Abstract
Diabetes mellitus induced hyperglycemia increases oxidative stress, which contributes to impairment of male reproductive function. Aquaporins (AQPs) belong to a transmembrane protein superfamily containing 13 isoforms (AQP0-12), differentially expressed in various organs, and play a pivotal role in male reproductive function. In the current study, we investigated the relationship between AQPs and testicular steroidogenesis under hyperglycemia in vivo and in vitro. The effect of high glucose on the role of AQPs in Leydig cell steroidogenesis was analyzed in diabetic rats (in-vivo) and LC540 rat Leydig cells (in vitro) via enzyme assays, quantitative RT-PCR, siRNA knock down and western blotting. AQP 9 was significantly up-regulated in STZ-induced diabetic rat testis and high glucose treated LC540 cells. Further, oxidative stress marker nuclear factor erythroid 2-related factor 2 (Nrf2) expression was decreased with impaired testicular steroidogenesis under hyperglycemia. Knock-down of AQP 9 resulted in increased Nrf2 expression and thus increased testicular steroidogenesis in hyperglycemia. Diabetes-associated hyperglycemia induced oxidative stress is a widely proven cause for diabetes-related male infertility. Our results collectively suggest that AQP 9 impairs testicular steroidogenesis via the regulation of oxidative stress in diabetes.
Introduction
Diabetes mellitus (DM) is a multifactorial metabolic disorder characterized by hyperglycemia, resulting from defects in insulin secretion, insulin action, or both (American Diabetes Association Citation2013). The prevalence of diabetes globally was 425 million people in 2017 and is estimated to rise by 629 million in 2045 (Saeedi et al. Citation2019). In United States, one in five adults and one in four young adults are prediabetic, providing an unfavorable condition for the development of diabetic complications, either macrovascular or microvascular such as diabetic nephropathy, retinopathy, neuropathy, cardiovascular diseases and sexual dysfunction, which can be prevented if diabetes is managed at early stages (Ahmad Citation2016; Papatheodorou et al. Citation2016; Khalil Citation2017; Maresch et al. Citation2018; Andes et al. Citation2020; Barkabi-Zanjani et al. Citation2020; Song et al. Citation2020). The widespread increase in DM worldwide affects young individuals in the reproductive age group as there is a rise in the number of childhood and adolescent males with DM (Nagarathna et al. Citation2020). Hyperglycemia increases oxidative stress, which contributes to the impairment of insulin function/secretion and further, plays a pivotal role in the impairment of male reproductive function (Newsholme et al. Citation2019; Lei et al. Citation2020). Recently, the diffusion of H2O2 across the plasma membrane to the extracellular fluid has also been regarded as a potential ROS elimination pathway (Sies Citation2014).
Aquaporins (AQPs) belong to a transmembrane protein superfamily containing 13 isoforms (AQP0-12), differentially expressed in various organs and tissues involved in fluid absorption and/or excretion, energy metabolism, solute diffusion across transmembrane, cellular trafficking, biological functions and plays a vital role in preserving the cellular environment (Azad et al. Citation2021). Alteration in the expression, function and/or regulation of AQPs affects pathophysiology of various clinical conditions viz edema, cataract, obesity, cell mobility, wound healing, Sjögren’s syndrome, cancer, diabetes, metabolic disorders and reproductive function (Wang et al. Citation2015; da Silva and Soveral Citation2017; Dajani et al. Citation2018; Ala et al. Citation2021; Mirabella et al. Citation2021; Ribeiro et al. Citation2021). AQPs differential expression and distribution in the efferent ductules and in the epididymal segments of cryptorchid canines suggests their involvement in luminal microenvironment modifications (Squillacioti et al. Citation2021). Recent studies suggest that, AQPs have a great potential for diagnostics and a target for therapy in diseases of altered water homeostasis (Dajani et al. Citation2018; Ichiyama et al. Citation2018; Meli et al. Citation2018; Su et al. Citation2020; Clarke-Bland et al. Citation2022)
Alterations in plasma osmolality and fluid body volume disturbs the water metabolism, which may give rise to adverse pathological conditions. AQPs expression is susceptible to modification, since stress response involves changes in cell volume and/or movement of specific solutes. Oxidative stress promotes detrimental changes during spermatogenesis, epididymal maturation, and sperm capacitation leading to infertility is also strongly associated with altered expression of AQPs in the male reproductive physiology and function (Calamita et al. Citation2001; Huang et al. Citation2006; Chauvigne et al. Citation2015; Pellavio and Laforenza Citation2021). Moreover, AQPs are also involved in the regulation of steroidogenesis and regulates steroid hormones in developing epididymis, maintaining male fertility (Oliveira et al. Citation2005; Shannonhouse et al. Citation2014).
Despite many treatment strategies available, patients with diabetes still develop associated complications; thus, it is necessary to understand the molecular mechanisms to prevent diabetic complications. The wide distribution of AQPs in male reproductive tissues emphasizes that water permeability is crucial in maintaining male fertility; hence we intended to investigate the role of AQPs in testicular steroidogenesis in diabetes.
Results
Effect of hyperglycemia on oxidative stress
STZ is an antibiotic extracted from Streptomyces achromogenes is a diabetogenic tool to induce hyperglycaemia in laboratory studies. In the present study, STZ administered diabetic animals showed a remarkable decrease in body weight along with a significant increase in serum glucose levels compared to control animals. However, body weight was significantly increased (p < 0.01), and glucose levels were dramatically decreased (p < 0.0001) on insulin treatment compared to diabetic animals (). Hyperglycemia determines an imbalance in the consumption of antioxidant enzymes, which are predominantly represented by the enzymes superoxide dismutase (SOD), catalase (CAT) and lipid peroxidation (LPO). The activities of enzymatic antioxidants, ROS levels and LPO of control and experimental groups of rat testis are represented in . The activities of SOD and CAT were significantly decreased in the testis of STZ-induced diabetic rats with a significant increase in ROS. The LPO levels were found to be significantly increased in diabetic rats and the addition of exogenous inducers such as H2O2, ascorbate and FeSO4 further increased LPO levels than basal in diabetic animals. Further, on insulin treatment SOD and CAT activity were increased with a significant decrease in LPO and ROS compared to the DM group.
Figure 1. Effect of hyperglycemia. (A) Body weight of rats received citrate buffer (Control), diabetes (DM) and diabetes rats with insulin treatment (DM + INS) were recorded at 0th day and after 4 weeks; (B) Effect of hyperglycemia on serum glucose levels of control rats (Control), diabetes (DM) and diabetes treated with insulin (DM + INS) were measured before the start of experiment (0th day and after administration of STZ at 1st, 2nd, 3rd and 4th weeks). Values represent mean ± S.D (n = 6). Values are statistically significant at *p < 0.05, **p < 0.01, ***p < 0.001, ****p < 0.0001 and #indicates comparison between DM + INS and DM ##p < 0.01, ####p < 0.0001.
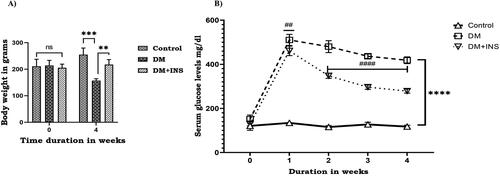
Figure 2. Effect of hyperglycemia on oxidative stress markers. (A) SOD, (B) CAT, (C) ROS and (D) LPO activity in rat testis (LPO was tested at basal level and also with the inducers FeSO4, Ascorbate and H2O2). Enzyme activity for SOD is expressed as unit/mg protein; where one unit is equal to the amount of enzyme required to inhibit auto oxidation of pyrogallol by 50% per minute; CAT is expressed as unit/mg protein; where one unit is equal to moles of H2O2 consumed per minute; ROS is expressed as DCF fluorescence/min/mg protein; lipid peroxidation is expressed as unit/mg protein; where one unit is equal to nmoles of MDA formed. Values represent mean ± S.D (n = 6). Values are statistically significant at *p < 0.05, **p < 0.01, ***p < 0.001 and ****p < 0.0001.
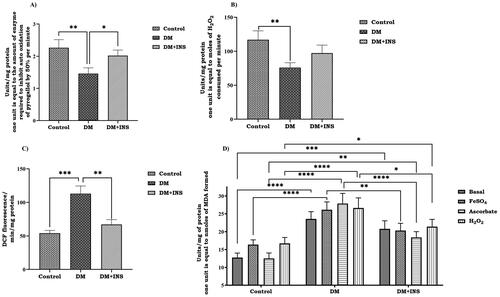
Effect of hyperglycemia on expression of aquaporins (AQPs) in vivo and in vitro
The expression of various AQP isoforms in the testis and Leydig cells were examined using RT-PCR. The total RNA extracted from the testis and Leydig cells contained gene transcripts for a variety of AQPs, including AQP 0, 1, 3, 4, 5, 6,7, 8, 9, 11, and 12 (). The transcript for AQP 2 was not detected either in the testis or Leydig cells and AQP10 was not analyzed as it is a pseudo gene. To determine whether experimental hyperglycemia in vivo or in vitro alters the expression of AQPs in the diabetic testis and Leydig cells, we compared the levels of gene transcripts for AQPs with diabetes and diabetes with insulin treated age-matched control animals. AQP 0 did not have any expression changes in diabetes or insulin-treated testis compared to controls further, AQP 3 and 4 were significantly decreased in diabetic testis compared to controls with a p-value less than 0.01. However, on insulin treatment, AQP3 and 4 were increased compared to DM. Our experimental results indicate a significant increase of AQPs 1, 7, 8, 9, 11, 12 in diabetic testis compared to controls and a significant decrease on insulin treatment compared to DM. AQPs 5 and 6 were significantly increased in diabetic testis compared to controls but on insulin treatment, AQP 5 remained unaltered with AQP 6 being downregulated. Specifically, AQP 9 was increased 36-fold compared to controls, on insulin treatment significantly reduced to DM with p-values less than 0.0001.
Figure 3. Effect of hyperglycemia on aquaporins gene expression. RNA levels in diabetic rat testis and also with insulin treatment (in vivo) were measured by RT-PCR, (A–K). All the results were normalized with β-actin. Values represent mean ± SD (n = 6) (*p < 0.05, **p < 0.01, ***p < 0.001 and ****p < 0.0001).
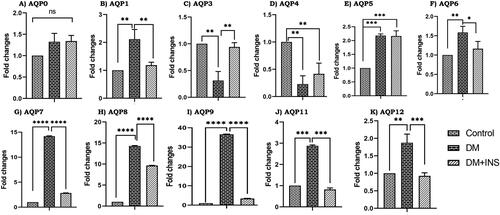
In our experiment, we did not find any expression of AQP 0 in LC540 rat Leydig cells and AQP isoforms 1, 3, 4, 7, 8, 9, 11, 12 were significantly increased with different glucose concentrations compared to normal glucose (5.5 mM). However, AQPs 5 and 6 did not show any significant changes with different glucose concentrations and also with mannitol, but AQP 6 was significantly decreased in 1 mM glucose compared to 5.5 mM glucose (). Moreover, AQPs 1, 4, 9, 12 did not show any significant expression changes on treatment with 30 mM mannitol compared to 5.5 mM glucose, surprisingly AQP 3 was found to be significantly downregulated on mannitol treatment compared to control 5.5 mM glucose. Furthermore, AQPs 7, 8 and 11 were significantly upregulated on mannitol treatment compared to normal glucose. Although AQP 9 was significantly increased to five-fold in high glucose (30 mM glucose) compared to controls, we didn’t observe any significant alteration on mannitol treatment which suggests that high glucose induces the expression of AQP 9. Overall, our results indicate that AQP 9 is significantly increased in-vivo and in-vitro compared to other isoforms under hyperglycemia. Hence, we intended to study the role of AQP 9 in testicular steroidogenesis under hyperglycemia.
Figure 4. Effect of glucose on aquaporins gene expression. RNA levels in LC540 Leydig cells (in-vitro) treated with different concentrations of glucose (1 mM glucose, 5.5 mM glucose, 20 mM glucose, 30 mM glucose, 30 mM mannitol) were measured by RT-PCR (A–J). All the results were normalized with β-actin. Values represent mean ± SD (n = 3) (*p < 0.05, **p < 0.01, ***p < 0.001 and ****p < 0.0001).
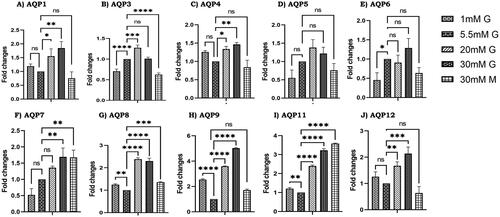
Effect of hyperglycemia on in-vivo and in-vitro expression of AQP9, nrf-2 and testicular steroidogenesis
Steroid hormones regulate essential physiological processes and are associated with various pathological conditions, finely regulated by rate-limiting steroidogenic enzymes 3β-HSD and 17β-HSD. We analyzed the steroidogenic enzymatic activity of both 3β-HSD and 17β-HSD, which were significantly decreased in diabetes testis compared to controls, however, on insulin treatment (DM + INS), enzyme activities were increased compared to DM group but were not significant as shown in . The testosterone levels of the rats observed were dramatically decreased in diabetes group compared with those of the control group and insulin treatment significantly increased the serum testosterone levels (). Our results indicate that insulin treatment promotes the enzyme activity of 3β-HSD and 17β-HSD increasing serum testosterone. In the present study, the expressions of StAR, 3β-HSD and 17β-HSD were markedly downregulated in the DM group as compared with those in the control group. Surprisingly, insulin treatment resulted in significant upregulation in the expressions of StAR, 3β-HSD and 17β-HSD compared to the DM group (). Furthermore, in vitro expression of these key enzymes were studied in LC540 rat Leydig cells treated with different concentrations of glucose. Our results indicated that on treatment with 20 mM and 30 mM glucose StAR, the enzymes 3β-HSD and 17β-HSD were significantly decreased compared to 5.5 mM treated control. There were no significant changes on treatment with 30 mM mannitol as osmotic stress control compared to 5.5 mM glucose treated normal control as shown in the .
Figure 5. Effect of hyperglycemia on 3β-HSD and 17β-HSD enzyme activity and serum testosterone levels. (Control, DM, DM + INS) enzyme activity expressed as units/mg protein, whereas for (A) 3β-HSD one unit is equal to nmoles of NADH formed per minute and for (B) 17β-HSD one unit is equal to nmoles of NADPH oxidized per minute. (C) Effect of hyperglycemia on serum testosterone levels of diabetes rats (Control, DM, DM + INS). Values represent mean ± SD (n = 6) (*p < 0.05, **p < 0.01, ***p < 0.001 and ****p < 0.0001).
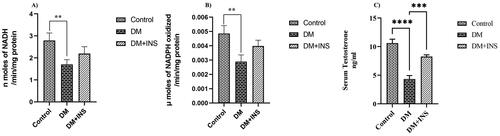
Figure 6. Effect of hyperglycemia on steroidogenesis. (A) In-vivo gene expression of StAR, steroidogenic genes 3β-HSD and 17β-HSD in diabetic rat testis was assessed using Real time PCR. (B) Effect of hyperglycemia on in vitro gene expression of StAR, steroidogenic genes 3β-HSD and 17β-HSD on LC540 rat Leydig cells treated with different concentrations of glucose using Real time PCR. (C) Effect of hyperglycemia on in vivo protein expression of AQP9; Nrf-2, StAR and steroidogenic genes (3β-HSD and 17β-HSD) in diabetic rat testis. (D) Effect of hyperglycemia on in-vitro protein expression of AQP9; Nrf2, StAR and steroidogenic genes (3β-HSD and 17β-HSD) in LC540 rat Leydig cells treated with different concentrations of glucose. All the results were normalized with β-actin (In vivo n = 6 & In vitro n = 3). Values represent mean ± SD (*p < 0.05, **p < 0.01, ***p < 0.001 and ****p < 0.0001).
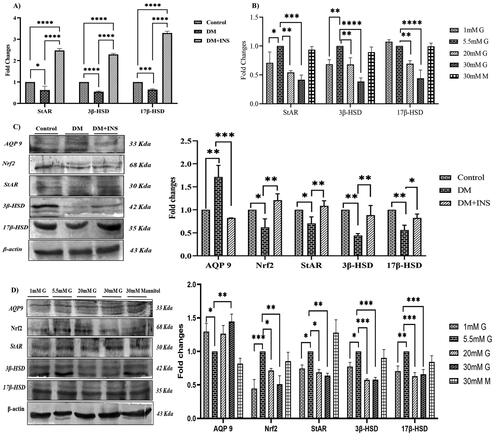
AQP 9 protein expression was significantly increased in-vivo in response to hyperglycemia compared to controls, however insulin treatment was significantly decreased compared to the DM group as shown in the . Moreover, in-vitro results also confirmed the same with significant increase in AQP 9 protein expression on high glucose treatment as shown in compared with 5.5 mM glucose.
Nuclear factor erythroid 2-related factor 2 (Nrf2) is a transcription factor regulating antioxidant response elements, decrease oxidative stress response associated with hyperglycemia and increases insulin sensitivity (Rahimi et al. Citation2021). Our in vivo results show a significant decrease of Nrf2 protein expression in the DM group compared to controls but on insulin treatment the expression was found to increase significantly with p ˂ 0.01 and p ˂ 0.001, respectively, as shown in the . In vitro Nrf2 protein expression was also analyzed with different concentrations of glucose and mannitol as an osmotic control. The expression was significantly decreased at 30 mM glucose concentration and mannitol did not show any significant changes compared to 5.5 mM glucose concentration with p-value (p ˂ 0.01), as shown in . Our results indicate significant changes in enzymatic activity and gene expression of 3β-HSD and 17β-HSD, the key players in testosterone synthesis. We did investigate the protein expression of the key enzymes in testosterone secretion and the steroidogenic genes (3β-HSD and 17β-HSD) were found to be decreased significantly in the DM group along with steroid transport protein StAR compared to control. On treatment with insulin, StAR, 3β-HSD and 17β-HSD were significantly increased compared to DM group rats as shown in the with p values (p ˂ 0.01). In vitro experiments with different concentrations of glucose treatment on LC540 Leydig cells significantly decreased the protein expression of StAR, 3β-HSD and 17β-HSD compared to 5.5 mM normal glucose but on treatment with mannitol did not have any significant changes compared to normal 5.5 mM glucose as shown in the .
Effect of AQP 9 knockdown on testicular steroidogenesis under hyperglycemia
We investigated the effect of AQP 9 knockdown on steroidogenic protein expression. The transfection of siRNA caused an almost 60% reduction in AQP 9 mRNA and protein levels in LC540 cells compared to their respective controls as shown (). To confirm the role of AQP 9 on steroidogenesis, AQP 9 and NC siRNA transfected LC540 rat Leydig cells were treated with high glucose (30 mM) and the expression of Nrf2, StAR and steroidogenic proteins were determined by western blotting. Our results demonstrated that AQP 9 knockdown significantly upregulated the protein levels of Nrf2, StAR, 3β-HSD and 17β-HSD in high glucose treated Leydig cells (). Thus, our results confirm that AQP 9 knockdown restores the expression of Nrf2, StAR and steroidogenic proteins (3β-HSD and 17β-HSD) in high glucose treated Leydig cells.
Figure 7. Effect of AQP 9 knockdown on Leydig cell steroidogenesis. Figures (A) and (B) are representative image to show AQP 9 knockdown in LC540 cells. (C) Effect of AQP 9 knockdown under hyperglycemia on protein expression of AQP 9, Nrf2, StAR and steroidogenic genes (3β-HSD and 17β-HSD) normalized with β-actin as internal control. Values represent mean ± SD (n = 3) (*p < 0.05, **p < 0.01, ***p < 0.001 and ****p < 0.0001).
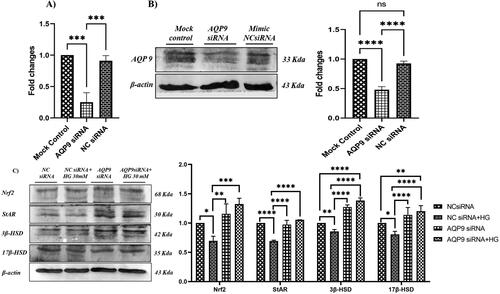
Discussion
Chronic hyperglycemia arising from glucose dysmetabolism owing to insulin resistance and pancreatic dysfunction has been linked to the complications associated with diabetes (Wolpin et al. Citation2013). Moreover, oxidative stress, hormonal modulation, impaired cellular pathways and altered testicular glucose metabolism have been implicated in the pathophysiology of testicular dysfunction in diabetes (Alves et al. Citation2013; Rato et al. Citation2013; Maresch et al. Citation2017). In the present study, body weight of diabetic rats was reduced along with a significant increase in serum glucose levels indicating severe hyperglycemia on STZ exposure. Further, treatment with insulin significantly increased body weight and decreased serum glucose levels indicating this effect is only due to hyperglycemia and our results were consistent with previous studies (Wang-Fischer and Garyantes Citation2018; Faisal Lutfi et al. Citation2021; Guven et al. Citation2021). A causative link among hyperglycemia, mitochondrial ROS generation, oxidative stress and the development of complications has been suggested to play a key role in the pathogenesis of diabetes (Kassab and Piwowar Citation2012). Moreover, the primary cause of hyperglycemia is due to excessive formation of ROS and simultaneously deteriorating antioxidant defense mechanism which leads to development of insulin resistance, increased lipid peroxidation, and cellular damage (Rehman and Akash Citation2017).
On the other hand, hyperglycemia induces testicular damage, impairs spermatogenesis, decreases sperm count by inducing large quantities of ROS including in the sperms, epididymis, and testes, leading to apoptosis induction. Earlier studies have shown that, hyperglycemia associated oxidative stress affecting SOD, CAT, LPO and GSH induced diabetic complications in different organs like liver, heart, kidney, pancreas, brain and testis (Singh et al. Citation2013; Yu and Huang Citation2015). Following severe hyperglycemia in our study, major radical scavenging enzymes SOD and catalase were reduced and increased ROS formation with enhanced lipid peroxidation. These changes lead to antioxidant imbalance; thereby affects steroidogenesis in diabetic rats and our results were consistent with the earlier studies (Singh et al. Citation2013; Yu and Huang Citation2015; Dludla et al. Citation2017; Malekiyan et al. Citation2019; Nna et al. Citation2019; Shoorei et al. Citation2019).
AQPs functional involvement in the transport of ROS is the key factor in dysfunctions underlying oxidative stress related disease physiology and pathophysiology (Yusupov et al. Citation2019). Recent discoveries suggest that, AQPs may play an important role in fat accumulation and regulation of oxidative stress, the two crucial aspects of diabetes and metabolic syndrome (Galli et al. Citation2021). AQP 3 and 5 expressed in cancerous tissues in response to oxidative stress plays an important role in cell survival and cancer progression (Milkovic and Gasparovic Citation2021; Wang et al. Citation2021). Moreover, under hyperglycemia, AQP 5 in eye lens controls ROS accumulation prevents cataractogenesis and also regulates diabetes induced dry mouth, hyposalivation, or xerostomia altering the antioxidant imbalance (Lee et al. Citation2020; Varadaraj and Kumari Citation2020). Increased AQP 8 expression induced by extracellular oxidative stress in retinal pigment epithelial cells is involved in pathology of diabetic retinopathy and age-related macular degeneration (Schnabel et al. Citation2020). In obese patients, AQP 9 prevents excessive lipid accumulation and the subsequent aggravation of hyperglycemia in response to AQP 3 glycerol reflux (Hou et al. Citation2018). Earlier studies have shown that, AQP 9 knockout mice showed decreased plasma glucose levels (Rojek et al. Citation2007; Jelen et al. Citation2011; Spegel et al. Citation2015). In liver, AQP 9 expression was found to be increased in both type 1 and type 2 diabetes but the expression was decreased with the circulating insulin levels (Carbrey et al. Citation2003; Hou et al. Citation2018). Following oxidative stress in our studies, we did analyze the expression changes of AQP isoforms under hyperglycemia. To the best of our knowledge, our study is the first to report differential expression of AQPs 0–12 in diabetes rats (in vivo) and LC540 Leydig cells (in vitro). AQP 9 was found to be significantly upregulated both in vivo and in vitro indicating that hyperglycemia regulates AQP 9 expression. AQPs 1, 8 and 9 expression in testis is found to be cell specific and their expression appear to be regulated by androgens (Badran and Hermo Citation2002). Oxidative stress induced AQP 9 and 12 in retina aids in lactic acidosis, preventing subretinal edema (Hollborn et al. Citation2012). Aqp9 Gene Deletion Enhances Retinal Ganglion Cell (RGC) death and dysfunction induced by optic nerve crush (Carbrey et al. Citation2003; Mori et al. Citation2020). It is clear from the earlier reports that, AQPs are involved in maintaining oxidative stress and involved in the pathophysiology of diabetic complications. Furthermore, elimination of hydrogen peroxide and ROS accumulation affects oxidative stress and in turn regulates AQPs (3, 7, 8 and 11) involved in sperm volume regulation, swelling and tail bending affecting motility (Pellavio and Laforenza Citation2021). Aquaporin water channels expressed in the male reproductive tract plays an important role in lumen fluid secretion of seminiferous tubules occurring during testis development and the fluid movements during spermatogenesis, sperm concentration and maturation.
Nuclear factor erythroid 2-related factor 2 (Nrf2) signaling pathway is the key regulatory process maintaining oxidative stress and prevents diabetic complications of multiple organs regulating antioxidant imbalance (Guo et al. Citation2021; Li et al. Citation2021; Mathur et al. Citation2021; Zhang et al. Citation2021). A recent study has shown that early brain injury after subarachnoid hemorrhage in rats, via activation of Nrf2 and inhibition of AQP4 reduced oxidative stress preventing injury (Zhang et al. Citation2020, Citation2021). Nrf2 plays a critical role in the long-term recovery of permanent cerebral ischemic damage and contribute to neuro protection. The reactive gliosis and regulation of transmembrane water channel AQP 4 confers neuro protection via Nrf2 (Liu et al. Citation2019). Upregulation of Nrf2 in testis suppressed oxidative stress and lowered lipid peroxidation. This in turn is shown to ameliorate antioxidant imbalance preventing the impairment of spermatogenesis and steroidogenesis (Nna et al. Citation2020; Arab et al. Citation2021; Khalil et al. Citation2021; Nasiri et al. Citation2021). We observed a significant decrease in Nrf2 expression in STZ exposed diabetic rats as shown in earlier studies (Zhao et al. Citation2016; Pan et al. Citation2017). Similarly, a significant decrease in Nrf2 expression was observed in high glucose treated LC540 Leydig cells and this could be due to oxidative stress following hyperglycemia. Nrf2 knockout mice exhibited significant diabetes-induced loss in testicular weight and sperm count, as well as increased testicular apoptotic cell death compared to wild-type mice indicating its crucial role in maintaining fertility (Pan et al. Citation2017).
A proven feature of DM is the reduction in male fertility, in terms of semen parameters, sperm structures and testosterone deficiency (Pitteloud et al. Citation2005; Condorelli et al. Citation2018). The steroidogenic enzyme activities 3β-HSD and 17β-HSD were found to be decreased in diabetic rat testis. Steroid acute regulatory protein (StAR) transfers cholesterol from the outer to the inner mitochondrial membrane, which in turn mediates synthesis of key testosterone regulating enzymes 3β-HSD and 17β-HSD. 3β-HSD is a steroidogenic enzyme in rat Leydig cells that catalyses the oxidative conversion of pregnenolone to progesterone and 17β-HSD aids in the conversion of androstenedione to testosterone, the main rate-limiting enzymes. Further, in vivo and in vitro gene and protein expression analysis indicated a significant decrease in key steroidogenic enzymes 3β-HSD and 17β-HSD along with StAR. This in turn, decreased testosterone in diabetic rats in concurrence with earlier studies (Nna et al. Citation2019; Shoorei et al. Citation2019). It has also been reported earlier that silencing AQP 11 increased 3β-HSD gene expression and other spermatogenesis related genes (Shannonhouse et al. Citation2014). However, the involvement of AQPs and Nrf2 in regulation of male fertility cannot be denied. We hypothesize that impairment of testicular steroidogenesis could be due to AQP 9 regulating Nrf2 therefore, we knocked down AQP 9 and interestingly we observed a significant increase in Nrf2, StAR 3β-HSD and 17β-HSD protein expression. Thus, we conclude that hyperglycaemia induced oxidative stress upregulates AQP 9 expression and in turn downregulates Nrf2 leading to impaired testicular steroidogenesis.
Conclusion
Diabetes associated hyperglycemia induced oxidative stress is a widely proven cause for male infertility. Although studies address the role of AQPs in spermatogenesis, only handful of studies are available to show the role of AQPs in steroidogenesis. Our study showed that, hyperglycemia induced oxidative stress and, in turn, upregulated AQP 9 resulted in impaired testicular steroidogenesis. Further, the knock down of AQP 9 significantly upregulated Nrf2 thereby reducing oxidative stress and upregulating the expression of steroidogenic proteins. However, more studies are required to further understand the molecular mechanisms behind AQP 9 mediated regulation of steroidogenesis under hyperglycemia.
Materials and methods
Chemicals
Streptozotocin (STZ) and cell culture reagents were purchased from HiMedia Laboratories Pvt. Ltd., Mumbai, India. All the other chemicals used were of analytical grade obtained from Sigma Chemicals Company, Saint Louis, MO, USA, HiMedia Laboratories Pvt. Ltd., and Sisco Research Laboratories Pvt. Ltd., Mumbai, India.
Experimental animals
Adult male albino rats of Wistar strain weighing 200 ± 25 g (10–12 weeks old) were used in this study. The animals were maintained under standard conditions of humidity, temperature (25 ± 2 °C) and light (12 h light/dark). The animals were housed in large spacious cages bedded with husk and fed with a standard pellet diet with free access to water. The animals were randomly divided into three groups with six animals each and citrate buffer treated animals served as controls (Group I; control), Group II (DM) animals received intraperitoneal (i.p) injection of 60 mg/kg body weight Streptozotocin (STZ) dissolved in 0.1 M Citrate buffer. Group III (DM + INS) animals received intraperitoneal (i.p) injection with 60 mg/kg body weight STZ dissolved in 0.1 M Citrate buffer along with 2 U/kg b.w of Insulin subcutaneously (Lantus; Sanofi-Aventis, France) after 48 h on alternate days for 4 weeks. The serum glucose levels of control, DM and DM + INS were measured before the start of experiment (0th day) and after administration of STZ at 1st, 2nd, 3rd and 4th weeks by tail vein bleed, using ONE TOUCH Select glucometer (Life Scan, Europe). The serum glucose levels higher than 200 mg/dl were considered diabetic (Qinna and Badwan Citation2015). The animals were sacrificed with overdose of ketamine intramuscularly after the study period of 4 weeks and the organs isolated were stored at −80 0C until the experiment. The serum testosterone levels were estimated by using enzyme immunoassay test kit (Alpco Diagnostics, USA) as per manufacturer protocol.
Cell culture and transfection
The LC-540 rat Leydig cells were purchased from National Centre for Cell Science (NCCS, Pune). Rat testis Leydig cells (LC-540) were cultured in a humidified atmosphere containing 5% CO2 in a complete medium composed of MEM Eagle (Earle’s BSS) supplemented with 2 mM L-glutamine, 0.1 mM non-essential amino acids (NEAA), 1 mM sodium pyruvate, 100 U/mL penicillin, 100 mg/mL streptomycin, and 10% fetal bovine serum (Sadasivam et al. Citation2015). To determine the effect of experimental hyperglycaemia on AQPs expression in LC540 rat Leydig cells, the growth medium was replaced by serum free medium and cells were exposed to different concentrations of glucose (1 mM, 5.5 mM, 20 mM and 30 mM) of which 5.5 mM served as control and 30 mM mannitol as an osmotic control for 24 h. For the AQP 9 knockdown experiment, LC540 cells were transfected with AQP 9 siRNA (Eurogentec, Belgium) or Silencer negative control (Sigma Aldrich, USA). siRNAs were obtained as annealed oligos and transfected at a final concentration of 60 nM. The transfection of siRNA was performed using lipofectamine RNAiMAX reagent according to the manufacturer’s protocol (Invitrogen, Thermo Fisher Scientific, Inc.) (Hattori et al. Citation2017).
Preparation of tissue homogenate and cell extract
Briefly, cell extracts from LC540 cells and the tissue homogenate from testes were prepared in lysis buffer (10 mM Tris, pH 8.0, 140 mM NaCl, 5 mM MgCl2, 0.2 mM EDTA, 0.5% NonidetP-40, 20% glycerol, 1 mM phenylmethylsulfonylfluoride, protease and phosphatases inhibitors). The cell extract/tissue homogenate was centrifuged and the supernatant was used for further analyses.
Estimation of protein
The total protein from the tissue extracts was estimated accorrding to Lowry et al. (Lowry et al. Citation1951). Briefly, 0.1 mL of the diluted tissue homogenate was made up to 1 mL with water. To this, 4.5 mL of alkaline copper reagent (2% Na2CO3 in 0.1 N NaOH was mixed with 0.5% CuSO4 containing 1% sodium potassium tartrate in the ratio of 50:1) was added, mixed and allowed to stand at room temperature for 20 min. Later, 0.5 mL of Folin’s Ciocalteau reagent was added and shaken well. The blue colour complex formed was measured at 640 nm after 15 min against the blank with biospectrometer basic (Eppendorf, Germany).
Assay of enzymatic antioxidant - superoxide dismutase (SOD)
The enzyme was assayed according to the method of Marklund and Marklund (Marklund and Marklund Citation1974). The degree of inhibition of auto-oxidation of pyrogallol, in an alkaline pH by SOD was used as a measure of the enzyme activity. The enzyme activity is defined as units/mg protein, where one unit is equal to the amount of enzyme required to inhibit auto oxidation of pyrogallol by 50%. The assay was performed and the absorbance was read at 470 nm at different time intervals in an ELISA iMARKTM microplate reader (Bio-Rad, USA).
Assay of enzymatic Antioxidant - Catalase (CAT)
The activity of catalase was assayed by the method of Sinha (Sinha Citation1972). The assay is based on the fact that chromic acetate is formed reducing dichromate in acetic acid in the presence of H2O2. The chromic acetate thus formed was measured at 610 nm in an ELISA iMARKTM microplate reader (Bio-Rad, USA). The activity of catalase was expressed as µmoles of H2O2 consumed/min/mg protein.
Assay of lipid peroxidation (LPO)
Thiobarbituric acid (TBA) was used to estimate LPO following the procedure of Hogberg et al. (Hogberg et al. Citation1974). The intensity of oxidative stress is proportional to the malondialdehyde (MDA) formed, an end product of peroxidation of lipids. MDA reacts with TBA to generate a coloured product that absorbs at 532 nm was read in an ELISA iMARKTM microplate reader (Bio-Rad, USA). The ferrous sulphate and ascorbate induced LPO system contained 10 mM ferrous sulphate and 0.2 mM ascorbate as inducers (Devasagayam and Tarachand Citation1987). The lipid peroxides were expressed as nmoles of MDA formed/mg protein.
Estimation of 3β -hydroxysteroid dehydrogenase (3β-HSD)
The activity of testicular 3β-HSD was measured by the method of Shivanandappa and Venkatesh (Shivanandappa and Venkatesh Citation1997). The assay buffer containing 0.1 M Tris HCl (pH 7.8), 500 µM NAD+, 0.08% iodonitrotetrazolium chloride in 1% Tween 20 and 0.1 mM pregnenolone was made up to a final volume of 3 mL. To which 50 µL of the enzyme was added and incubated at 37 °C for 60 min. And then, 2 mL of phthalate buffer was added to stop the reaction. The mixture was centrifuged at 3000 rpm for 20 min and the supernatant was read at 490 nm with Biospectrometer basic (Eppendorf, Germany). The activity of 3β-HSD was calculated from the standard curve of NADH and expressed as nmoles of NADH formed/min/mg protein.
Estimation of 17β-hydroxy steroid dehydrogenase (17β-HSD)
The activity of testicular 17β-HSD was determined by the method described previously (HU Citation1974). Briefly, the reaction mixture was prepared with 100 µL of LC540 cell lysate, 200 µL of 0.5 µM NADPH, to a final volume of 3 mL 100 µM phosphate buffer (pH 7.4). The reaction was initiated by the addition of 100 µL of 0.8 µM androsten-3,17-dione and the decrease in absorbance of NADPH was followed at 340 nm at 20 sec intervals for 5 min with Biospectrometer basic (Eppendorf, Germany). The enzymatic activity of 17β-HSD was given as nmoles of NADPH oxidized/min/mg protein.
Extraction of RNA and analysis of the gene expression by quantitative real time polymerase chain reaction (qRT-PCR)
Briefly, the total RNA from testes and LC540 cells were isolated according to instructions from the RNA isolation kit (One step RNA TRIzol Reagent; Biobasic Inc., Markham Ontario, Canada). First-strand cDNA synthesis was performed using the iScript cDNA synthesis kit Bio-Rad Laboratories, Inc., USA) following the manufacturer’s protocol. The real-time amplification of the cDNA was achieved using SSO Advanced Universal SYBR Green Supermix according to the manufacturer’s protocol (Bio-Rad Laboratories, Inc., USA). The Insta Q96 Himedia Real-Time PCR (RT-PCR) (HiMedia Laboratories Pvt. Ltd., Mumbai, India) was used to analyse the gene expression with specific sets of primers used for target of primers genes are given in . Fold differences in target genes expression were calculated using the formula 2ΔΔCt. The expression of target genes were normalized with respective internal controls.
Table 1. Primer sequences for target gene – real time PCR.
Western blot analysis
Proteins from cell extract/tissue homogenate were separated by SDS-PAGE and subsequently electroblotted onto nitrocellulose membranes. Membranes were blocked for 1 h at room temperature in 1× TTBS (20 mM Tris, pH 7.4, 150 mM NaCl, 0.1% Triton X-100) supplemented with 5% non-fat dry milk. After blocking, membranes were incubated overnight at 4 °C, with specific primary antibodies listed in . Membranes were washed four times for 10 min in TTBS and subsequently incubated for 3 h at room temperature with a secondary alkaline phosphatase-conjugated antibody and the proteins were visualized using 5-Bromo-4-chloro-3-indolyl phosphate (BCIP/NBT) chromogen substrate (Sigma Chemicals Company, Saint Louis, MO Inc., USA). Lab image platform ver 2.1 software by Kapelan Bio-Imaging GmbH was used for densitometric analysis. The expression of each target protein were normalized with corresponding internal control.
Table 2. Primary and secondary antibodies used for western blotting (source and dilutions).
Data analysis
The values are expressed as mean ± standard deviation (SD). Graph pad Prism 9.0 software was used to determine the differences between the groups assessed by one-way ANOVA. Inter-group comparisons were performed using Tukey’s multiple comparisons test. Values were considered significant at p ˂ 0.05.
Ethics approval for animal study
Experimental animals were handled according to university and institution legislation, regulated by the committee for the purpose of Control and Supervision of Experiments on Animals (CPCSEA), Ministry of Social justice and Empowerment, Government of India (BDU/IAEC/P04/2018).
Authors’ contributions
The study concept, experimental design and execution, data acquisition, analysis, interpretation and manuscript preparation: AK; Supervision, data interpretation and manuscript revision: CP; Critical comments and suggestions: CP, KA, LFMA, AP, CL and DKJ. All authors discussed the content and contributed to the final manuscript.
Abbreviations | ||
AQPs | = | Aquaporins |
RT-PCR | = | Real Time Polymerase Chain Reaction |
STZ | = | Streptozotocin |
Nrf2 | = | Nuclear factor erythroid 2-related factor 2 |
DM | = | Diabetes Mellitus |
SOD | = | Superoxide dismutase |
CAT | = | Catalase |
LPO | = | Lipid Peroxidation |
3β-HSD | = | 3β-hydroxysteroid dehydrogenase |
17β-HSD | = | 17β-hydroxysteroid dehydrogenase |
StAR | = | Steroidogenic Acute Regulatory Protein |
Disclosure statement
No potential conflict of interest was reported by the authors.
Additional information
Funding
References
- Ahmad J. 2016. The diabetic foot. Diabetes Metab Syndr. 10(1):48–60.
- Ala M, Mohammad Jafari R, Hajiabbasi A, Dehpour AR. 2021. Aquaporins and diseases pathogenesis: from trivial to undeniable involvements, a disease-based point of view. J Cell Physiol. 236(9):6115–6135.
- Alves MG, Martins AD, Rato L, Moreira PI, Socorro S, Oliveira PF. 2013. Molecular mechanisms beyond glucose transport in diabetes-related male infertility. Biochim Biophys Acta. 1832(5):626–635.
- American Diabetes Association. 2013. Diagnosis and classification of diabetes mellitus. Diabetes Care. 36 (Suppl 1):S67–S74.
- Andes LJ, Cheng YJ, Rolka DB, Gregg EW, Imperatore G. 2020. Prevalence of prediabetes among adolescents and young adults in the United States, 2005–2016. JAMA Pediatr. 174(2):e194498.
- Arab HH, Gad AM, Reda E, Yahia R, Eid AH. 2021. Activation of autophagy by sitagliptin attenuates cadmium-induced testicular impairment in rats: targeting AMPK/mTOR and Nrf2/HO-1 pathways. Life Sci. 269:119031.
- Azad AK, Raihan T, Ahmed J, Hakim A, Emon TH, Chowdhury PA. 2021. Human aquaporins: functional diversity and potential roles in infectious and non-infectious. Front Genet. 12(654865):654865.
- Badran HH, Hermo LS. 2002. Expression and regulation of aquaporins 1, 8, and 9 in the testis, efferent ducts, and epididymis of adult rats and during postnatal development. J Androl. 23(3):358–373.
- Barkabi-Zanjani S, Ghorbanzadeh V, Aslani M, Ghalibafsabbaghi A, Chodari L. 2020. Diabetes mellitus and the impairment of male reproductive function: possible signaling pathways. Diabetes Metab Syndr. 14(5):1307–1314.
- Calamita G, Mazzone A, Bizzoca A, Svelto M. 2001. Possible involvement of aquaporin-7 and -8 in rat testis development and spermatogenesis. Biochem Biophys Res Commun. 288(3):619–625.
- Carbrey JM, Gorelick-Feldman DA, Kozono D, Praetorius J, Nielsen S, Agre P. 2003. Aquaglyceroporin AQP9: solute permeation and metabolic control of expression in liver. Proc Natl Acad Sci USA. 100(5):2945–2950.
- Chauvigne F, Boj M, Finn RN, Cerda J. 2015. Mitochondrial aquaporin-8-mediated hydrogen peroxide transport is essential for teleost spermatozoon motility. Sci Rep. 5:7789.
- Clarke-Bland CE, Bill RM, Devitt A. 2022. Emerging roles for AQP in mammalian extracellular vesicles. Biochim Biophys Acta Biomembr. 1864(3):183826.
- Condorelli RA, La Vignera S, Mongioi LM, Alamo A, Calogero AE. 2018. Diabetes mellitus and infertility: different pathophysiological effects in type 1 and type 2 on sperm function. Front Endocrinol (Lausanne). 9:268.
- da Silva IV, Soveral G. 2017. Aquaporins in obesity. Adv Exp Med Biol. 969:227–238.
- Dajani S, Saripalli A, Sharma-Walia N. 2018. Water transport proteins-aquaporins (AQPs) in cancer biology. Oncotarget. 9(91):36392–36405.
- Devasagayam TP, Tarachand U. 1987. Decreased lipid peroxidation in the rat kidney during gestation. Biochem Biophys Res Commun. 145(1):134–138.
- Dludla PV, Joubert E, Muller CJF, Louw J, Johnson R. 2017. Hyperglycemia-induced oxidative stress and heart disease-cardioprotective effects of rooibos flavonoids and phenylpyruvic acid-2-O-β-D-glucoside. Nutr Metab (Lond). 14:45.
- Faisal Lutfi M, Abdel-Moneim AH, Alsharidah AS, Mobark MA, Abdellatif AAH, Saleem IY, Al Rugaie O, Mohany KM, Alsharidah M. 2021. Thymoquinone lowers blood glucose and reduces oxidative stress in a rat model of diabetes. Molecules. 26(8):2348.
- Galli M, Hameed A, Żbikowski A, Zabielski P. 2021. Aquaporins in insulin resistance and diabetes: more than channels! Redox Biol. 44:102027.
- Guo Y, Zhang C, Wang C, Huang Y, Liu J, Chu H, Ren X, Kong L, Ma H. 2021. Thioredoxin-1 is a target to attenuate alzheimer-like pathology in diabetic encephalopathy by alleviating endoplasmic reticulum stress and oxidative stress. Front Physiol. 12:651105.
- Guven H, Durmus N, Hocaoglu N, Guner O, Acar S, Akan P, Calan OG. 2021. Protective effects of wheat germ oil against erectile and endothelial dysfunction in streptozotocin-induced diabetic rats. Int J Impot Res.
- Hattori Y, Kikuchi T, Ozaki KI, Onishi H. 2017. Evaluation of in vitro and in vivo therapeutic antitumor efficacy of transduction of polo-like kinase 1 and heat shock transcription factor 1 small interfering RNA. Exp Ther Med. 14(5):4300–4306.
- Hogberg J, Larson RE, Kristoferson A, Orrenius S. 1974. NADPH-dependent reductase solubilized from microsomes by peroxidation and its activity. Biochem Biophys Res Commun. 56(3):836–842.
- Hollborn M, Rehak M, Iandiev I, Pannicke T, Ulbricht E, Reichenbach A, Wiedemann P, Bringmann A, Kohen L. 2012. Transcriptional regulation of aquaporins in the ischemic rat retina: upregulation of aquaporin-9. Curr Eye Res. 37(6):524–531.
- Hou J, Li Y, Ren X, Gao F, Chen Y, Yi Y, Kang L, Luo Y, Yang M. 2018. Similar aquaporin9 and mapk expression profiles in the liver of types 1 and 2 diabetes mellitus. Horm Metab Res. 50(5):414–421.
- Hu B. 1974. Steroid dehydrogenase 3rd ed. New York: Academic Press. (Methods of Enzymatic Analysis).
- Huang HF, He RH, Sun CC, Zhang Y, Meng QX, Ma YY. 2006. Function of aquaporins in female and male reproductive systems. Hum Reprod Update. 12(6):785–795.
- Ichiyama T, Nakatani E, Tatsumi K, Hideshima K, Urano T, Nariai Y, Sekine J. 2018. Expression of aquaporin 3 and 5 as a potential marker for distinguishing dry mouth from Sjögren’s syndrome. J Oral Sci. 60(2):212–220.
- Jelen S, Wacker S, Aponte-Santamaría C, Skott M, Rojek A, Johanson U, Kjellbom P, Nielsen S, de Groot BL, Rützler M. 2011. Aquaporin-9 protein is the primary route of hepatocyte glycerol uptake for glycerol gluconeogenesis in mice. J Biol Chem. 286(52):44319–44325.
- Kassab A, Piwowar A. 2012. Cell oxidant stress delivery and cell dysfunction onset in type 2 diabetes. Biochimie. 94(9):1837–1848.
- Khalil ASM, Giribabu N, Yelumalai S, Shahzad H, Kilari EK, Salleh N. 2021. Myristic acid defends against testicular oxidative stress, inflammation, apoptosis: restoration of spermatogenesis, steroidogenesis in diabetic rats. Life Sci. 278:119605.
- Khalil H. 2017. Diabetes microvascular complications-A clinical update. Diabetes Metab Syndr. 11 Suppl 1(Suppl 1):S133–S139.
- Lee HY, Gu M, Cheng J, Suh JW, Chae HJ. 2020. Ixeris dentata and Lactobacillus gasseri extracts improve salivary secretion capability in diabetes-associated dry mouth rat model. Nutrients. 12(5):1331.
- Lei X, Huo P, Wang Y, Xie Y, Shi Q, Tu H, Yao J, Mo Z, Zhang S. 2020. Lycium barbarum polysaccharides improve testicular spermatogenic function in streptozotocin-induced diabetic rats. Front Endocrinol (Lausanne). 11:164.
- Li Y, Zhou Y, Wu J, Li J, Yao H. 2021. Phelligridin D from Inonotus obliquus attenuates oxidative stress and accumulation of ECM in mesangial cells under high glucose via activating Nrf2. J Nat Med. 75(4):1021–1029.
- Liu L, Kelly MG, Wierzbicki EL, Escober-Nario IC, Vollmer MK, Dore S. 2019. Nrf2 plays an essential role in long-term brain damage and neuroprotection of Korean Red Ginseng in a permanent cerebral ischemia model. Antioxidants (Basel). 8(8):273.
- Lowry OH, Rosebrough NJ, Farr AL, Randall RJ. 1951. Protein measurement with the Folin phenol reagent. J Biol Chem. 193(1):265–275.
- Malekiyan R, Abdanipour A, Sohrabi D, Jafari Anarkooli I. 2019. Antioxidant and neuroprotective effects of lycopene and insulin in the hippocampus of streptozotocin-induced diabetic rats. Biomed Rep. 10(1):47–54.
- Maresch CC, Stute DC, Alves MG, Oliveira PF, de Kretser DM, Linn T. 2018. Diabetes-induced hyperglycemia impairs male reproductive function: a systematic review. Hum Reprod Update. 24(1):86–105.
- Maresch CC, Stute DC, Ludlow H, Hammes HP, de Kretser DM, Hedger MP, Linn T. 2017. Hyperglycemia is associated with reduced testicular function and activin dysregulation in the Ins2Akita+/− mouse model of type 1 diabetes. Mol Cell Endocrinol. 446:91–101.
- Marklund S, Marklund G. 1974. Involvement of the superoxide anion radical in the autoxidation of pyrogallol and a convenient assay for superoxide dismutase. Eur J Biochem. 47(3):469–474.
- Mathur A, Pandey VK, Khan MF, Kakkar P. 2021. PHLPP1/Nrf2-Mdm2 axis induces renal apoptosis via influencing nucleo-cytoplasmic shuttling of FoxO1 during diabetic nephropathy. Mol Cell Biochem. 476(10):3681–3699.
- Meli R, Pirozzi C, Pelagalli A. 2018. New perspectives on the potential role of aquaporins (AQPs) in the physiology of inflammation. Front Physiol. 9:101.
- Milkovic LC, Gasparovic A. 2021. AQP3 and AQP5-potential regulators of redox status in breast cancer. Molecules. 26(9):2613.
- Mirabella N, Pelagalli A, Liguori G, Rashedul MA, Squillacioti C. 2021. Differential abundances of AQP3 and AQP5 in reproductive tissues from dogs with and without cryptorchidism. Anim Reprod Sci. 228:106735.
- Mori S, Kurimoto T, Miki A, Maeda H, Kusuhara S, Nakamura M. 2020. Aqp9 gene deletion enhances retinal ganglion cell (RGC) death and dysfunction induced by optic nerve crush: evidence that aquaporin 9 acts as an astrocyte-to-neuron lactate shuttle in concert with monocarboxylate transporters to support RGC function and survival. Mol Neurobiol. 57(11):4530–4548.
- Nagarathna R, Bali P, Anand A, Srivastava V, Patil S, Sharma G, Manasa K, Pannu V, Singh A, Nagendra HR. 2020. Prevalence of diabetes and its determinants in the young adults Indian population-call for yoga intervention. Front Endocrinol (Lausanne). 11:507064.
- Nasiri K, Akbari A, Nimrouzi M, Ruyvaran M, Mohamadian A. 2021. Safflower seed oil improves steroidogenesis and spermatogenesis in rats with type II diabetes mellitus by modulating the genes expression involved in steroidogenesis, inflammation and oxidative stress. J Ethnopharmacol. 275:114139.
- Newsholme P, Keane KN, Carlessi R, Cruzat V. 2019. Oxidative stress pathways in pancreatic β-cells and insulin-sensitive cells and tissues: importance to cell metabolism, function, and dysfunction. Am J Physiol Cell Physiol. 317(3):C420–C433.
- Nna VU, Bakar ABA, Ahmad A, Mohamed M. 2019. Down-regulation of steroidogenesis-related genes and its accompanying fertility decline in streptozotocin-induced diabetic male rats: ameliorative effect of metformin. Andrology. 7(1):110–123.
- Nna VU, Ujah GA, Suleiman JB, Mohamed M, Nwokocha C, Akpan TJ, Ekuma HC, Fubara VV, Kekung-Asu CB, Osim EE. 2020. Tert-butylhydroquinone preserve testicular steroidogenesis and spermatogenesis in cisplatin-intoxicated rats by targeting oxidative stress, inflammation and apoptosis. Toxicology. 441:152528.
- Oliveira CA, Carnes K, Franca LR, Hermo L, Hess RA. 2005. Aquaporin-1 and -9 are differentially regulated by oestrogen in the efferent ductule epithelium and initial segment of the epididymis. Biol Cell. 97(6):385–395.
- Pan C, Zhou S, Wu J, Liu L, Song Y, Li T, Ha L, Liu X, Wang F, Tian J, et al. 2017. NRF2 plays a critical role in both self and EGCG protection against diabetic testicular damage. Oxid Med Cell Longev. 2017:3172692.
- Papatheodorou K, Papanas N, Banach M, Papazoglou D, Edmonds M. 2016. Complications of Diabetes 2016. J Diabetes Res. 2016:6989453.
- Pellavio G, Laforenza U. 2021. Human sperm functioning is related to the aquaporin-mediated water and hydrogen peroxide transport regulation. Biochimie. 188:45–51.
- Pitteloud N, Hardin M, Dwyer AA, Valassi E, Yialamas M, Elahi D, Hayes FJ. 2005. Increasing insulin resistance is associated with a decrease in Leydig cell testosterone secretion in men. J Clin Endocrinol Metab. 90(5):2636–2641.
- Qinna NA, Badwan AA. 2015. Impact of streptozotocin on altering normal glucose homeostasis during insulin testing in diabetic rats compared to normoglycemic rats. Drug Des Devel Ther. 9:2515–2525.
- Rahimi G, Heydari S, Rahimi B, Abedpoor N, Niktab I, Safaeinejad Z, Peymani M, Seyed Forootan F, Derakhshan Z, Esfahani MHN, et al. 2021. A combination of herbal compound (SPTC) along with exercise or metformin more efficiently alleviated diabetic complications through down-regulation of stress oxidative pathway upon activating Nrf2-Keap1 axis in AGE rich diet-induced type 2 diabetic mice. Nutr Metab (Lond). 18(1):14.
- Rato L, Alves MG, Dias TR, Lopes G, Cavaco JE, Socorro S, Oliveira PF. 2013. High-energy diets may induce a pre-diabetic state altering testicular glycolytic metabolic profile and male reproductive parameters. Andrology. 1(3):495–504.
- Rehman K, Akash MSH. 2017. Mechanism of generation of oxidative stress and pathophysiology of type 2 diabetes mellitus: how are they interlinked? J Cell Biochem. 118(11):3577–3585.
- Ribeiro JC, Alves MG, Yeste M, Cho YS, Calamita G, Oliveira PF. 2021. Aquaporins and (in)fertility: more than just water transport. Biochim Biophys Acta Mol Basis Dis. 1867(3):166039.
- Rojek AM, Skowronski MT, Fuchtbauer EM, Fuchtbauer AC, Fenton RA, Agre P, Frokiaer J, Nielsen S. 2007. Defective glycerol metabolism in aquaporin 9 (AQP9) knockout mice. Proc Natl Acad Sci USA. 104(9):3609–3614.
- Sadasivam M, Ramatchandirin B, Balakrishnan S, Prahalathan C. 2015. TNF-α-mediated suppression of Leydig cell steroidogenesis involves DAX-1. Inflamm Res. 64(7):549–556.
- Saeedi P, Petersohn I, Salpea P, Malanda B, Karuranga S, Unwin N, Colagiuri S, Guariguata L, Motala AA, Ogurtsova K, et al. 2019. Global and regional diabetes prevalence estimates for 2019 and projections for 2030 and 2045: results from the International Diabetes Federation Diabetes Atlas, 9th edition. Diabetes Res Clin Pract. 157:107843.
- Schnabel B, Kuhrt H, Wiedemann P, Bringmann A, Hollborn M. 2020. Osmotic regulation of aquaporin-8 expression in retinal pigment epithelial cells in vitro: dependence on KATP channel activation. Mol Vis. 26:797–817.
- Shannonhouse JL, Urbanski HF, Woo SL, Fong LA, Goddard SD, Lucas WF, Jones ER, Wu C, Morgan C. 2014. Aquaporin-11 control of testicular fertility markers in Syrian hamsters. Mol Cell Endocrinol. 391(1–2):1–9.
- Shivanandappa T, Venkatesh S. 1997. A colorimetric assay method for 3beta-hydroxy-delta5-steroid dehydrogenase. Anal Biochem. 254(1):57–61.
- Shoorei H, Khaki A, Khaki AA, Hemmati AA, Moghimian M, Shokoohi M. 2019. The ameliorative effect of carvacrol on oxidative stress and germ cell apoptosis in testicular tissue of adult diabetic rats. Biomed Pharmacother. 111:568–578.
- Sies H. 2014. Role of metabolic H2O2 generation: redox signaling and oxidative stress. J Biol Chem. 289(13):8735–8741.
- Singh R, Bhardwaj P, Sharma P. 2013. Antioxidant and toxicological evaluation of Cassia sopherain streptozotocin-induced diabetic Wistar rats. Pharmacognosy Res. 5(4):225–232.
- Sinha AK. 1972. Colorimetric assay of catalase. Anal Biochem. 47(2):389–394.
- Song J, Gao X, Tang Z, Li H, Ruan Y, Liu Z, Wang T, Wang S, Liu J, Jiang H. 2020. Protective effect of Berberine on reproductive function and spermatogenesis in diabetic rats via inhibition of ROS/JAK2/NFκB pathway. Andrology. 8(3):793–806.
- Spegel P, Chawade A, Nielsen S, Kjellbom P, Rutzler M. 2015. Deletion of glycerol channel aquaporin-9 (Aqp9) impairs long-term blood glucose control in C57BL/6 leptin receptor-deficient (db/db) obese mice. Physiol Rep. 3(9):e12538.
- Squillacioti C, Mirabella N, Liguori G, Germano G, Pelagalli A. 2021. Aquaporins are differentially regulated in canine cryptorchid efferent ductules and epididymis. Animals (Basel). 11(6):1539.
- Su W, Cao R, Zhang XY, Guan Y. 2020. Aquaporins in the kidney: physiology and pathophysiology. Am J Physiol Renal Physiol. 318(1):F193–F203.
- Varadaraj K, Kumari SS. 2020. Lens aquaporins function as peroxiporins to facilitate membrane transport of hydrogen peroxide. Biochem Biophys Res Commun. 524(4):1025–1029.
- Wang J, Feng L, Zhu Z, Zheng M, Wang D, Chen Z, Sun H. 2015. Aquaporins as diagnostic and therapeutic targets in cancer: how far we are? J Transl Med. 13:96.
- Wang Y, Chen D, Liu Y, Zhang Y, Duan C, Otkur W, Chen H, Liu X, Xia T, Qi H, et al. 2021. AQP3-mediated H2 O2 uptake inhibits LUAD autophagy by inactivating PTEN. Cancer Sci. 112(8):3278–3292.
- Wang-Fischer Y, Garyantes T. 2018. Improving the reliability and utility of streptozotocin-induced rat diabetic model. J Diabetes Res. 2018:8054073.
- Wolpin BM, Bao Y, Qian ZR, Wu C, Kraft P, Ogino S, Stampfer MJ, Sato K, Ma J, Buring JE, et al. 2013. Hyperglycemia, insulin resistance, impaired pancreatic beta-cell function, and risk of pancreatic cancer. J Natl Cancer Inst. 105(14):1027–1035.
- Yu B, Huang Z. 2015. Variations in antioxidant genes and male infertility. Biomed Res Int. 2015:513196.
- Yusupov M, Razzokov J, Cordeiro RM, Bogaerts A. 2019. Transport of reactive oxygen and nitrogen species across aquaporin: a molecular level picture. Oxid Med Cell Longev. 2019:2930504.
- Zhang B, Zhang CY, Zhang XL, Sun GB, Sun XB. 2021. Guan Xin Dan Shen formulation protects db/db mice against diabetic cardiomyopathy via activation of Nrf2 signaling. Mol Med Rep. 24(1):531.
- Zhang HB, Tu XK, Song SW, Liang RS, Shi SS. 2020. Baicalin reduces early brain injury after subarachnoid hemorrhage in rats. Chin J Integr Med. 26(7):510–518.
- Zhao Y, Kong C, Chen X, Wang Z, Wan Z, Jia L, Liu Q, Wang Y, Li W, Cui J, et al. 2016. Repetitive exposure to low-dose X-irradiation attenuates testicular apoptosis in type 2 diabetic rats, likely via Akt-mediated Nrf2 activation. Mol Cell Endocrinol. 422:203–210.