Abstract
A bio-based gear oil was developed from soybean oil (SBO). The SBO was first thermally polymerised and then mixed with additives and diluents. The effect of pour point depressants, co-base oils, antioxidants and anti-wear additives is reported. Lubricity, viscosity index and oxidation stability of the final formulation of the bio-based gear oil are compared with commercially available gear oils. The final formulation of bio-based gear oil gives test results: viscosity index 165, four-ball wear scar 0.375 mm. These numbers are comparable to or better than the commercially available gear oils tested for comparison. The oxidation onset temperature of the bio-based gear oil, 220°C, is lower than the evaluated commercial products, but still at an acceptable range for gear oil.
Vegetable oil-based materials comprise a growing part of the search for high performance lubricants that are based on renewable materials, a rapidly growing area (Hwang and Erhan Citation2002, Hill Citation2006). Because of their biodegradability, low ecotoxicity and excellent tribological properties, vegetable oils such as soybean oil (SBO) find application in many areas from greases to hydraulic oils, where bio-based lubricants offer lower coefficient of friction, improved wear characteristics, a higher viscosity index, lower volatility and higher flashpoints than mineral-based oils. However, the trade-off is poor with low temperature properties and oxidative stability (Cosgrove et al.Citation1987, Coscione and Artz Citation2005, Colakoglu Citation2007). Some studies utilising additives (Durak and Karaosmanoglu Citation2004, Tupotilov et al.Citation2006, Asadauskas et al.Citation2010) and chemical modification (Hwang and Erhan Citation2001, Pratap et al.Citation2005, Erhan et al.Citation2006, Sharma et al.Citation2008) have been carried out in order to mitigate these drawbacks.
Gear oils are essential for automotive and industrial lubrication, for which they are commonly used in transmissions, differentials, power take-offs and non-drive applications. They are required to minimise friction and wear, reduce noise, remove wear particles, inhibit corrosion, transfer heat and improve efficiency (Mang et al.Citation2007). In order to provide this protection in both the hydrodynamic and elastohydrodynamic regions, strict viscosity characteristics are required. In their natural state, vegetable oils do not have sufficient viscosity for these applications. This problem can be overcome by thermal polymerisation of the oil. Thermal polymerisation involves heating the oil under nitrogen to produce higher molecular weight products. This method has been used in various applications such as inks (Erhan and Bagby Citation1992), surfactants (Liu and Biresaw Citation2011), and polymers (Liu and Erhan Citation2010). SBO is an excellent candidate for thermal polymerisation due to its high concentration of linoleate (C18:2) and linolenate (C18:3) fatty chains and its easy availability. In this study, thermal polymerisation of SBO is formulated with additives and synthetic fluids to make fluids of various viscosities for gear oil applications. These fluids are compared with commercially available API GL-5 grade gear oils for reducing friction and wear and oxidation stability.
The bio-based oil used in this study (thermally polymerised soybean oil, TP-SO) was a SBO, which was thermally polymerised by literature-based methods (Erhan and Bagby Citation1992, Citation1998). In short, the SBO was heated to 330°C in a three-neck flask under a nitrogen atmosphere with constant stirring. A trap was used to collect volatile components. This method has been shown to produce highly viscous oils of reduced iodine value due to the double bond reactions. The basestock used in this work was polymerised for 240 min to achieve a material of sufficient viscosity for use as gear lubricant, which was measured by an Anton Paar Stabinger Viscometer (SVM 3000, Ashland, VA, USA) according to the American Society for Testing and Materials (ASTM) standard D7042. The desired viscosities of 283.7 mm2 s− 1 at 40°C and 40.3 mm2 s− 1 at 100°C give a viscosity index of 196 as calculated by ASTM D2270. The 100°C value shows that this oil, without additives or diluents, would be classified in the Society of Automotive Engineers (SAE) viscosity grade 190 category according to the SAE Axle and Manual Transmission Lubricant Viscosity Classifications (SAE J306). Other viscosities can be achieved by using the same methodology with different heating times and temperatures, and also by utilising the blending strategy employed in this report.
Bio-based oils suffer from two primary deficiencies: first, they have poor flow characteristics (Ming et al.Citation2005), and second, they oxidise at a higher rate than conventional oils (Kockritz and Martin Citation2008). These drawbacks have been addressed by a blending strategy utilising a series of additives and base co-oils (Table ).
Table 1 The additives and fluids formulated with the TP-SO and the commercially available gear oils used in this study.
Low temperature flow characteristics in the oil were measured by using an automated method, ASTM D-5949, which tests sample pourability. The pour point reported was the temperature increment above that which made the sample unpourable. The duplicate measurements were made with a Phase Technology Analyser (Model PSA-70S, Phase Technology, Hammersmith Gate, Richmond, BC, Canada). The pour point depressants (Table ) were blended in 0–10% (wt) concentrations with the TP-SO and pour points were reported (Figure ). The results show considerable effectiveness for two of the products, even at a 2% (wt) blending level. They reduce to a lower temperature at a 4% (wt) level. Further decreases are minimal showing the most efficient level to be 4% (wt), and giving a pour point of approximately − 15°C, compared to the starting value of approximately − 9°C.
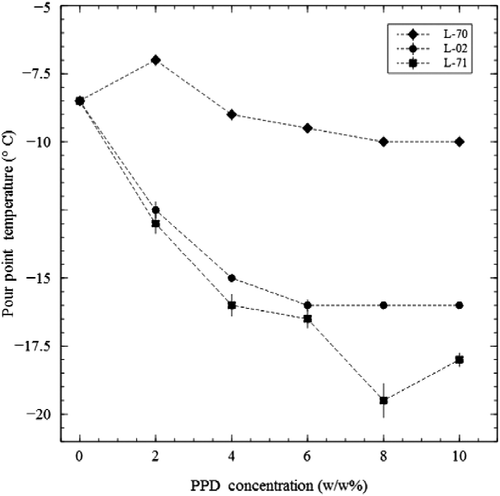
More significant decreases in the pour points are achieved by blending the bio-based oil with synthetic fluid. Poly alpha olefin and ester basestocks (Table ) were mixed with TP-SO, showing significantly lowered pour points from the starting value of approximately − 9°C. The pour point reduction observed with the addition of the synthetics was a roughly linear correlation (Figure ).
The oxidation of the gear oils was studied utilising a pressurised differential scanning calorimeter (PDSC, model Q20 TA Instruments, New Castle, NJ, USA), where the sample is heated under pressurised oxygen, and the onset of the exothermic oxidation reaction was determined. In these experiments, ∼2.0 mg samples were placed in hermetically sealed aluminium pans with a pinhole lid to give interaction of the sample with the reactant dry air, which was introduced to the system with a constant pressure of 1378.95 kPa. Additives (Table ) of different active chemical species were tested. All of the evaluated additives improved the oxidation stability of the unstable base oil. The most effective (Figure ) was 22-M, a hindered phenol. The possibility of combining this additive with an amine additive to achieve a synergitic effect was also evaluated. Using 22-M with the amine additives, N-640 or N-438, at 1% (wt) concentration each gave an onset of oxidation of ∼210°C, about 10°C better than the phenolic alone and ∼40°C better than the amine alone. This synergistic effect between the amine and phenol derivative additives is explained in the literature (Dong and Migdal Citation2009) through a mechanism where both diphenylamine and phenol are active against the alkyl peroxy radicals (RO• and ROO•). However, in this homosynergism, the diphenylamines are initially more reactive than the phenols in scavenging radicals. After reaction, they are then efficiently regenerated by the phenols resulting in an overall more effective scavenging.
Of course an industrial lubricant needs to do one thing well, and that is lubricate. This is an especially important factor in gear oil applications. The TP-SO is a good basestock in the protection against wear and the reduction of friction. The friction and wear test was conducted as per ASTM D4170-94 using a Falex apparatus (Model Multi-Specimen, Falex® Corporation, Sugar Grove, IL, USA). A top ball is rotated on three stationary balls immersed in 15 ml of lubricant. A 40 kg force was applied, and the lubricant was heated to 75°C. The ball was rotated at 1200 RPM for 60 min, and the coefficient of friction is determined from the torque required. The wear scar diameter of the three lower balls was measured microscopically. The lubrication additives used (Table ) range from borate esters, dialkyldithiocarbamate compounds and zinc dialkyldithiophosphates. From the data (Figure ), it can be shown that the combination of the boron ester and the dialkylthiophosphate compound resulted in the smallest scar. This indicates a possibly synergistic effect, in which different mechanisms of friction reduction are possibly acting together to produce the best effect.
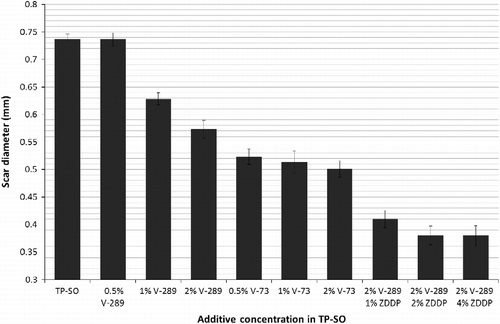
The US Department of Agriculture has established many categories with separate criteria for its bio-based labelling programme. In order for an oil to be a Biopreferred Gear Lubricant, it must contain 58% renewable carbon as listed on their website, http://www.biopreferred.gov/. With this number in mind, as well as the data presented in this work, a final gear oil formulation was developed which will both meet this criterion and also perform as a lubricant. This formulated bio-based gear oil (bio-GO) was compared to commercial products (Table ). The final lubricant comprises 59.5% (wt) polymerised SBO, 30.5% (wt) synthetic fluid (E-101), 4% (wt) pour point depressant (L-71)PPD (LZ 7671A), 2% (wt) antioxidant (1% 22-M and 1% N-640) and 4% (wt) anti-wear (2% V-289 and 2% zinc dialkyldithiophosphate).
The viscosity of the bio-based gear oil (Table ) was considerably higher than that of the commercial products, but those of lower viscosity could be formulated using either more of the synthetic co-lubricant or SBO. The viscosity index of the oil, however, was higher than four of the commercial products, trailing only Com-5. The wear scar results (Figure ) showed a similar trend. The bio-GO had a value of 0.375 mm. Only one of the commercial products, with values of 0.37–0.46 mm, was as good as the bio-based oil. Further testing, such as the 20 h CEC L-45–99 shear test, would also be of significant interest on the wear reduction capability in the bio-GO.
Table 2 The viscosity characteristics of the base oils and bio-based gear oil formulas from this work compared to those of commercial products.
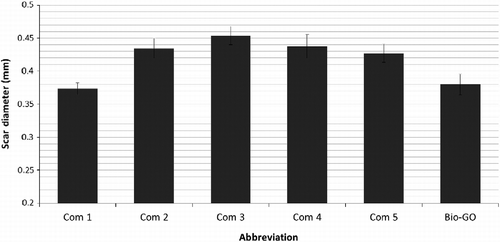
In the biggest problem area for bio-based lubricants, oxidation, the bio-based gear oil did not fare well (Figure ). This was monitored by Pressure Differential Scanning Calorimetry. In this experiment, the commercial products oxidised between 269 and 280°C, whereas the bio-GO oxidised at only 220°C. Although all of the commercial products are more stable in this test, the bio-based oil still has a value greater than 200°C.
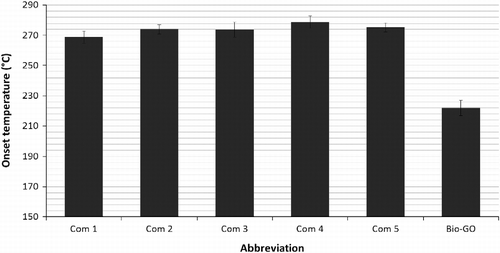
Overall, the need for bio-based lubricants is increasing rapidly. Polymerised SBOs are a good candidate for high viscosity lubricant applications, with many advantages including probable biodegradability. This study showed that a bio-based gear lubricant offering equivalent or better performance in bench tests, compared with commercial gear lubricants, can be formulated using TP-SO as the primary base fluid.
Acknowledgements
The authors acknowledge Cynthia M. Ruder for calorimetry work reported here. This research was part of a joint effort by the Agricultural Research Service of the United States Department of Agriculture, Bio-Oils Research Group, Peoria, IL and the Tribology Group, Chemical Engineering Department of the Pennsylvania State University, University Park, PA.
Notes
USDA/NCAUR/ARS: Mention of trade names or commercial products in this publication is solely for the purpose of providing specific information and does not imply recommendation or endorsement by the U.S. Department of Agriculture. USDA is an equal opportunity provider and employer.
REFERENCES
- Asadauskas, S.J., Biresaw, G., and McClure, T.G., 2010. Effects of chlorinated paraffin and ZDDP concentrations on boundary lubrication properties of mineral and soybean oils. Tribology Letters, 37 (2), 111–121.
- Colakoglu, A.S., 2007. Oxidation kinetics of soybean oil in the presence of monoolein, stearic acid and iron. Food Chemistry, 101 (2), 724–728.
- Coscione, A.R. and Artz, W.E., 2005. Vegetable oil stability at elevated temperatures in the presence of ferric stearate and ferrous octanoate. Journal of Agricultural and Food Chemistry, 53 (6), 2088–2094.
- Cosgrove, J.P., Church, D.F., and Pryor, W.A., 1987. The kinetics of the autoxidation of polyunsaturated fatty acids. Lipids, 22 (5), 299–304.
- Dong, J. and Migdal, C.A., 2009. Antioxidants. In: L.R.Rudnick, ed. Lubricant additives, chemistry and applications. 1st ed.Boca Raton, FL: CRC Press, 3–50.
- Durak, E. and Karaosmanoglu, F., 2004. Using of cottonseed oil as an environmentally accepted lubricant additive. Energy Sources, 26, 611–625.
- Erhan, S.Z., Adhvaryu, A., and Sharma, B.K., 2006. Poly(hydroxy thioether) vegetable oil derivatives useful as lubricant additives, 7279448, October, 9, 2007.
- Erhan, S.Z. and Bagby, M.O., 1992. Vegetable oil-based printing ink, 5122188, June 16, 1992.
- Erhan, S.Z. and Bagby, M.O., 1998. Vegetable oil-based offset printing inks, 5713990, February 3, 1998.
- Hill, K., 2006. Industrial development and application of biobased oleochemicals. In: B.Kamm, P.R.Gruber and M.Kamm, eds. Biorefineries – Industrial processes and products, status quo and future directions. 1st ed.Weinheim: Wiley-VCH, 291–314.
- Hwang, H.-S. and Erhan, S.Z., 2001. Modification of epoxidized soybean oil for lubricant formulations with improved oxidative stability and low pour point. JAOCS, Journal of the American Oil Chemists' Society, 78 (12), 1179–1184.
- Hwang, H.-S. and Erhan, S.Z., 2002. Lubricant base stocks from modified soybean oil. In: S.Z.Erhan and J.M.Perez, eds. Biobased industrial fluids and lubricants. 1st ed.Champaign, IL: AOCS Press, 20–34.
- Kockritz, A. and Martin, A., 2008. Oxidation of unsaturated fatty acid derivatives and vegetable oils. European Journal of Lipid Science and Technology, 110 (9), 812–824.
- Liu, Z. and Biresaw, G., 2011. Synthesis of soybean oil-based polymeric surfactants in supercritical carbon dioxide and investigation of their surface properties. Journal of Agricultural and Food Chemistry, 59 (5), 1909–1917.
- Liu, Z. and Erhan, S.Z., 2010. Preparation of soybean oil polymers with high molecular weight. Journal of Polymers and the Environment, 18 (3), 243–249.
- Mang, T., Freiler, C., and Horner, D., 2007. Metalworking fluids. In: T.Mang and W.Dresel, eds. Lubricants and lubrication. 2nd ed.Weinheim: Wiley-VCH.
- Ming, T.C., Ramli, N., Lye, O.T., Said, M., and Kasim, Z., 2005. Strategies for decreasing the pour point and cloud point of palm oil products. European Journal of Lipid Science and Technology, 107 (7–8), 505–512.
- Pratap, A.P., Kadam, A.S., and Bhowmick, D.N., 2005. Modified oils and fats as biolubricants. INFORM, 16 (6), 282–285.
- Sharma, B.K., Liu, Z., Adhvaryu, A., and Erhan, S.Z., 2008. One-pot synthesis of chemically modified vegetable oils. Journal of Agricultural and Food Chemistry, 56 (9), 3049–3056.
- Tupotilov, N.N., Ostrikov, V.V., and Kornev, A.Y., 2006. Plant oil derivatives as additives for lubricants. Chemistry and Technology of Fuels and Oils, 42 (3), 192–195.