Abstract
There is evidence of the existence of an intraovarian gonadotropin-releasing hormone (GnRH) system. There are also reports about the influence of extrinsic ovarian innervation in gonadal function. Therefore, it is interesting to study the relationship between ovarian sympathetic innervation and GnRH to shed light on possible physiological and pathophysiological implications. This work aimed to investigate whether noradrenergic stimulation of the superior mesenteric ganglion (SMG) can modify the levels of ovarian GnRH and cause functional and morphological changes in the gonad through the ovarian plexus nerve (OPN), during estrus and diestrus II in rats. The SMG-OPN-Ovary system and an ovary without extrinsic innervation were removed from Holtzman rats in estrus and diestrus II stages and placed in specially designed cuvettes containing Krebs-Ringer buffer. In the experimental groups, SMGs and denervated ovaries were stimulated with 10−6 M noradrenaline (NA). GnRH and progesterone levels (in the ovarian incubation medium) and the mRNA expression of 3beta-hydroxysteroid dehydrogenase (Hsd3b3), 20alpha-hydroxysteroid dehydrogenase (Akr1c18), Bax, and Bcl2 were analyzed. Histological studies of the ovaries were performed. In estrus, NA decreased GnRH levels in both experimental schemes. Furthermore, progesterone levels increased while the Akr1c18 expression and Bax/Bcl2 ratio decreased, without causing changes in ovarian morphology. In diestrus, the noradrenergic stimulation of the ganglion increased GnRH levels, decreased progesterone levels, and increased Akr1c18 expression and Bax/Bcl2 ratio. Follicles with histoarchitecture alterations and corpus luteum with signs of cell death were observed. In denervated ovaries, NA increased the levels of GnRH and progesterone. Furthermore, NA decreased the Bax/Bcl2 ratio and histological studies revealed signs compatible with a possible atretogenic effect. In conclusion, noradrenergic stimulation of the SMG-OPN pathway regulates ovarian cyclicity. The SMG modulates the cross-talk between NA and ovarian GnRH, protecting the ovary from atretogenic effects and luteal apoptosis during estrus while inducing luteal regression in the diestrus II.
Introduction
Extrinsic innervation of the mammalian ovary involves two sources: the superior ovarian nerve (SON), which is organized around the follicles, and the ovarian plexus nerve (OPN), which is mainly associated with the vasculature (del Campo et al. Citation2019). Both sources are constituted by noradrenergic/peptidergic nerve fibers (Aguado Citation2002; Domínguez and Cruz-Morales Citation2011). The superior mesenteric ganglion (SMG), a sympathetic prevertebral ganglion, contains neurons whose axons make up the OPN.
A wide variety of neurotransmitters (including noradrenaline) and specific receptors can be found in the SMG (Elfvin et al. Citation1993; Linares et al. Citation2019; Ernsberger et al. Citation2020). Tyrosine hydroxylase and dopamine beta-hydroxylase, two crucial enzymes in the synthesis of noradrenaline (NA), are expressed in the celiac ganglion-SMG complex (Ernsberger et al. Citation2020). In addition, literature suggests that alpha and beta-adrenergic receptors are present in this ganglia complex (Casais et al. Citation2006). In the ovary, alpha- and beta-adrenergic receptors are known to be present in follicular cells (the theca and granulosa cells), corpus luteum, and interstitial tissue (Itoh and Ishizuka Citation2005; Merz et al. Citation2015). Our research group has shown that adrenergic and noradrenergic stimulations of the celiac ganglion cause changes in physiological function of the gonad through SON (Sosa et al. Citation2000; Garraza et al. Citation2004; Bronzi et al. Citation2015). We have also reported that noradrenergic stimulation of both the celiac ganglion-SON sympathetic pathway and the ovary without peripheral neural influence modifies the concentration of ovarian gonadotropin-releasing hormone (GnRH) (Bronzi et al. Citation2015). In both humans and rodents, GnRH production and GnRH receptor expression by granulosa cells and granulosa-lutein cells have been reported (Schirman-Hildesheim et al. Citation2005; Choi et al. Citation2006; Shahed and Young Citation2011). Furthermore, the existence of a local, paracrine, GnRH-gonadotropic axis in the ovaries has been suggested (Schirman-Hildesheim et al. Citation2008). Through the use of GnRH agonists, it has been determined that this decapeptide can stimulate or inhibit ovarian steroidogenesis (Guerrero et al. Citation1993; Irusta et al. Citation2007), affect follicular development and luteinization (Singh and Krishna Citation2010), even promote luteal cell apoptosis (Goto et al. Citation1999; Scotti et al. Citation2011).
Some studies show that the SMG-OPN pathway can modulate steroidogenesis, apoptosis, and even angiogenesis and proliferation in the ovary (Orozco et al. Citation2006; Vega Orozco et al. Citation2010, Citation2012; Daneri et al. Citation2013; Cáceres et al. Citation2021). However, the implication of NA in the SMG-OPN sympathetic pathway has not been fully explored. In the ovary, it is known that NA can modulate steroidogenesis (Miszkiel and Kotwica Citation2001; Garraza et al. Citation2004; Itoh and Ishizuka Citation2005; Delgado et al. Citation2010; Bronzi et al. Citation2015; Delsouc et al. Citation2019), ovulation (Jørgensen et al. Citation1991), and corpus luteum function (Vega Orozco et al. Citation2017). Therefore, this work aimed to investigate whether noradrenergic stimulation of the SMG can modify the ovarian GnRH levels and cause functional and morphological changes in the gonad, through the OPN, during estrus and diestrus II in rats.
Results
Effect of ex vivo noradrenergic stimulation of SMG and denervated ovary on ovarian GnRH and progesterone levels in the estrus stage
We analyze the effects of noradrenergic stimulation of the SMG-OPN pathway on ovarian physiology (), beginning by identifying possible changes in ovarian GnRH release. In the absence of stimulus, the GnRH levels in the ovarian incubation medium increased during the incubation period of the ex vivo system (p < 0.001), but declined during incubation of the denervated ovaries (p < 0.01). The addition of NA into the SMG compartment decreased the ovarian GnRH release at both study times (60 min: p < 0.05; 120 min: p < 0.001), compared to the control group (). Our findings prove that the ex vivo SMG-OPN-Ovary system is viable, as shown in our previous publications, and that the pathway has implications for ovarian physiology.
Figure 1. Schematic diagram of experimental model. (A) Schematic representation of the extrinsic innervation of the ovary studied. Crosses mark the surgical sections made to extract the SMG-OPN-Ovary system. CG: Celiac ganglion; SMG: Superior mesenteric ganglion; SON: Superior ovarian nerve; OPN: Ovarian plexus nerve, L (1, 2, 3, 4): Lumbar ganglion of the sympathetic trunk. (B) Diagram of the cuvette used for the incubation of the ex vivo SMG-OPN-Ovary system with addition of 10−6M Noradrenaline (NA) in the ganglionic compartment. (C) Diagram of the cuvette used for the denervated ovary incubation, with the addition of the same stimulus.
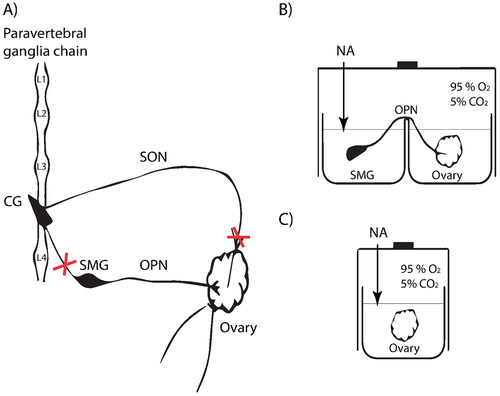
Figure 2. Effect of noradrenergic stimulation of SMG and denervated ovary on ovarian GnRH and progesterone levels in the estrus stage. The levels of GnRH (A), expressed as pg/mg ovary/ml, and progesterone (B), expressed as ng/mg ovary/ml, were measured in the incubation medium of the ovary with and without peripheral neural influence. These results are expressed as mean ± SEM (n = 6 rats per experimental group). Two-way ANOVA followed by Bonferroni test was used. The mRNA expression of Hsd3b3 (C) and Akr1c18 (D) were determined in the ovarian tissue. These results are expressed as mean ± SEM (n = 3 pools of 2 ovaries per pool). Densitometry analysis of the bands in the gel photographs was performed using the ImageJ software and expressed as relative units. Actb was used as the housekeeping gene. Data were analyzed by one-way ANOVA followed by Tukey’s test were used. *p < 0.05; ** p < 0.01; ***p < 0.001. Actb: actin beta; Akr1c18: 20alpha-hydroxysteroid dehydrogenase; GnRH: gonadotropin-releasing hormone; Hsd3b3: 3beta-hydroxysteroid dehydrogenase; NA: Noradrenaline; SMG: superior mesenteric ganglion.
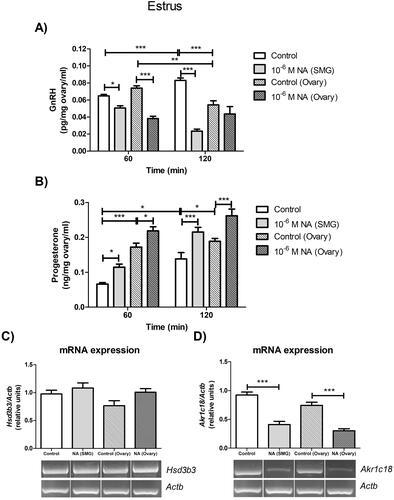
The noradrenergic stimulation of the gonad also caused a decrease in the GnRH levels that was significant at 60 min of incubation (p < 0.001) compared to the control group (). NA appears to have an inhibitory effect on GnRH in estrus, but the potential for other neurotransmitters in the ovary should not be ruled out.
It is known that NA can modulate steroidogenesis and, consequently, we analyzed the progesterone levels in the incubation medium of the ovary. In the estrus stage and without external stimuli, progesterone levels increased during the incubation period of the ex vivo system (p < 0.05). In the incubation medium of the denervated ovaries, similar levels of progesterone were determined at 60 and 120 min. However, progesterone release from denervated ovaries was higher compared to ovaries incubated under the influence of the SMG-OPN pathway (60 min: p < 0.001; 120 min: p < 0.05) (). In both experimental schemes, NA caused an increase in ovarian progesterone release (60 min: p < 0.05; 120 min: p < 0.001) compared to the control groups (). However, no changes were observed in the Hsd3b3 mRNA expression (), although the noradrenergic stimulus decreased the Akr1c18 mRNA expression in the ovary with and without peripheral neural influence (p < 0.001) (). These results continue to show that NA regulates ovarian steroidogenesis, and this seems to be associated with changes in GnRH levels.
Effect of ex vivo noradrenergic stimulation of SMG and denervated ovary on bax and Bcl2 mRNA expression in estrus ovary
Apoptosis is a physiological process of cell death that occurs in the ovary at various moments in the estrous cycle. Anomalies in this process can give rise to different pathologies. Therefore, we analyzed two apoptotic factors to determine whether NA can modify their mRNA expression, in addition to the observed changes in GnRH and progesterone. In the ex vivo SMG-OPN-Ovary system, the addition of 10−6 M NA into the SMG compartment decreased the Bax mRNA expression (p < 0.001) and increased the Bcl2 mRNA expression p < 0.05) in the ovarian tissue, as compared with the control group (). In denervated ovaries, the addition of 10−6 M NA also decreased the Bax mRNA expression (p < 0.05) and increased the Bcl2 mRNA expression (p < 0.01), as compared with the control group (). Consequently, the Bax/Bcl2 ratio decreased in both experimental schemes by stimulation with NA (p < 0.001) (). These results suggest that NA is a regulator of ovarian function and that, related to this, sympathetic innervation modulates apoptotic processes. It seems that NA prevents apoptotic processes in the estrus ovary and this effect would correspond to a decrease in GnRH levels and an increase in progesterone levels.
Figure 3. Effect of noradrenergic stimulation of SMG and denervated ovary on apoptotic gene expressions in estrus ovary. (A) Bax mRNA expression; (B) Bcl2 mRNA expression; (C) Bax/Bcl2 ratio. These results are expressed as mean ± SEM (n = 3 pools of 2 ovaries per pool). Densitometry analysis of the bands in the gel photographs was performed using the ImageJ software and expressed as relative units. Actb was used as the housekeeping gene. Data were analyzed by one-way ANOVA followed by Tukey’s test were used. *p < 0.05; **p < 0.01; ***p < 0.001. Actb: actin beta; NA: Noradrenaline; SMG: superior mesenteric ganglion.
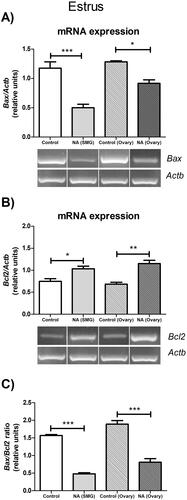
Effect of ex vivo noradrenergic stimulation of SMG and denervated ovary on ovarian GnRH and progesterone levels in the diestrus II stage
After obtaining interesting findings in the estrus, we analyzed the effects of noradrenergic stimulation of the SMG-OPN pathway on the ovary during the late luteal phase, starting with the analysis of GnRH. In the absence of stimulus, the GnRH levels in ovarian medium increased during the incubation period of the ex vivo system (p < 0.01) and remained constant during the incubation of the denervated ovaries, although at levels higher than those reported for the ex vivo system (p < 0.001) (). It is noteworthy that the addition of NA in the SMG compartment significantly increased the GnRH levels only at 60 min (p < 0.05), compared to the control group (). In the ovary without peripheral neural influence, the NA stimulus increased the GnRH levels at both study times (p < 0.001), compared to the control group (). Thus, noradrenergic stimulation of the SMG or ovary increases the release of ovarian GnRH in the late luteal phase, which is opposite to the effect observed in the estrus. Elevated levels of GnRH in the denervated ovary suggest the release of a neurotransmitter from OPN to the ovary, with an inhibiting effect on GnRH.
Figure 4. Effect of noradrenergic stimulation of SMG and denervated ovary on ovarian GnRH and progesterone levels in the diestrus II stage. The levels of GnRH (A), expressed as pg/mg ovary/ml, and progesterone (B), expressed as ng/mg ovary/ml, were measured in the incubation medium of the ovary with and without peripheral neural influence. These results are expressed as mean ± SEM (n = 6 rats per experimental group). Two-way ANOVA followed by Bonferroni test was used. The mRNA expression of Hsd3b3 (C) and Akr1c18 (D) were determined in the ovarian tissue. These results are expressed as mean ± SEM (n = 3 pools of 2 ovaries per pool). Densitometry analysis of the bands in the gel photographs was performed using the ImageJ software and expressed as relative units. Actb was used as the housekeeping gene. Data were analyzed by one-way ANOVA followed by Tukey’s test were used. *p < 0.05; **p < 0.01; ***p < 0.001. Actb: actin beta; Akr1c18: 20alpha-hydroxysteroid dehydrogenase; GnRH: gonadotropin-releasing hormone; Hsd3b3: 3beta-hydroxysteroid dehydrogenase; NA: Noradrenaline; SMG: superior mesenteric ganglion.
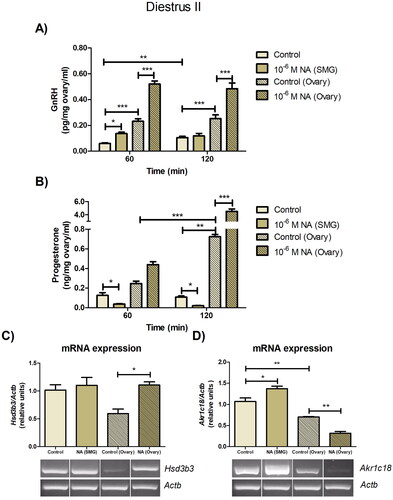
With regard to progesterone, its levels in ovarian medium did not change during the incubation period of the ex vivo system, although they increased during the incubation period of the denervated ovaries (p < 0.001). This result showed correspondence with a lower mRNA expression of Akr1c18 with respect to the ovary of the system (p < 0.01), suggesting that the SMG-OPN pathway has implications for the progesterone steroid in the late luteal phase. In fact, the addition of 10−6 M NA into the SMG compartment decreased the progesterone levels in ovarian medium at 60 and 120 min (p < 0.05), with respect to the control group (). Although noradrenergic stimulation of the ganglion did not alter the Hsd3b3 mRNA expression (), it increased the Akr1c18 mRNA expression in the ovarian tissue (p < 0.05), compared to the control group (). In contrast, the addition of 10−6 M NA into the ovary compartment increased progesterone levels (120 min: p < 0.001), in comparison with the control group (). Furthermore, NA increased the Hsd3b3 mRNA expression (p < 0.05) () and decreased the Akr1c18 mRNA expression (p < 0.01) (). It seems that the progesterone production is a complexly regulated matter in diestrus II. NA receptors and GnRH receptors are expressed in granulosa cells and luteal cells (essential sources of progesterone), so both would be involved in the regulation of the synthesis of this steroid.
Effect of ex vivo noradrenergic stimulation of SMG and denervated ovary on bax and Bcl2 mRNA expression in the diestrus II ovary
Noradrenergic stimulation of the SMG and ovary has an impact on the levels of GnRH and progesterone in the ovaries, two factors relevant to apoptosis. Therefore, we analyzed possible changes in the ovarian expression of Bax and Bcl-2 during late diestrus. In control conditions, ovaries without peripheral neural influence exhibited a decreased expression of Bcl2 and an increased Bax/Bcl2 ratio (p < 0.001), compared to the ovary of the SMG-OPN-Ovary system. The addition of 10−6 M NA into the SMG compartment increased the Bax mRNA expression (p < 0.05) () and decreased the Bcl2 mRNA expression (p < 0.001) (). In consequence, the SMG stimulation with NA increased the Bax/Bcl2 ratio in the ovarian tissue (p < 0.01) (), as compared with the control group. In the denervated ovary, the addition of 10−6 M NA also increased the Bax mRNA expression and decreased the Bcl2 mRNA expression (p < 0.05) () and, consequently, increased the Bax/Bcl2 ratio (p < 0.001) (), compared to the control group. These findings in diestrus II continue to argue that the SMG-OPN pathway modulates the apoptotic processes that occur in the ovary. However, contrary to what has been observed in estrus, noradrenergic stimulation of the SMG or ovary appears to favor apoptosis and this effect may be related to high levels of GnRH.
Figure 5. Effect of noradrenergic stimulation of SMG and denervated ovary on apoptotic gene expressions in diestrus ovary. (A) Bax mRNA expression; (B) Bcl2 mRNA expression; (C) Bax/Bcl2 ratio. These results are expressed as mean ± SEM (n = 3 pools of 2 ovaries per pool). Densitometry analysis of the bands in the gel photographs was performed using the ImageJ software and expressed as relative units. Actb was used as the housekeeping gene. Data were analyzed by one-way ANOVA followed by Tukey’s test were used. *p < 0.05; **p < 0.01; ***p < 0.001. Actb: actin beta; NA: Noradrenaline; SMG: superior mesenteric ganglion.
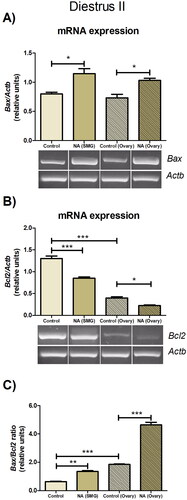
Effect of ex vivo noradrenergic stimulation of the SMG and denervated ovary on the ovarian histology
Interesting results on changes in apoptotic gene expression have led us to conduct histological studies to analyze the integrity of key ovarian structures. In the estrus stage, histological studies of the ovaries extracted from the ex vivo system and those incubated with NA did not show morphological changes compared to the controls (). However, in the diestrus II stage, the SMG stimulation with NA caused the presence of ovarian follicles with an altered histoarchitecture and corpus luteum with cellular disorganization and signs of nuclear and cytoplasmic alterations, compared to the control group. Furthermore, histological studies of NA-stimulated ovaries revealed signs consistent with a possible atretogenic effect (). Histological studies support our results described above suggesting a differential effect of NA throughout the estrous cycle. Norepinephrine stimulation of SMG and ovary appears to have a deleterious effect on ovarian structures during late diestrus, the stage considered most sensitive to neural influence.
Figure 6. Effect of ex vivo noradrenergic stimulation of SMG and denervated ovary on ovarian histology. Estrus stage: (A) Ovary control of the ex vivo system. Ovarian cortex, secondary follicles (arrow), and CL with normal histological structure are observed; (B) Ovary without peripheral neural influence. CL and antral follicle (*) with normal histological structure are observed; (C) Ovary under the influence of noradrenergic stimulation of SMG via OPN; (D) Ovary stimulated with 10−6 M NA. In both C and D, secondary follicles (arrow), antral follicle (*) and CL with preserved histology are observed. Diestrus II stage: (E) Ovary control of the ex vivo system. CL, primary follicle (arrowhead), and antral follicle (*) with normal histological structure are observed; (F) Ovary without peripheral neural influence. CL, secondary follicles (arrow), and antral follicle (*) with normal histological features are observed; (G) Ovary under the influence of noradrenergic stimulation of SMG via OPN. Some ovarian follicles present histoarchitecture alterations (white arrows). Inset: Disorganized luteal cells with signs of nuclear and cytoplasmic alterations (*); (H) Ovary stimulated with 10−6 M NA. Follicles with histological alterations are observed, compatible with a possible atretogenic effect (white arrows). n = 4 animals per experimental group. CL: corpus luteum; NA: Noradrenaline; OPN: ovarian plexus nerve; SMG: superior mesenteric ganglion. Hematoxylin-eosin. Scale bar: A, B, D, F, G and H: 250 µm; C and E: 100 µm; Inset: 12.5 µm.
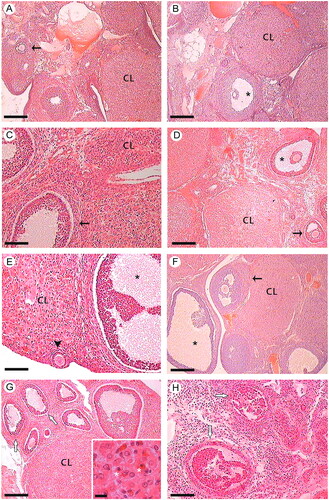
Discussion
In the present study, we investigate whether noradrenergic stimulation of the SMG can modify ovarian GnRH levels and cause morphofunctional changes in the gonad, via OPN, during estrus and diestrus stages. For this purpose, we used an ex vivo SMG-OPN-Ovary system since it avoids the influence of humoral factors that can interfere with neural action. Because NA is one of the main neurotransmitters released in the ovary via OPN, we also incubated denervated ovaries with and without the addition of this catecholamine. It is important to note that intraovarian neurons have not been shown to be present in the animals used here (D’Albora et al. Citation2000). In Holtzman rats, neurotransmitters present in the ovary would come from extrinsic innervation sources. Consequently, the two experimental schemes allowed us to make the necessary comparisons to better understand the role of NA on the parameters analyzed in the ovary.
During estrus, the stage related to the ovulatory process that begins the luteal phase (Ajayi and Akhigbe Citation2020), noradrenergic stimulation of SMG reduced the levels of GnRH in the ovary medium. This could be due to the influence of different neurotransmitters released in the ovary through OPN, such as neuropeptide Y (NPY), substance P, and even NA (Dees et al. Citation1986; Aguado Citation2002; Daneri et al. Citation2013). Indeed, the results obtained in the denervated ovary seem to support our assumption, because the inhibitory effect of NA on GnRH was significant at 60 min but could not be sustained, possibly due to the absence of other factors that reach the ovaries through the OPN. Thus, our ex vivo model highlights the importance of the sympathetic innervation of the ovary that involves OPN in the modulation of endogenous GnRH levels. GnRH can perform stimulatory or inhibitory effects on ovary, so its regulation is essential to favor the continuity of reproductive cycle.
It is interesting to note that, in both experimental schemes, NA caused a decrease in GnRH levels, accompanied by an increase in progesterone levels and a lower Akr1c18 mRNA expression. These results are consistent with previous reports indicating that GnRH or its analogs can act directly on the ovary inhibiting steroidogenesis (Bouchard et al. Citation1993; Yang et al. Citation2003), and that NA modulates the progesterone secretion in the gonad (Miszkiel and Kotwica Citation2001; Garraza et al. Citation2004; Itoh and Ishizuka Citation2005; Piccinato et al. Citation2012; Bronzi et al. Citation2015). NA can increase HSD3B activity and stimulate progesterone secretion by luteal beta-1 and beta-2 adrenergic receptors (Miszkiel and Kotwica Citation2001). With reference to the latter, NA can also stimulate progesterone production in granulosa cells through beta-2 adrenergic receptors; even the alpha adrenergic receptors present on these cells appear to modulate steroidogenesis (Piccinato et al. Citation2012). Corpora lutea remaining from prior estrous cycles and ovarian follicles, both potential sources of progesterone, are present in the estrus ovary and possess receptors for NA and GnRH. However, we did not observe changes in the Hsd3b3 mRNA expression and further studies are needed to clarify this. The high concentration of progesterone could also be due to the action of the steroid itself. Sugino et al. (Citation1997) reported that progesterone inhibits the expression of Akr1c18 in the rat corpus luteum, supporting our results. In addition, it cannot be ruled out that different neuronal signals can activate different receptors and, consequently, modulate the activity of the enzymes involved in ovarian steroidogenesis (Rosas et al. Citation2018).
In the denervated ovary, the stimulating effect of NA on progesterone may be due to its inhibitory action on GnRH in estrus. Furthermore, the lack of extrinsic innervation, a source of other factors that can inhibit progesterone such as NPY and substance P (Wuttke et al. Citation1989; Garraza et al. Citation2004), could also explain our results and the reason for the higher basal levels of progesterone.
In both experimental schemes, the administration of NA decreased the Bax/Bcl2 ratio in ovarian tissue and did not show changes in ovarian morphology or signs of cell death. It is known that progesterone can act as a survival factor in granulosa cells and luteal cells (Quirk et al. Citation2004; Liszewska et al. Citation2005; Regan et al. Citation2018), while GnRH has been attributed the opposite effect (Hong et al. Citation2008). Although several studies indicate that GnRH plays an important role in the apoptosis of ovarian granulosa cells during follicular atresia (Hong et al. Citation2008; Singh and Krishna Citation2010), it should be noted that NA was not analyzed. Our results here demonstrate that NA can promote the survival of major ovarian structures in the periovulatory period. In fact, using the same experimental model, it was recently shown that ex vivo stimulation of SMG with allopregnanolone decreases the concentration of NA in the ovarian incubation fluid and induces apoptosis in the gonad during the estrus stage (Cáceres et al. Citation2021). In accordance with Cáceres et al. (Citation2021), the maintenance of the SMG-OPN pathway is necessary for estrus ovarian regulation. Our results support this and become more relevant when considering that the SMG-OPN pathway is basically associated with the vasculature, which provides essential components to normal ovarian function. However, neural signaling (the main source of NA) must be adequate, since low or excessive levels of NA can trigger pathological processes.
Unlike estrus, diestrus II is the stage when luteal secretion of progesterone declines in non-fecund cycles in rats (Freeman Citation2006), and the drop-off in this steroid marks the onset of functional regression of the corpus luteum (Taketa Citation2022). The levels of ovarian GnRH in this case were higher than those reported in the estrus stage and even increased more in ovarian incubations without peripheral neural influence. These results demonstrate a modulatory role of extrinsic sympathetic pathway on decapeptide and agree with those reported by Schirman-Hildesheim et al. (Citation2005). These authors observed that the ovarian Gnrh mRNA expression increase during the diestrus II stage and reaches a maximum peak in the proestrus. Schirman-Hildesheim et al. (Citation2005) also reported that the expression of the GnRH receptor is lower in the estrus stage compared to the diestrus II stage, and that it shows an expression pattern somewhat similar to that of its ligand. In this sense, there is evidence showing the necessary involvement of GnRH in follicular atresia and luteal regression by directly stimulating apoptotic factors (Bauer-Dantoin and Jameson Citation1995; Scotti et al. Citation2011; Singh and Krishna Citation2010). In fact, in diestrus II, the Bax/Bcl2 ratio was higher in the denervated ovary-where higher GnRH levels prevailed- than in the ovary of the system. It is interesting to note that in estrus, in the ovarian tissue of the control groups, no significant changes were observed in the Bax/Bcl-2 ratio. Apparently, the diestrus ovary seems more sensitive to neuronal influence. Diestrus has even been reported to be the most sensitive stage of the estrous cycle to drugs that block neurotransmitters (Trujillo et al. Citation2015).
It should be noted that noradrenergic stimulation of the ganglion increased ovarian GnRH levels only at 60 min. This effect of NA on GnRH was more significant and was maintained throughout the incubation period when the adrenergic drug was added directly to the incubation medium of the ovaries. Our results support those reported by Herbison (Citation1997), who found an important role of NA in increasing the expression and secretion of the Gnrh mRNA necessary to drive follicle development. Therefore, we interpret that the tendency to normalization of GnRH levels in the ovary of the ex vivo system is due to the influence of this sympathetic pathway in an attempt to modulate the effects of noradrenergic stimulation in the gonad and, consequently, on cell death. Similarly, Bronzi et al. (Citation2015) demonstrated the importance of the celiac ganglion-SON sympathetic pathway to regulate cell death in the rat ovary, which seems to prevail when GnRH levels increase above basal levels during the diestrus II stage.
At late diestrus, we also demonstrated a decrease in progesterone levels due to the noradrenergic stimulation of the ganglion, consistent with an increase in the Akr1c18 mRNA expression. All of this is related to an increase in the Bax/Bcl2 ratio, follicles with early signs of atresia, and the presence of a corpus luteum with signs of cell death. This could be due to the action of NA released from the sympathetic nerves, which seems to support the luteal regression process once it has started (Bowolaksono Citation2012), allowing the continuity of the estrous cycle. Likewise, a potential role of GnRH in the observed changes in both progesterone levels and the Bax/Bcl-2 ratio cannot be excluded. The production of GnRH and expression of its receptors involves the ovarian structures that we find here affected, particularly in granulosa cells and granulosa-lutein cells (Schirman-Hildesheim et al. Citation2005; Choi et al. Citation2006; Shahed and Young Citation2011).
It is interesting to note that the direct addition of NA into the ovary compartment also increased GnRH levels and the Bax/Bcl2 ratio, although it stimulated the synthesis and release of progesterone. Other authors also obtained similar results when stimulating rat ovaries with NA (Garraza et al. Citation2004; Bronzi et al. Citation2015). Although there is evidence indicating that GnRH inhibits ovarian steroidogenesis (Bouchard et al. Citation1993; Yang et al. Citation2003), there are also studies that highlight a dual effect. Early research suggests that the stimulating effect of GnRH on ovarian steroidogenesis could be due to its direct action on steroidogenic enzymes or to an indirect action on gonadotropin release (Singh and Krishna Citation2010). In fact, in our experimental scheme, elevated progesterone levels are consistent with an increase in the Hsd3b3 mRNA expression and a decrease in the Akr1c18 mRNA expression. In addition, our research group has reported that the ovarian GnRH released during the diestrus II stage can induce LH release in pituitary incubations (Daneri et al. Citation2013). This is intriguing in light of the fact that the existence of a local, paracrine, GnRH – gonadotropic hormones axis in the gonads has been postulated (Schirman-Hildesheim et al. Citation2008). In this sense, it is possible that several tasks, such as fine control of follicular development and steroidogenesis, may be regulated by this local axis in anticipation of the preovulatory surge of pituitary gonadotropins. Our histological studies of NA-stimulated ovaries revealed signs consistent with a possible atretogenic effect, and different studies in mammals have shown that granulosa cells from the atretic follicles can produce progesterone when exposed to LH (Hubbard and Greenwald Citation1983; McNatty et al. Citation1985). Progesterone levels are known to decrease towards the end of diestrus II in a normal estrous cycle in the rat (Freeman Citation2006). Therefore, our study suggests that ovarian processes are orchestrated by the SMG-OPN pathway during diestrus II to give rise to the follicular phase.
In conclusion, our experimental results showed that noradrenergic stimulation of the SMG-OPN pathway regulates the cyclicity of the ovary. The SMG, through the OPN, modulates the cross-talk between NA and ovarian GnRH, protecting the ovary from atretogenic effects and luteal apoptosis during the estrus stage while inducing luteal regression in the diestrus II. Our results contribute to elucidate the mechanisms of action of NA in the mammalian ovary, and to the knowledge base necessary for a better understanding of ovarian pathologies where the participation of extrinsic innervation and GnRH has been demonstrated (Kang et al. Citation2003; Cole et al. Citation2015; Chaudhari et al. Citation2018; del Campo et al. Citation2019). Future studies are required to delve into the adrenergic receptors that mediate these effects.
Materials and methods
Animals
Virgin Holtzman strain adult female rats (Rattus norvegicus) weighing 250 ± 50 g were used in all the experiments (n = 6 animals per experimental group). Breeding colonies were established at the Animal Facility of the Universidad Nacional de San Luis (San Luis, Argentina) under rigorous light conditions (12 h light, 07:00 a.m.–07:00 p.m., and 12 h darkness), controlled temperature (22 ± 2 °C), with water and food ad libitum.
Surgical and experimental procedure
For this study, two experimental schemes were designed, in the estrus and diestrus II stages: the SMG-OPN-Ovary system and the ovary without peripheral neural influence. Both were removed by dissection between 3:00 p.m. and 4:00 p.m. Previously, the female rats were anesthetized with a combination of ketamine hydrochloride (80 mg/kg; Holliday Scott, Buenos Aires, Argentina) and xylazine (10 mg/kg; Richmond, Buenos Aires, Argentina) by intraperitoneal injection. In the first experimental design, the SMG-OPN-Ovary system was extracted in a single piece, taking into account earlier descriptions of the anatomical trajectory of this neural pathway as a guide (Vega Orozco et al. Citation2012; Cáceres et al. Citation2021). The system was immediately placed in a cuvette with two separate compartments, one for the SMG and one for the ovary. Both remained attached by OPN throughout the incubation period.
The incubation medium used was Krebs-Ringer Bicarbonate Buffer with 0.1 g/L of glucose and 0.1 g/L of albumin. Incubations were performed at 37 °C in an atmosphere of 95% O2 and 5% CO2. After a 15-min preincubation, the Krebs-Ringer buffer was changed in both compartments (3 ml final volume) and 0.1 mg/mL of ascorbic acid was added to the SMG incubation medium to provide protection against potential oxidative damage. One group received NA (Clorhidrate de L-D-Noradrenaline, Sigma-Aldrich, St. Louis, MO) at a final concentration of 10−6 M in the Krebs-Ringer buffer added to the SMG compartment. The other group was untreated and considered a control group.
In the second experimental scheme, the contralateral ovaries (right ovaries) were extracted, disconnecting them from all peripheral neural connections and incubated under the same conditions described above. In this case (denervated ovaries), the noradrenergic stimulus was added directly into the ovarian compartment.
All incubations were carried out for 120 min after changing the medium. At 60 and 120 min, aliquots of the ovarian incubation medium (250 µL) were removed and frozen until the measurement of GnRH and progesterone. Immediately after incubation, the ovaries were weighed and stored at − 80 °C until the analysis of mRNA expression of Hsd3b3 (the enzyme that catalyzes the progesterone synthesis), Akr1c18 (the enzyme that catalyzes the progesterone degradation), Bax (a pro-apoptotic regulator), and Bcl2 (an anti-apoptotic regulator). In addition, ovarian samples corresponding to both experimental schemes were fixed in Bouin’s solution for further analysis by routine histological technique.
GnRH and progesterone assay
GnRH and progesterone levels were measured in ovary incubation liquid by radioimmunoassay (RIA), as described by Bronzi et al. (Citation2015). In each case, appropriate corrections were made based on the volume of sample (incubation medium) extracted and remaining in the cuvette at each specific experimental time.
RNA isolation and reverse transcription-polymerase chain reaction (RT-PCR) analysis
Total RNA was isolated from ovarian tissue using TRIzol® reagent (Life Technologies, Thermo Fisher Scientific, Inc.), according to the manufacturer’s protocol. Two micrograms of high purity and integrity total RNA were reversely transcribed using random primers and M-MLV Reverse Transcriptase (Promega, Buenos Aires, Argentina) in a 26 µL reaction mixture. For amplification of the reverse transcription (RT) products, the reaction mixture consisted of 1× Green GoTaq reaction buffer, 0.2 mM deoxynucleoside triphosphates, 0.5 µM specific oligonucleotide primers and 1.25 U Go Taq DNA polymerase (Promega, Buenos Aires, Argentina) in a final volume of 50 µL. contains all required information about the primers used in this study.
Table 1. Primer gene symbols, GenBank access numbers, sequences, and sizes of amplicons.
The amplification of the cDNA was performed using a thermalcycler (My Cycler, BioRad, Buenos Aires, Argentina). Amplified products were analyzed on 2% agarose gels. Band intensities of RT-PCR products were quantified using ImageJ software (Image Processing and Analysis in Java from http://rsb.info.nih.gov/ij/). The ratio between the signal intensities of the target genes and the housekeeping gene (Actb) was used to express the relative levels of messenger RNA (mRNA).
Histological procedure
Fixed tissues were treated, stained with hematoxylin-eosin and evaluated according to Vega Orozco et al. (Citation2006).
Statistical analysis
Statistical analysis was performed using GraphPad Prism (Version 5, GraphPad Software Inc. San Diego, CA). Data are presented as mean ± standard error of the mean (SEM) in each group. For multiple comparisons made throughout the time of incubation, a repeated-measures Two-way ANOVA followed by Bonferroni test was used. In order to analyze the difference between the means of more than two groups, one-way ANOVA followed by Tukey’s test was used. A value of p < 0.05 was considered statistically significant.
Ethics approval
All animal care and experimental procedures were performed were performed according to the National Institutes of Health Guide for the Care and Use of Laboratory Animals (NIH Publications No. 8023, revised 1978) and comply with the ARRIVE guidelines 2.0. The experimental protocol was approved by the Comité Institucional de Cuidado y Uso de Animales de Experimentación (Acronym: CICUA) of the Universidad Nacional de San Luis, Argentina (Resol. No. 296-17).
Authors’ contributions
Conceived and designed the experiments: ASVO, MC; Performed the experiments: CDB, ASVO; Analyzed and interpreted the data: MBD, SV; Contributed reagents/materials: FHM, MF; Wrote the manuscript–original draft: MBD, SV; Final edit of paper: MBD, MC.
Abbreviations | ||
Actb | = | actin beta |
Akr1c18 | = | 20alpha-hydroxysteroid dehydrogenase |
GnRH | = | gonadotropin-releasing hormone |
Hsd3b3 | = | 3beta-hydroxysteroid dehydrogenase |
LC | = | luteal cells |
LH | = | luteinizing hormone |
NA | = | Noradrenaline |
OPN | = | ovarian plexus nerve |
SMG | = | superior mesenteric ganglion |
SON | = | superior ovarian nerve |
Acknowledgment
We also thank Dra. Zulema Sosa for her valuable comments and suggestions during the preparation of this manuscript.
Disclosure statement
No potential conflict of interest was reported by the author(s).
Data availability statement
The data that support the findings of this study are available from the corresponding author, MC, upon reasonable request.
Additional information
Funding
References
- Aguado LI. 2002. Role of the central and peripheral nervous system in the ovarian function. Microsc Res Tech. 59(6):462–473.
- Ajayi AF, Akhigbe RE. 2020. Staging of the estrous cycle and induction of estrus in experimental rodents: an update. Fertil Res Pract. 6:5.
- Bauer-Dantoin AC, Jameson JL. 1995. Gonadotropin-releasing hormone receptor messenger ribonucleic acid expression in the ovary during the rat estrous cycle. Endocrinology. 136(10):4432–4438.
- Bouchard P. Caraty A, Coelingh Bennink HJT, Pavlou SN, editors. 1993. GnRH, GnRH analogs, gonadotropins, and gonadal peptides. London: The Parthenon Publishing Group.
- Bowolaksono A. 2012. Molecular regulation of noradrenaline in bovine corpus luteum. HAYATI J. Biosci. 19(2):81–87.
- Bronzi CD, Vega Orozco AS, Rodriguez D, Rastrilla AM, Sosa ZY, Casais M. 2015. Noradrenaline modulates the presence of gonadotropin-releasing hormone in ovary. The importance of its interrelation on the ovarian steroidogenesis and apoptosis on dioestrus II in rat. J Steroid Biochem Mol Biol. 154:39–46.
- Cáceres ARR, Campo Verde Arboccó F, Cardone DA, Sanhueza MLÁ, Casais M, Vega Orozco AS, Laconi MR. 2021. Superior mesenteric ganglion neural modulation of ovarian angiogenesis, apoptosis and proliferation by the neuroactive steroid allopregnanolone. J Neuroendocrinol. 34:e13056.
- Casais M, Delgado SM, Sosa Z, Telleria CM, Rastrilla AM. 2006. The celiac ganglion modulates LH-induced inhibition of androstenedione release in late pregnant rat ovaries. Reprod Biol Endocrinol. 4:66.
- Chaudhari N, Dawalbhakta M, Nampoothiri L. 2018. GnRH dysregulation in polycystic ovarian syndrome (PCOS) is a manifestation of an altered neurotransmitter profile. Reprod Biol Endocrinol. 16(1):37.
- Choi JH, Gilks CB, Auersperg N, Leung PC. 2006. Immunolocalization of gonadotropin-releasing hormone (GnRH)-I, GnRH-II, and type I GnRH receptor during follicular development in the human ovary. J Clin Endocrinol Metab. 91(11):4562–4570.
- Cole SW, Nagaraja AS, Lutgendorf SK, Green PA, Sood AK. 2015. Sympathetic nervous system regulation of the tumour microenvironment. Nat Rev Cancer. 15(9):563–572.
- D'Albora H, Lombide P, Ojeda SR. 2000. Intrinsic neurons in the rat ovary: an immunohistochemical study. Cell Tissue Res. 300(1):47–56.
- Daneri C, Orozco AV, Bronzi D, Mohn C, Rastrilla AM, Sosa ZY. 2013. Involvement of the ganglion cholinergic receptors in gonadotropin-releasing hormone, catecholamines, and progesterone release in the rat ovary. Fertil Steril. 99(7):2062–2070.
- del Campo M, Piquer B, Witherington J, Sridhar A, Lara HE. 2019. Effect of superior ovarian nerve and plexus nerve sympathetic denervation on ovarian-derived infertility provoked by estradiol exposure to rats. Front Physiol. 10:349.
- Delgado SM, Escudero CG, Casais M, Gordillo M, Anzulovich AC, Sosa Z, Rastrilla AM. 2010. Ovaric physiology in the first oestral cycle: influence of noradrenergic and cholinergic neural stimuli from coeliac ganglion. Steroids. 75(10):685–694.
- Delsouc MB, Bronzi CD, Becerra CD, Bonaventura MM, Mohamed FH, Casais M. 2019. Ganglionic and ovarian action of acetylcholine during diestrous II in rats. Neuroendocrine control of the luteal regression. Steroids. 148:19–27.
- Dees WL, Ahmed CE, Ojeda SR. 1986. Substance P- and vasoactive intestinal peptide-containing fibers reach the ovary by independent routes. Endocrinology. 119(2):638–641.
- Domínguez R, Cruz-Morales SE. 2011. The ovarian innervation participates in the regulation of ovarian functions. Endocrinol Metab Syndr. S4:001.
- Elfvin LG, Lindh B, Hökfelt T. 1993. The chemical neuroanatomy of sympathetic ganglia. Annu Rev Neurosci. 16:471–507.
- Ernsberger U, Deller T, Rohrer H. 2020. The diversity of neuronal phenotypes in rodent and human autonomic ganglia. Cell Tissue Res. 382(2):201–231.
- Freeman ME. 2006. Neuroendocrine control of the ovarian cycle of the rat. In: Neill JD, editor. Knobil and Neill’s physiology of reproduction. San Diego (CA): Academic Press; p. 2327–2388.
- Garraza MH, Aguado LI, De Bortoli MA. 2004. In vitro effect of neuropeptides on ovary or celiac ganglion affects the release of progesterone from ovaries in the rat. Med Sci Monit. 10(12):BR440–BR446. PMID: 15567974
- Goto T, Endo T, Henmi H, Kitajima Y, Kiya T, Nishikawa A, Manase K, Sato H, Kudo R. 1999. Gonadotropin-releasing hormone agonist has the ability to induce increased matrix metalloproteinase (MMP)-2 and membrane type 1-MMP expression in corpora lutea, and structural luteolysis in rats. J Endocrinol. 161(3):393–402.
- Guerrero HE, Stein P, Asch RH, de Fried EP, Tesone M. 1993. Effect of a gonadotropin-releasing hormone agonist on luteinizing hormone receptors and steroidogenesis in ovarian cells. Fertil Steril. 59(4):803–808. PMID: 8458500
- Herbison AE. 1997. Noradrenergic regulation of cyclic GnRH secretion. Rev Reprod. 2(1):1–6.
- Hong IS, Cheung AP, Leung PC. 2008. Gonadotropin-releasing hormones I and II induce apoptosis in human granulosa cells. J Clin Endocrinol Metab. 93(8):3179–3185.
- Hubbard CJ, Greenwald GS. 1983. In vitro effects of luteinizing hormone on induced atretic graafian follicles in the hamster. Biol Reprod. 28(4):849–859.
- Irusta G, Parborell F, Tesone M. 2007. Inhibition of cytochrome P -450 C17 enzyme by a GnRH agonist in ovarian follicles from gonadotropin-stimulated rats. Am J Physiol Endocrinol Metab. 292(5):E1456–1464.
- Itoh MT, Ishizuka B. 2005. alpha1-Adrenergic receptor in rat ovary: presence and localization. Mol Cell Endocrinol. 240(1–2):58–63.
- Jørgensen JC, Kannisto P, Liedberg F, Ottesen B, Owman C, Schmidt G. 1991. The influence of neuropeptide Y and norepinephrine on ovulation in the rat ovary. Peptides. 12(5):975–982.
- Kang SK, Choi KC, Yang HS, Leung PC. 2003. Potential role of gonadotrophin-releasing hormone (GnRH)-I and GnRH-II in the ovary and ovarian cancer. Endocr Relat Cancer. 10(2):169–177.
- Linares R, Rosas G, Vieyra E, Ramírez DA, Velázquez DR, Espinoza JA, Morán C, Domínguez R, Morales-Ledesma L. 2019. In adult rats with polycystic ovarian syndrome, unilateral or bilateral vagotomy modifies the noradrenergic concentration in the ovaries and the celiac superior mesenteric ganglia in different ways. Front Physiol. 10:1309.
- Liszewska E, Rekawiecki R, Kotwica J. 2005. Effect of progesterone on the expression of bax and bcl-2 and on caspase activity in bovine luteal cells. Prostaglandins Other Lipid Mediat. 78(1-4):67–81.
- McNatty KP, Lun S, Heath DA, Kieboom LE, Henderson KM. 1985. Influence of follicular atresia on LH-induced cAMP and steroid synthesis by bovine thecae interna. Mol Cell Endocrinol. 39(3):209–215.
- Merz C, Saller S, Kunz L, Xu J, Yeoman RR, Ting AY, Lawson MS, Stouffer RL, Hennebold JD, Pau F, et al. 2015. Expression of the beta-2 adrenergic receptor (ADRB-2) in human and monkey ovarian follicles: a marker of growing follicles? J Ovarian Res. 8:8.
- Miszkiel G, Kotwica J. 2001. Mechanism of action of noradrenaline on secretion of progesterone and oxytocin by the bovine corpus luteum in vitro. Acta Vet Hung. 49(1):39–51.
- Orozco AV, Sosa Z, Fillipa V, Mohamed F, Rastrilla AM. 2006. The cholinergic influence on the mesenteric ganglion affects the liberation of ovarian steroids and nitric oxide in oestrus day rats: characterization of an ex vivo system. J Endocrinol. 191(3):587–598.
- Piccinato CA, Montrezor LH, Collares CA, Vireque AA, Rosa e Silva AA. 2012. Norepinephrine stimulates progesterone production in highly estrogenic bovine granulosa cells cultured under serum-free, chemically defined conditions. Reprod Biol Endocrinol. 10(1):95.
- Quirk SM, Cowan RG, Harman RM. 2004. Progesterone receptor and the cell cycle modulate apoptosis in granulosa cells. Endocrinology. 145(11):5033–5043.
- Regan SLP, Knight PG, Yovich JL, Leung Y, Arfuso F, Dharmarajan A. 2018. Granulosa cell apoptosis in the ovarian follicle—a changing view. Front Endocrinol (Lausanne). 9:61.
- Rosas G, Linares R, Ramírez DA, Vieyra E, Trujillo A, Domínguez R, Morales-Ledesma L. 2018. The neural signals of the superior ovarian nerve modulate in an asymmetric way the ovarian steroidogenic response to the vasoactive intestinal peptide. Front Physiol. 9:1142.
- Schirman-Hildesheim TD, Bar T, Ben-Aroya N, Koch Y. 2005. Differential gonadotropin-releasing hormone (GnRH) and GnRH receptor messenger ribonucleic acid expression patterns in different tissues of the female rat across the estrous cycle. Endocrinology. 146(8):3401–3408.
- Schirman-Hildesheim TD, Gershon E, Litichever N, Galiani D, Ben-Aroya N, Dekel N, Koch Y. 2008. Local production of the gonadotropic hormones in the rat ovary. Mol Cell Endocrinol. 282(1-2):32–38.
- Scotti L, Irusta G, Abramovich D, Tesone M, Parborell F. 2011. Administration of a gonadotropin-releasing hormone agonist affects corpus luteum vascular stability and development and induces luteal apoptosis in a rat model of ovarian hyperstimulation syndrome. Mol Cell Endocrinol. 335(2):116–125.
- Shahed A, Young KA. 2011. Intraovarian expression of GnRH-1 and gonadotropin mRNA and protein levels in Siberian hamsters during the estrus cycle and photoperiod induced regression/recrudescence. Gen Comp Endocrinol. 170(2):356–364.
- Singh P, Krishna A. 2010. Effects of GnRH agonist treatment on steroidogenesis and folliculogenesis in the ovary of cyclic mice. J Ovarian Res. 3(1):26.
- Sosa ZY, Casais M, Rastrilla AM, Aguado L. 2000. Adrenergic influences on coeliac ganglion affect the release of progesterone from cycling ovaries: characterisation of an in vitro system. J Endocrinol. 166(2):307–318.
- Sugino N, Telleria CM, Gibori G. 1997. Progesterone inhibits 20alpha-hydroxysteroid dehydrogenase expression in the rat corpus luteum through the glucocorticoid receptor. Endocrinology. 138(10):4497–4500.
- Taketa Y. 2022. Luteal toxicity evaluation in rats. J Toxicol Pathol. 35(1):7–17.
- Trujillo A, Morales L, Domínguez R. 2015. The effects of sensorial denervation on the ovarian function, by the local administration of capsaicin, depend on the day of the oestrous cycle when the treatment was performed. Endocrine. 48(1):321–328.
- Vega Orozco A, Bronzi C, Vallcaneras S, Sosa Z, Casais M. 2017. Neuromodulatory effect of oestradiol in the metabolism of ovarian progesterone and oestradiol during dioestrus II: participation of the superior mesenteric ganglion. Reprod Fertil Dev. 29(11):2175–2182.
- Vega Orozco A, Daneri C, Anesetti G, Cabrera R, Sosa Z, Rastrilla AM. 2012. Involvement of the oestrogenic receptors in superior mesenteric ganglion on the ovarian steroidogenesis in rat. Reproduction. 143(2):183–193.
- Vega Orozco A, Sosa Z, Delgado S, Casais M, Rastrilla AM. 2010. Involvement of ganglionic cholinergic receptors on the steroidogenesis in the luteal phase in rat. J. Steroid Biochem. Mol. Biol. 120(1):45–52.
- Wuttke W, Hallmann B, Pitzel L. 1989. Substance P and neuropeptide Y inhibit progesterone release from porcine granulosa and luteal cells. Eur. J. Endocrinol. 120(3_Suppl):S178.
- Yang H, Bhat GK, Wadley R, Wright KL, Chung BM, Whittaker JA, Dharmarajan AM, Sridaran R. 2003. Gonadotropin-releasing hormone-agonist inhibits synthesis of nitric oxide and steroidogenesis by luteal cells in the pregnant rat. Biol Reprod. 68(6):2222–2231.