Abstract
Anaerobic digestion is increasingly applied to stabilise sludge and to reduce operating costs of the wastewater treatment plant by generating biogas, considered as a sustainable energy source. The process suffers from many drawbacks such as slow and incomplete degradation rates, the result of the slow and rate‐limiting sludge hydrolysis step, due to the low biodegradability of the cell walls and the presence of extra‐cellular biopolymers.
Methods to enhance the biogas formation should hence make the substrate more accessible to the anaerobic micro‐organisms. Research on increasing the biogas production is extensive, with low‐frequency ultrasound treatment emerging as the technical and economic most appropriate technology. Although ultrasound is now widely applied in practice, underlying phenomena and optimum operating conditions are still open to debate. Laboratory ultrasound treatment was coupled with digestion experiments (at 37°C) using 12 parallel digesters, two of them being used as control digesters with untreated sludge.
The present paper demonstrates that ultrasound treatment can – within specific ranges of energy‐input – achieve (i) an increased disintegration of the sludge, as witnessed by an increasing soluble COD‐fraction accompanied by an increasing presence of BOD; (ii) an increased release of volatile fatty acids as a result of the oxidising radicals being formed through cavitation; and (iii) a slightly reduced dewaterability of the residual sludge, needing an increased dosage of poly‐electrolyte to obtain a high degree of cake dryness.
As pre‐treatment to digestion, ultrasound enhances the biogas production by more than 40% at low specific energy‐inputs (SE), and approximately 15% at higher SE‐values.
1. Introduction
Sludge disposal is a growing problem when treating wastewater, representing up to 50% of the current operation costs of a waste water treatment plant (WWTP) (Neyens et al. Citation2004). Municipal WWTPs generate sludge as a by‐product of the various treatment processes used, and current amounts, expressed as dry solids (DS) range from 60 to 90 grams DS per population equivalent (p.e.) and per day, i.e. nearly 10 million tons of dry sludge per year for the EU.
This sludge must undergo a treatment to alter its character and reduce the associated health problems and hindrance. This treatment aims to reduce the water content of the raw sludge, to transform the highly putrescible organic matter into a relatively stable or inert organic and inorganic residue, and to further condition this residue to meet disposal acceptance rules.
Sludge stabilisation is a common practice with anaerobic digestion as dominant process since both organic matter is transformed into net energy in the form of biogas (60 to 70 vol% methane), thereby also reducing the amount of final sludge to be disposed, and pathogens present in the sludge are significantly destroyed, thus limiting the hazards associated with the sludge.
Anaerobic digestion however suffers from some problems including poor organic solids (ODS) destruction, poor degradation of refractory materials leading to low biogas production, operational problems such as foaming mostly associated with the presence of filamentous micro‐organisms, and finally, problems during dewatering including higher polymer consumption to achieve a dewatering with high dry solids cake.
Although digestion is a combined result of successive hydrolysis, acidogenesis and methanogenesis, hydrolysis is generally accepted as being the rate limiting step in the anaerobic digestion process (Ghyoot and Verstraete Citation1997, Wang et al. Citation1999, Qasim Citation1999, Tiehm et al. Citation2001, Vavilin et al. Citation2002). Various pre‐treatments have been studied and include mechanical, thermal, chemical and biological interventions (Chiu et al. Citation1997, Kopp et al. Citation1997, Tiehm et al. Citation1997, Baier and Schmidheiny Citation1997, Clark and Nujjoo Citation1998, Müller et al. Citation1998, Quarmby et al. Citation1999, Barjenburch and Kopplow 2003, Neyens and Baeyens Citation2003, Climent et al. Citation2007, Dewil et al. Citation2007). All pre‐treatments result in a certain degree of lysis or disintegration of sludge cells, hereby releasing and solubilising intracellular material into the water phase and transforming refractory organic compounds into biodegradable material. Previous research on the ultrasound treatment of industrial wastewater (Dewil et al. Citation2006) has demonstrated that it causes sludge flocs to be disrupted, altering its constituent structure and solubilising organics. A flow chart of the overall sludge processing, positioning the pre‐treatment and digestion stages, is shown in Figure .
The effects of ultrasonic treatment are associated with a phenomenon called cavitation. It is the result of the formation and implosion of gas bubbles due to alternating expansion and compression cycles and causes locally extreme conditions of temperature and pressure (Laborde et al. Citation1998a, Citation1998b, Portenlanger Citation1999, Tiehm et al. Citation2001, Neis Citation2002). Cavitation is only encountered at frequencies below 1 MHz and the energy released by the imploding bubbles is inversely related to the ultrasound frequency (Young Citation1989, Tiehm et al. Citation2001). It was indeed shown that sludge disintegration is more pronounced at low frequencies (20 kHz) by Laborde et al. (Citation1998a).
Cavitation will result in the promotion of chemical reactions due to locally high temperature and pressure; in creating extreme shear forces in the liquid, thereby mechanically attacking components; in the formation of highly reactive radicals (H• and OH•) which can again facilitate chemical reactions to destroy organic contaminants. The reader is referred to more general literature for a detailed discussion of the cavitation phenomenon (Lauterborn and Ohl Citation1997, Neis and Tiehm Citation1999, Neis Citation2000, Citation2002).
Using ultrasound treatment on waste activated sludge (WAS) will result in the breakdown of organic material into smaller, more biodegradable fractions (Bougrier et al. Citation2006, Vera Citation2007).
The effect of intensity on enhanced biogas yield is not unanimously confirmed despite the enhanced biological hydrolysis, the preliminary step in the anaerobic treatment of WAS. Literature reports results of an increased biogas yield by as low as 10 to as high as 48%.
The dry solids content of the sludge is an important parameter for the disintegration with increased effectiveness at increased concentration of DS in the WAS, which is explained by the fact that more DS created more nuclei for cavitation and that more particles were exposed to the resulting shear forces (Nickel Citation1999, Tiehm et al. Citation2001, Onyeche et al. Citation2002, Dewil et al. Citation2006). This finding is at the basis of the introduction of the specific energy (SE), which is defined by Onyeche et al. (Citation2002) as:
Since the concentration of DS has an important effect on the results of ultrasound treatment, data are commonly presented in terms of SE, i.e. per kg DS. For anaerobic digestion, a definition on the basis of kJ/kg (ODS)o [(ODS)o being the initial ODS‐content, before digestion] seems more appropriate, since only ODS participates in the biogas synthesis reaction.
Moreover, some other effects on the sludge quality and properties are seen when treating it ultrasonically. The shear forces generated by bubble implosion destroy the extracellular polymeric substances (EPS) and the cell walls of the micro‐organisms in the sludge (Onyeche et al. Citation2002). Extracellular polymeric substances are highly hydrated polymeric molecules such as polysaccharides, lipids and proteins, formed by the micro‐organisms. They adsorb pollutants such as heavy metals and persistent organic molecules. Their high adsorption capacity binds a lot of water onto their structures (Neyens et al. Citation2004). The impact of ultrasound treatment on the dewaterability is difficult to predict since it is the effect of two counter‐active phenomena. The destruction of EPS is expected to lead to an improved dewaterability, whereas the supply of external energy to the sludge provokes a reduction of the average floc size (Dewil et al. Citation2006), resulting in clogging of the cake and a larger surface area on which water can adsorb.
A recent paper (Vera Citation2007) confirms that ultrasound treatment can completely suppress digestion foaming.
The formation of foam or a scum blanket can be responsible for a poor quality of the supernatant and restrained release of the formed biogas.
This paper addresses the use of ultrasonic pre‐treatment to enhance the anaerobic digestion of waste activated sludge (WAS) in order to define the optimum operating conditions, to quantify the different associated phenomena and to present an overall picture of occurring effects.
2. Experimental set‐up and procedures
2.1 Ultrasonic equipment
Waste activated sludge was pumped through a tubular ultrasound treatment reactor (Bandelin SONOREX TECHNNIK Sonobloc® SB 5.1‐1002). The energy‐input, expressed in kJ/kg DS or in kJ/kg (ODS)o, was determined by the applied power and the exposure time of the sludge together with the DS/ODS‐content of the sludge volume under scrutiny. A field of 25 kHz was used in accordance with literature recommendations, and the ultrasound treatment time was varied from 0 to 250 min by using recirculation of the WAS. The characteristics of the ultrasonic reactor are summarised in Table . The generator supplies alternating current to a piezo‐ceramic crystal, located at the extremity of the sonotrode and cooled (cold water) to avoid excess heating. The sonotrode is positioned eccentrically in the reactor to limit damp‐down effects. The frequency is set at 25 kHz. The amplitude and hence the intensity of the ultrasound waves can be varied. The maximal energy‐output is 1 kW. A maximum ultrasonic efficiency is obtained when steady waves with large pressure amplitude are generated. This is the case when the thickness of the water layer is a multiple half of the wavelength. The positioning of the disintegrator was adapted to attain this configuration. The maximum flow rate is 50 L min−1.
Table 1. Characteristics of the ultrasonic equipment.
2.2 Anaerobic digester set‐up
Twelve anaerobic digesters were built as parallel operating mesophilic single stage batch reactors, each of 1 L content and mechanically stirred. Specific attention was paid towards avoiding the scum layer by adapting the mixing rate. The digestion experiments were run for eight days.
A mixture of 300 g WAS and 400 g seed sludge was used. For each experimental run, two control digesters were operated with non‐ultrasound treated sludge. The ratio of 300 g WAS for 400 g seed sludge differs from the ratio of 1/4 to 8 used in practise. By choosing a higher fraction of WAS for the experiments, the effects of the ultrasonic treatment were more pronounced and became more noticeable: they would be masked if using a higher degree of (obviously untreated) seed sludge.
Only the 300 g of WAS were ultrasound treated at different energy input levels (as described above) prior to mixing with the seed sludge.
The produced gas was collected in calibrated glass cylinders. The cylinders were filled with acidified de‐ionised water to avoid losses of CO2 due to the formation of carbonates.
Moreover, the digesters were fitted with airtight septum sampling points for direct extraction of samples of the sludge mix at regular intervals for measurements of COD, VFA and biogas composition.
2.3 Analytical methods
The dry solids content (wt‐% DS) of the wastewater and of the sludge cake and filtrate after dewatering was determined as the residue after drying a sludge sample at 105°C to constant weight. Further heating at 605°C (to constant weight) drives off the organic dry solids (ODS), usually called ignitable solids, leaving the mineral dry solids content (MDS) as residual ash. These methods are described in Standard Methods (APHA Citation1992).
Prior to COD analysis, the sludge samples were centrifuged at 10,000 rpm for five minutes. The sludge supernatant was diluted 10 times and filtered through 1.3 µm glass microfibre filter paper (Merck Eurolab). In doing so, only the easily hydrolysable, soluble fraction of the organic material and hence the soluble COD (sCOD) can be determined. The sCOD was subsequently determined by a HACH® DR/890 Colorimeter using a HACH® COD reactor within a range of 0–1500 mg L−1.
Prior to volatile fatty acids (VFA) analysis, the sludge samples were acidified using H2SO4. A fixed amount of an internal standard was added to the acidified sample and mixed with di‐ethyl‐ether. This mixture was shaken for approximately two minutes. The supernatant ether‐phase was transferred to a volumetric flask using a Pasteur pipette. A 2‐µL volume of the extract was subsequently injected into the GC system. The concentrations of the individual VFA were analysed on a Chrompack CP‐9100 gas chromatograph equipped with a CP‐WAX 58 FFAP (Varian) column (length 25 m, diameter 0.25 mm) and a flame ionisation detector. The operational temperatures for the injection port, the oven and the detector were 240, 200 and 240°C, respectively. Helium was used as the carrier gas at a flow rate of 25 mL min−1.
The biogas composition was analysed on a Varian CP‐4900 Micro‐GC equipped with a Molecular Sieve 5A PLOT column (length 10 m, diameter 0.32 mm), a PoraPLOT Q column (length 10 m, diameter 0.15 mm) and a thermal conductivity detector. On the first column, hydrogen, nitrogen, methane and CO are analysed. The second column allows the analysis of CO2 and H2S. The operational temperatures for the injector of column 1, the injector of column 2, the oven of column 1, the oven of column 2 and the detector were 60, 50, 60, 50 and 60°C respectively. Helium was also used as the carrier gas.
2.4 Sludge characteristics
All sludge samples were obtained from the WWTP of Deurne‐Antwerp (B). This 300,000 p.e. WWTP is operated without primary settling and with a low‐load combined aerobic‐anoxic plug flow reactor. The WAS was obtained from the sludge thickeners. The digested (seed) sludge was sampled from the underflow of the digesters. The sludge characteristics are given in Table .
Table 2. Characteristics of thickened WAS, seed sludge and seeded mixture.
3. Results and discussion
3.1 Validation of the experiments
Prior to examining the effect of ultrasound treatment, the digester design and operation procedures were validated by repeating the experiments four times on untreated WAS collected at three subsequent days. Essential digestion parameters (DS‐content, ODS‐percentage, COD, biogas production) were measured.
The DS‐content of the seeded mixture was 48.34 g/kg of sludge with an organic fraction of 60.94%. The initial COD of the seeded mixture was 1523 mg L−1. The COD was reduced by digestion to 1356 mg L−1.
The biogas production was between 2460 and 2610 mL, i.e. within ±5% of the average production. The biogas production varied nearly proportionally with the initial amount of ODS [(ODS)0] present. The average values of the digestion results are given in Table , for kJ/kg DS equal to zero corresponding to no ultrasound treatment.
3.2 Effect of ultrasound treatment on the COD release
The destruction of the sludge flocs can be measured in terms of the range of the change of COD (ΔCOD) in the filtrate of the ultrasound treated WAS. ΔCOD was determined by measuring both the total and the soluble fraction of COD (sCOD) before and after the ultrasonic treatment. This effect on the COD can also be expressed in terms of a destruction‐ratio, defined on the basis of the total COD, as:
Figure illustrates both the change of COD in the filtrate and the destruction rate in terms of the specific energy (SE) applied. The DS‐concentration of the sludge was 48.34 g DS/kg. The power of the ultrasonic waves was set at 1000 W.
Figure 2 Total COD‐release of the filtrate and destruction yield as a function of the specific energy‐input.
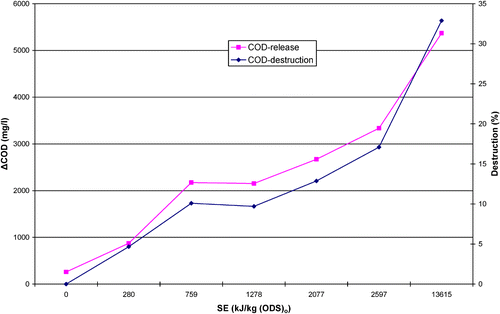
The destruction rate and hence the sCOD‐fraction increases significantly with increasing specific energy (and consequently with increased duration of the experiments). Destruction starts at very low SE‐values.
The released organics measured by the change in the sCOD of the filtrate are shown in Figure .
Figure 3 Effect of the dosage of ultrasound treatment on the sCOD and BOD of the filtrate at 20 kHz.
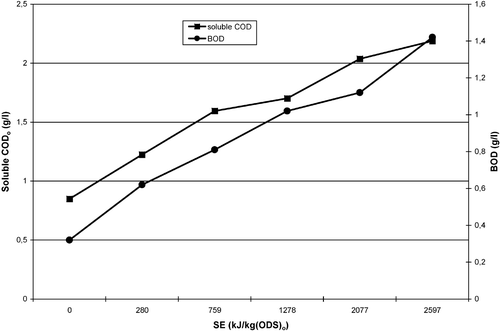
Both the soluble COD and BOD are seen to increase with increasing SE, implying that a considerable fraction of the released COD is transformed into biodegradable organic components, as witnessed by the increasing values of BOD.
3.3 Effect of ultrasound treatment on the biogas production
Although high inputs of ultrasonic energy are economically not justified as illustrated in the final section of this paper, a full range of specific energy inputs were examined, in line with common ranges previously applied in literature and to elucidate the very wide spread in biogas enhancements reported in literature.
Experiments were performed in triplicate, and average data of Table are further used. These averages were within 5% of the maxima and minima measured.
Ultrasound treatment results in a marked enhancement of the biogas production at low SE‐values with a gain of between approx. 20 and 45%, as illustrated in Figure . At moderately high values, the effect is less pronounced although still with an average 15% gain. Very high SE‐inputs do not significantly increase the biogas production. Clearly, the digestion is less efficient and despite a continued sludge disintegration with more COD released (Figure and Table ): the released COD is not transformed in extra biogas. In parallel to the COD‐effect, the rate of biogas formation can also be coupled to the VFA release, as will be discussed below: here too, high SE‐values do not further promote VFA release.
These observations are tentatively explained by the fact that an increasing specific energy input solubilises more robust and non‐degradable organics. These fragments formed and present in the solution, are not necessarily bio‐degradable and hence not suitable as substrate for the anaerobic micro‐organisms. Moreover, an increase in SE will induce the release of heavy metals and other inhibiting compounds, which were originally adsorbed on the EPS in the sludge, hence slowing down the digestion process. Obviously more research is needed to confirm these hypotheses and will be the subject of some follow‐up papers.
It is also noteworthy that the decrease in ODS is limited to some 15%. The differences in biogas yields can hence only be explained by the VFA release, and subsequent methanogenesis.
Table 3. Experimental data obtained at different US energy‐inputs, expressed in kJ/kg (ODS)o, for t = 8 days (0 kJ/kg DS corresponds to the control digesters).
3.4 Release of volatile fatty acids
To discuss the release of VFA, a clear distinction must be made between the effects of ultrasound treatment, and the effects of digestion. During digestion, VFA are formed and consumed in the hydrolysis and/or acidogenetic digestion phase. It is therefore difficult to determine the exact VFA concentration during digestion, since the formation rate is expected to be equal (or proportional) to the further disappearance during methanogenesis (Appels et al. 2008). During digestion, organic substance degraded by micro‐organisms is indeed the only source of organic carbon, the basic source of easily assimilated organic carbon is made by short‐chain carboxylic acids. According to McCarthy and Mosey (Citation1991), 72% of the organic substance defined as COD is disintegrated to acetic acid, from which, due to carboxylation, methane and CO2 are formed, which in turn are the main components of biogas being the products of methanogenesis.
The results of the VFA concentration after ultrasound treatment (VFAo) and after eight days of digestion (VFAt) are shown in Table and Figure , where ΔODS‐values are taken from Table .
Table 4. VFA.
From these results, it is clear that VFA are increasingly formed during ultrasound treatment, in‐line with the expected radical reactions (H• and OH•) of cavitation, up to an energy input of about 1250 kJ/kg DS or 2100 kJ/kg (ODS)o: organic compounds of the WAS are disrupted and oxidised to their carboxylic acid form.
The reason why this transformation stops at higher SE‐values has yet to be established, although it is expected that higher energy‐inputs will lead to the disruption of the cells into larger building molecules, less prone to oxidation to VFA, although the soluble COD continues to increase.
The initial significant release of VFA at low SE also explains the higher biogas synthesis (through acidogenesis) at lower SE‐values. The available VFA (up to five times the value of the non‐treated WAS) increase the biogas production.
Since the biological hydrolysis is the rate limiting step in a conventional anaerobic treatment, it is seen that the ultrasound release of VFA has a significant influence on the biogas yield.
3.5 Dewaterability
It was previously demonstrated (Dewil et al. Citation2006) that ultrasound treatment reduced the floc size from an average (50%) size of 15 µm for untreated sludge to about 3 µm for a sludge submitted to an ultrasound intensity of 750 kJ/kg DS, thereby increasing the capillary suction time (Vesilind and Davis Citation1988) from 12.5 to 20 s respectively.
The DS content of the cake was reduced from 25.4 to 24.3% DS. The effect of the ultrasound‐induced disintegration of WAS flocs is photographically illustrated in Vera (Citation2007).
The modified sludge structure after ultrasound treatment is also seen through differential scanning calorimetry (DSC Q10 with refrigerated cooling system, at 50 mL min−1 N2 flow and 10 K min−1). Figures show the heat flow associated with the heating of Buchner‐dewatered sludge at 10 K min−1, expressed in W g−1, for both untreated (a) and ultrasound treated (b) WAS.
The differences are mainly related to the temperature associated with the water evaporation, respectively 104.45°C for the untreated and within a range of 40 to 101.17°C for the ultrasound treated sample. The heat flow of the ultrasound treated sample (in W g−1 or J s−1 g−1) should be integrated over the time period of the heat flow (s) which is possible since over the experimental temperature range, the temperature increased by 10°C min−1 (or 0.17°C s−1). For the untreated sludge, the total heat absorbed is ∼1540 J g−1 against 1460 J g−1 for the ultrasound treated sample.
Clearly, both sludges contain about the same amount of water, but the onset of evaporation in ultrasound treated sludge is situated at lower temperatures, confirming the more open structure of the ultrasound flocs with more loosely bound water.
4. Economic assessment of the ultrasound treatment
The economy of the ultrasound can be assessed on the basis of the extra amounts of biogas formed, when compared to the energy‐input needed. Only operations at low energy‐input seem viable: if biogas at 65% methane has an average calorific value of 22,500 kJ Nm−3, the amount of biogas formed can be compared on the basis of the kJ‐input.
Anaerobic digestion itself, with a biogas production of approximately 0.4 Nm3/kg ODS‐removed, has an economic benefit, despite the higher dosage of PE to be applied for dewatering. The additional amount of biogas formed when applying 168 kJ/kg DS, or approximately 280 kJ/kg (ODS)o, accounts to ∼0.18 Nm3/kg ODS removed or an extra 1050 kJ/kg (ODS)o thus providing a net gain of 770 kJ/kg (ODS)o. Beyond 280 kJ/kg (ODS)o, the extra amount of biogas formed is ∼20% only.
The range of economic ultrasound‐application is hence certainly restricted to lower energy‐inputs, certainly not exceeding ∼4000 kJ/kg (ODS)o or 2400 kJ/kg DS. The economic evaluation hereafter summarises the economic benefits.
One should however not forget that WAS dewaterability becomes more difficult due to sludge floc size reduction, needing an extra dosage of about 20% poly‐electrolyte to achieve the same degree of dewatering as obtained for non‐ultrasound treated sludge (Dewil et al. Citation2006).
Table 5. Economic evaluation for a sludge treatment of 20 m3 h−1, containing 5 wt‐% of DS (i.e. 1000 kg DS/h) and 60% ODS, i.e. 600 kg/h of ODS (i.e. ∼10,000 p.e.), with a 15% ODS removal through digestion.
Considering the investment cost of an ultrasound treatment, with a maximum rating of 20 kW, to be around €40,000, capable of treating sludge from a WWTP of 10,000 p.e., the benefit yields a payback period of less then two years since the biogas generated will in some countries, like Belgium, benefit from green‐power certificates. These findings are confirmed by industrial applications. Ultrasound treatment units are modular and available in a wide range of capacities (between 1 and 20 kW). Capital costs today are roughly €20,000/kW, with 1 kW capable of treating sludge from a WWTP of 10,000 p.e.
Operation and maintenance costs are minimal although the ultrasound probes need replacement every one and a half to two years.
The use of ultrasound enhancement has been tested in several WWTPs, ranging from 50,000 to 750,000 p.e. (for a total of several Mp.e.). Improved ODS destruction was reported at ∼30%, enhancing the biogas production by ∼30%. Improvements were also found in the dewatering plants, where cake dryness increased by 5% in spite of using 33% less polymer. Since the degradation rate is accelerated, the dimensions of the disgesters can moreover be reduced for a given load, thus reducing the impact of high capital requirements.
This has been applied in Bamberg (Germany, 250,000 p.e.), (Ultrawaves, Citation2008) where the application of ultrasound avoided the construction of a third 3000 m3 digester.
As a result of the present investigations, some of these statements, i.e. better dewaterability at lower PE dosage, should be questioned.
5. Conclusions
The present paper demonstrated that ultrasound treatment can at low specific energy‐input achieve (i) an increased disintegration of the sludge, as witnessed by an increasing soluble COD‐fraction accompanied by an increasing presence of BOD; (ii) an increased release of volatile fatty acids (VFA) as a result of the oxidising radicals being formed through cavitation; and (iii) an increased biogas production of up to 40% at low SE‐values, and about 15% at moderate SE. High SE‐values do not promote digestion.
The economics of the treatment are very good.
The improved dewaterability at lower addition of poly‐electrolyte, as often reported in literature is not confirmed.
References
- APHA . 1992 . Standard methods for the examination of water and wastewater , Washington DC : American Public Health Association . 18th ed
- Baier , U. and Schmidheiny , P. 1997 . Enhanced anaerobic degradation of mechanically disintegrated sludge. . Water Science and Technology , 36 (11) : 137 – 143 .
- Bougrier , C. , Albasi , C. , Delgenes , J. P. and Carrere , H. 2006 . Effect of ultrasonic, thermal and ozone pre‐treatments on waste activated sludge solubilisation and anaerobic biodegradability. . Chemical Engineering and Processing , 45 (8) : 711 – 718 .
- Chiu , Y.‐C. , Chang , C.‐N. , Lin , J.‐G. and Huang , S.‐J. 1997 . Alkaline and ultrasonic pretreatment of sludge before anaerobic digestion. . Water Science and Technology , 36 (11) : 155 – 162 .
- Clark , P. P. and Nujjoo , I. 1998 . Ultrasonic sludge pre‐treatment for enhanced sludge digestion. . Proceedings of Innovation 2000 Conference , Cambridge
- Climent , M. , Ferrer , I. , del Mar Baeza , M. , Artola , A. , Vázquez , F. and Font , X. 2007 . Effects of thermal and mechanical pretreatments of secondary sludge on biogas production under thermophilic conditions. . Chemical Engineering Journal , 133 (1–3) : 335 – 342 .
- Dewil , R. , Baeyens , J. and Goutvrind , R. 2006 . Ultrasonic treatment of waste activated sludge. . Environmental Progress , 25 (2) : 121 – 128 .
- Dewil , R. , Appels , L. , Baeyens , J. and Degrève , J. 2007 . Peroxidation enhances the biogas production in the anaerobic digestion of biosolids. . Journal of Hazardous Materials , 146 (3) : 577 – 581 .
- Dewil , R. , Baeyens , J. and Goutvrind , R. 2006 . The use of ultrasonics in the treatment of waste activated sludge. . Chinese Journal of Chemical Engineering , 14 (1) : 105 – 113 .
- Ghyoot , W. and Verstraete , W. 1997 . Anaerobic digestion of primary sludge from chemical pre‐precipitation. . Water Science and Technology , 36 (6–7) : 357 – 365 .
- Kopp , J. , Muller , J. , Dichtl , N. and Schwedes , J. 1997 . Anaerobic digestion and dewatering characteristics of mechanically disintegrated excess sludge. . Water Science and Technology , 36 (11) : 129 – 136 .
- Laborde , J. L. , Bouyer , C. , Caltagirone , J. P. and Gérard , A. 1998a . Acoustic bubble cavitation at low frequencies. . Ultrasonics , 36 (1–5) : 589 – 594 .
- Laborde , J. L. , Bouyer , C. , Caltagirone , J. P. and Gérard , A. 1998b . Acoustic cavitation field prediction at low and high frequency ultrasounds. . Ultrasonics , 36 (1–5) : 581 – 587 .
- Lauterborn , W. and Ohl , C. D. 1997 . Cavitation bubble dynamics. . Ultrasonics Sonochemistry , 4 (2) : 65 – 75 .
- McCarthy , P. L. and Mosey , F. E. 1991 . Modelling of anaerobic digestion processes. . Water Science and Technology , 24 : 17 – 33 .
- Müller , J. , Lehne , G. , Schwedes , J. , Battenberg , S. , Näveke , R. , Kopp , J. , Dichtl , N. , Scheminski , A. , Krull , R. and Hempel , D. C. 1998 . Disintegration of sewage sludges and influence on anaerobic digestion. . Water Science and Technology , 38 (8–9) : 425 – 433 .
- Neis , U. and Tiehm , A. 1999 . Ultrasound in wastewater and sludge treatment Reports on Sanitary Engineering No.25, Technical University Hamburg‐Harburg
- Neis , U. 2000 . Ultrasound in water, wastewater and sludge treatment. . Water , 21 : 36 – 39 .
- Neis , U. 2002 . Intensification of biological and chemical processes by ultrasound Reports on Sanitary Engineering No.35, Technical University Hamburg‐Harburg
- Neyens , E. and Baeyens , J. 2003 . A review of thermal sludge pre‐treatment processes to improve dewaterability. . Journal of Hazardous Materials , 98 (1–3) : 51 – 67 .
- Neyens , E. , Baeyens , J. , Dewil , R. and de heyder , B. 2004 . Advanced sludge treatment affects extracellular polymeric substances to improve activated sludge dewatering. . Journal of Hazardous Materials , 106 (2–3) : 83 – 92 .
- Nickel , K. 1999 . Improving anaerobic degradation by ultrasonic disintegration of sewage sludge Reports on Sanitary Engineering No.25, Technical University Hamburg‐Harburg
- Onyeche , T. I. , Schläfer , O. , Bormann , H. , Schröder , C. and Sievers , M. 2002 . Ultrasonic cell disruption of stabilized sludge with subsequent anaerobic digestion. . Ultrasonics , 40 (1–8) : 31 – 35 .
- Portenlanger , G. 1999 . “ Mechanical and radical effects of ultrasound. ” . In Ultrasound in environmental engineering Edited by: Tiehm , A and Neis , U . Reports on Sanitary Engineering No.25, Technical University Hamburg‐Harburg
- Qasim , S. R. 1999 . Wastewater treatment plants: planning, design and operation , Boca Raton : CRC Press . 2nd ed
- Quarmby , J. , Scott , J. R. , Mason , A. K. , Davies , G. and Parsons , S. A. 1999 . The application of ultrasound as pre‐treatment for anaerobic digestion. . Environmental Technology , 20 (11) : 1155 – 1161 .
- Tiehm , A. , Nickel , K. and Neis , U. 1997 . The use of ultrasound to accelerate the anaerobic digestion of sewage sludge. . Water Science and Technology , 36 (11) : 121 – 128 .
- Tiehm , A. , Nickel , K. , Zellhorn , M. and Neis , U. 2001 . Ultrasonic waste activated sludge disintegration for improving anaerobic stabilisation. . Water Research , 35 (8) : 2003 – 2009 .
- Ultrawaves. 2008. Ultrasound sludge disintegration for anaerobic digestion acceleration and enhancement. Bamberg WWTP, Germany. Available from: www.ultrawaves.de/downloads/case‐studie‐bamberg.pdf (www.ultrawaves.de/downloads/case-studie-bamberg.pdf) [Accessed15 February 2008]
- Vavilin , V. A. , Rytov , S. V. , Lokshina , L. Y. , Pavlostathis , S. G. and Barlaz , M. A. 2002 . Distributed model of solid waste digestion‐effects of leachate recirculation and pH adjustment. . Biotechnology and Bioengineering , 81 (1) : 66 – 73 .
- Vera , M. A. 2007 . Improved anaerobic digestion with ultrasound disintegration. Proceedings of Hawaii Water Environment Association, Oahu [CD‐ROM], Pre‐Conference Session 6 February 2007, session 2_145
- Vesilind , P. A. and Davis , H. A. 1988 . Using the CST device for characterising sludge dewaterability. . Water Science and Technology , 20 : 203 – 205 .
- Wang , Q. , Kuninobu , M. , Kamimoto , K. , Ogawa , H. I. and Kato , Y. 1999 . Upgrading of anaerobic digestion of waste activated sludge by ultrasonic pre‐treatment. . Bioresource Technology , 68 (3) : 309 – 313 .
- Young , F. R. 1989 . Cavitation , London : McGraw‐Hill .