Abstract
Cassava tubers produced under hot, dry and monsoon governed climate in the Salem region, Tamil Nadu, South India, consume together with the about 1000 starch extracting industries daily, 9 millions of litres of groundwater which have to be pumped from a depth of 80 m below ground level. Because of water shortage, adjacent fields are irrigated with organically highly enriched sago factories wastewater and the land becomes unproductive. One-third of the wastewater is presently channelled through a few installed biogas plants with a purification efficiency, biological oxygen demand, of only around 30%. Microbiologically and chemically analysed in- and effluents of biogas plants exhibited nutrient contents sufficient to maintain a rice crop and significantly higher population densities of fermenting bacteria and methanogenic archaea, despite C/N ratio of about 250. CO2 and CH4 emissions from aerobic and anaerobic incubated sago factory biogas plants in- and effluents indicated that the present purification efficiency of 30% can be further enhanced. This investigation has given clues for designing a purification system that connects the already installed biogas plants with a well-aerated, hydro-cultured, constructed wetland and a Stirling motor device for electric power gains and heat distillation, for enabling reuse of process water and saving groundwater.
Abbreviations
16S rRNA | = |
16S ribosomal ribonucleic acid |
ARD | = |
A.R. Durai Sago factory |
ARDRA | = |
amplified ribosomal DNA restriction analysis |
BDL | = |
below detection limit |
BLAST | = |
basic local alignment search tool |
BOD | = |
biological oxygen demand |
COD | = |
chemical oxygen demand |
EDTA | = |
ethylenediaminetetraaceticacid |
NTSYS | = |
numerical taxonomy system |
PCR | = |
polymerase chain reaction |
SSS | = |
Sree Selliamman Sago factory |
TDS | = |
total dissolved solid |
TE | = |
Tris–EDTA |
TS | = |
total solid |
TSS | = |
total suspended solid |
VMS | = |
Velmurugan Sago factory |
WHO | = |
World Health Organization |
1. Introduction
The World Health Organization (WHO) prophesises water shortage for about 118 countries and recommends economical water saving concepts for agro-climatic regions such as the monsoon governed South Indian state, Tamil Nadu, receiving major rainfalls between July and September and facing water shortage for almost half of the year. Cassava plants (Manihot esculenta Crantz) prefer well-aerated soils as found in Salem district of Tamil Nadu, which recorded in an average 24 ton tubers ha− 1 (world average 10 ton ha− 1; Abraham et al. Citation2000). Cassava tubers serve as staple food for 780 million people, which are cultivated covering 16.5 m ha in Asia, 3.28 m ha in India, 0.86 m ha in Tamil Nadu and 0.25 m ha in Salem region and 0.102 m ha worldwide. Thousands of farmers, processors and traders benefit from cassava starch that is industrially processed to sago, dextrin, liquid glucose, dextrose, fructose syrup, maltose, malt dextrin, gum, biodegradable plastics or used for cotton yarn sizing and paper coating (Philips Citation1983, Balagopalan Citation2002, Onwueme Citation2002, Srinivas and Anantharaman Citation2004, Edison et al. Citation2006, Srinivas Citation2007). For improving crop breeding and counteracting the world hunger, the Melinda and Bill Gates foundation has financed the sequencing of the cassava genome (Science Citation2009).
In the Salem region, around 5.8 × 105 ton cassava tubers are annually harvested with starch contents up to 35%, appreciable amounts of calcium and vitamin C. Cassava plants with a cropping period of 10 months consume about 1.500 m3 water ha− 1 of crop. Sago factories separating starch from cassava tuber components by an indigenous technology need 30–40 m3 groundwater ton− 1 of starch produced. The sago factories of the Salem region, extracting on an average 5 ton starch from cassava tubers daily, must pump out 150–200 × 103 m3 of groundwater from a depth of about 80 m (Ranjithkumar et al. Citation2010). Corresponding amounts of wastewater, enriched with residual tuber material and hydrocyanic acid (CN), are released into the environment. CN, released through an enzymatic break down from cyanogenic glycosides and linamarins due to the crushing of white inner tissue parts of the tubers, is a strong respiration inhibitor (Oboh and Akindahunsi Citation2003, Ayyasamy et al. Citation2008). Sago factory wastewater with CN and the high organic loads, frequently used for irrigating adjacent fields, presumably make 1000 ha of land unproductive and spread an anoxic odour, as reported in the local newspaper (Dinamalar, June 6th ed., 2009).
Irrigating cassava crop (80% of the cassava cropping area of Tamil Nadu is in the Salem region) and industrial processing of its tubers for production of starch overexploit the groundwater by more than 100%, resulting in continuous decline in the groundwater level. Consequently, cassava cropping decreases despite the increasing demands for cassava tuber starch for industrial, nutritional and renewable energy requirements (Edison et al. Citation2006).
To overcome water shortage and environmental impacts, some sago factory owners have established biogas plants with the aim of reusing process water and saving groundwater. The biogas plants installed in the sago factories presently treat one-third of the wastewater produced everyday with a retention time of 1 day. For assessing the efficiency of biogas plants, the in- and effluents of three differently sized sago industries were chemically and microbiologically analysed and the results are reported and discussed in this paper. On the basis of the observed chemical and bacterial changes during wastewater purification in the biogas plants, a concept for a reusing of process water by sago factories was developed and is also discussed.
2. Materials and methods
2.1 Sampling of cassava sago factory wastewater
The distribution of rainfall and temperature, the cassava varieties grown and tuber yields in the monsoon governed by Tamil Nadu State of South India (11°34″ N; 78°8″ E), the starch extraction procedure followed in the sago factories of Salem district, the amounts of organically enriched wastewater generated and the groundwater overexploitation of this region are reported in detail by Ranjithkumar et al. (Citation2010). To analyse biogas plants in- and effluents chemically and microbiologically, 500 ml of unfiltered (raw) water samples was collected from a small-scale sago industry [A.R. Durai Sago factory (ARD) with production capacity of < 2000 kg day− 1], a medium-scale sago industry [Sree Selliamman Sago (SSS) with production capacity of ∼5000 kg day− 1] and a large-scale sago industry [Vel Murugan Sago (VMS) with production capacity of >10,000 kg day− 1] on 24 June (off-season) and 29 November 2008 (peak season). The samples were transported in a cooling box to the Tamil Nadu Agricultural University in Coimbatore, India, and further to Justus Liebig University, Giessen, Germany. For microbial counts and incubation experiments, the samples were stored in a cold room (+4°C); and for chemical and molecular biological analysis, the samples were stored in a deep freezer ( − 20°C) and experiments were carried out either at Coimbatore or at Giessen.
2.2 BOD, COD, pH, total solids, micronutrients and macronutrients
At each sampling, pH (Jackson Citation1973), dry weight of total suspended solids (TSSs) retarded on a Whatman filter grade 1 (pore size, 11 μm), dry weight of total dissolved solids (TDSs) in the filtrate (Degen and Numberger Citation1956, Sokoloff Citation1933), the biological and chemical oxygen demands (BOD and COD; Bridge Citation1969, Hey et al. Citation1969), the total organic carbon (Walkley and Black Citation1934), Kjeldahl N (Keeney and Nelson Citation1982), phosphorus (Jackson Citation1973) and the macronutrients and micronutrients K, Ca, Mg, Na, Fe, Mn, B, Co, Ni, Cu, Zn and Mo by an inductively coupled plasma mass spectrometer, equipped with an octopole reaction system (Agilent 7500 EC, Agilent, Santa Clara, California, USA or Waldbronn, Germany; Jakubowski Citation2008), were determined besides sugars and organic acids.
2.3 Sugars and organic acids
Sugars in the sago factory wastewater samples were identified and quantified with Dionex DX 500 HPLC system (CarboPac TM PA20 analytical column, 250 mm length, 3 mm in diameter, connected to a 50 mm guard column, flow rate: 0.5 ml min− 1, column temperature: 70°C; van Bel and Hess Citation2008) and organic acids with the Sykam HPLC (column: Aminex HPX-87P, BioRad, Berkerly, California, USA; UV detector: UVIS-2005, Erna-ERC-3311, Tokyo, Japan; eluent: 1 mM H2SO4; flow rate: 0.8 ml min− 1; column and detector temperatures: 65 and 40°C, respectively; Krumbock and Conrad Citation1991). Before thawing of the frozen 1 ml wastewater samples in boiling water for 10 min, 0.025 g of Polyclar, a phenol-binding substance (Serva, Heidelberg, Germany), was added to the 2-ml Eppendorf cups. The samples, cooled down on ice, were centrifuged (4°C and 1000g for 10 min; Hettich-Mikro-22R, Hamburg, Germany) and the supernatants were injected into the corresponding HPLC system (0.6 ml of sugars and 100 μl of organic acids). The standards for sugars contained myoinositol, d(+)-trehalose, d(+)-glucose, d( − )-fructose, d(+)-sucrose, d(+)-melizitose monohydrate, d(+)-raffinose pentahydrate and stachyose trihydrate in a concentration of 10 mg l− 1 each and for organic acids citrate, malate, succinate, ethanol, fumarate, acetate, propionate, butyrate, valerate and capronate in 100 μM each (detection limit 5–20 μM).
2.4 Incubation of sago factory wastewater and assessment of CO2 and CH4 gas emissions
Ten millilitres of biogas plants in- and effluents were incubated in airtight, rubber-stoppered bottles of about 115 ml capacity (three replicates) at 37°C, and the emission of CO2 and CH4 gases was estimated by injecting 0.5 ml headspace samples and standard gases of known CO2 and CH4 concentrations with an airtight syringe (Pressure-lock®A-2 series, Dynatech, Baton Rouge, LA, USA) into a gas chromatograph (PerkinElmer Autosystem XL B 5902, 3-m Poropak Q column, 50°C), equipped with an electron capture detector (275°C) and flame ionisation detector. The samples were incubated either under initial aerobic or anaerobic conditions. In the anaerobic set, the bottles were flushed with sterile N2 for 3 min before they were closed airtight with rubber stoppers. The concentration of the standards and samples corresponding to their respective peak areas were integrated by a SRI-PeakSimple Chromatography Software, Model 202, Torrance, USA) and the CO2 and CH4 gas emissions in the samples were calculated in μmol ml− 1 wastewater by the gas equation: n = pV/RT (p, pressure in kPa; V, headspace volume in ml; R, gas constant; T, temperature in K).
2.5 Bacterial cell counts
Fermenting and denitrifying bacteria and methanogenic archaea in the sago factory wastewater were quantified by the anaerobic most probable number (MPN) technique (De Man Citation1983, Widdel and Bak Citation1992). All three media used for enumeration of fermenting, denitrifying and methanogenic archaea had a common sterile basal salts medium (NaCl, MgSO4·7H2O, CaCl2·2H2O, KCl, KH2PO4 and NH4Cl at concentrations of 1.0, 0.486, 0.15, 0.5, 0.2 and 0.25 g l− 1, respectively). For fermenting bacteria, the sterile basal salts medium was added with filter sterilised 5 mM glucose, 5 mM xylose, vitamin B12 (1.0 ml l− 1), a pyridine-di-hydrochloride-nicotinic acid-Ca-d(+)-pantothe-nate-4-aminobenzoic acid d(+)-biotin vitamin-mixture (1.0 ml l− 1), riboflavin (1.0 ml l− 1), thiamine solution (1.0 ml l− 1), cysteine (0.6 ml l− 1), 1.0 ml l− 1 of a trace elements solution (Na2–EDTA, FeSO4·7H2O, CoCl2·6H2O, ZnSO4·7H2O, MnCl2·4H2O, Na2MoO4·2H2O, H3BO3, NiCl2·6H2O, CuCl2·2H2O and CuSO4·5H2O in the concentrations of 5200, 2100, 190, 144, 100, 36, 30, 24, 2 and 29 mg l− 1, respectively), NaHCO3 buffer (30 ml l− 1) and Na2S·9H2O (5 ml l− 1). Sterile 9 ml medium was transferred into sterile rubber-stoppered test tubes with Durham tubes (for gas collection with three replicates). The medium was inoculated with 1 ml diluted biogas in- and effluents (10− 10). Each inoculated tube was flushed with sterile N2 for 1 min, using closed airtight rubber stoppers, and incubated at 25°C. Growth was evaluated by turbidity and gas formation and the results were expressed in MPN ml− 1 wastewater.
The procedure for counting denitrifying bacteria resembled that of fermenting bacteria except that the medium contained 0.425 g l− 1 NaNO3 instead of the sugars (5 mM). After flushing the tubes with sterile N2 for 1 min, they were inoculated with 10− 6 diluted wastewater and incubated at 28°C. The growth was evaluated by turbidity and gas production and the counts were expressed in MPN ml− 1 wastewater.
Methanogenic archaea were counted in the sterile basal salts solution, to which 40 mM filter sterilised trimethylamine, 0.35 mM mercaptoethanesulphonic acid, 0.5 g l− 1 yeast extract, 0.5 g l− 1 peptone, 5 mM acetate, 5 mM methanol, 100 mM formic acid, 1.0 ml l− 1 of the above vitamins and trace elements solutions were added under a continuous N2/CO2 stream (80/20 v/v). The final pH was adjusted to 7.2 with 1 M HCl or Na2CO3. Growth in the airtight tubes flushed with N2/CO2 was evaluated by turbidity. The counts are expressed in MPN ml− 1 wastewater.
CN-tolerating bacteria were enumerated aerobically on agar plates, which contained the medium used for fermenting bacteria, to which 5 mM NaCN, 3 g l− 1 peptone and yeast extract and 15 ml l− 1 of sodium phosphate buffer were added. The plates were inoculated with 1 ml of 10− 6 diluted wastewater and aerobically incubated at 28°C. Colony-forming units (cfu) were counted and expressed in ml− 1 wastewater.
The cfu of fungi (aerobically incubated at 28°C) were estimated in Martin's Rose Bengal agar containing glucose, peptone, KH2PO4, MgSO4, streptomycin (filter sterilized), Rose Bengal and agar in the concentration of 10, 5, 1, 0.05, 0.03, 15, 0.035 and 15 g l− 1 distilled water, respectively (Martin Citation1950).
2.6 DNA extraction, amplification and 16S rRNA clone library construction
Briefly, 10 ml of sago factories' wastewater samples were centrifuged (13,708g, 5 min, room temperature). For extracting DNA from the pellets, cells were suspended in 600 μl of sodium phosphate buffer (120 mM, pH 8), 100 μl lysozyme (10 mg ml− 1) and after incubation (1 h, 37°C) 10% sodium dodecyl sulphate (567 μl) and 15 μl of proteinase K (20 mg l− 1) were added. The samples were incubated at 60°C for 15 min and centrifuged. Then, 700 μl sodium phosphate buffer and ammonium acetate solution (7.5 mM, 560 μl) were added to dissolve the pellets. The collected supernatants after centrifugation (13,708g, 6 min, 4°C) were spiked with 1372 μl isopropanol (99%) and stored overnight in the deep freezer. After thawing and centrifugation (13,708g, 1 h, 4°C), the supernatants were discarded, the pellets were added with 600 μl of ethanol (70%), vortexed, centrifuged (16,808g, 5 min), the supernatants discarded and the pellets air dried. The DNA concentration in the resuspended pellets [150 μl Tris–EDTA (TE) buffer, pH 8] was estimated by UV spectrophotometry, and electrophoresis, and the samples were stored at 4°C.
In the following polymerase chain reaction (PCR), the genomic DNA acted as template. The PCR mixture (total volume 25 μl) contained about 20 ng DNA μl− 1, 50 mM Tris (pH 8.3), 0.16 mg bovine serum albumin ml− 1, 2.5 mM MgCl2, 200 μM deoxynucleotide triphospates, 0.2 μM and 0.03 U Taq polymerase, Mr forward primer (GAGTTTGATCMTGGCTCAG) and Mr reverse primer (ACGGYTACCTTGTTACGACTT; Invitrogen, Carlsbad, California, USA) each (Weisburg et al. Citation1991). The PCR was carried out in a thermocycler (MyCyclerTM, BioRad, Berkerly, California, USA; 92°C for 1 min, 32 PCR cycles at 94°C for 45 s, 58°C for 45 s, 72°C for 60 s and 72°C for 3 min). The amplified and cloned 16 rRNA genes (pGEM-T Easy Vector Systems, PromegaCorp., Madison, USA) resulted in 600 colonies. Correct transformed, with M13f (GTAAAAGGACGGCCAG) and M13r (CAGGAAACAGCTATGAC) primers, PCR-screened and positive clones were subjected to an amplified rDNA restriction analysis (ARDRA, ‘FastDigest kit’, Fermentas, Leon-Rot, Germany; 5 μl cloned PCR products, 2 μl 10 × FastDigest® buffer, 0.1 μl of the restriction enzymes HpaII and Hin6I, 12.9 μl PCR water, incubation: 37°C, 10 min). The ARDRA patterns on a 2.7% agarose gel were documented (Gel Doc) and compared (Photoshop). From the grouped clones [Numerical Taxonomy System (NTSYS), version 1.1b, Applied Biostatistics, Inc, Port Jefferson, USA], one representative of each group was sequenced (AGOWA, Berlin, Germany), improper proportions from the received sequences were deleted, an alignment and a Basic Local Alignment Search Tool (BLAST) with MEGA4 were carried out and phylogenetic trees were constructed by neighbour-joining method (1000 bootstrap replicates, Higgins et al. Citation1994).
3. Results
The population density changes of respiring, fermenting, denitrifying and cyanide-tolerating bacteria, methanogenic archaea and aerobically growing fungi in the in- and effluent samples of biogas plants, shown in Table , unveil that fermenting bacteria dominate the sago factories wastewater. Fermenting bacteria increased in the biogas plants by 1000 times, cyanide-tolerating bacteria by 100–10,000 times and the methanogenic archaea by more than 106 times, whereas the denitrifiers, aerobic bacteria (106 cfu) and fungi (103 cfu), with low population densities, did not change or changed slightly during biomethanation.
Table 1 Bacterial, fungal and archaea populations in biogas plants in- and effluents of three sago industries (ARD, SSS, VMS: daily starch production of 2, 5 and 10 ton, respectively) in the Salem region of Tamil Nadu, South India.
The changes in bacterial species in the biogas plants fed with sago factory wastewater, shown in Figure , exhibited that Lactobacillus spp. dominate the bacterial spectrum of the biogas plants' influents by 77%. Besides Lactobacillus spp., Bacteroidetes (Prevotella spp.), Proteobacteria (Rhizobium spp., Defluvibacter sp.) and Firmicutes (Megasphaera spp. and Dialister spp.) were also present in the influents, but at a significantly lower percentage than Lactobacillus spp. The presence of Lactobacillus spp. in the biogas plants effluents decreased from 67 to 21%, whereas the proportion of Firmicutes Proteobacteria and Clostridium spp., not detectable in the biogas plants influents, enhanced by 30 or 32%, respectively, in the effluents. The bacterial phyla Cyanobacteria (Synechococcus spp., Hapalosiphon spp.), Verrucomicrobia (Verrucomicrobium spp.), Synergistetes (Thermanaerovibrio spp.) and Fusobacteria (Fusobacterium spp.), which were also not detectable in the biogas plants influents, were enriched by 7% in the effluents.
Figure 1 Distribution of microbial communities in biogas plants in- and effluents of the three sago industries in the Salem region of Tamil Nadu, South India (ARD, SSS, VMS: daily starch production of 2, 5 and 10 ton, respectively).
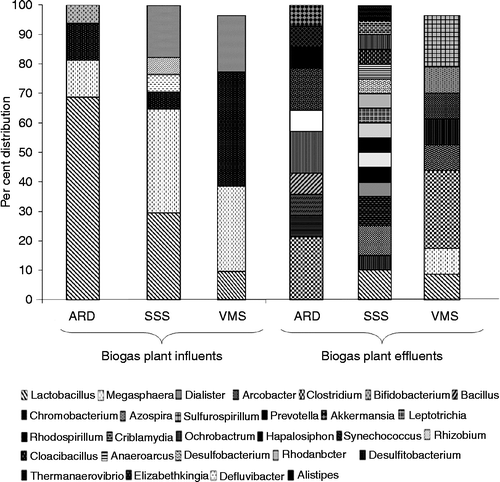
Biogas plants fed with sago factory wastewater were found to provide good growth conditions for sugars and amino acids fermenting Clostridia, where besides glucose, fructose and saccharose, the pectin component myoinositol were highly concentrated (Table ). The conversion of sugars into organic acids in the biogas plants (Table ) contributed assumingly to the shift in the fermenting bacterial population to the methanogenic archaeal population (Table , Figure ).
Table 2 Sugars in the biogas plants in- and effluents of three sago factories (ARD, SSS, VMS: daily starch production of 2, 5 and 10 ton, respectively) in the Salem region of Tamil Nadu, South India.
More than sugars (μmol l− 1, Table ), the three sago factory biogas plant influents were dominated by acetate, propionate and butyrate (82.5%; mean 27 mmol ml− 1; Table ). The organic acids concentration in the biogas plants effluents was lower by around 95% than the influents, and the presence of these acids presumably helped to increase the methanogenic archaea (Table ).
Table 3 Acids in the biogas plants in- and effluents of the three sago factories (ARD, SSS, VMS: daily starch production of 2, 5 and 10 ton, respectively) in the Salem region of Tamil Nadu, South India.
The CO2 and CH4 gas emissions from incubated biogas plant in- and effluents, shown in Figures ), indicated increasing CO2 emissions, after 6 h of incubation. In the bottles with air atmosphere, oxygen has been respired after 24 h (data not shown). Around 48 h aerobic incubation [130–350 μmol CO2 ml− 1, Figure (B),(D),(F)] and 96 h anaerobic incubation [36–93 μmol CO2 ml− 1, Figure (B),(D),(F)], the CO2 emissions were 80% higher under initial aerobic conditions than under subsequent completely anaerobic conditions. As the incubation periods changed from aerobic to anaerobic phase, CO2 gradually decreased. Overall, the incubated biogas plants influents produced 62% more CO2 than the effluents.
Figure 2 CO2 and CH4 emitted from biogas plant in- (A, C, E) and effluents (B, D, F) of three sago factories (ARD, SSS, VMS: daily starch production of 2, 5 and 10 ton, respectively) in the Salem region of Tamil Nadu, South India, incubated under anaerobic condition (CO2: ▪, on-season; ▪, off-season and CH4: •, on-season; ○, off-season).
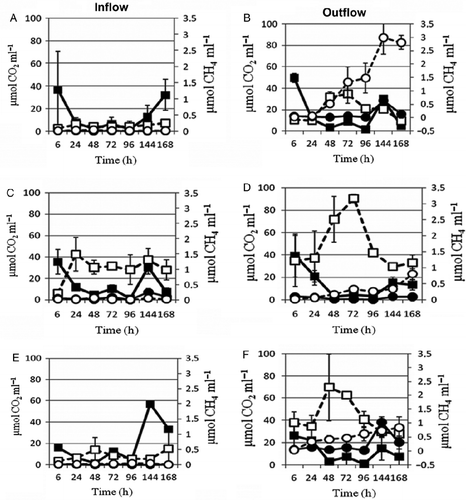
Figure 3 CO2 and CH4 emitted from biogas plant in- (A, C, E) and effluents (B, D, F) of three sago factories (ARD, SSS, VMS: daily starch production of 2, 5 and 10 ton, respectively) in the South Indian region of Salem, Tamil Nadu, incubated under initial aerobic condition (CO2: ▪, on-season; ▪, off-season and CH4: •, on-season; ○, off-season).
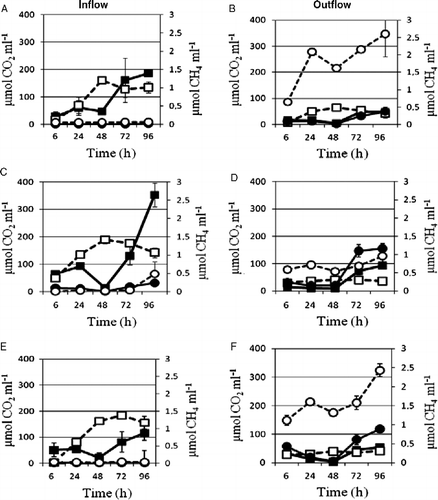
The CH4 emissions exhibited a reverse trend. The incubated biogas plants influents have recorded between 0.01 and 3 μmol CH4 ml− 1 wastewater, which was less by 90% than the effluents (Figures and (A),(C),(E)). The higher methane emissions from sago factory biogas plants effluents (Figures )) are in agreement with the increasing population densities of methanogenic archaea in the biogas plants (Table ). Since sugars have six carbons and four acids, assumingly around 28% of the available sugars C and 4% of the acids C were released as CO2. Most of the sugars C and acids C was evidently consumed by the increasing fermenting, cyanide-tolerating bacterial and archaea populations (Tables ) and only a small proportion of CO2 produced was seemingly converted into CH4. A comparison of the CO2 and CH4 emissions in between the three investigated sago factories and the on-season and off-season samplings showed that the results obtained are not statistically significant.
Organic carbon, TDS+TSS, BOD decreased in the investigated biogas plants with a 1- day retention time by 7.1, 28.3 and 32%, respectively (Table ), which revealed its limited purification potential in the given conditions. Nitrogen deficiency expressed by the wide C/N ratio of the influents (∼250), and the short retention time of the wastewater in the biogas plants could explain the low purification efficiency (Table ).
Table 4 Physio-chemical properties of the biogas plant in flows and outflows of the three sago factories (ARD, SSS, VMS: daily starch production of 2, 5 and 10 ton, respectively) in the Salem region of Tamil Nadu, South India.
Cyanides, known as strong respiration inhibitors, completely disappeared in the wastewater during the 1 day biogas plant retention time, and it can be speculated that they served as an additional nitrogen source. The raise in the pH from 3 in the biogas plant influents to 7.3 in the effluents may have contributed to the shift from the acid-tolerant Lactobacillus spp. to Clostridia, Proteobacteria and presumably methanogenic archaea (Figure , Table ). The nutrients except nitrogen per cubic meter of sago factory wastewater seem to be sufficient for supporting the growth of a high-yielding rice crop (Table ), and it can be hypothesised that rice plants, hydro-cultured in an aerated constructed wetland, could be a tool in achieving process water reuse (Figure ).
Table 5 Macronutrients and micronutrients contents in sago factory wastewater in comparison with the demand of a high-yielding wetland rice crop.
Figure 4 Proposed sago factory wastewater treatment system for achieving process water reuse (Ranjithkumar et al. Citation2010).
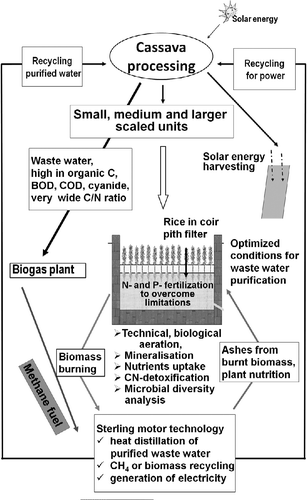
4. Discussion
Wastewater treatment engineering and ecology have complementary goals and need to interact much more closely. They share strong interests in the structure and function of biological communities, yet rarely engage in extensive interdisciplinary dialogue. Typically, untreated sago factory wastewater of all the three sago factories of Salem region in Tamil Nadu consisted of C/N ratios of around 250, an acidic pH, accumulating cyanides and enough nutrients to maintain a rice crop (Tables ). When the organically highly enriched sago factory wastewater is channelled through biogas plants, the pH raised to about 7 accompanied by significant increase in population densities of fermenting, cyanide-tolerating bacteria and methanogenic archaea. Although the introduced cyanides diminished due to its utilisation as nitrogen source by bacteria, the BOD decreased only by around 32%. Limited nitrogen and eventually phosphorus availabilities together with the relatively short retention time of 1 day in the anaerobic biogas plants environment may explain the lower efficiency of conversion of organic matter into CO2 and CH4, but for achieving the aims expressed by the sago factory owners, viz. process water reuse for saving groundwater and higher energy gains from the wastewater channelled through the installed biogas plants, the hidden potential as indicated by the incubation experiments (Tables and , Figures and ) must be better used. Therefore, the purification system, shown in Figure , is proposed for achieving the twin objectives of near total purification of wastewater and reuse of the treated water for sago processing.
The amount of energy in the organically enriched wastewater (E) is the product of the amount of water (m) and the square of the speed of light in a vacuum (E = mc 2, Einstein). Since the square of the speed of light is a very large number, a tiny bit of matter may contain an enormous amount of energy, which is only insufficiently exploited as revealed by a mere 32% BOD decrease in significantly higher population densities (Tables and ). The multiplication of anaerobes in the biogas plants and the related metabolisms that led to the disappearance of cyanides in the wastewater provoke a shift in microbial population from more acid-tolerant Lactobacillus sp. to Proteobacteria and Clostridia (Figure ) and signalise that at appropriate conditions, more organic compounds including solid fractions could be converted into energy-yielding molecules. The raising pH of wastewater could have contributed to the shift towards Clostridia, which are involved in the fermentation of sugars and amino acids and the presumably better nitrogen availability allowed other microbial groups to multiply.
Enhanced nitrogen content in the sago factory wastewater channelled through the biogas plants could have stimulated the archaeal population with known capabilities of fixing N2, by having a similar broad spectrum of anabolic and catabolic pathways as bacteria (Dekas et al.
Citation2009, Benckiser and Bamforth Citation2010, Jarrell et al.
Citation2011). Archaea grow under aerobic, phototrophic, lithotrophic, fermenting, nitrifying, denitrifying and H2-dependent sulphur reducing conditions. They possess several proton-translocating ATP synthases and translocate only one ion per catabolic cycle. Proton reduction in archaea is directly coupled to proton translocation and the stoichiometry of ion translocation can be varied with the availability of energy from substrates. Archaea consume only a fraction of ATP from the net energy driving catabolism and maintain a better chemiosmotic potential than bacteria. The conversion of carbon dioxide into CH4 and anaerobic oxidation of CH4 are exclusively carried out by archaea having membranes consisting of isoprenoidal alcohols instead of fatty acids esterified to a glycerol in bacteria. Archaeal membranes are less permeable to ions than those of bacteria, and energy losses can drastically be reduced as evident from the growth, maintenance and survival ratio of 106:103:1, respectively. Overall, archaea are superior adapted to chronic stress and bacteria to fertile conditions. As soon as CO2 becomes major electron acceptor, bacteria are out-competed. But the interrelations between bacteria, archaea and fungi in biogas plants fed with sago factory wastewater of extremely wide C/N ratios are not yet fully understood.
The preliminary microscopic counts of fungi in the biogas plant in- and effluent samples (Table ) indicated that many environmental processes, particularly those associated with the extracellular conversion of polymeric structures into monomers, may be influenced (Blackwell Citation2010, Sime-Ngando et al. Citation2010). Recent environmental 18S rDNA surveys of microbial eukaryotes have helped (1) to unveil a large reservoir of an unexpected fungal diversity, (2) to emphasise their ecological potentials for ecosystem functioning, (3) to open and suggest new perspectives in context of food–web dynamics and (4) to study the cascading concept of ‘microbial loop’ among zoosporic fungi including their hidden diversity and ecological potentials in converting organic solids into fermentable monomers (sugars and amino acids) in anaerobic- and aerobic-treated environments like sago factory wastewater.
In the context of enhancing the purification efficiency and achieving process water reuse, it is suggested to connect the already installed biogas plants of the sago factories with a well-aerated constructed wetland and a Stirling motor-based distillation unit (Figure ). This would mean a better use of available nutrients by recycler microflora and plants and could be a better way to save groundwater by process water reuse. Concomitantly, the methane released by the biogas plant can be converted into electricity by Stirling motor units (Higgins et al. Citation2002). The sago factory owners become independent from the public energy supply system that presently limits their productivity due to its shortage in availability. Prerequisite for reducing environmental pollution by the suggested wastewater treatment system for the sago factory is to find out how the organic matter conversion and CH4 formation in the biogas plants and the other units can be improved with special emphasis on the archaeal and fungal functioning in the system as a next step.
5. Concluding remarks
Wastewater engineers are increasingly emphasising on ecological applications and, in turn, the ecologists should view engineered biosystems as valuable new platforms for ecological research. The presented data sets give closer insights into anaerobic-treated sago factory wastewater, but are still weak resolution of cryptic functions. A more accurate identification of microbial consortia and their functions are absolutely essential. New approaches unravelling the role of the microbial diversity in matter recycling, particularly in sago factory wastewater with nutritionally unbalanced resources and a high proportion of suspended solids are needed. First of all, the observed nitrogen deficiency in sago factory wastewater, relatively high percentage of suspended solids, signalised by very wide C/N ratios and the elimination of cassava tuber-derived toxic cyanides through the microbial detoxification in biogas plants, has to be better understood. Presently, lacking capacities due to costly investments shorten the wastewater retention times to 1 day and also contribute to the lower purification efficiency. Since the cloning/sequencing diversity of archaea and fungi, especially for the tiniest ( < 5 μm) individuals, is not yet completed, it is very important to study these groups in more detail. This is a challenge for environmental scientists and a prerequisite for approaching process water reuse in sago factories.
Acknowledgements
We thank the Ministry of Education and Research of the Federal Republic of Germany (BMBF) and the Department of Science and Technology, Government of India (DST), for generous financial support of the project and are grateful to Dr Dagobert Kotzur, Sunmachine, for introducing the idea of heat distillation by a Stirling engine, Prof. Dr Sylvia Schnell, Dr Stephan Ratering, Rita Geissler-Plaum and Heinz Schneider, Department of Applied Microbiology, Interdisciplinary Research Centre (IFZ), Justus Liebig University, for their support during experimentation, Prof. Dr Stephan Gäth, Dipl. Geoökol. Dipl Ing Dorit Zörner, Heike Weller and Beate Lindenstruth, Department of Landscape Ecology and Resource Management, for analysing the nutrients in the Sago factory wastewater and Prof. Dr Art van Bel and Helene Krufczik, Department of Botany, for analysing the sugars. We especially want to thank the sago factory owners for their cooperation during all of the sampling.
References
- Abraham , K. , Nair , S.G. and Naskar , S.K. 2001 . “ Cassava breeding and varietal dissemination in India. Major achievements during the past 25–30 years ” . In Cassava's potential in Asia in the 21st century: present situation and future research and development needs , Edited by: Howeler , R.H. and Tan , S.L. 174 – 184 . Vietnam : Centro International de Agricultura Tropical (CIAT) . Available from: www.ciat.cgiar.org/work/…/cassavas_potential_in_asia.pdf
- Ayyasamy , P.M. 2008 . Bioremediation of sago industry effluent and its impact on seed germination (green gram and maize) . World Journal of Microbiology and Biotechnology , 24 : 2677 – 2684 .
- Balagopalan , C. 2002 . “ Cassava utilization in food, feed and industry ” . In Cassava: biology, production and utilization , Edited by: Hillocks , R.J. , Thresh , J.M. and Bellotti , A.C. 301 – 318 . Wallingford : CAB International .
- Benckiser , G. and Bamforth , S.S. 2010 . Role of pathogens, signal recalcitrance, and organisms shifting for ecosystem recuperation . A review: Agronomy for Sustainable Development , Doi:10.1051/agro/2010024
- Blackwell , M. 2010 . Fungal evolution and taxonomy . BioControl , 55 : 7 – 16 .
- Bridge , A.L.A.M. 1969 . Determination of biochemical oxygen demand with continuous recording of oxygen uptake . Water Research , 3 : 157 – 165 .
- De Datta , S.K. 1981 . Principles and practices of rice production , 619 New York : Wiley .
- Degen , J. and Numberger , F.E. 1956 . Notes on the determination of suspended solids . Sewage Ind. Wastes , 28 : 237 – 240 .
- Dekas , A.E. , Poretsky , R.S. and Orphan , V.J. 2009 . Archaea fix and share nitrogen in methane-consuming consortia . Science , 326 : 422 – 426 .
- De Man , J.C. 1983 . MPN tables, corrected . European Journal of Applied Microbiology and Biotechnology , 17 : 301 – 305 .
- Edison , S. , Anantharaman , M. and Srinivas , T. 2006 . Status of cassava in India: An overall view, Central Tuber Crops Research Institute (Indian Council of Agricultural Research) . : 172 Technical Bulletin Series 46. Sreekariyam, Thiruvananthapuram, Kerala, India: St. Joseph's Press
- Hey , A.E. , Green , A. and Harkness , N. 1969 . An automated system for the determination of chemical oxygen demand . Water Research , 3 : 873 – 886 .
- Higgins , D. 1994 . CLUSTALW: improving the sensitivity of progressive multiple sequence alignment through sequence weighting, position, specific gap penalties and weight matrix choice . Nucleic Acids Research , 22 : 4673 – 4680 .
- Higgins , J. 2002 . Survey of users and providers of recycled water: quality concerns and directions for applied research . Water Research , 20 : 5045 – 5056 .
- Jackson , M.L. 1973 . Soil chemical analysis , 498 New Delhi : Prentice Hall of India Pvt Ltd .
- Jakubowski , N. 2008 . Analytical plasma ion sources for elemental mass spectrometry: where are we coming from–where are we going to . Journal of Analytical Atomic Spectrometry , 23 : 673 – 684 .
- Jarrell , K.F. 2011 . Major players on the microbial stage: why archaea are important . Microbiology , 157 : 919 – 936 .
- Keeney , D.R. and Nelson , D.W. 1982 . “ Nitrogen-inorganic forms ” . In Methods of soil analysis, Part 2: Agronomy monograph 9: chemical and microbiological methods , 2nd ed. , Edited by: Page , A.L. , Miller , D.R. and Keeney , D.R. 643 – 698 . Madison, WI : ASA, SSSA .
- Krumbock , M. and Conrad , R. 1991 . Metabolism of position-labelled glucose in anoxic methanogenic paddy soil and lake sediment . FEMS Microbiology Ecology , 85 : 247 – 256 .
- Martin , J.P. 1950 . Use of acid, rose bengal and streptomycin in the plate method for estimation soil fungi . Soil Science , 69 : 215 – 232 .
- Oboh , G. and Akindahunsi , A.A. 2003 . Biochemical changes in Cassava products (flour and gari) subjected to Saccharomyces cerevisae solid media fermentation . Food Chemistry , 82 ( 4 ) : 599 – 602 .
- Onwueme , I.C. 2002 . “ Cassava in Asia and the Pacific ” . In Cassava: biology, production and utilization , Edited by: Hillocks , R.J. , Thresh , J.M. and Bellotti , A.C. 55 – 65 . Wallingford : CAB International .
- Philips , T.P. 1983 . “ An overview of cassava consumption and production ” . In Cassava toxicity and thyroid , Edited by: Delange , F. and Ahluwalia , R. 83 – 88 . Ottawa, ON : International Development Research Centre .
- Ranjithkumar , R. 2010 . Cassava-Anbau, Stärkeextraktion, Grundwasserabsenkung in der Salemregion, Tamil Nadu, Südindien oder wie könnte das regionale Wassermanagement verbessert werden? . VDLUFA-Schriftenreihe , 65 : 149 – 161 . (Kongreßband 2)
- Science . 2009 . Rating last year's areas to watch . Science , 326 : 1602 Doi:10.1126/science. 326.5960.1602
- Sime-Ngando , T. , Lefevre , E. and Gleason , F.H. 2010 . Hidden diversity among aquatic heterotrophic flagellates: ecological potentials of zoosporic fungi . Hydrobiologia , 659 : 5 – 22 . Doi:10.1007/s10750-010-0230-y/
- Sokoloff , V.P. 1933 . Water of crystallization in total solids of water analysis . Industrial and Engineering Chemistry, Analytical Edition , 5 ( 5 ) : 336 – 337 .
- Srinivas , T. 2007 . Industrial demand for cassava starch in India . Starch , 59 : 477 – 481 .
- Srinivas , T. and Anantharaman , M. 2004 . “ Industrial demand for cassava in India ” . In Proceedings of national seminar on Sago Sago India 2004 101 – 107 . Thiruvananthapuram: St. Joseph's Press
- van Bel , A.J.E. and Hess , P.H. 2008 . Hexoses as transport sugars in the phloem. The end of a dogma? . Journal of Experimental Botany , 59 : 261 – 272 .
- Walkley , A. and Black , I.A. 1934 . An examination of the Degtjareff method for determining organic carbon in soils: effect of variations in digestion conditions and of inorganic soil constituents . Soil Science , 63 : 251 – 263 .
- Weisburg , W.G. 1991 . 16S ribosomal DNA amplification for phylogenetic . Journal of Bacteriology , 173 : 697 – 703 .
- Widdel , F. and Bak , F. 1992 . “ Gram-negative mesophile sulfate-reducing bacteria ” . In The prokaryote , Edited by: Balows , A. , Truper , G. , Dworkin , M. , Harder , W. and Schleifer , K.H. 3352 – 3378 . Berlin : Springer .