 50(4):659–661.
- Nagaraja AK, Andreu-Vieyra C, Franco HL, Ma L, Chen R, Han DY, Zhu H, Agno JE, Gunaratne PH, DeMayo FJ. 2008. Deletion of Dicer in somatic cells of the female reproductive tract causes sterility. Mol Endocrinol. 22(10):2336–2352.
- Nothnick WB. 2012. The role of micro-RNAs in the female reproductive tract. Reproduction. 143(5):559.
- Oonk RB, Krasnow J, Beattie W, Richards J. 1989. Cyclic AMP-dependent and-independent regulation of cholesterol side chain cleavage cytochrome P-450 (P-450scc) in rat ovarian granulosa cells and corpora lutea. cDNA and deduced amino acid sequence of rat P-450scc. J Biol Chem. 264(36):21934–21942.
- Reza AMMT, Choi YJ, Han SG, Song H, Park C, Hong K, Kim JH. 2019. Roles of microRNAs in mammalian reproduction: from the commitment of germ cells to peri‐implantation embryos. Biol Rev. 94(2):415–438.
- Robker RL, Richards JS. 1998. Hormone-induced proliferation and differentiation of granulosa cells: a coordinated balance of the cell cycle regulators cyclin D2 and p27Kip1. Mol Endocrinol. 12(7):924–940.
- Sparmann A, Van Lohuizen M. 2006. Polycomb silencers control cell fate, development and cancer. Nat Rev Cancer. 6(11):846.
- Tong Z, Cai M, Wang X, Kong L, Mai S-J, Liu Y, Zhang H, Liao Y, Zheng F, Zhu W. 2012. EZH2 supports nasopharyngeal carcinoma cell aggressiveness by forming a co-repressor complex with HDAC1/HDAC2 and Snail to inhibit E-cadherin. Oncogene. 31(5):583–594.
- Wong CF, Tellam RL. 2008. MicroRNA-26a targets the histone methyltransferase Enhancer of Zeste homolog 2 during myogenesis. J Biol Chem. 283(15):9836–9843.
- Yang GH, Wang F, Yu J, Wang XS, Yuan JY, Zhang JW. 2009. MicroRNAs are involved in erythroid differentiation control. J Cell Biochem. 107(3):548–556.