ABSTRACT
Tissue engineering is gaining use to investigate the application of its techniques for infertility treatment. The use of pluripotent embryonic cells for in vitro production of viable spermatozoa in testicular scaffolds is a promising strategy that could solve male infertility. Due to cell–extracellular matrix (ECM) interactions, here we aim to investigate the differentiation of embryoid bodies (EBs) in cultured into decellularized rat testis scaffolds. Decellularized testis (P = 0.019) with a low concentration of gDNA (30.58 mg/ng tissue) was obtained by sodium dodecyl sulfate perfusion. The structural proteins (collagens type I and III) and the adhesive glycoproteins of ECM (laminin and fibronectin) were preserved according to histological and scanning electron microscopy (SEM) analyses. Then, decellularized rat testis were cultured for 7 days with EB, and EB mixed with retinoic acid (RA) in non-adherent plates. By SEM, we observe that embryonic stem cells adhered in the decellularized testis ECM. By immunofluorescence, we verified the positive expression of HSD17B3, GDNF, ACRV-1, and TRIM-36, indicating their differentiation using RA in vitro, reinforcing the possibility of EB in male germ cell differentiation. Finally, recellularized testis ECM may be a promising tool for future new approaches for testicular cell differentiation applied to assisted reproduction techniques and infertility treatment.
Abbreviations: ACRV-1: Acrosomal vesicle protein 1; ATB: Penicillin-streptomycin; DAPI: 4,6-Diamidino-2-phenylindole; EB: Embryoid bodies; ECM: Extracellular matrix; ESCs: Pluripotent embryonic stem cells; GAGs: Glycosaminoglycans; gDNA: Genomic DNA; GDNF: Glial cell line-derived neurotrophic factor; H&E: Hematoxylin and eosin; HSD17B3: 17-beta-Hydroxysteroid dehydrogenase type 3; PBS: Phosphate-buffered saline; PGCLCs: Primordial germ-cell-like cells; RA: Retinoic acid; SDS: Sodium dodecyl sulfate; SEM: Scanning electron microscopy; SSCs: Spermatogonial stem cells; TRIM-36: Tripartite Motif Containing 36;
Introduction
Nearly 15% of couples suffer from infertility problems and have difficulties to conceive. Reports show a variance of 8–50% of the cause of infertility being from the male partner (Forbes et al. Citation2018; Dong et al. Citation2019; Kumar and Singh Citation2020). Infertility is a key concern related to animals and human health. In order to solve this problem, new techniques are being developed to increase the infertility treatment by tissue engineering (Ogawa et al. Citation1997; Nagano et al. Citation1998; Bhongade et al. Citation2015; Greil et al. Citation2010; Mahabadi et al. Citation2020; Amini Mahabadi et al. Citation2020; J. J. Lim et al. Citation2014).
Tissue bioengineering based on the combination of scaffolds and cells has offered a huge perspective for gonadal treatment, previously considered incurable (Costa et al. Citation2017). Fertility preservation and assisted reproduction requires effective culture systems for the successful proliferation and differentiation. An alternative applied to infertility treatment is the coculture of decellularized extracellular matrix (ECM) with induced pluripotent stem cells (iPSCs), aiming to produce viable male germ cells in vitro (Alves-Lopes and Stukenborg Citation2018). However, due to iPSCs’ specific characteristics, such as differentiation, pluripotency, and expression, they are eligible for spermatozoa generation (Mahabadi et al. Citation2020a). Additionally, the interaction between the pluripotent embryonic stem cells (ESCs) and ECM supports ESC differentiation into all of the three embryonic layers. Based on this, primordial germ-cell like cells (PGCLCs) can differentiate in spermatogonial stem cells (SSCs) (Nayernia et al. Citation2006; Zogbi et al. Citation2012; Pieri et al. Citation2019).
Moreover, with the ECM embryoid bodies (EBs) enhance the induction of ESCs to a blastocyst-like state with potential for random differentiation (de Araújo Ramos et al., 2017). Some molecules can be used to stimulate this process, such as the retinoic acid (RA) that balances differentiation of the SSCs, and their self-renewal regulating the expression of the KIT protein, found in differentiated spermatogonia (Busada et al. Citation2015; Helsel and Griswold Citation2019; X. Li et al. 2019). RA signaling is also protects various cells from mutation, spermatozoa from apoptosis, and following meiotic division the release of the spermatocyte and gametes from the seminiferous tubules. To date, the shortage of RA has been linked to the greatest number of pathways disrupting fertility in males (X. Li et al. 2019; Teletin et al. Citation2017; Helsel and Griswold Citation2019).
Thus, this study aimed to develop a viable three-dimensional ECM for coculture with EB, and EB + RA from decellularized rat testis. This would provide a new technique for animal reproduction-assisted technologies and male infertility treatment. In this report, the cell adhesion and differentiation potential on the ECM were verified,
Results and discussion
At the end of the decellularization protocol, it was possible to observe a change on the surface color of the biomaterials, which became whitish with a translucent aspect ( ), as also noted by Akbarzadeh et al. Citation2019. These changes in aspect and color occur due to the ECM cell removal, which was also confirmed by genomic DNA quantification associated with 4,6-diamidino-2-phenylindole (DAPI) staining () and histological (H&E) evaluation. The scaffolds showed an amount of genetic material below of 50 ng per mg of tissue according to genomic DNA quantification test (). This is considered ideal for a decellularization protocol (Crapo et al. Citation2011). The histological evaluation with H&E staining shows an ECM in pink (hematoxylin) and cell nuclei in purple (eosin) (Gupta and Mishra Citation2018), revealing the almost complete removal of cells in decellularized testis compared to control samples ( ). The presence of nuclei in control tissue is evident (), whereas none was observed in decellularized ECMs (). These results testify to the efficacy of the 1% sodium dodecyl sulfate (SDS) protocol for mouse testis decellularization. In addition, decellularization protocol with PBS and SDS promoted a lower macrophage response when scaffolds were transplanted in vivo in accord with another study (Keane et al. Citation2012).
Figure 1. Rat testis histological analysis. Control (A–D) and decellularized (E–H) testis under macroscopic view, hematoxylin and eosin and Picrosirius Red staining. (E) Through the macroscopic view, it is possible to observe a color change in the samples, becoming translucent after decellularization. (B,F) Hematoxylin and eosin staining shows the presence of cells in the control tissue and the absent of it in decellularized scaffolds. (H) Picrosirius Red staining under polarized light shows an apparent greater amount of collagen in decellularized testis. Seminiferous tube (ST), decellularized seminiferous tube (dST), and interstice (I); spermatogonia (thick arrow); Sertoli cell (thin arrow); myoid cells (M); slender (in yellow) and thick (in red) collagen fibers (G–H). Scale bar: (A, E) 1 cm; (B) 50 µm; (C,D) and (F, H) 20 µm.
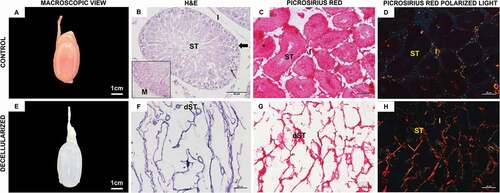
Figure 2. gDNA quantification and DAPI fluorescence. (A) The level of decellularization was quantified with genomic DNA extracted from the control tissue and decellularized testis. DNA quantification revealed a reduction in DNA amount between the control and decellularized rat testis (p = 0.019, Student’s t-test) subjected to decellularization treatment. (B, C) Through DAPI fluorescence, it is possible to observe the absence of cell nuclei in decellularized testis according to the results of DNA quantification. Scale bar: (B, C) 100 µm.
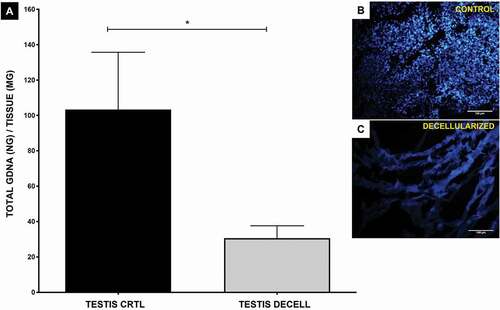
The preservation of the ECM structure and its components are pivotal for an effective recellularization procedure since cells must attach and multiply on its three-dimensional structure. The main known components of ECMs are fibrous proteins such as collagens, fibronectin, laminin, elastin, glycoproteins, and glycosaminoglycans (GAGs) (Theocharis et al. Citation2016). The histology and the immunohistochemistry assays performed after the decellularization process demonstrated that some of these ECM components were preserved. The Picrosirius Red staining allows the observation mainly of collagens types I and III in the ECMs based on their birefringent properties (Rittié Citation2017). The decellularized and control samples showed the presence of slender and thick collagen fibers (, ), similar to previous observations (Akbarzadeh et al. Citation2019). In relation to immunohistochemistry, a high expression of fibronectin and collagen types I and III in the interstitial and interlobular area of the decellularized tissue compared with the control was shown ( and ). The laminin is present throughout the testicular tissue, including the lumen, the interstitial space and myoid cells (, H). These results indicate that ECM architecture of these samples was not damaged since the decellularized tissues preserved the structural proteins (collagens) and adhesive glycoproteins (fibronectin and laminin). It is possible to observe the presence of cells within seminiferous tubules of the control samples. In decellularized samples, these cells were not present, being visible only the elastic fibers of the ECM (). This is an indication that the decellularization was efficient and the matricellular structure was preserved.
Figure 3. Rat testis immunohistochemistry. (A–D) Control testis samples and decellularized samples ECM (E–H). Both control and decellularized testis showed a positive reaction for collagen I (A, E), collagen III (B, F), fibronectin (C, G), and laminin (D, H), with laminin expression being apparently higher in both control and decellularized testis. From this, it is possible to observe that the components of the ECM were preserved after decellularization (EH). Arrow (positive expression); asterisk (negative control); seminiferous tube (ST); interstice (I); Testicular parenchyma (P). (B) and (D–H) 50 µm; (C) 20 µm.
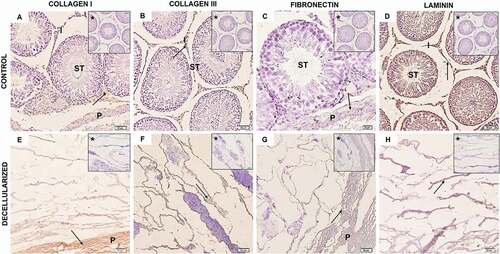
The preservation of the ECM is necessary for stem cell adhesion on the surface of the testicular basement membrane epithelium (Baert et al. Citation2015; Vermeulen et al. Citation2018; Talebi et al. Citation2019). ECM not only promotes the mechanical support of a tissue, but also plays an important role in biological processes such as cell growth, migration, and differentiation. By SEM, the technique used for characterization of cell and tissue surface topography (Cohen Hyams, Mam, and Killingsworth Citation2020), it is possible to observe the presence of cells at the seminiferous tubes in control samples (), and in decellularized samples it is only visible the maintenance of collagen and elastic fibers of the ECM without nuclei (). This indicates that the decellularization was efficient and that the matricellular structure was preserved.
Figure 4. Rat testis SEM. (A, B) The seminiferous tubules and their cells can be seen in the testis of a control rat (yellow arrow). (C, D) It is possible to view the decellularized testis ECM architecture, without cells, and preserved structural proteins, such as collagens fibers (red star). (A, C, G) 30 µm; (B) 300 µm; (D) 1 µm; (E, F) 10 µm.
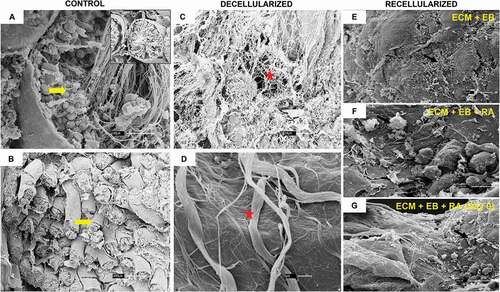
The use of bioscaffolds for the in vitro cell culture is an established method with multiple ways of being achieved (Macedo Citation2012; Keane et al. Citation2012; Laronda et al. Citation2015; Forbes et al. Citation2018; Vermeulen et al. Citation2018; Pieri et al. Citation2019; Talebi et al. Citation2019). In this report, we show that the procedure of rat decellularization using 1% SDS, the same used throughout this research, allowed the culture of SSCs. In an innovative manner, we chose the culture of EB in the presence of RA on decellularized rat testis ECM.
RA is produced from the metabolism of vitamin A and is required for spermatogenesis. Once absorbed, it signals the differentiation of spermatogonial type A to spermatogonial type B (Helsel and Griswold Citation2019; Li et al., Citation2019a; Teletin et al. Citation2017). For example, vitamin A-deficient mice are unable to produce male gametes since there is a lack of RA. This problem is easily solved with the exogenous administration of vitamin A (N. Li et al. 2019; Teletin et al. Citation2017; Helsel and Griswold Citation2019). Moreover, RA has been linked to the differentiation of EBs in SSCs instead of oocytes in cultured colonies (Kerkis et al. Citation2007). It was also established that germ cells also play a role in regulating the level of RA production (Helsel and Griswold Citation2019). Amini Mahabadi et al. (Citation2020) demonstrated mouse iPSC (miPSCs) differentiation into male germ cells in vitro via the formation of embryoid body by RA /EB induction, causing in cell capacity for expressing genes of germ cells. Furthermore, with the addition of RA and progesterone (P) on day 7. Mahabadi et al. (Citation2020) improved the efficiency of differentiating miPSCs into male germ cells.
By SEM () and DAPI, antibodies and protein staining analysis verified the growth and attachment of the EBs to the decellularized testis and the change in the ECM pattern according to the development and differentiation of the cells. As expected, the control group samples () I–DIVshowed a lower number of cells than samples T1 () and T2 I–DIV (), I–AIVwhile the higher number of stained cells were marked with HSD17B3 that indicates Sertoli cells in embryonic and prepubertal development. The HSDB3 enzyme acts in the final’s steps of testosterone production, and it is expressed in the Sertoli cells during embryonic development and prepubertal period. Atrophy and feminization of the genitals occur during the deficiency of HSDB3 in male patients, while in mice its absence is correlated with puberty delay and reduced maturation of Leydig cells (Rebourcet et al. Citation2020; Sipilä et al. Citation2020).
Figure 5. Recellularization Immunofluorescence analysis of control. group. (AI–DIV) Control group: ECM + EB. Embryoid bodies (EB); extracellular matrix (ECM). Red arrow indicates regions of cellular antibody-positive expression, and yellow arrow indicates cellular antibody and matrix antibody overlap. Observe positive expression of ACRV1 (AI–AIV), indicating the differentiation in prostate gland cells; GDNF (BI–BIV), indicating the presence of Sertoli cells and SSCs; HSD17B3 (CI–CIV), indicating the proliferation of Leydig cells or the presence of EBs; TRIM36 (DI–DIV) express the proliferation of prostate cells in testis scaffold. Laminin antibody evidencing the ECM. Scale bar: (AI–DIV) 20 µm.
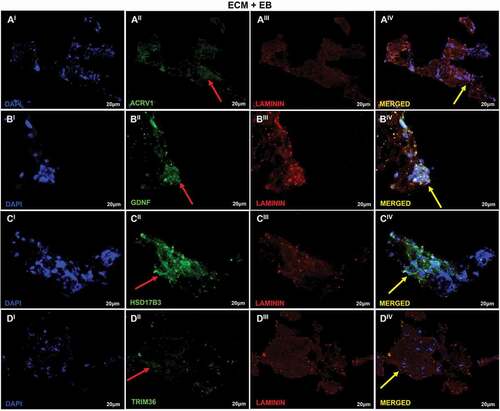
Figure 6. Recellularization Immunofluorescence analysis of T1 group. (AI–DIV) T1: ECM + EB + 0.1 µM RA (added when EBs were formed). Embryoid bodies (EB); extracellular matrix (ECM); retinoic acid (RA). Red arrow indicates regions of cellular antibody-positive expression, and yellow arrow indicates cellular antibody and matrix antibody overlap. In this analysis, the cells show positive expression for the antibodies ACRV1 (AI–AIV), indicating the differentiation in prostate gland cells; GDNF (BI–BIV), indicating the presence of Sertoli cells and SSCs; HSD17B3 (CI–CIV), indicating the proliferation of Leydig cells or the presence of EBs; TRIM36 (DI–DIV) shows the proliferation of prostate cells in testis scaffold. Laminin antibody evidencing the ECM. Scale bar: (AI–DIV) 20 µm.
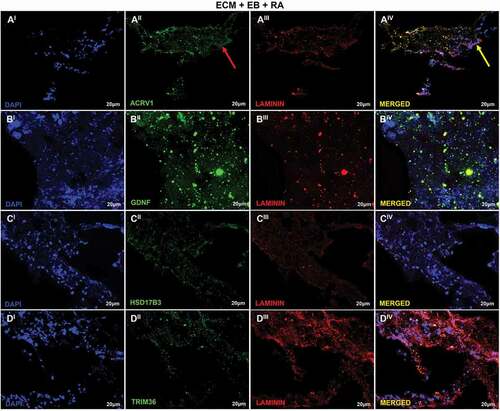
Figure 7. Recellularization Immunofluorescence analysis of T2 group. (AI–DIV) T2: ECM + EB + AR – day 0 (RA added at first day of recellularization assay). Embryoid bodies (EB); extracellular matrix (ECM); retinoic acid (RA). Red arrow indicates regions of cellular antibody-positive expression, and yellow arrow indicates cellular antibody and matrix antibody overlap. Positive expression of ACRV1 (AI–AIV) showing the differentiation in prostate gland cells; GDNF (BI–BIV), with high expression evidencing the presence of Sertoli cells and SSCs; HSD17B3 (CI–CIV), high expression indicating Sertoli cells in embryonic and prepubertal development; TRIM36 (DI–DIV), reasonable expression indicating some proliferation of prostate cells. Laminin antibody evidencing the ECM. Scale bar: (AI–DIV) 20 µm.
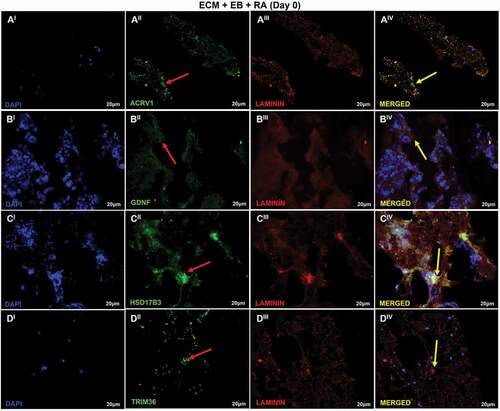
HSD17B3 was the only antibody that stained higher in control and T2 samples compared to T1 group. This could indicate a lower Leydig cells proliferation or the differentiation of EBs in the T1 sample. Even though there was a significant amount of cell proliferation in the T2 group, there was an even higher staining of cells in the T1 samples. Since the use of anti-GFRA-1 showed that cells lacking GFRA1 tend to yield into colonies, the possibilities of germ cells being able to survive in a GDNF-poor environment have been questioned (Takashima et al. Citation2015). Germ cells can also be verified by GFRα1 staining (Wang et al. Citation2016).
The T1 group showed a higher marker for GDNF that indicates a greater presence of Sertoli cells and SSCs. GDNF is produced by Sertoli cells in increased levels right after birth in rats and prompts the SSC’s self-renewal. In low levels, the GDNF has been associated with SSC’s differentiation. For these reasons, the GDNF acts as a marker for SSCs (Huleihel et al. Citation2013; Takashima et al. Citation2015). The samples of T1 group were higher stained for GDNF than the control group and T2 samples, reinforcing the importance of RA in the SSC’s metabolism.
Group T2 further stained for HSD17B3. There was also a superior staining with ACRV-1 that indicates prostate gland cells in T1 and T2 samples when compared to control, with no remarkable difference between the amount of staining of these groups. ACRV1 rabbit polyclonal antibody can stain SP-10 proteins that take part in the regulation of spermatogenesis. Its presence in both mice and human testis serves to pinpoint spermatozoids in the semen and spermatids in the seminiferous epithelium (Tang et al. Citation2012; Kumar and Singh Citation2020). ACRV1 rabbit polyclonal antibody stained the same number of cells in both T1 and T2 groups. Thus, both groups could produce spermatids in their environment even though there was a higher staining for SSCs in T1 sample. The TRIM-36 gene is responsive to androgen and has been shown to be a component of = yak and cattle spermatogenesis. It is also correlated with a higher survival rate in human prostate cancer patients, being highly expressed in testis cells (Yoshigai et al. Citation2009; Cai et al. Citation2017). TRIM-36 gene was present in higher amount in the control and T1 samples with higher proliferation of prostate cells in these samples.
In vitro differentiation of germ cells and gamete production in mice was first accomplished (Nayernia et al. Citation2006) and since then has been repeated with multiple techniques (de Rooij and Grootegoed Citation1998; Hayashi et al. Citation2011; Baert et al. Citation2015). Factors such as Eif2s3y, GDNF, and FGF2-dependent were shown to promote spermatogonial stem cells differentiation, propagation, and population renewal (Mirzapour et al. Citation2012; Huleihel et al. Citation2013; Busada et al. Citation2015; Takashima et al. Citation2015; Clotaire et al. Citation2019; Helsel and Griswold Citation2019). The principal barrier to germ cell specification in humans is replicating the pluripotency of epiblast-like cells (EpiLCs) of mice and other murine species, even though SSCs of pigs cultivated in vitro and xenotransplant to mice produced in vivo spermatozoa (Hayashi et al. Citation2011).
Substantial additional studies are required before we can promote SSC’s transplantation and in vitro differentiation from pluripotent embryonic stem cells in human patients with male infertility. The present study reinforced the importance of RA in the cultivation of in vitro SSCs and the potential of EB during the differentiation of testicular epithelial cells, SSCs, and spermatids. Due to the limitation of RA-conjugated EB culture, further analysis aiming at cell/ECM interaction should be carried out for extended periods in vitro.
Materials and methods
Samples
Five adults male Wistar rats (body mass range: 350–550 g) were obtained from Central Animal Facility of University of São Paulo. Then, animals were euthanized by administering 30 mg/kg (I.P.) of xylazine and 300 mg/kg (I.P.) of ketamine. Euthanasia was confirmed due to the heart and respiratory rate absence, and the testis were collected for decellularization assay.
Decellularization assay
Three (03) testis were cannulated with a 26 G catheter by vas deferens, tied with surgical nylon 6.0 thread (1.5 cm). A peristaltic pump (Harvard Peristaltic Pump – Harvard Apparatus) was used for tissue perfusion. Initially, blood excess was removed by a slow infusion of 0.5 ml/min (2 h) in phosphate-buffered saline (PBS). Afterward, it was perfused 1 ml/min for 24 h of 1% sodium dodecyl sulfate (SDS, #13-1313-01, LGC Biotechnology). Then, it waswashed with distilled water (0.5 ml/min) for 2 h. The protocol was conducted at room temperature. All solutions were supplemented with 0.5% antibiotic penicillin/streptomycin (ATB, #BR30110-01, LGC Biotechnology).
Decellularization validation
Firstly, snap-frozen control and decellularized samples from rat testis were used to genomic DNA isolation as described by Matias et al. Citation2018. For histological analysis, the 4% PFA fixed sample was routinely processed for hematoxylin and eosin (H&E) and fluorescence with DAPI and Picrosirius Red stains to verify the nuclei absence and collagen maintenance, respectively. To verify the ECM architecture maintenance and cell adhesion, scanning electron microscopy (SEM) analysis was performed as described by Matias et al. Citation2018.
Immunohistochemistry analysis
The control and decellularized testis samples were analyzed by immunohistochemistry using Dako EnVision™ FLEX detection system according to the manufacturer’s instructions. For ECM evaluation, the following primary antibodies were applied: primary antibodies anti-collagen I (#600-401-103S, 1:400; Rockland), anti-collagen III (#sc-8779, 1:100; Santa Cruz), anti-laminin subunit α-2 (#bs-8561R, 1:200; Bioss Antibodies), and anti-fibronectin (#NBP1-91258, 1:200; Novus Biologicals). Finally, the slides were mounted and visualized using a Nikon Eclipse 80I microscope.
mESC testis recellularization assay
After decellularization validation, rat testis scaffolds were sliced in fragments (0.5 × 0.5 cm) and sterilized according to Fratini et al. Citation2018. Briefly, the samples were pre-sterilized using critical point machine (LEICA IN CPD 300). Then, they were washed thrice with PBS and 0.5% ATB for 5 min each. Later, they were kept under ultraviolet light for another 15 min. The mouse embryonic cells (mESCs) were derived from a stem cell lineage (H106 male) established by Dr. Joaquim Manzano Garcia and Dr. Lawrence Charles Smith (Montreal University). Decellularized testis scaffolds were placed in non-adherent culture plate (24 wells, Sarstedt) and cultured with 5 × 104 cells of mESC. EB production was carry out by fetus fibroblast washing with Dulbecco’s phosphate buffered saline (DPBS) dissociated with EDTA 0.5% (Invitrogen) neutralized with DMEM (Invitrogen) and fetal bovine serum 10% (Invitrogen). The cell suspension was prepared placing the embryonic fibroblast with 0.2% jelly, providing a quick adherence. The EBs were separated in three groups: (1) control: ECM + EB; (2) T1: ECM + 0.1 µM RA (RA, Sigma, St. Louis, MO); and (3) T2: ECM + EB + AR (day 0 – RA added at first day of recellularization assay). Scaffolds and cells were maintained in contact for 7 days at 37°C with 5% CO2. Afterward, samples were collected for immunofluorescence analysis.
Immunofluorescence analysis
Recellularized testis were frozen using Tissue-Tek OCT solution (# 4583-1, Sakura-Torrance, USA). Cryostat sections were performed (CM1520, Leica) and transferred to silanized slides. Then, the cuts were 4% PFA fixed for 10 min, washed thrice with PBS supplemented with 0.5% of Tween-20, followed by primary antibody incubation: anti-HSD17B3, Proteintech, #13415-1-AP; anti-GDNF, Abcam, #ab84106; anti-ACRV1, Proteintech, #14040-1-AP; and anti-TRIM36, Abcam, #ab116212 at 37°C for 1 h. Then, they were incubated for 1 h at 4°C with secondary antibody Alexa Fluor 568 (#A30008, Thermo Fisher) or Alexa Fluor 488 (#A-11094, Thermo Fisher), and nuclear stained with DAPI for 15 min. Between each step, samples were washed thrice with PBS supplemented with 0.5% of Tween-20 (#178019, Synth). Finally, samples were analyzed with a confocal microscope (Olympus Fluo View™ 1000).
Ethics approval for animal study
The manuscript`s experiments were conducted by the rules of Ethics Committee in Use of Animals from School of Veterinary Medicine and Animal Science of University of São Paulo (number 67862210195).
Authors’ contributions
Conducted experiments: VFB, GSSM, ACOC, DRS, FJMJ, JMG, MAM; project conceptualization: MAM, JMG; wrote and interpreted data: VFB, GSSM, ACOC, LCS, RR, MSA, MAM; funding: MAM, JMG. All authors read and approved the final manuscript.
Acknowledgments
To Advanced Center of Image Diagnosis (CADI-FMVZ-USP) for supporting the microscope analyses (Rose Eli Rici and Diogo Palermo). To Dr. Antônio Assis Neto (FMVZ-USP) for antibodies donating to evaluation of the recellularization process and to Diogo Palermo for helping with histological sections.
Disclosure statement
No potential conflict of interest was reported by the author(s).
Additional information
Funding
References
- Akbarzadeh A, Kianmanesh M, Fendereski K, Ebadi M. 2019. Decellularised whole ovine testis as a potential bio-scaffold for tissue engineering. Reprod Fertil Dev. 31(11):1665–1673. doi:https://doi.org/10.1071/RD19070.
- Alves-Lopes JP, Stukenborg JB. 2018. Testicular organoids: a new model to study the testicular microenvironment in vitro? Hum Reprod Update. 24(2):176–191. https://doi.org/10.1093/humupd/dmx036. Oxford Academic:.
- Baert Y, Stukenborg JB, Landreh M, De Kock J, Jornvall H, Soder O, Goossens E. 2015. Derivation and characterization of a cytocompatible scaffold from human testis. Human Reproduction. 30(2):256–267. doi:https://doi.org/10.1093/humrep/deu330.
- Bhongade MB, Prasad S, Jiloha RC, Ray PC, Mohapatra S, Koner BC. 2015. Effect of psychological stress on fertility hormones and seminal quality in male partners of infertile couples. Andrologia. 47(3):336–342. doi:https://doi.org/10.1111/and.12268.
- Busada JT, Chappell VA, Niedenberger BA, Kaye EP, Keiper BD, Hogarth CA, Geyer CB. 2015. Retinoic acid regulates kit translation during spermatogonial differentiation in the mouse. Dev Biol. 397(1):140–149. doi:https://doi.org/10.1016/j.ydbio.2014.10.020.
- Cai X, YuShumin S, Mipam T, Yang F, Zhao W, Liu W, Cao S, et al. 2017. Comparative analysis of testis transcriptomes associated with male infertility in cattleyak. Theriogenology. 88(January):28–42. doi:https://doi.org/10.1016/j.theriogenology.2016.09.047.
- Clotaire D, John Z, Wei Y, Xiuwei Y, Ousman T, Hua J. 2019. Functions of promyelocytic leukaemia zinc finger (Plzf) in male germline stem cell development and differentiation. Reprod Fertil Dev. 31(8):1315. doi:https://doi.org/10.1071/RD18252.
- Costa A, Naranjo JD, Londono R, Badylak SF. 2017. Biologic scaffolds. Cold Spring Harb Perspect Med. 7(9):a025676. doi:https://doi.org/10.1101/cshperspect.a025676.
- Crapo PM, Gilbert TW, Badylak SF. 2011. An overview of tissue and whole organ decellularization processes. Biomaterials. 32(12):3233–3243. doi:https://doi.org/10.1016/j.biomaterials.2011.01.057.
- de Rooij, Dirk G DG, Anton Grootegoed J. 1998. Spermatogonial stem cells. Curr Opin Cell Biol. 10(6):694–701. doi:https://doi.org/10.1016/S0955-0674(98)80109-9.
- Dong G, Hildorf P, Kristensen H, Cortes T, Andersen. 2019. Xeno-free propagation of spermatogonial stem cells from infant boys. Int J Mol Sci. 20(21):5390. doi:https://doi.org/10.3390/ijms20215390.
- Forbes CM, Flannigan R, Schlegel PN. 2018. Spermatogonial stem cell transplantation and male infertility: current status and future directions. Arab Journal of Urology. 16(1):171–180. doi:https://doi.org/10.1016/j.aju.2017.11.015.
- Fratini P, Rigoglio NN, Gustavo DSSM, Carreira ACO, Rose EGR, Maria AM. 2018. Canine placenta recellularized using yolk sac cells with vascular endothelial growth factor. Biores Open Access. 7(1):101–106. doi:https://doi.org/10.1089/biores.2018.0014.
- Greil AL, Slauson-Blevins K, McQuillanJulia J. 2010. The experience of infertility: a review of recent literature. Sociol Health Illn. 32(1):140–162. doi:https://doi.org/10.1111/j.1467-9566.2009.01213.x.
- Gupta SK, Mishra NC. 2018. Decellularization methods for scaffold fabrication. Methods in Molecular Biology (Clifton, N.J.). 1577:1–10. doi:https://doi.org/10.1007/7651_2017_34
- Hayashi K, Ohta H, Kurimoto K, Aramaki S, Saitou M. 2011. Reconstitution of the mouse germ cell specification pathway in culture by pluripotent stem cells. Cell. 146(4):519–532. doi:https://doi.org/10.1016/j.cell.2011.06.052.
- Helsel A, Griswold MD. 2019. Retinoic acid signaling and the cycle of the seminiferous epithelium. Current Opinion in Endocrine and Metabolic Research. 6:1–6. doi:https://doi.org/10.1016/j.coemr.2019.01.001
- Huleihel M, Fadlon E, AbuElhija A, Piltcher Haber E, Lunenfeld E. 2013. Glial Cell Line-Derived Neurotrophic Factor (GDNF) induced migration of spermatogonial cells in vitro via MEK and NF-KB pathways. Differentiation. 86(1–2):38–47. doi:https://doi.org/10.1016/j.diff.2013.06.005.
- Hyams C, Tzipi KM, Killingsworth MC. 2020. Scanning electron microscopy as a new tool for diagnostic pathology and cell biology. Micron. 130:1027–1097. Elsevier Ltd:. doi:https://doi.org/10.1016/j.micron.2019.102797
- Keane TJ, Londono R, Turner NJ, Badylak SF. 2012. Consequences of ineffective decellularization of biologic scaffolds on the host response. Biomaterials. 33(6):1771–1781. https://doi.org/10.1016/j.biomaterials.2011.10.054. Elsevier.
- Kerkis A, Simone AS, Fonseca RC, Serafim, Lavagnolli TMC, Abdelmassih S, Roger A, Irina K. 2007. In vitro differentiation of male mouse embryonic stem cells into both presumptive sperm cells and oocytes. Cloning Stem Cells. 9(4):535–548. doi:https://doi.org/10.1089/clo.2007.0031.
- Kumar N, Singh NK. 2020. ‘Emerging role of novel seminal plasma bio-markers in male infertility: a review.’. European Journal of Obstetrics & Gynecology and Reproductive Biology. 253(October):170–179. doi:https://doi.org/10.1016/j.ejogrb.2020.08.015.
- Laronda MM, Jakus AE, Whelan KA, Wertheim JA, Shah RN, Woodruff TK. 2015. Initiation of puberty in mice following decellularized ovary transplant. Biomaterials. 50(May):20–29. doi:https://doi.org/10.1016/j.biomaterials.2015.01.051.
- Li N, MaWentao W, Shen Q, Zhang M, DuZhaoyu Z, WuChongyang C, Niu B, Liu W, Hua J. 2019a. Reconstitution of male germline cell specification from mouse embryonic stem cells using defined factors in vitro. Cell Death Differ. 26(10):2115–2124. doi:https://doi.org/10.1038/s41418-019-0280-2.
- Li X, Long X-Y, Xie Y-J, Zeng X, Chen X, MoZhong-cheng Z-C. 2019b. The roles of retinoic acid in the differentiation of spermatogonia and spermatogenic disorders. Clinica Chimica Acta. 497(October):54–60. doi:https://doi.org/10.1016/j.cca.2019.07.013.
- Lim JJ, Shim MS, Lee JE, Lee DR. 2014. “Three-step method for proliferation and differentiation of Human Embryonic Stem Cell (HESC)-derived male germ cells.” Edited by Hyunjung Jade Lim. PLoS ONE. 9(4):e90454. https://doi.org/10.1371/journal.pone.0090454. Public Library of Science:.
- Macedo C. 2012. Uso Do Sistema de Cultivo Bidimensional e Tridimensional Para Diferenciação de Células-Tronco Espermatogoniais e Indução Da Espermatogênese in Vitro Em Murinos. Aleph. 14:56. Universidade Estadual Paulista:.
- Mahabadi A, Javad MK, Aghighi F, Enderami SE, Hosseini ES, Talaei SA, Hayat SMG, Nikzad H. 2020a. Retinoic acid and 17β‐estradiol improve male germ cell differentiation from mouse‐induced pluripotent stem cells. Andrologia. 52(2):e13466. https://doi.org/10.1111/and.13466. John Wiley & Sons, Ltd:.
- Mahabadi JA, Tameh AA, Talaei SA, Karimian M, Rahiminia T, Enderami SE, Seyed MGH, NikzadHossein H. 2020b. Retinoic acid and/or progesterone differentiate mouse induced pluripotent stem cells into male germ cells in vitro. J Cell Biochem. 121(3):2159–2169. https://doi.org/10.1002/jcb.29439. John Wiley & Sons, Ltd:.
- Matias GDSS, Rigoglio NN, Carreira ACO, Romagnolli P, Barreto RDSN, Mess AM, Miglino MA, Fratini P. 2018. Optimization of Canine Placenta decellularization: an alternative source of biological scaffolds for regenerative medicine. Cells Tissues Organs. 205(4):217–225. doi:https://doi.org/10.1159/000492466.
- Mirzapour T, Movahedin M, Tengku Ibrahim TA, Koruji M, Haron AW, Nowroozi MR, Rafieian SH. 2012. Effects of basic fibroblast growth factor and leukaemia inhibitory factor on proliferation and short-term culture of human spermatogonial stem cells. Andrologia. 44(SUPPL.1):41–55. doi:https://doi.org/10.1111/j.1439-0272.2010.01135.x.
- Nagano M, Avarbock MR, Leonida EB, Brinster CJ, Brinster RL. 1998. Culture of mouse spermatogonial stem cells. Tissue Cell. 30(4):389–397. doi:https://doi.org/10.1016/S0040-8166(98)80053-0.
- Nayernia K, Nolte J, Michelmann HW, Lee JH, Rathsack K, Drusenheimer N, Dev A, et al. 2006. In vitro-differentiated embryonic stem cells give rise to male gametes that can generate offspring mice. Dev Cell. 11(1):125–132. doi:https://doi.org/10.1016/j.devcel.2006.05.010.
- Ogawa T, Aréchaga JM, Avarbock MR, Brinster RL. 1997. Transplantation of testis germinal cells into mouse seminiferous tubules. International Journal of Developmental Biology. 41(1):111–122. doi:https://doi.org/10.1387/ijdb.9074943.
- Pieri N, Godoy C, Mançanares ACF, de Souza AF, Fernandes H, Diaza AMG, Bressan FF, Roballo KCS, et al. 2019. Xenotransplantation of Canine Spermatogonial Stem Cells (CSSCs) regulated by FSH promotes spermatogenesis in infertile mice. Stem Cell Res Ther. 10(1):135. doi:https://doi.org/10.1186/s13287-019-1250-9.
- Rebourcet D, Mackay R, Darbey A, Curley MK, Jørgensen A, Frederiksen H, Mitchell RT, O’Shaughnessy PJ, Nef S, Smith LB. 2020. Ablation of the canonical testosterone production pathway via knockout of the steroidogenic enzyme HSD17B3, Reveals a novel mechanism of testicular testosterone production. The FASEB Journal. 34(8):10373–10386. doi:https://doi.org/10.1096/fj.202000361R.
- Rittié L. 2017. Fibrosis. In: Laure R, editor. Methods in molecular biology.
- Sipilä P, Junnila A, Hakkarainen J, Huhtaniemi R, Mairinoja L, Zhang FP, Strauss L, et al. 2020. The Lack of HSD17B3 in male mice results in disturbed leydig cell maturation and endocrine imbalance akin to humans with HSD17B3 deficiency. The FASEB Journal. 34(5):6111–6128. doi:https://doi.org/10.1096/fj.201902384R.
- Takashima S, Kanatsu-Shinohara M, Tanaka T, Morimoto H, Inoue K, Ogonuki N, Jijiwa M, Takahashi M, Ogura A, Shinohara T. 2015. Functional differences between GDNF-dependent and FGF2-dependent mouse spermatogonial stem cell self-renewal. Stem Cell Reports. 4(3):489–502. doi:https://doi.org/10.1016/j.stemcr.2015.01.010.
- Talebi A, Sadighi-Gilani MA, Koruji M, Ai J, Navid S, Rezaie MJ, Jabari A, et al. 2019. Proliferation and differentiation of mouse spermatogonial stem cells on a three-dimensional surface composed of PCL/Gel nanofibers. International Journal of Morphology. 37(3):1132–1141. doi:https://doi.org/10.4067/S0717-95022019000301132.
- Tang A, Yan Q, Sun L, Diao R, Yu Z, Zhang Z, Gui Y, Cai Z. 2012. Developmental expression of ACRV1 in humans and mice. Andrologia. 44(1):16–22. doi:https://doi.org/10.1111/j.1439-0272.2010.01095.x.
- Teletin M, Vernet N, Ghyselinck NB, Mark M. 2017. Roles of retinoic acid in germ cell differentiation. Curr Top Dev Biol. 125:191–225. doi:https://doi.org/10.1016/bs.ctdb.2016.11.013
- Theocharis AD, Skandalis SS, Gialeli C, Karamanos NK. 2016. Extracellular matrix structure. Adv Drug Deliv Rev. 97(February):4–27. doi:https://doi.org/10.1016/j.addr.2015.11.001.
- Vermeulen M, Del VentoFederico F, de MicheleFrancesca F, Poels J, Wyns C. 2018. Development of a cytocompatible scaffold from pig immature testicular tissue allowing human sertoli cell attachment, proliferation and functionality. Int J Mol Sci. 19(1):227. doi:https://doi.org/10.3390/ijms19010227.
- Wang H, Xiang J, Zhang W, LiJunhong J, Wei Q, Zhong L, Ouyang H, Han J. 2016. Induction of germ cell-like cells from porcine induced pluripotent stem cells. Sci Rep. 6(1):27256. doi:https://doi.org/10.1038/srep27256.
- Yoshigai E, Kawamura S, Kuhara S, Tashiro K. 2009. Trim36/Haprin plays a critical role in the arrangement of somites during xenopus embryogenesis. Biochem Biophys Res Commun. 378(3):428–432. doi:https://doi.org/10.1016/j.bbrc.2008.11.069.
- Zogbi C, Tesser RB, Encinas G, Miraglia SM, Stumpp T. 2012. Gonocyte development in rats: proliferation, distribution and death revisited. Histochem Cell Biol. 138(2):305–322. doi:https://doi.org/10.1007/s00418-012-0955-y.