Abstract
Current carbon footprinting (CF) and life cycle assessment (LCA) methods do not treat recycled biogenic carbon adequately, because the calculation rules for recycled products and biogenic carbon stored in products are defined independently from each other. Therefore, an improved and consistent calculation rule for the CF of product systems containing both recycling processes and carbon stored in products is proposed. The methodological approach consists of the application of the same allocation principles for both greenhouse gas (GHG) releases and GHG removals: (1) explicit accounting of inputs (GHG removals) and outputs (GHG releases) of biogenic carbon flows instead of assuming carbon neutrality per se; (2) consistent application of allocation rules for environmental benefits and environmental burdens. It is shown that the different modelling approaches (e.g. polluter pays, conservative or partitioning) lead to different results in LCA and CF calculations, e.g. the GHG emissions of first life cycle of the product system calculated here range between − 1.6 units in the polluter pays approach and 4 units in the conservative approach. It is shown that the currently common modelling is an average approach for primary biogenic material, a worst-case approach for recycled biogenic material and a best-case approach for disposed biogenic material. This paper proposes to improve the currently developed standards for CF by adding a requirement to the goal and scope definition phase that ensures the consistent and transparent documentation, how biogenic carbon removal credits are allocated between life cycles.
1. Introduction
The substitution of fossil carbon with biogenic carbon is one promising strategy to abate climate change and towards a more sustainable development in general. The substitution can either take the form of biofuels, renewable fibres in plastics or bio-based chemicals and polymers. In addition, biogenic carbon has traditional technical applications in wood products (e.g. furniture, floor coverings) or the pulp and paper sectors. Whenever biogenic carbon is stored in products that are not intended for direct use in the feed, food or energy sector, recycling is an option to keep the biogenic carbon longer in the technosphere and to avoid its release back to the atmosphere. Recycled paper is a prominent example of such a case. For the assessment of the environmental performance of materials including materials that store biogenic carbon life cycle assessment (LCA) is the internationally accepted method. In the case that only climate change effects are assessed, carbon footprinting (CF) is applied.
The LCA is nowadays a well-established environmental management tool, and the international standards ISO 14040 and ISO 14044 (ISO Citation14040:2006, ISO Citation14044:2006) represent the stakeholder consensus for its methodological framework (Finkbeiner et al. Citation2007). The use and application of LCA has been growing continuously over recent years (Schnoor Citation2009, Guinee et al. Citation2011). One of the developments that boosted the application of life cycle thinking is CF. The CF quantifies the total set of greenhouse gas (GHG) emissions caused by an organisation, event or product. It is a tool for mitigating climate change by providing fact-based information to producers and consumers about the climate effects of their choices. According to all relevant international standards and guidelines that are currently available or under development, a CF is basically a ‘single-aspect LCA’ (Finkbeiner Citation2009, p. 93).
The scientific relevance and challenges of CF have been summarised recently by Finkbeiner (Citation2009). While the review paper of Finnveden et al. (Citation2009) does address neither the challenge to model recycling systems nor the issue of dealing with carbon storage, both topics were identified by Finkbeiner as practical challenges in CF.
There is extensive literature on the issue of the treatment of recycling processes in general (e.g. Klöpffer Citation1996, Ekvall and Tillman Citation1997, Newell and Field Citation1998, Frischknecht Citation2010) and with regard to biogenic products like wood in particular (e.g. Jungmeier et al. Citation2002, Hischier et al. Citation2005, Werner et al. Citation2007). However, none of these treat the issue of biogenic carbon and especially biogenic carbon credits in multiple life cycles in an explicit and comprehensive way. Werner et al. (Citation2007) give a comprehensive list of allocation problems and procedures to be considered in their paper on the context-specific evaluation of allocation procedures of post-consumer waste wood in attributive product LCA, but they do not address the challenge and need to deal with biogenic CO2 in the allocation of recycled biogenic products at all, because they simply disregarded biogenic carbon in their calculations.
The Confederation of European Paper Industries (Citation2007) published a ‘Framework for the development of Carbon Footprints for paper and board products’ that deals mainly with other aspects of the end-of-life phase like methane emissions and does not address the issue of inter-life cycle allocation of biogenic carbon removals. The American Forest & Paper Association (Citation2011) noted that there are several methodologies that can be applied for systems with recycled content.
In their own study the burden of virgin fibre manufacturing is allocated to recycled fibre based on the fact that the majority of virgin fibre is used subsequently outside of the boundary of the system. As such, when paper recovery rates are increased, the manufacturing footprint is decreased because the burden of manufacturing gets shifted to the recovered fibre. An alternative approach would be to place 100% of the burden on virgin materials, which, as a result, would reduce the burden (or impact) when using recycled fibre. (American Forest & Paper Association Citation2011)
A currently common modelling approach for such product systems can lead to results that seem not only counterintuitive but also not sustainable. An example for such artefacts is revealed by the comparison of paper produced from virgin biomass with recycled paper (Carbon Trust Citation2006). In this example, the paper based on virgin biomass had a lower carbon footprint than recycled paper. Main reason is the fact that the carbon removed from the atmosphere was allocated to the first user who mainly used these renewable sources for production and energy provision while the recycling process is usually operated with fossil energy. It is also confirmed by the review of Jungmeier et al. (Citation2002, p. 292) that ‘… the recycling issue has commonly been solved by the cut-off procedure’.
This modelling result is in conflict with the real-world benefits of paper recycling that are well documented and many, e.g., reducing the energy required for a number of paper products, extending the fibre supply and contributing to carbon sequestration, saving landfill space, reducing water consumption, preserving forests and generating less pollution during manufacturing (Environmental Defense Fund Citation2011, EPA Citation2011).
The International Organization of Standardisation (ISO) is developing an international standard ISO 14067 (ISO/DIS Citation14067:2011) on the carbon footprint of products, and the World Business Council for Sustainable Development (WBCSD) and the World Resources Institute (WRI) develop two standards under their Greenhouse Gas Protocol Product/Supply Chain Initiative: A Product Life Cycle Accounting and Reporting Standard (GHG Citation2011a) and a Corporate Accounting and Reporting Standard: Guidelines for Value Chain (Scope 3) Accounting and Reporting (GHG Citation2011b). These processes reveal some of the challenges to find internationally accepted methods to cover either recycling or carbon storage as a separate issue. However, this paper specifically addresses the situation when product systems contain processes that include both recycling and carbon stored in products, e.g. in biogenic materials.
The standardisation processes show that a consistent modelling framework for recycled biogenic carbon products is necessary, but missing. While the different methods to deal with GHG releases in recycling systems are well established, the proposed expansion of the modelling approach to include GHG releases is rather new. This paper introduces different modelling approaches to deal with recycling systems of biogenic materials. The advantages and disadvantages with regard to robustness and applicability of the different approaches will be discussed. It will be shown that the choice of the modelling approach requires value choices that as a result imply different incentives to the market.
2. Methodology
2.1. Modelling recycling systems in LCA and CF
The life cycle inventory modelling of recycling of materials has been discussed since the 1990s (Klöpffer Citation1996, Ekvall and Tillman Citation1997). In a nutshell, the LCA and CF calculation rules for recycling need to address whether and how the environmental burden of the primary production of a material is shared between the first user and the subsequent users of that material. Recently, Frischknecht (Citation2010) provided an analysis of sustainability and risk perception of different methods and argued that the proposed methodologies can roughly be classified into two main approaches that are used in daily LCA practice: (1) the recycled content approach (also known as the cut-off approach) based on the principle of the first responsibility, and (2) the end of life recycling approach (also known as the avoided burden approach) based on the principle of the last responsibility.
These approaches represent the two extremes, how recycling can be treated in LCA and CF. The recycled content approach means that a product has to carry the full environmental burden of the production of its primary material, even if it is subsequently recycled. Therefore, this approach provides incentives to use recycled materials due to their lower burden, but provides no incentive to develop recyclable products, because there will be no benefit from it. This is different in the end of life recycling approach because there a product gets a benefit if a recyclable material is produced from the end of life product, i.e. it gets a credit. This approach means that the environmental burden of the primary material production does not remain with the original user of this material because this burden is transferred to the subsequent users of the recycled material. This type of modelling provides incentives for recyclable products, but no incentives to use secondary materials. It also comes with the risk that primary materials are used excessively, if the recycling potential is overestimated.
Several approaches have been proposed that sit between these two extremes, i.e. they partition the burden of primary material production between different life cycles. Examples are the approach that the primary material burden is to be shared equally among all life cycles, cascade recycling approaches, economic partitioning approaches or approaches that try to use the material quality as partitioning criterion (EPA Citation1993, Ekvall and Tillman Citation1997, Kim et al. Citation1997, Vogtlander et al. Citation2001). While there is currently no consensus which of these methods is generally most suitable, the different options are widely acknowledged. It is understood that they require value choices with consequences on the result and the incentives derived from the specific LCA or CF calculation.
The existing standards of LCA and CF, e.g. ISO Citation14044 (2006), GHG (Citation2011a), PAS Citation2050 (2011), do not universally prescribe any of these approaches for the treatment of recycling credits. As a consequence, different approaches can be in compliance with these standards, if used in a consistent manner. In practice, the two extreme approaches are typically applied in a vast majority of studies. As the end of life recycling approach appears to be used most frequently, this procedure is discussed as ‘common practice’ in Section 3.1.
It should be noted that no specific methodological treatment of recycling processes for biogenic materials has been proposed yet. All currently discussed approaches focus on the allocation of the environmental burden of the primary production of a material between the first user and the subsequent users. This approach is consistent for non-biogenic materials as the primary production for those comes with net GHG emissions. However, in the case of biogenic materials, primary production often comes with an environmental benefit, if the removal of atmospheric CO2 is larger than the fossil CO2 emissions caused by its production. As a consequence, the CF calculation rules for the recycling of biogenic materials do not need to address the allocation of burdens between life cycles, but need to address how the environmental benefit of the primary production is shared between the first user and the subsequent users of that material. This special feature of materials containing biogenic carbon is not accounted for in commonly applied methods and represents a relevant methodological gap.
2.2. Modelling biogenic carbon in LCA and CF
Currently, two distinct ways exist, how CO2 flows associated with biogenic carbon are modelled in LCA and CF. There is a simplified approach that basically excludes all biogenic CO2 flows from the calculation by assuming that emissions arising directly from biogenic carbon sources are ‘carbon-neutral’, i.e. the amount emitted equals the amount removed from the atmosphere. The alternative approach specifically models and accounts for biogenic carbon removal from the atmosphere during plant growth of, e.g., a tree and the later CO2 release of the wood product during disposal (Rabl et al. Citation2007, van der Voet et al. Citation2010).
Several authors (Rabl et al. Citation2007, Luo et al. Citation2009) and even industry associations (Biotechnology Industry Organization Citation2011) have already argued against the simple exclusion of biogenic carbon from the LCA and CF models and have proposed to include them as individual flows at each stage of the inventory. Some of the reasons given include the better transparency, the fact that biogenic carbon can be transformed into other flows than CO2 (e.g. CH4) or the fact that biogenic carbon can ‘escape’ co-product allocations (Luo et al. Citation2009).
The motivation for this paper is the fact that that no specific methodological treatment of the recycling stage of carbon flows has been proposed yet. The simplified ‘carbon neutrality’ approach excludes the recycling of biogenic carbon by definition. As a consequence, the methodological treatment of recycled biogenic carbon requires explicit modelling of biogenic carbon flows. Therefore, the methodological gap identified in this paper and the approach proposed to deal with it are further arguments for requiring explicit modelling of biogenic carbon flows in LCA and CF.
2.3. Modelling approaches for recycling of biogenic carbon in LCA and CF
The existing LCA and CF literature so far lacks a specific approach to include the recycling of biogenic carbon stored in products, especially with regard to the distribution of GHG removals from the atmosphere. In the absence of an explicit treatment, both the removal of the biogenic carbon from the atmosphere and the subsequent release of biogenic carbon during the end of life phase of the product are often implicitly attributed by 100% to the first life cycle. The central hypothesis of this paper is that for a consistent LCA and CF method, the same allocation principles have to apply for both burdens (GHG releases) and benefits (GHG removals). That means that if burdens are shared between life cycles, also benefits need to be shared between them.
A common practice to deal with biogenic carbon recycling assumes that all burdens and benefits are borne by the first life cycle. This treatment is technically similar to the first responsibility recycling approach for non-biogenic materials, even though for biogenic materials the burden of the associated GHG release may also be attributed to the last life cycle. For non-biogenic materials, this method allocates all burdens of primary production to the first user. While these approaches are technically the same, the value choices and incentives involved are quite opposite when it comes to benefits rather than burdens. While this approach is rather a worst-case approach for burdens, because the first user cannot transfer them to the following life cycles, it is close to a best-case approach for benefits, because the first user keeps the full benefit of the biogenic CO2 removal without sharing it with the subsequent users. If applied explicitly, it can still be a consistent approach to deal with recycled biogenic carbon.
If the substitution method for non-biogenic materials is transferred to biogenic materials, the result is exactly the opposite. While the credit for the provision of sources for secondary materials can be seen as a best-case modelling for burdens, it is a worst case from the perspective of benefits. If this approach is applied to biogenic materials, the benefit to remove CO2 from the atmosphere does not remain with the first user, but is transferred to the subsequent users. This situation is rather challenging, because it means that if preference is given to the same technical treatment of burdens and benefits, it is inconsistent from a value and incentive perspective. If the modelling is done from a consistent value perspective, it requires different technical approaches to model biogenic and non-biogenic materials.
Any of the approaches developed for the treatment of recycled products between these two extremes as described in the relevant section above can, in principle, be applied to biogenic materials as well, but the implications are different and need to be interpreted with proper caution. In the result section, several examples are presented to demonstrate the effect that choosing different modelling options may have.
Even though the resulting LCA and CF models get more complex, it is necessary to model the recycling of biogenic carbon contained in products in an explicit and consistent way. If burdens are shared between life cycles, also benefits need to be shared between life cycles. It is therefore proposed in this paper that the approaches developed for non-biogenic materials can be technically used to model the biogenic materials as well. However, the LCA and CF practitioners have to acknowledge that the implications of these approaches for benefits are different, in cases opposite compared to its original application on burdens. As a consequence, the recycled content approach that puts a strong responsibility on the first user gets rather a ‘first come, first serve’ approach for biogenic materials. The avoided burden approach for non-biogenic materials can be seen as a ‘lost benefit’ method for the first user of biogenic materials.
3. Results and discussion
In this section, exemplary results for the different modelling approaches for recycling of biogenic carbon are presented to demonstrate the relevance and implications they have on the application LCA and CF for decision-making support. The common practice of not explicitly modelling the recycling of biogenic carbon is compared with the different options developed in this paper to include this feature in LCA and CF calculations.
The results are presented by using a schematic case study with exemplary data rather than a real case study. This is done by intention to put focus on the method rather than a particular case study. However, the schematic example chosen is based on a real case study to ensure that the data represent feasible orders of magnitude for the different GHG flows shown. Any losses of biogenic material between the life cycles have been excluded for simplicity reasons. For all the results shown below, it is assumed that the example product leads to the GHG removals and releases shown in Table .
Table 1 Schematic data in exemplary amounts for the example product.
It is assumed that the biogenic material contained in the exemplary product has removed 2.8 kg CO2e from the atmosphere during its growth. If the product is finally disposed, the same amount of biogenic carbon is released back to the atmosphere (assuming that no conversion from biogenic CO2 to CH4 occurs in this case). The GHG emissions from the primary production process, the recycling process and the disposal process are assumed to be 1.2 kg CO2e, 1.9 kg CO2e and 0.9 kg CO2e, respectively. These GHG emissions are all non-biogenic in nature (assuming no biomass-based energy is contained in the underlying energy mix). In reality, there is a broad range of biogenic products, so the actual amounts and quantitative relations between the process steps could be quite different, e.g. for another biogenic product the GHG emissions caused by primary production might be higher compared to those originating from recycling. However, this would only change the absolute and relative results of the example presented below, but not the methodological concept and conclusions of the paper, because they are valid in general and do not depend on a particular case. The example case has been intentionally defined with the simplifying assumptions documented above, because the paper does not provide results on a particular case study, it just uses the exemplary case to illustrate the relevance and consequences of the methodological amendment proposed.
3.1. Common practice
The removal of the biogenic carbon from the atmosphere and the subsequent release of biogenic carbon are commonly attributed by 100% to the first life cycle. Figure shows the resulting distribution of the GHG emissions between the different life cycles of the product. In addition to the biogenic carbon removal and release, the first life cycle carries the GHG emissions from the primary production process. The second and third life cycles (as examples for life cycles that keep the biogenic carbon in the technosphere by recycling) only carry the GHG emissions from the recycling process, because no biogenic carbon is accounted for in this approach. The last life cycle (indicated as xth life cycle) that terminates the recycling loops and leads to the final disposal of the product gets the GHG emissions from the disposal process in addition to the recycling emissions.
Figure 1 Distribution of GHG emissions and removals over the life cycles for the common practice approach.
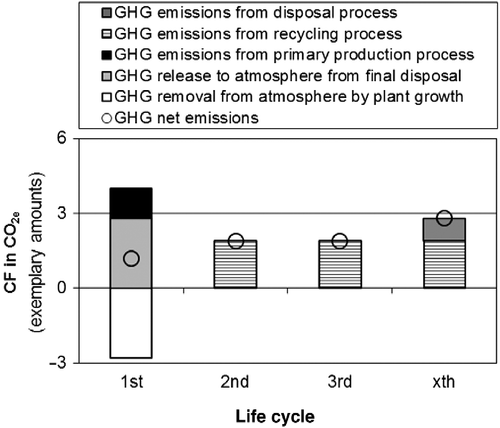
The resulting net GHG emissions (indicated by the circles) show the case described earlier for paper products, that by the common modelling practice the virgin product may get a lower environmental burden than the recycled one. Therefore, this accounting method leads to incentives to use biogenic materials, but it does not provide incentives to recycle them. It just provides incentives for improved recycling efficiency. The following sections detail and discuss alternative methods to this common accounting practice.
3.2. Polluter pays approach
The polluter-pays approach basically allocates the removals and releases to the life cycles in which they actually occur. The resulting life cycle distribution is shown in Figure . The only difference compared to common practice is that the GHG release to the atmosphere is allocated to the last life cycle, because this is the life cycle that leads to the release in reality.
Figure 2 Distribution of GHG emissions and removals over the life cycles for the ‘polluter pays’ approach.
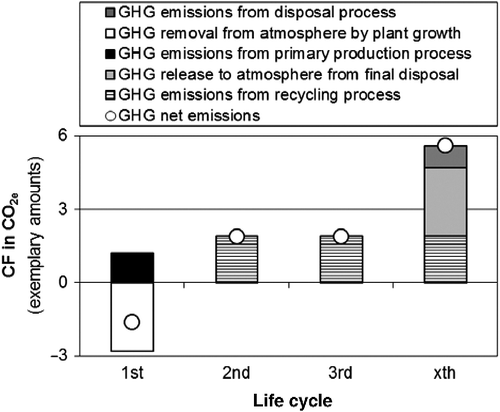
As a result, the burden of the first life cycle is even lower than in the common practice approach and even comes with a ‘negative’ emission value. At the other end of the scale, the last life cycle gets the highest burden in this approach. It could be argued that this approach provides incentives for both the use and the recycling of biogenic carbon. However, this favourable modelling of the first use of biogenic materials could actually lead to its overuse beyond a sustainable, renewable rate.
3.3. Conservative approach
An approach that is rather conservative with regard to incentives for using biogenic material does not focus on the real-time releases and removals of GHG, but the causes for them. A conservative approach does not look at the life cycle in which the emissions and removals actually happen, such an approach would rather allocate based on the responsibilities. In that sense, the first life cycle has to bear the emissions of the biogenic carbon, because the fact that these are released at some point is already generated by the first use. The credits are transferred from one recycling life cycle to the next, because the recycling is responsible for keeping the biogenic carbon in the technosphere.
The result shown in Figure shows that this approach indeed reduces the incentives to use biogenic materials significantly, while recycled biogenic carbon gets a lower burden than the first life cycle. For applications that require a risk-aversive attitude, this approach is consistent. On the other hand, the resulting level of use of biogenic materials might get too low and not the full potential of beneficial, sustainable use of biogenic materials might be realised.
3.4. Partitioning approaches
The current practice, the polluter pays and the conservative approaches are similar in the sense that they allocate the total amount of removals and releases of biogenic carbon to individual life cycles. They just differ in the choice of the life cycles that have to bear the releases or benefit from the removals. Between these extremes, different forms of partitioning approaches are possible. While they can differ in the choice of what share is allocated to the life cycles, they are similar in the sense that they do not allocate the total amounts to specific life cycles, but that they partition the total amount between life cycles.
The example shown in Figure partitions the biogenic carbon removals and releases equally between all life cycles. This leads to the result, that – in the case of the four life cycles shown – the first life cycle performs better than the second and third. Several variants to this example are possible. A more conservative approach may just partition the benefits, but not the burdens. Another variant could do the partitioning just between the recycling cycles, in this case the second and third life cycles, instead of all life cycles.
Figure 4 Distribution of GHG emissions and removals over the life cycles for a partitioning approach.
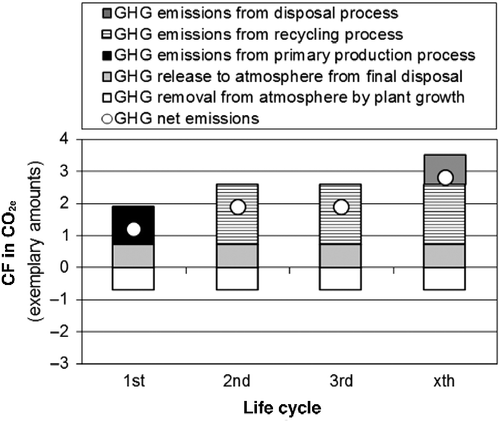
All these approaches suffer from the difficulty that the total number of life cycles, i.e. recycling cycles, needs to be known for the calculation of burdens and releases for specific life cycles. Another feature is that the resulting shares for each individual life cycle may get negligible in the case of many life cycles.
3.5. Comparison of results based on different modelling approaches
The results shown above clearly demonstrate that the different modelling approaches lead to significantly different CF and LCA results for the different life cycles of product systems containing recycling processes of biogenic carbon. Figure shows an overview of the ranges obtained. For the first life, i.e. primary biogenic material, the range is the largest with resulting net CF values from − 1.6 units in the polluter pays approach up to 4 units in the conservative approach. For recycled biogenic materials the range is smaller, but still covers negative values from − 0.9 units up to positive values of 2.6 units of net GHG emissions. The life cycle in which the material is disposed of gets only positive values, i.e. burdens, between 2.8 und 5.6 units.
Figure 5 Ranges of resulting net GHG emissions for the different modelling approaches for primary, recycled and disposed biogenic material; circles indicate values of the common modelling.
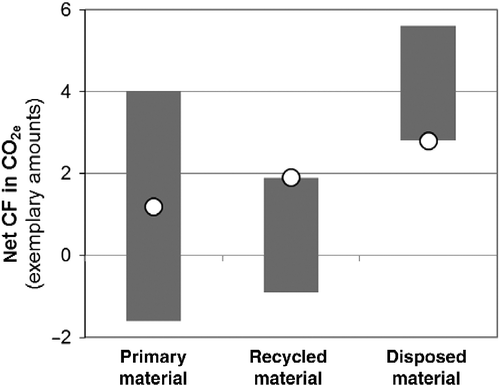
Figure shows another interesting feature, if the position of the white circles (that indicate the net GHG values resulting from the currently common modelling approach) is analysed with regard to its position within the possible ranges for each of the life cycles. While the common practice value for the primary material is almost in the middle of the range of the first life cycle values, the situation is quite different for the recycled and disposed material. For recycled material, the common modelling approach represents the upper limit of the range of GHG emissions, while it is the opposite for disposed material, i.e. the values are at the lower end of the possible range. This observation supports to the conclusion that the current modelling practice is not capable of modelling recycled biogenic products in a consistent and adequate manner stressing the main hypothesis of this paper that proper modelling decisions for these products are still needed.
4. Discussion
The intention of this paper is to introduce the methodological concept that biogenic carbon credits have to be allocated between different life cycles in an explicit way – just like it is an established practice for GHG releases. It is not the intention of this paper to address a specific case study or material – therefore a schematic case with arbitrary units was chosen to illustrate the approach. For real case studies, the specific results are determined by many parameters like recycling efficiency, recycling in different products, loss of quality in recycling process, carbon storage, geographic location and energy mix, etc. Therefore, the results shown in the schematic example might look quite different for particular cases, e.g. if the recycling of the biogenic product occurs in a country with a low carbon footprint electricity mix. However, these issues need to be addressed by the practitioner in the goal and scope definition like in any case study. The approach proposed here does not have any influence on these general goal and scope elements. However, it is proposed in this paper to add in the goal and scope definition explicitly and consistently, how biogenic carbon credits are treated between life cycles.
This paper challenges the common modelling approach of CF and LCA studies of product systems that contain biogenic carbon, because it can lead to wrong incentives for sustainable production and consumption. A consistent modelling framework for recycled biogenic carbon is proposed that consists of two elements: (1) explicit accounting of inputs and outputs of biogenic carbon flows instead of assuming neutrality per se, and (2) consistent application of allocation rules for removal benefits and release burdens. This modelling framework is based on the assumption that if environmental burdens are shared between life cycles, also environmental benefits need to be shared between them. While the sharing of burdens represents currently established practice, the sharing of benefits does not. This is inconsistent and needs to be improved in the methodological literature of LCA and CF. It should also be included in the currently developed standards for CF.
The paper introduces different modelling approaches (polluter pays, conservative approach and partitioning approaches) to deal with recycling systems of biogenic materials. There is unfortunately no general or unambiguous preference for any of these because each approach represents different perspectives and implies different incentives. The selection of the approach best suited for a particular study has to be done in the goal and scope definition phase based on the target and application of the specific study. Like with other allocation challenges in LCA and CF, there is no clear scientific superiority of any of the approaches; the decision between them represents a value choice. If the interpretation step of a particular study reveals that the chosen approach is a significant parameter for the results, sensitivity analyses with alternative allocation solutions should be performed to determine the robustness of the results for this particular study.
Even though value choices are the least preferred option in CF and LCA methodology, according to the principle ‘priority of scientific approach’ defined in ISO Citation14040 (2006), a well-justified and documented value choice is still better than simply ignoring the relevance of an explicit treatment of recycled biogenic carbon in CF and LCA studies. The relevance of this topic for the results of LCA and CF studies of product systems containing biogenic carbon has been demonstrated above.
The topic of this paper has been introduced with the comparison of virgin and recycled biogenic products, but it is obviously just as relevant for comparing biogenic and non-biogenic products. In such cases, the assessment typically focuses on the first product life cycle only or – if there are particular production pathways for secondary products – the second life cycle. For these applications, the LCA practitioner should pay close attention to the potential bias introduced by the modelling of biogenic carbon. A comprehensive comparison should assess all life cycles of a material and not just the first or second one. If such material comparisons focus on one life cycle only, the results may rather reflect the modelling approach taken than the performance of the material.
To avoid such methodological artefacts, it is proposed here to include specific requirements into the currently developed standards and guidelines on CF that the inter-life cycle partitioning of biogenic carbon needs to be treated explicitly, consistently and transparently. As it is a value choice, it is premature to fix a particular approach in the standards, but the standards should require that the approach chosen is documented and justified as well as subject to sensitivity analyses. Future research should try to expand the fact-based domain on this topic to reduce the need for value choices. Apart from explicit case studies and the assessment of incentives of the different approaches, one interesting feature could be to look into multi-recycling systems that try to model the environmental interventions caused by all life cycles of a material rather than using data for any arbitrarily chosen life cycle of that material. Such an approach can be seen as a special form of system expansion as the only way to avoid the partitioning between the life cycles. In the absence of subdivided product systems, the sharing of burdens and benefits is obviously no longer applicable.
References
- American Forest & Paper Association, 2011. Printing & writing papers – life-cycle assessment summary report [online]. Available from: http://www.afandpa.org/PWLCAMaterials/ [Accessed 27 July 2011]
- Biotechnology Industry Organization, 2011. Biotechnology Industry Organization position carbon footprint [online]. Available from: http://bio.org/ind/renewbiobased/Position_Carbon_Footprint_PCF.pdf [Accessed 8 January 2011]
- Carbon Trust . 2006 . Carbon footprints in the supply chain: the next step for business , London : Carbon Trust .
- Confederation of European Paper Industries, 2007. Framework for the development of carbon footprints for paper and board products [online]. Available from: http://www.ncasi.org/support/downloads/documents/CEPI_Carbon_Footprint_report_2007.pdf [Accessed 27 July 2011]
- Ekvall , T. and Tillman , A.M. 1997 . Open-loop recycling: criteria for allocation procedures . International Journal of Life Cycle Assessment , 2 : 155 – 162 .
- Environmental Defense Fund, 2011. FAQs: environmental benefits of recycled paper [online]. Available from: http://www.edf.org/page.cfm?tagid = 24437 [Accessed 27 July 2011]
- EPA . 1993 . Life-cycle assessment: inventory guidelines and principles , Washington, DC : United States Environmental Protection Agency . EPA/600/R-92/245
- EPA, 2011. Wastes – resource conservation – common wastes & materials – paper recycling [online]. Available from: http://www.epa.gov/osw/conserve/materials/paper/basics/ [Accessed 27 July 2011]
- Finkbeiner , M. 2007 . The new international standards for life cycle assessment: ISO 14040 and ISO 14044 . International Journal of Life Cycle Assessment , 11 : 80 – 85 .
- Finkbeiner , M. 2009 . Carbon footprinting – opportunities and threats . International Journal of Life Cycle Assessment , 14 : 91 – 94 .
- Finnveden , G. 2009 . Recent developments in life cycle assessment . Journal of Environmental Management , 91 : 1 – 21 .
- Frischknecht , R. 2010 . LCI modelling approaches applied on recycling of materials in view of environmental sustainability, risk perception and eco-efficiency . International Journal of Life Cycle Assessment , 15 : 666 – 671 .
- GHG, 2011a. World Resources Institute & World Business Council for Sustainable Development: GHG Protocol Product Accounting and Reporting Standard, Draft for Stakeholder Review [online]. Available from: http://www.ghgprotocol.org/files/ghg-protocol-product-standard-draft-november-20101.pdf [Accessed 28 January 2011]
- GHG, 2011b. World Resources Institute & World Business Council for Sustainable Development: GHG Protocol Corporate Value Chain (Scope 3) Accounting and Reporting Standard, Draft for Stakeholder Review [online]. Available from: http://www.ghgprotocol.org/files/ghg-protocol-scope-3-standard-draft-november-20101.pdf [Accessed 28 January 2011]
- Guinee , J.B. 2011 . Life cycle assessment: past, present, and future . Environmental Science & Technology , 45 : 90 – 96 .
- Hischier , R. , Althaus , H.J. and Werner , F. 2005 . Developments in wood and packaging materials life cycle inventories in ecoinvent . International Journal of Life Cycle Assessment , 10 : 50 – 58 .
- ISO 14040 . 2006 . Environmental management – life cycle assessment – principles and framework , Geneva : ISO .
- ISO 14044 . 2006 . Environmental management – life cycle assessment – requirements and guidelines , Geneva : ISO .
- ISO/DIS 14067 . 2011 . Carbon footprint of products – requirements and guidelines for quantification and communication , Geneva : ISO .
- Jungmeier , G. 2002 . Allocation in LCA of wood-based products; experiences of Cost Action E9; part I. methodology . International Journal of Life Cycle Assessment , 7 : 290 – 294 .
- Kim , S. , Hwang , T. and Lee , K.M. 1997 . Allocation for cascade recycling system . International Journal of Life Cycle Assessment , 2 : 217 – 222 .
- Klöpffer , W. 1996 . Allocation rule for open-loop recycling in life cycle assessment – a review . International Journal of Life Cycle Assessment , 1 : 27 – 31 .
- Luo , L. 2009 . Allocation issues in LCA methodology: a case study of corn stover-based fuel ethanol . International Journal of Life Cycle Assessment , 14 : 529 – 539 .
- Newell , S.A. and Field , F.R. 1998 . Explicit accounting methods for recycling in LCI . Resources, Conservation and Recycling , 22 : 31 – 45 .
- PAS 2050 . 2011 . Specification for the assessment of the life cycle greenhouse gas emissions of goods and services , London : British Standards Institution .
- Rabl , A. 2007 . How to account for CO2 emissions from biomass in a LCA . International Journal of Life Cycle Assessment , 12 : 281
- Schnoor , J.L. 2009 . LCA and environmental intelligence? . Environmental Science & Technology , 43 : 2997
- van der Voet , E. , Lifset , R.J. and Luo , L. 2010 . Life-cycle assessment of biofuels, convergence and divergence . Biofuels , 1 : 435 – 449 .
- Vogtlander , J.G. , Brezet , H.C. and Hendriks , C.F. 2001 . Allocation in recycling systems – an integrated model for the analyses of environmental impact and market value . International Journal of Life Cycle Assessment , 6 : 344 – 355 .
- Werner , F. , Althaus , H.J. , Richter , K. and Scholz , R.W. 2007 . Post-consumer waste wood in attributive product LCA – context specific evaluation of allocation procedures in a functionalistic conception of LCA . International Journal of Life Cycle Assessment , 12 : 160 – 172 .