Abstract
Prostate cancer (PCa) is as a serious threat to male’s health around the world. Recent studies have indicated that long non-coding RNAs (lncRNAs) occupy an important position in various human cancers. However, the function and mechanism of lncRNA DNMBP antisense RNA 1 (DNMBP-AS1) in PCa is rarely investigated. RT-qPCR analysis was used to test gene expression. CCK-8, colony formation, EdU staining and transwell assays were conducted to assess the function of DNMBP-AS1 on PCa cell behaviors. RNA pull down, RIP and luciferase reporter assays were implemented to verify the mechanism of DNMBP-AS1. DNMBP-AS1 was obviously up-regulated in PCa cell lines. Functionally, DNMBP-AS1 knockdown weakened cell proliferation, migration and invasion of PCa. Mechanistically, DNMBP-AS1 sponged microRNA-6766-3p (miR-6766-3p) to regulate lysocardiolipin acyltransferase 1 (LCLAT1) expression. Furthermore, DNMBP-AS1 could stabilize LCLAT1 expression by recruiting ELAV like RNA binding protein 1 (ELAVL1). Consequently, rescue assays demonstrated that DNMBP-AS1 regulated PCa cell proliferation, migration and invasion through enhancing LCLAT1 expression. Collectively, we elucidated the function and regulatory mechanism of DNMBP-AS1 and provided the first evidence of DNMBP-AS1 as a driver for PCa.
Introduction
As one of the most common cancers in men, prostate cancer (PCa) has been identified as a very malignant and frequent clinical cancer all over the world (Siegel et al. Citation2015; Prostate cancer Citation2016). Most patients were diagnosed with PCa at advanced stage and the prognosis of patients under conventional treatment is poor (Qi et al. Citation2019). Since the current treatment options may have side effects (Litwin and Tan Citation2017), it is increasingly urgent to search an effective therapeutic strategy for PCa.
As one large class of nonprotein-coding ranscripts, long noncoding RNAs (lncRNAs) have been verified to participate in various essential cellular processes via diverse molecular mechanism (Charles Richard and Eichhorn Citation2018). LncRNAs are defined as transcripts composed of more than 200 nucleotides, lacking the capacity of coding proteins (Guo et al. Citation2015). The important biological functions of lncRNAs have been extensively studied in diverse cancers (Bolha et al. Citation2017; Zhang and Tang Citation2018). For instance, Wang et al have identified that lncRNA HOXA-AS2 is a therapeutic target in human cancers (Wang et al. Citation2018). Zhu et al have clarified that CASC2 is of great significance in cancers (Zhu et al. Citation2018). Furthermore, lncRNAs have been elucidated to be aberrantly expressed in PCa and may be used as novel biomarkers in PCa (Liu et al. Citation2015; Mitobe et al. Citation2018). For example, Long et al have proposed that lncRNA LOXL1-AS1 promotes cell proliferation and cell cycle progression in PCa and may be used as a biomarker for PCa treatment (Long et al. Citation2018). Wang et al have proved that lincRNA-p21 acts as a tumor suppressor in PCa (Wang et al. Citation2017). Bai et al have elucidated that lncRNA NEAT1 is associated with the prognosis of PCa patients (Bai and Huang Citation2020).
In the present study, we probed into the expression of lncRNA DNMBP antisense RNA 1 (DNMBP-AS1) in PCa and its regulatory functions in PCa progression.
Results
DNMBP-AS1 knockdown suppressed cell proliferation, migration and invasion in PCa
Firstly, the expression profile of DNMBP-AS1 in tumor tissues and normal tissues was obtained from the GEPIA database (http://gepia2.cancer-pku.cn/#index). As shown in , DNMBP-AS1 was significantly up-regulated in PRAD tissues. In addition, used the starBase database (https://starbase.sysu.edu.cn/) to further explore DNMBP-AS1 expression in PRAD tissues and normal tissues. As shown in supplemental Figure S1A, DNMBP-AS1 was significantly overexpressed in PRAD tissues compared with normal tissues. Subsequently, RT-qPCR was performed to detect the expression of DNMBP-AS1 in PCa cells (DU145, PC3, LNCAP and VCaP). In comparison with the human normal prostatic epithelial cell line (RWPE-1), DNMBP-AS1 was significantly up-regulated in PCa cells, particularly in PC3 and VCaP cells (). DNMBP-AS1 in PC3 and VCaP cells was successfully silenced after the transfection of three specific short hairpin RNAs (shRNAs) against DNMBP-AS1 (). The sh-DNMBP-AS1#1 and sh-DNMBP-AS1#3 were chosen for subsequent functional experiments owing to their better interference efficiency. CCK-8, colony formation and EdU staining assays were performed for testing cell proliferative ability. The experimental data presented that DNMBP-AS1 depletion markedly suppressed cell proliferation in PCa (). Moreover, the obvious inhibition of cell migration and invasion by DNMBP-AS1 knockdown was observed in the results of transwell assay (). Overall, all above data suggested that DNMBP-AS1 down-regulation inhibits PCa progression.
Figure 1. DNMBP-AS1 knockdown suppressed cell proliferation, migration and invasion in PCa. (A) GEPIA database predicted DNMBP-AS1 expression in tumor tissues and normal tissues. (B) RT-qPCR result of DNMBP-AS1 expression in PCa cells (DU145, PC3, LNCAP and VCaP) and human normal prostatic epithelial cell line (RWPE-1). (C) The efficiency of silencing DNMBP-AS1 in PC3 and VCaP cells. (D) CCK-8 assay was utilized to detect cell viability after DNMBP-AS1 knockdown. (E) Cell proliferation was detected by colony formation assay when DNMBP-AS1 was knocked down in cells. (F) EdU-positive cells were detected in PC3 and VCaP cells transfected with sh-DNMBP-AS1#1 or sh-DNMBP-AS1#3. (G) The impact of DNMBP-AS1 depletion on cell migration and invasion were assessed by transwell assay. *p < 0.05, **p < 0.01.
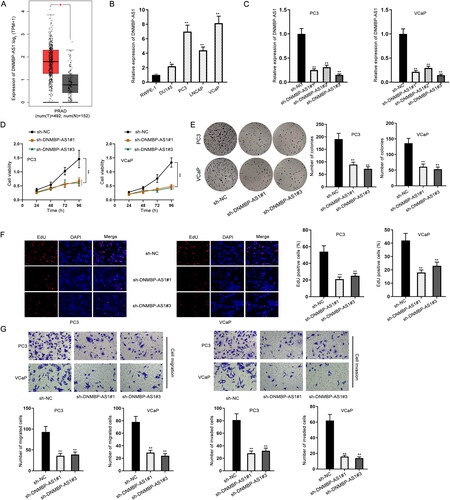
DNMBP-AS1 binds to miR-6766-3p
Recently, Accumulating studies have indicated that lncRNAs can act to compete against endogenous RNAs (ceRNAs) to sponge microRNAs (miRNAs) at the post-transcriptional level (Paraskevopoulou and Hatzigeorgiou Citation2016). Based on the subcellular fractionation and FISH assays, DNMBP-AS1 was found to be distributed mainly in cell cytoplasm (). To explore whether DNMBP-AS1 functioned as a ceRNA, miR-6766-3p was screened out since it had the highest binding score using starBase and DIANA (https://diana.e-ce.uth.gr/lncbasev3) database. We clearly observed the down-regulation of miR-6766-3p in PCa cells (). The binding sites of miR-6766-3p to DNMBP-AS1 are provided in . RNA pull-down assays revealed that DNMBP-AS1 was enriched in biotinylated miR-6766-3p probe containing the wild-type binding sites (). The expression of miR-6766-3p was enhanced by the transfection of miR-6766-3p mimics (). After transfection of VCaP and PC3 cells with miR-6766-3p mimics or NC mimics, luciferase reporter gene assay was carried out. It was observed that overexpression of miR-6766-3p decreased the luciferase activity of DNMBP-AS1-WT, while no significant change was observed in DNMBP-AS1-Mut (). To be concluded, miR-6766-3p can be sponged by DNMBP-AS1 in PCa cells.
Figure 2. DNMBP-AS1 binds to miR-6766-3p. (A–B) Subcellular fractionation and FISH assays were performed to identify the cellular location of DNMBP-AS1 in PC3 and VCaP cells. (C) RT-qPCR result of miR-6766-3p expression in PCa cells and human normal cell line. (D) The binding sites of miR-6766-3p to DNMBP-AS1. (E) RNA pull down assay was performed using the biotinylated miR-6766-3p probes to detect the enrichment of DNMBP-AS1. (F) The efficiency of miR-6766-3p mimics in PC3 and VCaP cells was detected by RT-qPCR. (G) Luciferase reporter gene assay was carried out to confirm the combination of DNMBP-AS1 and miR-6766-3p. *p < 0.05, **p < 0.01.
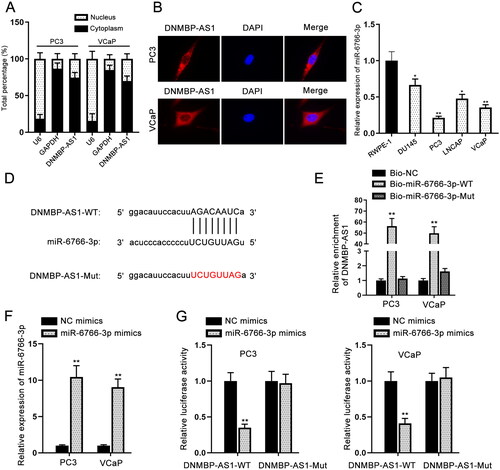
MiR-6766-3p directly targets LCLAT1 in PCa cells
The 11 downstream target genes of miR-6766-3p were obtained by starBase and GEPIA databases (). Among these mRNAs, LCLAT1 expression in PCa cells was most up-regulated via RT-qPCR analysis (). We then implemented GEPIA and starBase databases to detect LCLAT1 expression in PRAD tissues and normal tissues. The results demonstrated that LCLAT1 was significantly up-regulated in PRAD tissues compared with normal tissues (supplemental Figure S1B–C). Moreover, the data from starBase database showed that DNMBP-AS1 was positively correlated with LCLAT1 in PRAD tissues (supplemental Figure S1D). Furthermore, the high enrichment of LCLAT1 was found in Bio-miR-6766-3p-WT group (), implying the binding of miR-6766-3p to LCLAT1. The binding sequence between miR-6766-3p and LCLAT1 was demonstrated in . Luciferase reporter gene assay was further conducted and presented that the luciferase activity of LCLAT1-WT was markedly weakened in response to miR-6766-3p overexpression (). Thereafter, RIP assay was undertaken using the antibody against Ago2 protein, a key component of RNA induced silencing complex (RISC). As illustrated in , in comparison with the control Immunoglobulin G (IgG), endogenous DNMBP-AS1, miR-6766-3p and LCLAT1 enriched in Ago2-immunoprecipitate. Taken together, LCLAT1 can be targeted by miR-6766-3p in PCa cells.
Figure 3. MiR-6766-3p directly targets LCLAT1 in PCa cells. (A) Eleven candidate target genes were predicted from starBase. (B) RT-qPCR data for the expression profiles of mRNAs in PCa cells. (C) RNA pull down assay was performed using the biotinylated miR-6766-3p probes to detect LCLAT1 enrichment. (D) The binding sites of miR-6766-3p and LCLAT1 were predicted by starBase. (E) Luciferase reporter gene assay was carried out for proving the interaction of miR-6766-3p and LCLAT1. (F) RIP assay was undertaken for detecting whether DNMBP-AS1, miR-6766-3p and LCLAT1 existed in RISC complex. *p < 0.05, **p < 0.01.
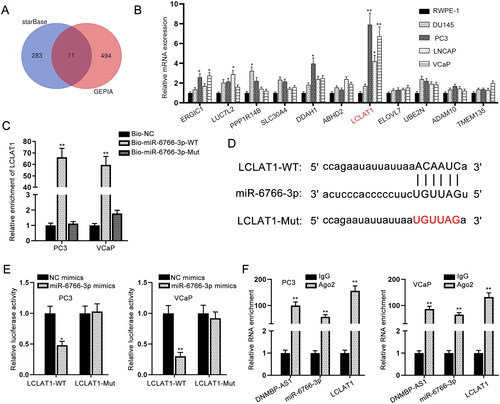
DNMBP-AS1 acts as a tumor promoter in PCa via targeting miR-6766-3p/LCLAT1 axis
By the transfection of pcDNA3.1/LCLAT1, LCLAT1 was overexpressed in PC3 cells. Similarly, miR-6766-3p expression was down-regulated after the transfection of miR-6766-3p inhibitor (). Through experimental results of cell proliferation assays, we discovered that LCLAT1 overexpression could completely rescue suppressed cell proliferation due to DNMBP-AS1 silencing, while miR-6766-3p inhibitor only partly restored this inhibitory effect (). Transwell migration and invasion assays displayed that DNMBP-AS1 inhibition repressed migratory and invasive capacities in PC3 cells. However, the effect was completely offset by up-regulation of LCLAT1 and partly countervailed by miR-6766-3p inhibitor (). Collectively, DNMBP-AS1 is involved in PCa development through miR-6766-3p/LCLAT1 axis.
Figure 4. DNMBP-AS1 acts as a tumor promoter in PCa via targeting miR-6766-3p/LCLAT1 axis. (A) The overexpression efficiency of LCLAT1 and interference efficiency of miR-6766-3p were detected by RT-qPCR. (B–D) Cell proliferation was detected in the sh-NC group, sh-DNMBP-AS1#3 group, sh-DNMBP-AS1#3 + miR-6766-3p inhibitor group and sh-DNMBP-AS1#3 + pcDNA3.1/LCLAT1 group. (E) Transwell assay was implemented to assess cell migration and invasion in PC3 cells transfected with sh-NC, sh-DNMBP-AS1#3, sh-DNMBP-AS1#3 + miR-6766-3p inhibitor and sh-DNMBP-AS1#3 + pcDNA3.1/LCLAT1 plasmids. *p < 0.05, **p < 0.01.
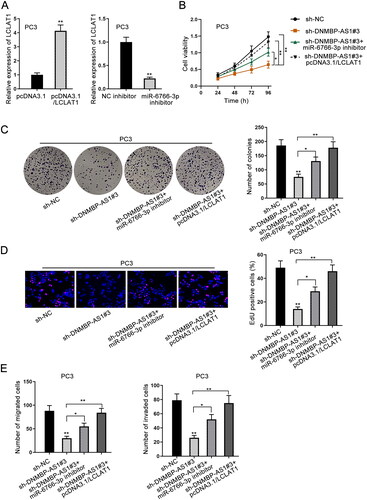
DNMBP-AS1 recruited ELAVL1 to stabilize LCLAT1 expression
Through previous rescue experiments, we speculated that DNMBP-AS1 might regulate LCLAT1 expression in another manner. RNA binding protein (RBP) network is emerging as a typical manner whereby lncRNAs can modulate gene expression at post-transcriptional level (Kim et al. Citation2009). Herein, DNMBP-AS1 might regulate LCLAT1 expression by binding with certain proteins. With the application of starBase, we found four RBPs (ELAVL1, U2AF2, HNRNPA1 and SRSF1) could bind to both DNMBP-AS1 and LCLAT1 (). Among the four RBPs, only ELAVL1 has been reported to be able to stabilize mRNA expression (Chen et al. Citation2020). RIP and RNA pull down assays were both carried out and the results elucidated that ELAVL1 can bind with DNMBP-AS1 or LCLAT1 (). ELAVL1 was knocked down at the mRNA and protein levels by sh-ELAVL1#1 and sh-ELAVL1#2 (). More importantly, LCLAT1 expression also decreased owing to inhibition of ELAVL1 (). After that, Actinomycin D (Act D) assay was performed. The positive regulation of ELAVL1 and DNMBP-AS1 on LCLAT1 mRNA stability was verified (). All in all, DNMBP-AS1 stabilizes LCLAT1 mRNA expression by recruiting ELAVL1.
Figure 5. DNMBP-AS1 recruited ELAVL1 to stabilize LCLAT1 expression. (A) Four RBPs of both DNMBP-AS1 and LCLAT1 were predicted by starBase. (B) RIP assay was undertaken by use of ELAVL1 antibody to assess the enrichment of DNMBP-AS1 and LCLAT1. (C) RNA pull down assay was performed using biotinylated probes of DNMBP-AS1 for confirming the binding of ELAVL1 and DNMBP-AS1. (D–E) ELAVL1 expression was reduced by sh-ELAVL1#1 and sh-ELAVL1#2 at mRNA level and protein level in PC3 and VCaP cells. (F–G) LCLAT1 expression was decreased at mRNA level and protein level due to depletion of ELAVL1. (H) The regulation of DNMBP-AS1 and ELAVL1 on LCLAT1 mRNA stability was verified via Actinomycin D (Act D) treatment assay. **p < 0.01.
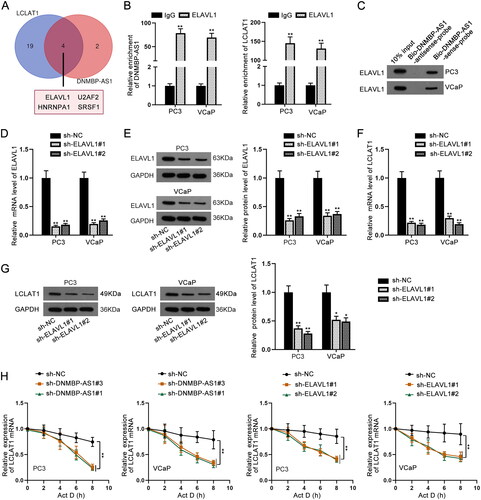
Scientific concept map describes the functional mechanism of DNMBP-AS1 in PCa cells
We concluded that DNMBP-AS1 promoted PCa cell proliferation, migration and invasion through sponging miR-6766-3p to upregulate LCLAT1 expression. Also, DNMBP-AS1 recruited ELAVL1 to stabilize LCLAT1 mRNA level ().
Discussion
Prostate cancer (PCa) is a commonly diagnosed malignant male cancer and is one of the prime reasons for cancer-related death (Li and Zhang Citation2017). Lacking biomarkers in early diagnosis hinders the treatment and prognosis of PCa. Increasing numbers of researches have demonstrated that dysregulation of lncRNAs participates in the recurrence, metastasis and prognosis of PCa (Guo et al. Citation2013). In our current study, the obvious up-regulation of lncRNA DNMBP-AS1 was identified in PCa cells, which prompted us to further explore its regulatory roles in PCa. Loss-of-function assays testified that DNMBP-AS1 depletion repressed cell proliferation, migration and invasion, suggesting the oncogenic property of DNMBP-AS1 in PCa cells.
Numerous studies have indicated that the ceRNA network occupies an important position in PCa (Xu et al. Citation2018). In terms of post-transcriptional regulation, lncRNAs have been acknowledged to be ceRNAs by competitively binding to miRNA response elements to upregulate mRNAs. MiR-6766-3p has been identified to be a crucial miRNA in OPLL, and LCLAT1 has been reported to be associated with lipid metabolism (Zhao et al. Citation2020). According to the bioinformatics analysis and mechanism investigation, we determined that DNMBP-AS1 served as a miRNA sponge of miR-6766-3p to elevate LCLAT1 expression in PCa cells. Rescue assays demonstrated that miR-6766-3p inhibitor partly counteracted the suppressive effect of DNMBP-AS1 knockdown on PCa process while LCLAT1 completely offset the repressed PCa progression caused by DNMBP-AS1 depletion.
Targeting RBP-RNA interactions plays a major role in PCa (Fei Citation2017). Briefly, RBP modulates mRNAs at post-transcriptional level, thereby regulating human cancer progression. For instance, lncRNA AC245100.4 interacts with HSP90 protein to maintain the stability of IκB kinase (Cui et al. Citation2020). HuR is associated with PCa via stabilizing the mRNA of COX-2 (Niesporek et al. Citation2008). ELAVL1 has been reported to have the capacity to stabilize mRNA. For instance, ELAVL1 is recruited by lncRNA HOXB-AS1 and stabilizes FUT4 mRNA in multiple myeloma (Chen et al. Citation2020). LncRNA ZEB1-AS1 stabilizes ZEB1 mRNA via binding with ELAVL1 in triple negative breast cancer (Luo et al. Citation2020). In addition, ELAVL1 has been verified to be an independent predictor of PCa progression (Melling et al. Citation2016). In our study, bioinformatics analyses and mechanism assays disclosed that DNMBP-AS1 could stabilize LCLAT1 mRNA expression by recruiting RBP ELAVL1 in PCa cells.
Overall, these experimental results could draw a conclusion that lncRNA DNMBP-AS1 up-regulates LCLAT1 expression by sponging miR-6766-3p and recruiting RBP ELAVL1, thus contributing to PCa cell proliferation, migration and invasion. The function and mechanism of DNMBP-AS1 in PCa were elucidated. These findings might be considered as novel therapeutic targets for PCa in the future.
Materials and methods
Cell lines and reagents
Human normal prostatic epithelial cell line RWPE-1 was commercially acquired from American Type Culture Collection (ATCC, Manassas, VA, USA) and cultured in Keratinocyte Serum Free Medium (K-SFM; GIBCO, Rockville, MD, USA). Human PCa cell lines (DU145, PC3, LNCAP and VCaP) were also purchased from ATCC. DU145 cell line was cultured in EMEM. PC3 cell line was maintained in F-12K Medium. LNCAP cell line was routinely maintained in RPMI-1640 medium and VCaP cell line was incubated in DMEM. All mediums were added with 10% fetal bovine serum (FBS; GIBCO).
Total RNA extraction and quantitative real-time PCR (RT-qPCR)
Total RNA extraction was achieved by TRIzol Reagent (Invitrogen, Carlsbad CA, USA). Then, cDNA synthesis was performed using PrimeScript™ II Reverse Transcriptase (2690 A; TaKaRa, Shiga, Japan) in line with the standard method. Quantitative analysis was conducted with application of SYBR Green PCR Kit (Takara) on ABI Prism 7900HT sequence detector (Applied Biosystems, Foster City, CA). Results were analyzed using 2−ΔΔCt method and standardized to GAPDH or U6.
Cell transfection
The specific short hairpin RNAs (shRNAs) targeting DNMBP-AS1 or ELAVL1 were synthesized by GenePharma (Shanghai, China), and nonspecific shRNAs were used as negative control (NC). The pcDNA3.1 vectors (GenePharma) targeting LCLAT1, and the empty pcDNA3.1 vectors were acquired for gene overexpression study. MiR-6766-3p mimics/inhibitor and NC mimics/inhibitor were designed by Ribobio (Guangzhou, China). Cell transfection was performed for 48 h with Lipofectamine 3000 (Invitrogen) in line with user guides.
Cell counting kit-8 (CCK-8) assay
5 × 103 transfected cells of PC3 and VCaP were cultured into the 96-well plates for CCK-8 assay. 10 μL of CCK-8 solution (Dojindo Laboratories, Kumamoto, Japan) was then added for 2 h. Cell viability was examined at indicated time points using spectrophotometer (Thermo Fisher Scientific, Waltham, MA). The experiment was independently performed at least three times.
Colony formation assay
After transfection, PC3 and VCaP cells were seeded into 6-well plates with 600 cells in each well for 14 days. Then, colonies were fixed in methanol for staining with 0.5% crystal violet solution. Visible clones were manually counted. The experiment was independently performed at least three times.
5-Ethynyl-20-deoxyuridine (EdU) staining assay
1 × 104 transfected PC3 and VCaP cells were collected and cultured in 96-well plates for 2 h of incubation with EdU staining kit (Ribobio). After that, cells were treated in DAPI staining solution for 5 min and observed under fluorescence microscope (Olympus, Tokyo, Japan). The experiment was independently performed at least three times.
Transwell assay
2 × 104 PC3 and VCaP cells were collected after transfection, and then seeded with serum-free medium into the upper chamber of Transwell chambers (24-well; Corning Incorporated, Corning, NY) for testing cell migration. Cell invasion was assessed using Matrigel membrane (BD Biosciences, Franklin Lakes, NJ). Lower chamber was filled with the complete culture medium. 24 h later, migrated or invaded cells were fixed, stained by crystal violet, and finally visualized using optical microscope (Olympus). The experiment was independently performed at least three times.
Subcellular fractionation
Subcellular fractionation assay was undertaken as per the instruction of PARIS™ Kit (Invitrogen). Cell fractionation buffer was then added to isolate cell cytoplasm, followed by addition of cell disruption buffer to acquire cell nucleus. DNMBP-AS1 content was examined by RT-qPCR. The experiment was independently performed at least three times.
Fluorescent in situ hybridization (FISH)
RNA FISH probe (Ribobio) was designed for DNMBP-AS1 and utilized as guided by provider. After hybridization with FISH probe, cells were counterstained with DAPI solution and finally detected using fluorescence microscope (Olympus). The experiment was independently performed at least three times.
RNA pull down assay
RNA pull down assay was undertaken by Pierce Magnetic RNA-Protein Pull-Down Kit (Thermo Fisher Scientific, Waltham, MA), in light of the provided instruction. The cell extracts from PC3 and VCaP cells were used for incubation with biotinylated probes of DNMBP-AS1 or miR-6766-3p-WT/Mut. After the addition of magnetic beads, relative RNA enrichment was assessed by RT-qPCR or western blot. The experiment was independently performed at least three times.
Luciferase reporter gene assay
The fragments of DNMBP-AS1 or LCLAT1 3’-UTR covering wild-type and mutant miR6766-3p binding sites were inserted into pmirGLO luciferase reporter gene (Promega, Madison, WI). PC3 and VCaP cells were co-transfected with miR-6766-3p mimics or NC mimics and pmirGLO luciferase reporter gene for 48 h, followed by analysis of Dual-luciferase reporter assay system (Promega). The experiment was independently performed at least three times.
RNA-binding protein immunoprecipitation (RIP)
RIP assay was achieved by Magna RIP™ RNA-Binding Protein Immunoprecipitation Kit (Millipore, Bedford, MA). After the lysis in RIP lysis buffer, cell lysates were cultivated with the magnetic beads conjugated with Ago2 antibody or ELAVL1 antibody. IgG antibody was used in NC control. All results were monitored using RT-qPCR. The experiment was independently performed at least three times.
Western blot
Total protein from PC3 and VCaP cells were shifted onto PVDF membranes after separation on 12% SDS-PAGE, and then blocked in 5% nonfat milk. The primary antibodies against ELAVL1 (Abcam), LCLAT1 (Abcam) and GAPDH (Abcam) were used for incubation with cellular protein overnight at 4 °C. Secondary antibodies were labeled with Horseradish Peroxidase (HRP) and added for 2 h at room temperature. The protein signals were studied using ECL luminous liquid (Pierce, Rockford, IL). The experiment was independently performed at least three times.
Statistical analyses
Experimental results were represented as the mean ± standard deviation (SD) for triplicate samples and analyzed by GraphPad PRISM 6 (GraphPad, San Diego, CA). Group difference was monitored by Student’s t-test or one-way/two-way ANOVA, which was considered to be significant when p < 0.05.
Authors’ contributions
Contributed to the design of the study and wrote the manuscript: XY. Performed the experiments: SW, RG. Analyzed the data: JC, YG. Prepared all figures: XY, SX. All authors read and approved the final manuscript.
Abbreviations | ||
PCa | = | Prostate cancer |
lncRNAs | = | long non-coding RNAs |
DNMBP-AS1 | = | DNMBP antisense RNA 1 |
miR-6766-3p | = | microRNA-6766-3p |
LCLAT1 | = | lysocardiolipin acyltransferase 1 |
ELAVL1 | = | ELAV like RNA binding protein 1 |
ATCC | = | American Type Culture Collection |
FBS | = | fetal bovine serum |
RT-qPCR | = | Quantitative real-time PCR |
shRNAs | = | short hairpin RNAs |
CCK-8 | = | Cell counting kit-8 |
EdU | = | 5-ethynyl-20-deoxyuridine |
FISH | = | Fluorescent in situ hybridization |
RIP | = | RNA-binding protein immunoprecipitation |
3’-UTR | = | 3’-untranslated region |
SD | = | standard deviation |
Act D | = | Actinomycin D |
ceRNA | = | competing endogenous RNA |
miRNAs | = | microRNAs |
IgG | = | Immunoglobulin G |
RBP | = | RNA binding protein |
mRNAs | = | messenger RNAs |
MREs | = | miRNA response elements |
Supplemental Material
Download TIFF Image (1 MB)Supplemental Material
Download MS Word (14.7 KB)Supplemental Material
Download Zip (1,014.8 KB)Acknowledgments
We are grateful to the help from all lab personnel in this research. Part of the experiment in this paper was completed by Beijing Jinglai Huake Biotechnology Co., Ltd. We thank each partner in our team and the kind people who gave us a hand during this work.
Disclosure statement
No potential conflict of interest was reported by the author(s).
Additional information
Funding
References
- Bai J, Huang G. 2020. Role of long non-coding RNA NEAT1 in the prognosis of prostate cancer patients. Medicine. 99(22):e20204.
- Bolha L, Ravnik-Glavač M, Glavač D. 2017. Long noncoding RNAs as biomarkers in cancer. Dis Markers. 2017:7243968.
- Charles Richard JL, Eichhorn PJA. 2018. Platforms for investigating LncRNA functions. SLAS Technol. 23(6):493–506.
- Chen R, Zhang X, Wang C. 2020. LncRNA HOXB-AS1 promotes cell growth in multiple myeloma via FUT4 mRNA stability by ELAVL1. J Cell Biochem. 121(10):4043–4051.
- Cui R, Liu C, Lin P, Xie H, Wang W, Zhao J, Jiang S, Shi J, Yu X. 2020. LncRNA AC245100.4 binds HSP90 to promote the proliferation of prostate cancer. Epigenomics. 12(15):1257–1271.
- Fei T. 2017. Targeting RNA binding protein in prostate cancer. Mol Cell Oncol. 4(5):e1353855.
- Guo G, Kang Q, Zhu X, Chen Q, Wang X, Chen Y, Ouyang J, Zhang L, Tan H, Chen R, et al. 2015. A long noncoding RNA critically regulates Bcr-Abl-mediated cellular transformation by acting as a competitive endogenous RNA. Oncogene. 34(14):1768–1779.
- Guo XQ, Lan T, Wang YM. 2013. [LncRNA in prostate cancer: an update]. Zhonghua Nan Ke Xue. 19:826–830.
- Kim MY, Hur J, Jeong S. 2009. Emerging roles of RNA and RNA-binding protein network in cancer cells. BMB Rep. 42(3):125–130.
- Li HZ, Zhang YS. 2017. [Review of hot topics in the diagnosis and treatment of prostate cancer in 2016] Zhonghua wai ke za zhi. Zhonghua Wai Ke Za Zhi. 55(1):59–62.
- Litwin MS, Tan HJ. 2017. The diagnosis and treatment of prostate cancer: a review. Jama. 317(24):2532–2542.
- Liu Y, Zhang R, Qiu F, Li K, Zhou Y, Shang D, Xu Y. 2015. Construction of a lncRNA-PCG bipartite network and identification of cancer-related lncRNAs: a case study in prostate cancer. Mol Biosyst. 11(2):384–393.
- Long B, Li N, Xu XX, Li XX, Xu XJ, Liu JY, Wu ZH. 2018. Long noncoding RNA LOXL1-AS1 regulates prostate cancer cell proliferation and cell cycle progression through miR-541-3p and CCND1. Biochem Biophys Res Commun. 505(2):561–568.
- Luo N, Zhang K, Li X, Hu Y. 2020. ZEB1 induced-upregulation of long noncoding RNA ZEB1-AS1 facilitates the progression of triple negative breast cancer by binding with ELAVL1 to maintain the stability of ZEB1 mRNA. J Cell Biochem. 121(10):4176–4187.
- Melling N, Taskin B, Hube-Magg C, Kluth M, Minner S, Koop C, Grob T, Graefen M, Heinzer H, Tsourlakis MC, et al. 2016. Cytoplasmic accumulation of ELAVL1 is an independent predictor of biochemical recurrence associated with genomic instability in prostate cancer. Prostate. 76(3):259–272.
- Mitobe Y, Takayama KI, Horie-Inoue K, Inoue S. 2018. Prostate cancer-associated lncRNAs. Cancer Lett. 418:159–166.
- Niesporek S, Kristiansen G, Thoma A, Weichert W, Noske A, Buckendahl A-C, Jung K, Stephan C, Dietel M, Denkert C, et al. 2008. Expression of the ELAV-like protein HuR in human prostate carcinoma is an indicator of disease relapse and linked to COX-2 expression. Int J Oncol. 32:341–347.
- Paraskevopoulou MD, Hatzigeorgiou AG. 2016. Analyzing MiRNA-LncRNA Interactions. Methods Mol Biol. 1402:271–286.
- Prostate cancer 2016. Nursing standard (Royal College of Nursing (Great Britain): 1987) 30:17.
- Qi M, Hu J, Cui Y, Jiao M, Feng T, Li X, Pang Y, Chen X, Qin R, Su P, et al. 2019. CUL4B promotes prostate cancer progression by forming positive feedback loop with SOX4. Oncogenesis. 8(3):23.
- Siegel RL, Miller KD, Jemal A. 2015. Cancer statistics, 2015. CA Cancer J Clin. 65:5–29.
- Wang J, Su Z, Lu S, Fu W, Liu Z, Jiang X, Tai S. 2018. LncRNA HOXA-AS2 and its molecular mechanisms in human cancer. Clin Chim Acta. 485:229–233.
- Wang X, Xu Y, Wang X, Jiang C, Han S, Dong K, Shen M, Xu D. 2017. LincRNA-p21 suppresses development of human prostate cancer through inhibition of PKM2. Cell Prolif. 50(6):e12395.
- Xu N, Wu YP, Yin HB, Xue XY, Gou X. 2018. Molecular network-based identification of competing endogenous RNAs and mRNA signatures that predict survival in prostate cancer. J Transl Med. 16(1):274.
- Zhang Y, Tang L. 2018. The application of lncRNAs in cancer treatment and diagnosis. Recent Pat Anticancer Drug Discov. 13(3):292–301.
- Zhao L-J, Zhao H-Y, Wei X-L, Guo F-F, Wei J-Y, Wang H-J, Yang J, Yang Z-G, Si N, Bian B-L, et al. 2020. The lipid homeostasis regulation study of arenobufagin in zebrafish HepG2 xenograft model and HepG2 cells using integrated lipidomics-proteomics approach. J Ethnopharmacol. 260:112943.
- Zhu ZM, Liu FT, Chen X. 2018. Low expression of LncRNA cancer susceptibility candidate 2 and its clinical significance in cancer tissues cellular physiology and biochemistry: international journal of experimental cellular physiology. Cell Physiol Biochem. 46(4):1643–1649.