Abstract
Among the separation techniques used in industry, the triboelectric separation of insulating particles using rotary tube is an efficient technology employed in waste recovery and mineral industries. This process, also called free-fall triboelectric separation, is widely used for the sorting and the purification of granular materials resulting from industrial plastic wastes. This paper aims to achieve a comprehensive description of the laboratory triboelectric separator built up by the authors, and its utilisation for an experimental study carried out on granular samples containing particles of polyvinyl chloride and polyethylene. Thus, among the variable factors of the process, we analysed the influence of the most important ones, i.e. the rotational speed of the cylinder n (rpm), the applied high voltage U (kV), the charging time of the particles t (s), the mass of the sample m (g) and the composition percentage of the sample C p (%). Furthermore, the output process was optimised using the methodology of experimental designs. The obtained results are very instructive and confirmed the efficiency of this separation method for the processing of industrial plastic wastes.
1 State of the art
There are three electrostatic separation processes of granular mixtures that are widely employed for the recycling of industrial wastes (Lawver and Dyrenforth Citation1973, Dascalescu et al. Citation1998, Inculet et al. Citation1998, Iuga et al. Citation2001) and the beneficiation of mineral products (Ralston Citation1961, Higashiyama and Asano Citation1998, Haga Citation1995, Dance et al. Citation1991).
1.1 Roll-type corona-electrostatic separator
The roll-type corona-electrostatic separator is the appropriate solution for sorting granular mixtures containing plastic and metal particles as copper/plastic granules (Tilmatine et al. Citation2003, Citation2004, Citation2009). Figure shows the various elements of this type of separator. Electric charges are created by the air ionisation due to the corona discharge generated by an electrode with emitting tips (1) called ‘corona electrode’, connected to a high-voltage direct-current supply. Metal/plastic particles are deposited on the rotating cylinder (2) by the vibratory feeder (3) and acquire an electric charge with the same polarity as the potential of the high voltage. The particles behave differently according to whether they are electrically, conducting or insulating. Due to the image electric force, insulating granules remain attached to the rotating roll and they adhere to its surface. They fall down into the collector (4) when the gravitational force becomes greater than the image electric force. In addition, a brush (5) is used to detach mechanically the remaining granules from the surface of the cylinder. Conducting particles quickly lose the charge, acquired by ion bombardment, through the grounded cylinder. When reaching the zone of the electrostatic field generated by the high-voltage electrode of elliptic shape (6), called ‘static electrode’, they acquire by electrostatic induction a charge of opposed polarity. They are then attracted and collected at the right side of the collector.
Figure 1 Descriptive schematic of the role-type electrostatic separator. (1) Corona electrode connected to a DC high voltage supply; (2) grounded cylindrical rotating electrode; (3) electromagnetic vibratory feeder; (4) collecting hoppers; (5) brush and (6) static electrode connected to the same high voltage supply.
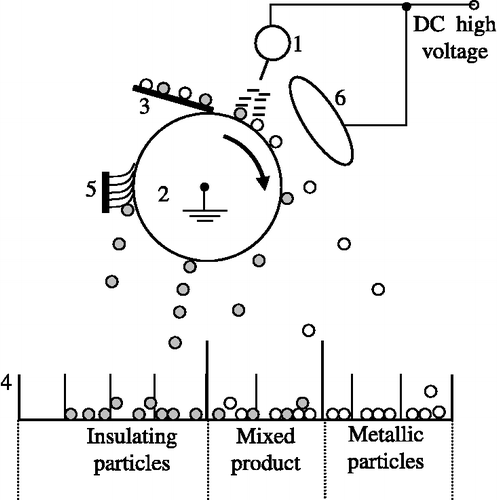
1.2 Plate-type electrostatic separator
The plate-type electrostatic separator is used when sorting mixtures of metal particles, like aluminium/copper particles (Das et al. Citation2007). The granules (more or less good conductors) are deposited by a vibratory electromagnetic feeder on the grounded plate electrode (Figure ). The particles then ‘slip’ on the surface of the plate and behave differently according to their electrical conductivity. The granules with good conductivity, reaching the zone of the electric field generated by the high voltage static electrode of elliptic shape, are subject to an attractive electric force exerted by this electrode and thus are collected in the appropriate compartment. The granules with poor conductivity do not acquire a sufficiently large charge. They are, however, not attracted by the static electrode and thus drop under the action of their mass and their speed on the left side of the collector.
1.3 Triboelectric free-fall separator
The triboelectric free-fall separator is used for separating insulating particles resulting from two or more different materials (Inculet and Castle Citation1991, Inculet et al. Citation1994, Finseth and Gerstler Citation1994, Soong et al. Citation1998). A schematic representation of the process is shown in Figure . The granular mixture to be separated is introduced in a tribocharging device. The granules of one type of material get a positive charge, while the others acquire a negative charge. Therefore, they can be separated while falling freely in a horizontal electric field, created between two vertical plate electrodes, which are connected to two DC high voltage power supplies of opposite polarities.
The separated products are recovered in a collector constituted of three compartments, two compartments for the pure products and one compartment for the mixed product.
The three processes are nowadays a subject of further research, especially for their optimisation using different mathematical methods (Mihailescu et al. Citation2002, Dascalescu et al. Citation2005, Medles et al. Citation2007a, Citation2007b, Younes et al. Citation2007, Citation2008).
Many tribocharging devices were employed for charging the particles and a lot of patents were proposed for this purpose. Early triboelectric separators for coal and mineral beneficiation usually had particles sliding down, or pneumatically transported through chutes, pipes or nozzles. Up to the present, this classic type of particle tribocharging systems has still been the subject of study in the field of triboelectrostatic separation. In the charging process, the fine coal powder was pneumatically conveyed to make an impact on copper walls and blades, creating a turbulent condition and causing the selective charging of the particles. Cyclone and fluidised bed have also been the techniques of attention for particle charging, since these techniques provide better particle-wall and particle–particle contacts.
Evidently, the tribochargers mentioned above are pneumatically charging devices with rarely mechanical parts involved. If the number of contacts made is multiplied, the quantity of charge transferred should increase; this gives rise to frictional charging or rubbing. Let us note that we recommend the rotating tribocharging device for separation of plastic particles because it processes great quantities of material.
2 Acquisition of electric charge by triboelectric effect
Triboelectric charging occurs when any two different surfaces contact one another. The resultant static charge is not usually noticeable unless it occurs in a system where the conductivity of the material surface is low, allowing the charge to build up to high levels. This static build-up, which is a significant burden in the handling of large amounts of fine dry powders such as flour or powdered chemicals and polymers, can be effectively employed for sorting differently-charged particles in an electric field (Dascalescu et al. Citation2005).
Thus, when two kinds of materials are rubbed, one acquires a positive electric charge and the other will be negatively charged. This phenomenon of electron transfer is still now not totally explained, and is called ‘triboelectricity’. Therefore, the separation is produced by forces acting on charged particles passing through an intense electrostatic field.
When the particles are introduced into the rotating cylinder, they undergo two types of collisions: particle/particle and particle/cylinder wall collisions (Figure ).
Triboelectric series is a classification of substances such that, when two of these substances are rubbed, the first one in the ranking will be positively charged while the other will be negatively charged. Figure shows a triboelectric series of some polymers. For example, if polyvinyl chloride (PVC) is rubbed with Teflon, the latter gets a negative charge and the PVC acquires a positive charge. However, if the PVC is rubbed with the PolyEthylene Terephthalate (PET), this one will be positively charged while the PVC will be negatively charged: the polarity of the charge depends on the position of the material in the triboelectric series.
3 Application to industrial plastic wastes
The triboelectric separation process of plastic particles is a multifactorial process depending on several parameters, each of them having one or more factors to be controlled:
-
Rotating tube: material of the tube, rotation speed, diameter and inclination.
-
High voltage: level.
-
Electrodes: dimensions, position and slope.
-
Time: charging time of the particles.
-
Mass: masses of the two materials and percentage composition.
The granular material to be separated is processed in a custom-designed tribocharging device (Figure ) consisting of a 10 cm diameter, 120 cm long PVC cylinder, inclined at 10° to the horizontal and rotating at a variable speed n = 0,…,550 rpm. The charged granules were then separated in the electrical field generated by two vertical plate electrodes (dimensions: 30 × 65 cm) spaced at 20 cm and inclined at 30°, and collected in three compartments: two for the ‘pure’ products and one for the ‘middling’.
The experimental study presented in this paper was carried out with a granular sample of total mass 200 g, containing particles of PVC and polyethylene (PE). Average size of the particles lies between 2 and 4 mm (Figure ). The tests were carried out in stable conditions of ambient temperature and humidity (T = 20–25°C and RH = 40–50%).
Four parameters were analysed in this section:
-
rotation speed of the tube n (rpm);
-
high voltage level U (kV);
-
charging time t (s) and
-
composition percentage of the PVC C p (%)*.
A set of four ‘one-factor-at-a-time’ experiments have been carried out, by varying one factor and keeping the others constant:
-
Experiment 1: variable high voltage level from 10 to 50 kV while the other factors are constant (n = 300 rpm, t = 60 s and C p = 50/50%).
-
Experiment 2: variable rotation speed from 100 to 550 rpm while other factors are constant (U = 50 kV, t = 60 s and C p = 50/50%).
-
Experiment 3: variable charging time from 10 to 300 s while other factors are constant (n = 300 rpm, U = 50 kV and C p = 50/50%).
-
Experiment 4: variable composition percentage C p of PVC from 20 to 80%, while other factors are constant (n = 300 rpm, U = 50 kV and t = 60 s).
4 Obtained results and discussion
After each experience, the collected products in each compartment are measured using a digital balance (precision = 0.01 g). The performance of the experimental device is estimated using three criteria:
-
Purity:
-
Recovery:
-
Amount of middling:
The recovery of PVC and its purity were considered as significant for the evaluation of the process and are represented as functions of the four factors in Figures .
Figure 11 Purity and recovery of PVC as a function of composition percentage of PVC C p (experiment 4).
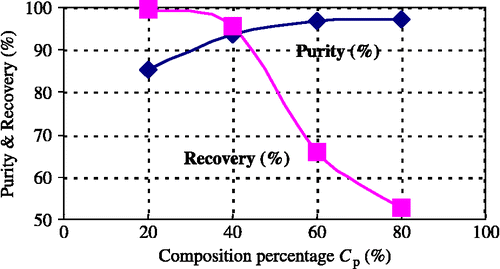
Experimental results show clearly the influence of the high-voltage level U (Figure ). We note that the purity of the separated products becomes higher than 90% when the applied voltage is greater than approximately 40 kV. Nevertheless, it is recommended to apply the highest voltage possible to ensure the best conditions for the separation process. The separation process is more efficient when the voltage is greater, because the electrostatic field generated between the electrodes is intense. However sparks occur between the electrodes for great values of voltage, when U is greater than 50 kV, this limits the level of the applied voltage. Furthermore, in all the experiments, except in experiment 1 for which the applied voltage was varied, we use U = 50 kV corresponding to an electrical field of 250 kV/m.
The obtained results, concerning the influence of the rotational speed of the cylinder, illustrate that this parameter also has a considerable effect on the separator functioning (Figure ). Values of the Purity and Recovery become high at about 280 rpm. The efficiency of the process decreases at higher values of n, due to the centrifugal force proportional to n 2. Indeed, the granules ‘stick’ on the cylinder wall when the tube is running at high speed. The particles do not undergo more collisions, and therefore do not acquire sufficient electric charge to be separated by the electrostatic field. Thus, in the case of our experimental device, the speed should not be too high and not exceed 280 rpm.
The efficiency of the separator also depends on the charging time of the materials being processed. Obtained results illustrated in Figure show that best separation is achieved when the time spent in the tube reaches 60 s. Therefore, the particles are highly and uniformly charged and could be separated when falling in the intense horizontal electric field. Notice that if the particles remain longer inside the tube, they lose a quantity of their charge by neutralisation, and thus the process efficiency decreases. Therefore, an appropriate charging time enables the particles to acquire an optimal electric charge equal to its saturation value.
Moreover, the results of Figure demonstrate that the ratio between the masses, i.e. the composition percentage of the granular mixture, greatly affects the separation process. The best performance for PVC recovery is obtained for a composition C p (M PVC/M PE) = (40/60)%. Thus, the composition of the waste material is important and influences the outcome of the process. Each type of granular composition has its specific characteristics, we cannot predict before experimentation at which composition ratio the best performance will be obtained, because this factor is an uncontrollable parameter.
5 Optimisation of the process
The difficulty of the problem resides in the fact that triboelectrostatic separation is a multifactorial process. In a free-fall triboelectrostatic separator, for instance, the list of factors influencing the outcome of the process includes the composition of the granular mixture, the feed rate, the granule size, the charging time of the particles, the electrode configuration, the applied high voltage, and many others. The question to be answered is: how can a process engineer find the set of values of the above-listed factors for which the outcome of the separation is optimal?
5.1 Design of experiments (DOE) methodology
DOE is a mathematical methodology based on the statistical analysis of experimental data (Wu and Moore Citation1986, Peace Citation1992, Frigon and Mathews Citation1996, Hicks and Turner Citation1999, Roy Citation2001).
The optimisation of this experimental process should enable the identification of the operation ‘set-point’, i.e. the values of the factors for which the response of the process is maximum, minimum or close to a target. For triboelectrostatic separation, maximisation of process recovery and process purity of the separated products are the criteria considered for modelling and optimisation. The composite centred faces design (CCF) is generally used, because it proposes quadratic polynomials models. With such models, the response of the process is expressed as a function of factors u i (i = 1,…,e):
A normalised centred value can be defined for each factor as follows:
With these notations, the function of response becomes:
In this section, optimisation of the process was studied with the most influent factors, easily controllable in our laboratory experimental bench, while keeping the applied high voltage at its maximum value, i.e. 50 kV. In the case of the rotating-cylinder-type tribocharging device represented in Figure , the charge of the particles and hence the efficiency of the electrostatic separation is likely to depend specifically on the mass m of the granular material introduced in the cylinder, the charging time t and the speed n at which the cylinder rotates. Thus, the factors selected for optimisation and the limit values of each one are:
-
Rotation speed n (rpm) of the tribocharging cylinder
-
Charging time t (s)
-
Mass of the sample m (g)
According to the factors considered in the present study: x 1 = n *, x 2 = t * and x 3 = m *, the quadratic model will take the following form:
5.2 DOE software
The experimental data were analysed with a DOE software: MODDE 5.0 (Umetrics, Umeå, Sweden; MODDE Citation5.0 1999). The program calculates the coefficients of the mathematical model, draws the response contours and identifies the best adjustments of the parameters for optimising the process. Moreover, the program calculates two statistical criteria: the goodness of fit: R 2, and the predictive power: Q 2. The latter is a measure of how well the model will predict the responses for the new experimental condition.
A good mathematical model must have criteria R 2 and Q 2 with the numerical value close to the unit.
5.3 Obtained results and discussion
The response considered for this optimisation purpose is the mass of the mixed product. The latter is lower when the recovery and the purity of PVC are higher and vice-versa.
The results of the CCF are given in Table . Software MODDE.05 proposes a table of 17 experiments to carry out, with well defined factor values for each experiment. The numerical values of factors n, m and t in Table represent limit and central values of each factor.
Table 1 Results of the CCF experiment design.
The mathematical model of the response considered for optimisation (i.e. the mass of middling) was obtained with MODDE 5.0 as follows:
The factors in Equation (Equation9) are defined as follows.
n *, t* , m* are the normalised centred value of speed n, time t and sample mass m, respectively
According to this model, the optimum of the process should be obtained for, n = 200 rpm, t = 45 s and m = 160 g. By using the optimisation routine of MODDE 5.0 it was found that this set-point maximises also the recovery of PVC (Figure ).
6 Conclusion
Current results using research on triboelectrostatic recycling of plastics indicate that the principle can be applied to a variety of different types of plastics and under the appropriate conditions, triboelectrostatic separation can be very effective for removal of impurities. The future focus of research is in the area of continuous operation in order to determine the optimum design of a practical separator and to determine the influence of important processing parameters on performance.
The preliminary data, obtained from PVC and PE wastes separation, indicate that simple triboelectric separator is effective in producing pure materials. The degree of the purity and recovery was found to be dependent on the material composition, high voltage, rotation speed and the charging time of the particles.
Taguchi's methodology is a powerful tool for the optimisation of triboelectrostatic separation process, within which there is an inherent variability due to many factors. Through the proper selection of the operating conditions of the electrostatic separation installation, the process can be made less sensitive to variations, thus avoiding the costly eventualities of product rejection and/or retreatment.
References
- Dance, A.D., Kojovic, T., and Morrison, R.D., 1991. Development of electrostatic separation models for the mineral sands industry. In: Proceedings of Extractive Metallurgy, Perth, 13–18
- Das , S. 2007 . Separation of fine granular mixtures in S-plate-type electrostatic separators . Industry Applications, IEEE Transactions , 43 ( 5 ) : 1137 – 1143 .
- Dascalescu , L. 2005 . Robust control of electrostatic separation processes . Industry Applications, IEEE Transactions , 41 ( 3 ) : 715 – 720 .
- Dascalescu , L. 1998 . Electrostatic separation of insulating and conductive particles from granular mixes . Particulate Science and Technology , 16 ( 1 ) : 25 – 42 .
- Finseth, D.H. and Gerstler, W., 1994. Triboelectrostatic coal separators: Design and development. 10th Korea-USA joint workshop on Coal Utilization Technology, 5, 18–19 October
- Frigon , N.L. and Mathews , D. 1996 . Practical Guide to Experimental Design , New York, NY : Wiley .
- Haga , K. 1995 . “ Applications of the electrostatic separation technique ” . In Handbook of electrostatic processes , Edited by: Chang , J.S. , Kelly , A.J. and Crowley , J.M. 365 – 386 . New York, NY : Dekker .
- Hicks , C.R. and Turner , K.V. Jr . 1999 . Fundamental concepts in the design of experiments , Oxford, UK : Oxford University Press .
- Higashiyama, Y. and Asano, K., 1998. Recent progress in electrostatic separation technology. Particulate Science and Technology, 16(1), 77–90; Inculet, I.I., 1986. Electrostatic mineral separation. New York, NY: Wiley
- Inculet , I.I. and Castle , G.S.P. 1991 . Tribo-electrification of commercial plastics in air . Proceedings of the 8th International Conference Electrostatics Electrostatics'91, Oxford, England; Inst. Phys. Conf. Set. No. 118 , : 217 – 222 .
- Inculet , I.I. , Castle , G.S.P. and Brown , J.D. 1994 . Tribo-electrification system for electrostatic separation of plastics . Proceedings of the Annual Meeting IEEE IAS , 2 : 1397 – 1399 .
- Inculet , I.I. , Castle , G.S.P. and Brown , J.D. 1998 . Electrostatic separation of plastics for recycling . Particulate Science and Technology , 16 ( 1 ) : 77 – 90 .
- Iuga , A. 2001 . Electrostatic separation of metals and plastics from granular industrial wastes . IEE Proceedings – Science, Measurement and Technology , 148 : 47 – 54 .
- Lawver , J.E. and Dyrenforth , W.P. 1973 . “ Electrostatic separation ” . In Electrostatics and its applications , Edited by: Moore , A.D. 221 – 249 . New York, NY : Wiley .
- Medles , K. 2007a . Set point identification and robustness testing of electrostatic separation processes . Industry Applications, IEEE Transactions , 43 ( 3 ) : 618 – 626 .
- Medles , K. 2007b . Experimental modeling of the electrostatic separation of granular materials . Particulate Science and Technology , 25 ( 2 ) : 163 – 171 . ISSN: 1548-0046
- Mihailescu , M. 2002 . Computer-assisted experimental design for the optimisation of electrostatic separation processes . Industry Applications, IEEE Transactions , 38 ( 5 ) : 1174 – 1181 .
- Ralston , O.C. 1961 . Electrostatic separation of mixed granular solids , Amsterdam : Elsevier .
- MODDE 5.0 . 1999 . User Guide and Tutorial , Umea, Sweden : Umetrics AB .
- Peace , G.S. 1992 . Taguchi Methods , New York, NY : Addison-Wesley .
- Roy , R.K. 2001 . Design of the Experiments Using Taguchi Approach. 16 Steps to Product and Process Improvement , New York, NY : Wiley .
- Soong , Y. 1998 . Triboelectrostatic separation of mineral matter from Slovakian Coals . Acta Montanistica Slovaca Ročník , 3 ( 3 ) : 393 – 400 .
- Tilmatine , A. 2003 . Electrostatic separation of granular particles . Journal of Materials Technology , 18 ( 4 )
- Tilmatine , A. 2004 . Séparation électrostatique: complément des procédés mécaniques de recyclage des déchets industriels . Journal of Electrostatics , 61 ( 1 ) : 21 – 30 .
- Tilmatine , A. 2009 . Electrostatic separators of particles: Application to plastic/metal, metal/metal and plastic/plastic mixtures . Waste Management , 29 : 228 – 232 .
- Younes , M. 2007 . Numerical modeling of conductive particle trajectories in roll-type corona-electrostatic separators . Industry Applications, IEEE Transactions , 43 ( 5 ) : 1130 – 1136 .
- Younes , M. 2008 . Fuzzy control of an electrostatic separation process . IEEE Transactions on Industry Applications , 44 ( 1 ) : 9 – 14 .
- Wu , Y. and Moore , W.H. 1986 . Quality engineering product and process optimization , Dearborn, MI : American Supplier Institute .