Abstract
The use of solar energy as a disintegration unit operation for waste activated sludge was tested in a pilot plant. The effect of the treatment process on chemical oxygen demand (COD), total suspended solids (TSS), settling time and filtration index was investigated in single (solar) and sequential processes (solar & biological). Solar oxidation was found to be a good sludge disintegration technology. Available solar photons were utilised to degrade the soluble organic matter and release the cell liquids. The highest COD and TSS removal efficiencies were obtained by the month of June. Sequential process efficiencies were related to accumulate solar photons, aerobic treatment time and operational condition. Sequential process resulted in COD and TSS removal efficiency of 16% and 13%, respectively. Increasing aerobic treatment time and/or mixing the oxidised sludge with fresh sludge improved COD and TSS removal efficiencies to 23% and 27%, respectively. The use of sequential processes improves the settling tendency of sludge by bio-oxidation of the released portions and eliminates protein charge effect on sludge settling.
Nomenclature
WAS | = |
Waste activated sludge |
SDT | = |
Sludge disintegration technologies |
COD | = |
Chemical oxygen demand (mg/L) |
TSS | = |
Total suspended solids (g/L) |
SFI | = |
Specific filtration index (s/g) |
SVI | = |
Sludge volume index (mL/gTSS) |
Q | = |
Flow rate (L/s) |
A | = |
Area of filter media (m2) |
c | = |
Feed concentration (g/L) |
R | = |
Resistance filter medium |
Δp | = |
Pressure drop (Pa) |
α | = |
Specific cake resistance (m/kg) |
μ | = |
Viscosity of filtrate (Ns/m2) |
V | = |
Filtrate volume (L) |
t | = |
Time (s) |
t s | = |
Time elapsed (s) |
V s | = |
Filtrate volume when feed pressure becomes constant (L) |
1. Introduction
Waste activated sludge (WAS) is produced in abundant quantities during secondary treatment of wastewater. Although a major part of this sludge is recycled to the biological reactor, part of this sludge should be treated and disposed of properly. Landfill and ocean dumping were successful disposal methods. Nevertheless, the production of secondary pollutants and the diffusion of pollutants through the ecosystem motivate different countries to restrict these methods (Nowak Citation2006). Other sludge treatment processes were also proposed such as ultrasonic treatment, ball milling process, homogeniser treatment, thermal decomposition and enzymic treatment (Dichtl et al. Citation1997, Sakai et al. Citation1997, Barjenbruch and Kopplow Citation2003, Xuan et al. Citation2004, Yoon et al. Citation2004, Manterola et al. Citation2008, Wawrzynczyk et al. Citation2008, Feng et al. Citation2009, Yamashita et al. Citation2010). However, the applicability of these methods was limited due to different reasons: (1) they were considered complicated treatments, (2) expensive treatment cost, (3) needs for high energy and (4) needs for special material.
A recent successful solution that was proposed to deal with excess WAS is sludge disintegration technologies (SDT) (Ray et al. Citation1990, Weemaes et al. Citation2000, Goel et al. Citation2003, Yeom et al. Citation2003). SDT aim to break WAS into smaller materials that can be treated by conventional treatment (e.g. aerobic reactor). In this context, the objective of SDT is to hydrolyse solids and to reduce excess biomass production (Sakai et al. Citation1997).
Several chemical processes can be used as SDT but the selection of any process will be based on different factors, namely strong degradation capacity, short reaction time, normal conditions and safe by-products. Among various proposed approaches, advanced oxidation processes (AOPs) were most widely proposed and adopted in many commercialised processes (Muruganandham et al. Citation2007, Chu et al. Citation2008, Tian et al. Citation2009, Zhang et al. Citation2009). AOPs have strong oxidising capacities and react with inorganic and organic compounds via the formation of hydroxyl radicals. They preferentially oxidise electron-rich molecules containing carbon–carbon double bonds and aromatics. AOP as treatment splits the long-chain compounds making them biodegradable. AOPs are advantageous because: (1) they are destructive processes that do not transfer contaminants from one phase to another, (2) oxidation reaction is very fast, (3) they can be conducted at ambient temperatures and (4) the primary catalysts (e.g. iron and titanium) are non-toxic, readily available and relatively inexpensive.
Despite the fact that AOPs are attractive treatment, these processes are expensive. Different solutions were proposed to minimise the treatment cost. These solutions involve catalyst recycling, integration of chemical and biological processes and the use of renewable energy (e.g. sunlight). The combination of AOP and bio-treatment was demonstrated to be an effective method for treating a wide variety of pollutants (Erden et al. Citation2010, Mohapatr et al. Citation2010, Pham et al. Citation2010). Thus, oxidation of WAS can facilitate sludge treatment in bio-reactor (Kamenev Citation2003, Bierbaum and Oller Citation2005, Carballa et al. Citation2007, Chu et al. Citation2008, Mohapatra et al. Citation2009). Catalyst recycling was also studied in different occasions, results are promising but do not reduce the treatment cost significantly.
Several studies were conducted to examine the use of solar photo-oxidation for water pollutants (Malato et al. Citation2000, Amat et al. Citation2005, Munoz et al. Citation2005, Andreozzi et al. Citation2006, Milena et al. Citation2006, Mohapatra et al. Citation2009). These studies were carried out to remove one specific pollutant. The results showed that the effectiveness of oxidation processes depended on reactant concentration, temperature, pH, solar intensity and water matrix. The literature review did not reveal any work that elaborated on the possibility of using solar energy in treating WAS.
Accordingly, the present work was conducted to follow up the treatment of WAS by the solar pilot plant. This study also aimed to follow up the behaviour of organic matter during the oxidation process and to assist WAS behaviour in sequential processes (AOP and aerobic treatment). Moreover, the effect of oxidation process on sludge characteristics such as settling ability, specific filtration index (SFI) and sludge volume index (SVI) was investigated.
2. Material and methods
2.1 Solar experiments
The experimental set-up used in this study is presented in Figure . The set-up consisted of (1) the influent tank (25 L) and (2) the solar reactor that consisted of (a) the lower header, (b) the upper header and (c) 20 solar elements. The elements were made of a low iron quartz tube with an inside diameter of 5.8 cm and a length (L) of 180 cm, (3) the effluent tank (25 L) and (4) the circulation pump.
2.2 Aerobic reactor
The aerobic digestion was carried out in a 25 L aerobic reactor, which is supplied with an agitator and air supply. The air-flow rate was controlled within a range of 8–10 L/h. The digestion was carried out at room temperature and in the pH range of 6.8–7.2. When required, the pH was adjusted by NaOH or H2SO4.
2.3 Experimental procedure
2.3.1 Sludge oxidation and bio-treatment
All the trials were conducted at Mutah University (Jordan) latitudes 29° 30′ and 34° N as batch experiments according to the following procedure: the sludge from the wastewater treatment plant was stabilised for 24 h in external aerobic reactor. Then, a volume of 22 ± 1 L of sludge was charged to the influent tank. The circulation pump was switched on and the oxidation reaction proceeded for an elapsed time of 2–4 h, the specific retention time was in the range of 75—150 mL/min. After the first oxidation step, the oxidised sludge was diverted to aerobic reactor and aerated for certain time (24, 48 or 96 h). The same procedure was performed for subsequent oxidations and biological aerations. Each biological oxidation step was carried out three times; the results presented are the average of these values validated at 95% confidence level.
2.3.2 Sludge oxidation and bio-treatment with mixing effect
A volume of 20 L of sludge was oxidised by solar irradiation to a certain time (2 or 4 h), then was mixed with fresh activated sludge in a volume ratio of 3:1 (i.e. 15 L oxidised sludge with 5 L of fresh sludge). The sludge mixture was then charged to biological reactor and aerated for a specific period of time (24 or 48 h) after which 20 L of the sludge was oxidised with solar energy for the same time (i.e. second oxidation stage). A similar procedure was used for subsequent steps.
2.4 Analytical methods
Chemical oxygen demand (COD ± 0.3 mgO2 L− 1) was measured following the standard method. Measurement was carried out via a photometer (Hach Company, Loveland, Colorado, USA) using a dichromate solution as an oxidant in strong acidic media. All COD tests were carried out on water samples filtered with Whatman® Grade 4 cellulose filters.
The total suspended solids (TSS) were accomplished following Standard Methods (APHA-AWWA Citation1992).
The sludge settling was correlated with the SVI. The sludge settlement was followed by measuring the volume in millilitres (mL) occupied by 1 g of a suspension after 30 min settling.
Protein quantification was carried out by the use of inductively coupled plasma optical emission spectrometry.
2.5 Specific filtration index
The specific filtration index (SFI) was measured in a pressure filtration device. The set-up and procedure used were adapted from previous work (Al Momani et al.
Citation2010). Classical filtration theory by Svarovsky (Citation1981 p. 242) was used to model the oxidised SFI. The general filtration equation is given by Equation (Equation1)
Equation (Equation1) can be rewritten in reciprocal form as
For practical purpose, it is necessary to avoid high initial flow rates through a clean medium to prevent the penetration of solids through the filter media as well as to ensure an even deposition of the cake. Accordingly, it is recommended to precede the constant pressure period with a gradual increase in applied pressure (this may be a nearly constant rate period). At constant Δp, Equation (Equation2) can be integrated from a point (t
s,V
s) at the beginning of the truly constant pressure period to final values (t, V): the resulting Equation is (Equation3
)
In batch experiment, the values of t
s, V
s and R can be considered equal to zero. Equation (Equation3) can be reduced to
To eliminate the dependence on TSS, the filtering value can be converted to the specific filtration index (SFI),
According to the SFI value, higher SFI values indicate lower filterability. Combining Equations (Equation4) and (Equation5
) leads to
Equation (Equation6) can be reduced to
3. Results and discussion
3.1 Single sludge solar oxidation
Figure presents the evolution of COD during solar oxidation of sludge as a function of time during different months of 2009 (March, June, September and November). All the experiments were carried out three times under clear sky conditions (error bar on Figure was calculated based on 95% CI). The trends indicate that the chemical content (measured by COD) of sludge (C i ≈ 380 mg/L) was affected by solar oxidation: at the beginning of oxidation COD increased progressively reaching a maximum value (485 mg/L for June experiment), after that the COD value decreased to 347 mg/L for the same conditions. The observed tendency was aimed to the destruction of sludge cell boundaries by means of solar photons, and elimination of cell membrane led to the release of cell liquids. These liquids are known to have high organic matter contents, which make the COD of the solution increase. Proceeding with oxidation, intercepted photons were used to oxidise the released cell liquids. Accordingly, COD value started to decrease.
Figure 2 Evolution of COD for WAS as a function of solar oxidation time in different months. Inner: monthly average solar radiations during 2009.
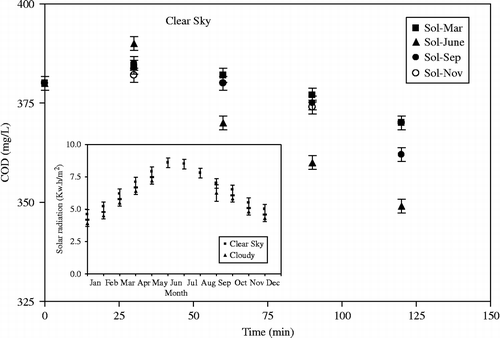
The maximum decrease in COD (i.e. highest cell disintegration) of 8.5% was achieved for the experiment carried out in June (Sol-Jun). On the other hand, moderate COD reduction of 5.7% and 5.4% was observed in March (Sol-Mar) and September (Sol-Sep), respectively. The minimal COD reduction of 2.3% was obtained in the experiments carried out in November (Sol-NOV). Similar oxidation tendencies were observed for experiments carried out in different months; a COD removal of 2 and 2.1 was achieved in January and December, respectively (results not shown).
Although the percentage COD removal from the sludge appears to be very low, the objective of solar oxidation is to disintegrate sludge cells rather than to mineralise the organic matter. One parameter to indicate cell disintegration is the increase in filtrated solution COD as an indication of WAS cell rapture, after that the sludge can be diverted to less expensive process (e.g. biological treatment) for further treatment. As it can be seen in Figure , the COD increased for all the studied months during the first 40 min of oxidation. Thus, the oxidation process can be cut off at this point and the sludge can bio-oxidise in subsequent biological treatment.
The differences in oxidation efficiency can be related to the monthly average solar radiations for 2009 (inserted Figure ). The measured solar radiation for all months was higher than 2.7 kWh/m2 with the highest value occurring in June (8.6 kWh/m2).
Another parameter proposed in the literature to measure solar radiation intensities is Q UV,1/2, defined as the accumulated energy required to reach half of initial concentration (Malato et al. Citation2000). Similarly, in this work, this parameter was calculated for the time needed for concentration (COD) to reach a constant limit. Values of this parameter were found to be 32.5, 34.7 and 85 kJ/l for Sol-Nov, Sol-Mar and Sol-Jun, respectively. The difference between these values explained the obtained degradation trend by solar radiation in these months.
Another set of experiments was carried out for cloudy sky conditions. Unexpectedly, the degradation efficiency on cloudy days was higher than the degradation on clear sky days. In an attempt to explain unexpected results, the average radiation energies for cloudy days were measured and found to be lower on cloudy days. The unexpected results were related to the difference in intercepted light regimes; intercepted irradiations on cloudy days contain a large portion of short wavelength light (i.e. ultraviolet light (UV)) than clear sky days, more specific measurement of these radiation beams will be presented in future works.
The reduction in TSS was also measured during each experiment (see Figure ). It was observed that the amount of TSS decreased as a function of oxidation reaction time. As an average, the TSS content of the solution was decreased by 2.8%. The pre-treatment step reducing the TSS by this amount is considered significant as other treatments do not reduce the TSS content of the solution but only eliminate the organic matter content of the solution.
3.2 Sequential solar oxidation & biological treatment
Oxidised sludge was diverted to aerobic biological reactor and aerated for specific time. Table summarises the experimental conditions for the studied sequential processes.
Table 1 Operational conditions of sequential solar oxidation & biological treatment processes.
Figure illustrates the evolution of COD and per cent COD removal of the different processes presented in Table (results are average of three experiments under the same conditions). The sludge disintegration was dependent on the hydraulic retention time (HRT) of both biological treatment and solar oxidation.
Figure 4 Evolutions of COD and % COD removal during sequential solar oxidation & biological treatment (a) SQ1, (b) SQ2 and (c) SQ3.
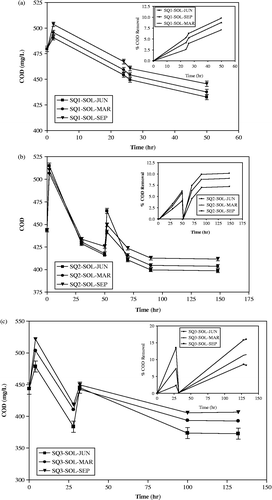
The effect of biological treatment HRT can be observed in Figure and b. There is a noticeable difference in the slope of bio-degradation line between SQ1(HRT = 24 h) and SQ2 (HRT = 48 and 96 h). The difference in slope was related to biomass uptake capacity. Experiments carried out with low HRT showed a small percentage of COD removal, meanwhile high HRT showed a high percentage of COD removal. For experiments carried out under SQ1 conditions the low biological treatment capacity (oxidation time 24 h) resulted in partial removal of organic matter from the solution (low COD reduction), the presence of low molecular weight organic matter in the solution affected the subsequent solar oxidation steps as most of the intercepted solar oxidation was used to oxidise the organic matter rather than disintegrating new cells. Consequently, the overall efficiency of the process decreased. Different tendencies were observed for experiments carried out under SQ2 conditions; allowing sufficient time for bio-oxidation resulted in more cell disintegration and an increase in the efficiencies of removal. The difference in oxidation capacity was clear during second oxidation step where the increase in COD of the solution for SQ2 was 10 times higher than the SQ1 experiments. The tendency can be explained by the fact that during SQ1 experiments most of the solar irradiations were used to oxidise the by-products resulting from cell disintegration rather than attacking new cells, meanwhile during SQ2 experiments the solutions had no remaining by-products and most of the intercepted radiations were used for new cells disintegration.
On the other hand, it was observed in SQ2 experiments that most of the organic matters were eliminated within HRT of 48 h, which suggests that an optimum HRT of 48 h is recommended for efficient biomass uptake.
The effect of solar irradiation time on sludge disintegration was studied in SQ3 experiments. An increase in the solar oxidation time led to an increase in the efficiency of sludge disintegration. This was shown by raising the COD value throughout the solar oxidation. Increasing the irradiation time from 2 to 4 h led to an increase in COD removal efficiency by a factor of 3; for SQ1 with irradiation time of 2 h, per cent COD removal ranged from 2.20% to 4.8%, while in SQ3 (irradiation time = 4 h) the per cent COD removals were in the range of 8–15%. Obtained results indicated improvement on oxidation capacity of SQ3 compared with other processes.
3.3 Sludge mixing effect
Another set of experiments was performed by mixing the oxidised sludge with non-oxidised sludge (see experiments SQ4 in Table ).
Table presents the per cent COD removal and per cent TSS reduction for SQ4 set of experiments. For all the operational conditions sludge mixing strategy enhanced both COD and TSS removal percentage; COD and TSS reduction for March (SQ4-SOL-MAR) experiments were found to be 16% and 20%, respectively. Maximum COD and TSS removal efficiencies were found to be 23% and 27%, respectively in June (SQ4-SOL-JUN). This enhancement can be related to SDT, which produces organic biodegradable materials. These organic materials can be used biologically by fresh sludge. It was mentioned previously that oxidation broke up the cell structure, which makes cell ingredients more available for bio-degradation; as a result, removal efficiency increased. Other experiments were carried out in other months (January, February and October), results not shown. Although the radiations during these months are low the SDT still give promising results; the per cent COD removal during these months ranges from 13% to 15.5%.
Table 2 Experimental results obtained during sequential WAS oxidation.
3.4 Settling effect
The effect of the solar oxidation process and sequential processes on the sludge settlement was investigated. The fraction of sludge settled during a 30-min settling time for different processes is presented in Table . The settling efficiency was highly dependent on the degree of sludge oxidation. High settling ability was observed for sludge at low degree of oxidation. Oxidation of sludge leads to disintegration of sludge cells and an increase in the fraction of small solid particles. Cell disintegration leads to release of proteins into the aqueous solution. Smaller particles need more time to settle and proteins have surface charges, which reduces their tendency to settle down. As a result, the settling tendency of this sludge decreases. Similar results were reported by Ried et al. (Citation2002) and Sievers et al. (Citation2004).
On the other hand, biological processes have noticeable effects on the improvement of the settling tendency of oxidative sludge (see Table ). The ability of biological process to eliminate portions from the water solution improves the settling tendency of the sludge.
3.5 Specific filtration index and sludge volume index
The outer part of Figure presents the evolution of SFI with time for single solar oxidation and different times of the year). The inner part of Figure shows the values of SVI at different times of the year. The original sludge (SFI = 74) was compared to oxidised sludge. Sludge oxidised by March (Sol-Mar) and November (Sol-Nov) have SFI values of 133 and 136, respectively. Higher SFI (SFI = 155) values were observed for the sludge oxidised by June (Sol-June). As indicated before, high SFI values imply low filterability of the sludge. In conclusion, single solar oxidation has a negative effect on SFI of sludge. High SFI values were related to high amounts of proteins at aqueous solution, which has negative effect on SFI. Similar tendency was observed regarding the SVI. More solar oxidation leads to a decrease in SVI values.
Figure 5 Effect of single solar oxidation on SFI of sludge. Insert sludge volume index during solar oxidation.
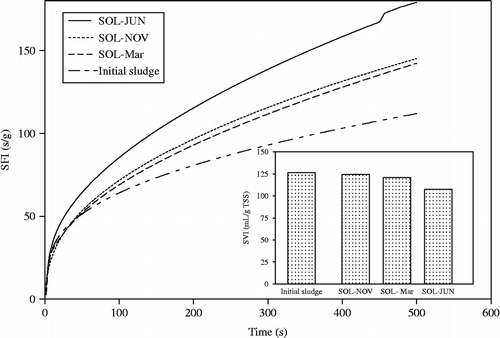
The SFI of solutions treated by sequential processes is presented in Table . It was observed that the degree of oxidation, biological treatment conditions and sludge COD content affect the sludge SFI in these processes. High degree of oxidation has a negative effect on SFI. However, a combination of solar oxidation and biological treatment improves the SFI of sludge; solar photons attack the cells leading to the release of the biodegradable cell liquids and the biological process utilises these liquids. The net result is a decrease in organic content of the sludge, reduction in TSS and improvement in both SFI and SVI. If the oxidation degree is high (e.g. SOL-JUN) and the biological treatment time is low, the SFI and SVI are both low because the released proteins cannot be removed by short bio-oxidation time. Similar tendencies were reported by other researchers (Ried et al. Citation2002).
The effect of mixing the treated sludge with fresh sludge on SFI and SVI was also followed up (see SQ4 of Table ). It was observed that mixing the oxidised sludge with fresh sludge improves the SFI of sludge, for the reasons stated above. Moreover, mixing oxidised sludge with fresh sludge is advantageous as it decreases the volume of the reactor and improves the treatment feasibility.
4. Conclusions
Solar energy can be used as sludge disintegration technology to reduce the production of excess sludge in bio-reactors. The reduction in COD and suspended solids in the bioreactor after solar oxidation treatment was related to the strength of solar irradiation and biological reactor HRT. Solar oxidation during different months of the year (January to October) increased the soluble biodegradable COD in the solution due to the release of cell liquid. The COD removals in single oxidation step ranged from 2.0% at an average solar radiation 2.7 kWh/m2 to 8.5% at an average solar radiation of 8.6 kWh/m2. Sequential processes showed a total COD removal of 4.8% at lower solar radiation intensity and 23% for higher solar radiation intensity. Maximum removal efficiency can be achieved by increasing the bio-oxidation time or by mixing fresh sludge with bio-oxidised disintegrated sludge.
The disintegrated sludge was found to have low settling ability due to the presence of high surface charge proteins in the solution. The settling efficiency can be enhanced by the removal of these proteins either by chemical or by biological oxidation.
Acknowledgements
The author greatly acknowledges the financial support provided by Support to Research and Technological Development & Innovation Initiative & Strategies in Jordan (SRTD) and Mutah University.
References
- Al Momani , F. , Schaefer , S. and Sievers , M. 2010 . Improved sludge dewaterability for sequential ozonation – aerobic treatment . Ozone: Science and Engineering , 32 : 252 – 258 .
- Amat , A.M. , Arques , A. , Lopez , F. and Miranda , M.A. 2005 . Solar photocatalysis to remove paper mill wastewater pollutants . Solar Energy , 79 : 393 – 401 .
- Andreozzi , R. , Canterito , M. , Giudice , R.L. , Marotta , R. , Pinto , G. and Pollio , A. 2006 . Lincomycin solar photodegradation, algal toxicity and removal from wastewaters by means of ozonation . Water Resource , 40 ( 3 ) : 630 – 638 .
- APHA-AWWA-WEF . 1992 . Standard methods for the examination of water and wastewater , 18th ed. , Washington, DC : American Public Health Association, American Water Works Association, and Water Environment Federation .
- Barjenbruch , M. and Kopplow , O. 2003 . Enzymatic, mechanical and thermal pre-treatment of surplus sludge . Advances in Environmental Research , 7 ( 3 ) : 715 – 720 .
- Bierbaum , S. and Oller , H.-J. 2005 . “ Online-controlled ozone dosage for the treatment of paper mill effluents ” . In Proceedings of the IOA 17th World Ozone Congress Strasbourg, France VII.1.2-1–VII.1.2-10
- Carballa , M. , Manterola , G. , Larrea , L. , Ternes , T. , Francisco , O. and Juan , M. 2007 . Influence of ozone pre-treatment on sludge anaerobic digestion: Removal of pharmaceutical and personal care products . Chemosphere , 67 ( 7 ) : 1444 – 1452 .
- Chu , L.B. , Sang-Tian , Y. , Xin-Hui , X. , An-Feng , Y. , Xu-Lin , S. and Benjamin , J. 2008 . Enhanced sludge solubilization by microbubble ozonation . Chemosphere , 72 ( 2 ) : 205 – 212 .
- Dichtl , N. , Muller , J. , Braunschweig , E.E. , Gunthert , F.W. and Osswald , M.O. 1997 . Waste sludge disintegration – a recent overview . Korrespondenz Abwasser , 44 : 1726 – 1739 .
- Erden , G. , Emir , O. and Filibeli , A. 2010 . Disintegration of biological sludge: effect of ozone oxidation and ultrasonic treatment on aerobic digestibility . Chemical Engineering Journal , 163 : 273 – 283 .
- Feng , X. , Deng , J. , Lei , H. , Bai , T.F. and Qingjuan , Z. 2009 . Dewaterability of waste activated sludge with ultrasound conditioning . Bioresource Technology , 100 ( 3 ) : 1074 – 1081 .
- Goel , R. , Tokytomi , T. , Yasui , H. and Noike , T. 2003 . Optimal process configuration for anaerobic digestion with ozonation . Water Science Technology , 48 ( 10 ) : 86 – 96 .
- Kamenev, I., 2003. Aerobic bio-oxidation combined with ozonation in recalcitrant water treatment, Doctoral thesis. Tallinn Technical University
- Malato , S. , Blanco , J. , Richter , C. , Fernández , P. and Maldonado , M.I. 2000 . Solar photocatalytic mineralization of commercial pesticides . Oxamyl, Solar Energy Materials and Solar Cells , 6 ( 1 ) : 1 – 14 .
- Manterola , G. , Uriarte , I. and Sancho , L. 2008 . The effect of operational parameters of the process of sludge ozonation on the solubilisation of organic and nitrogenous compounds . Water Resource , 42 ( 12 ) : 3191 – 3197 .
- Milena , L. , Pulgarin , C. , Fernandez-Ibanez , P. , Maldonado , M.I. , Perez- Estrada , M.I. , Oller , I. , Gernjak , W. and Malato , S. 2006 . Enhancing biodegradability of priority substances (pesticides) by solar photo-Fenton . Water Resource , 40 : 1086 – 1094 .
- Mohapatr , D.P. , Brar , S.K. , Tyagi , R.D. and Surampalli , R.Y. 2010 . Physico-chemical pre-treatment and biotransformation of wastewater and wastewater Sludge – Fate of bisphenol A (Review) . Bioresource Technology , 101 : 8093 – 8098 .
- Mohapatra , D.P. , Brara , S.K. , Tyagi , R.D. and Surampalli , R.Y. 2009 . Degradation of endocrine disrupting bisphenol A during pretreatment and biotransformation of wastewater sludge . Solar Energy , 83 : 306 – 315 .
- Munoz , I. , Rieradevall , J. , Torrades , F. , Peral , J. and Domenech , X. 2005 . Environmental assessment of different solar driven advanced oxidation processes . Solar Energy , 79 : 369 – 375 .
- Muruganandham , M. , Chen , S.H. and Wu , J.J. 2007 . Evaluation of water treatment sludge as a catalyst for aqueous ozone decomposition . Catalysis Communications , 8 ( 11 ) : 1609 – 1614 .
- Nowak , O. 2006 . Optimizing the use of sludge treatment facilities at municipal WWTPs . Journal of Environmental Science and Health A , 41 ( 9 ) : 1807 – 1817 .
- Pham , T.T.H. , Brar , S.K. , Tyagi , R.D. and Surampalli , R.Y. 2010 . Influence of ultrasonication and Fenton oxidation pre-treatment on rheological characteristics of wastewater sludge . Ultrasonics Sonochemistry , 17 : 38 – 45 .
- Ray , B.T. , Lin , J.G. and Rajan , R.V. 1990 . Low-level alkaline solubilization for enhanced anaerobic digestion . Journal of the Water Pollution Control Federation , 62 ( 1 ) : 81 – 87 .
- Ried , A.H. , Stapel , R. , Koll , M. , Schettlinger , F. , Wemhoner , A. , Hamann-Steinmeier , S. , Miethe , M. and Brombach , A. 2002 . Optimierungsmo “glichkeiten beim Betrieb von biologischen Kla” ranlagen durch den Einsatz von Ozon . Korrespondenz Abwasser , 49 ( 5 ) : 648 – 661 .
- Sakai , Y. , Fukase , T. , Yasui , H. and Shibata , M. 1997 . An activated sludge process without excess sludge production . Water Science Technology , 36 ( 11 ) : 163 – 170 .
- Sievers , M. , Ried , A. and Koll , R. 2004 . Sludge treatment by ozonation— evaluation of full-scale results . Water Science Technology , 49 ( 4 ) : 247 – 532 .
- Svarovsky , L. 1981 . “ Filtration fundamentals ” . In Solid liquid separation , 2nd ed. , 242 – 255 . London : Butterworths . Chapter 9
- Tian , Y.S. , Zheng , H. , Li , A. , Zhang , X. , Xing , X.-H. , Chu , Li-B. , Ding , G. , Sun , X.-L. and Benjamin , J. 2009 . Systematic analysis of biochemical performance and the microbial community of an activated sludge process using ozone-treated sludge for sludge reduction . Bioresource Technology , 100 ( 21 ) : 5002 – 5009 .
- Wawrzynczyk , J. , Recktenwald , M. , Norrlöw , O. and Dey Estera , S. 2008 . The function of cation-binding agents in the enzymatic treatment of municipal sludge . Water Resource , 42 ( 6–7 ) : 1555 – 1562 .
- Weemaes , M. , Grootaerd , H. , Simoens , F. and Verstraete , W. 2000 . Anaerobic digestion of ozonized biosolids . Water Research , 34 ( 8 ) : 2330 – 2336 .
- Xuan , Y. , Pingfang , H. , Xiaoping , Lu and Yanru , W. 2004 . A review on the dewaterability of bio-sludge and ultrasound pretreatment . Ultrasonics Sonochemistry , 11 ( 6 ) : 337 – 348 .
- Yamashita , Y. , Sasaki , C. and Nakamura , Y. 2010 . Development of efficient system for ethanol production from paper sludge pre-treated by ball milling and phosphoric acid . Carbohydrate Polymers , 79 ( 2 ) : 250 – 254 .
- Yeom , I.T. , Lee , K.R. , Ahn , K.H. , Lee , Y.H. and Lee , S.H. 2003 . Effects of ozone treatment on the biodegradability of sludge from municipal wastewater treatment plants . Water Science Technology , 46 ( 4–5 ) : 421 – 425 .
- Yoon , S.H. , Kim , H.S. and Lee , S.H. 2004 . Incorporation of ultrasonic cell disintegration into a membrane bioreactor for zero sludge production . Process Biochemistry , 39 ( 12 ) : 1923 – 1929 .
- Zhang , G. , Yang , J. , Liu , H. and Zhang , J. 2009 . Sludge ozonation: Disintegration, supernatant changes and mechanisms . Bioresource Technology , 100 ( 3 ) : 1505 – 1509 .