Abstract
Alternative and renewable fuels have numerous advantages compared with fossil fuels as they are renewable and biodegradable, besides providing food and energy security and foreign exchange savings and addressing environmental and socio-economic issues. Therefore, these renewable fuels can be used predominantly in compression ignition (CI) engines for transportation purposes and power generation applications. Today, the use of biomass-derived producer gas is more relevant for addressing rural power generation and is also a promising technique for controlling both NO x and soot emission levels. Although a producer gas–biodiesel-operated dual-fuel diesel engine exhibits lower performance, they are independent from the use of fossil fuels. The lower performance of the engine could be due to the slow-burning and lower calorific value of producer gas. For this purpose, exhaustive experiments on the use of Honge oil methyl ester (HOME)–producer gas in a dual-fuel CI engine were carried out for the improvement of its fuel efficiency. This paper presents the effect of the compression ratio (CR) on the performance, combustion and exhaust emission characteristics of a single-cylinder, four-stroke, direct injection stationary diesel engine operated using HOME and producer gas in a dual-fuel mode. The results indicated that the HOME–producer gas combination exhibited lower brake thermal efficiency (BTE) with comparable emission levels with the diesel–producer gas combination at different CRs. Comparative measures of BTE, peak pressure, pressure–crank angle variation, heat release rate, smoke opacity, and hydrocarbon (HC), carbon monoxide (CO) and nitric oxide (NO x ) emission levels are presented and analysed.
1. Introduction
In India, there are approximately 580,000 villages and 70% of the population in rural areas depends on agriculture for their daily living. India has a huge potential for biomass and agricultural residues, and it has been estimated that approximately 620 million tonnes of biomass reserves were available in the country during 2004–2005 and total biomass reserve was more than 700 million tonnes during 2010–2011. It is expected to be 1127 million tonnes during 2024–2025. Due to the shortage in crude oil supply, India is still dependent on non-commercial fuel sources such as cow dung, firewood, agricultural waste and biofuels to the extent of 30–35%. At present, it is estimated that India will be able to produce 288 metric tonnes of biodiesel by the end of 2012, which will supplement about 41.14% of the total demand of diesel fuel consumption (Deepak and Kumar Citation2007; Nwafor Citation2000, Citation2003; Scholl and Sorenson Citation1993).
Given the energy needs of the rural population, India has launched several programmes on biomass gasifier-based power units with as high as mega watt-level grid-connected plants that operate on a dual-fuel mode and 100% producer gas-run engines. In view of this, the promotion of biomass-based power generation is currently being encouraged in India. This is due to the fact that dual-fuelling of a diesel engine mainly offers fuel flexibility, lower exhaust gas emissions, better efficiency and easy conversion of existing diesel engines by retaining higher compression ratio (CR) and with minor hardware modifications (Banapurmath et al. Citation2011b; Ramadas, Jayaraj, and Muralidharan Citation2006; Singh, Singh, and Pathak Citation2007).
Biodiesels derived from vegetable oils exhibit slightly lower performance with reduced emission levels (Banapurmath et al. Citation2009; Nwafor Citation2000, Citation2003; Parikh et al. Citation1989; Ramadas, Jayaraj, and Muralidharan Citation2006; Scholl and Sorenson Citation1993; Singh, Singh, and Pathak Citation2007). The effects of various engine parameters such as CR, injection timing (IT) and engine loading (L) on the performance and exhaust emissions of a single-cylinder diesel engine operated using biodiesel and blends with diesel fuel have been reported in the literature (Muralidharan and Vasudevan Citation2011; Rehaman and Ghadge Citation2008; Sridhar et al. Citation2005). Previous studies have reported that brake-specific fuel consumption, brake thermal efficiency (BTE) and emission levels are considerably improved with an increase in the CR (Banapurmath et al. Citation2011a; Muralidharan and Vasudevan Citation2011; Sridhar et al. Citation2005; Sahoo, Sahoo, and Saha Citation2009). Biomass gasifier projects for decentralized power supply and financial evaluation have been reported in the literature (Ravindranath and Balachandra Citation2009; Nouni, Mullick, and Kandpal Citation2007). Many investigators have extensively studied gasifier–engine systems for both rural and urban power applications (Banapurmath, Tewari, and Hosmath Citation2008; Banapurmath et al. Citation2009, Citation2011a; Sayin and Gumus Citation2011; Muralidharan and Vasudevan Citation2011; Nouni, Mullick, and Kandpal Citation2007; Parikh et al. Citation1989; Ramadas, Jayaraj, and Muralidharan Citation2006, Ravindranath and Balachandra Citation2009; Rehaman and Ghadge Citation2008; Singh, Singh, and Pathak Citation2007; Sridhar et al. Citation2005). The dual-fuel engine with producer gas induction has been found to result in higher thermal efficiency with reduced emission levels at a higher CR and advanced IT. It is also reported in literature that a dual-fuel engine fuelled with producer gas exhibits higher exhaust gas temperature (EGT) when operated at fixed IT and CR than diesel fuel operated engines (Banapurmath et al. 2009, 2011a; Sahoo, Sahoo, and Saha 2009; Samir, Lalit, and Shashikant 2008). The higher octane number of producer gas allows the use of a higher CR without engine knocking. Producer gas can act as a promising alternative gaseous fuel, especially for diesel engines, by substituting a considerable amount of liquid fuel oil. However, diesel engines cannot be operated with producer gas without the injection of a small amount of diesel/biodiesel because the producer gas will not ignite under the prevailing conditions of temperature and pressure. The major problem associated with producer gas-operated engines is their power-derating. Producer gas-fuelled dual-fuel engines always operate with lower efficiency and increased HC and CO emission levels with decreased NO x and smoke emission levels. Diesel savings up to 70–90% have been reported for dual fuelling (Banapurmath, Tewari, and Hosmath Citation2008; Banapurmath et al. Citation2009, Citation2011a; Sayin and Gumus Citation2011; Karim, Raine, and Jones Citation1988; Karim, Jones, and Raine Citation1989; Muralidharan and Vasudevan Citation2011; Nouni, Mullick, and Kandpal Citation2007; Parikh et al. Citation1989; Ramadas, Jayaraj, and Muralidharan Citation2006, Ravindranath and Balachandra Citation2009; Rehaman and Ghadge Citation2008; Roy et al. Citation2009; Sahoo, Sahoo, and Saha Citation2009; Samir, Lalit, and Shashikant Citation2008; Singh, Singh, and Pathak Citation2007; Sridhar et al. Citation2005). The effects of the carburettor type and biomass feedstock properties on the performance and emission characteristics of a dual-fuel engine have been reported in the literature (Banapurmath, Tewari, and Hosmath Citation2008; Banapurmath et al. Citation2011a; Parikh et al. Citation1989; Ramadas, Jayaraj, and Muralidharan Citation2006; Samir, Lalit, and Shashikant Citation2008). Some investigators have reported decreased BTE, peak pressure and heat release rate and increased specific energy consumption, ignition delay and combustion duration for a dual-fuel engine operation (Banapurmath, Tewari, and Hosmath Citation2008; Banapurmath et al. Citation2009; Sridhar et al. Citation2005; Sahoo, Sahoo, and Saha Citation2009; Roy et al. Citation2009; Karim, Raine, and Jones Citation1988; Karim, Jones, and Raine Citation1989). The effects of engine speeds, loads, pilot injection angle, pilot fuel quantity and CRs on combustion noise, knocking torque, thermal efficiency and maximum pressure of a natural gas-fuelled dual-fuel engine have been investigated (Selim Citation2004). Many researchers have conducted experiments on a dual-fuel engine using a variety of biodiesels and producer gas that are derived from biomass feedstock of different origin (Skoulou et al. Citation2008; Churchill et al. Citation1986; Parikh et al. Citation1989; Sridhar et al. Citation2005; Banapurmath, Tewari, and Hosmath Citation2008; Banapurmath et al. Citation2011a). In the case of a producer gas-fuelled dual-fuel engine, a suitable carburettor was used to provide a mixture of appropriate pre-defined air-to-fuel ratios to the engine over the entire range of engine operation (Banapurmath, Tewari, and Hosmath Citation2008; Banapurmath et al. Citation2009, Citation2011a).
Major attention and interest is given to enhance the thermal efficiency of a producer gas-operated dual-fuel engine with decreased emission levels by advancing the IT and increasing the injection pressure and CRs. The use of biodiesel and producer gas-based power generation will create new industries and bring increased economic activity. In this context, the present study conducted experiments on a water-cooled, single-cylinder, direct injection (DI) compression ignition (CI) engine operated under a dual-fuel mode with HOME and producer gas induction at different CRs, and the results were compared with the diesel–producer gas operation.
1.1 Present work
In the present study, an effort has been made to evaluate the feasibility of alternative and renewable fuels in the form of Honge oil methyl ester (HOME) and producer gas as a complete replacement for fossil fuels. In view of this, experiments were conducted on a single-cylinder, four-stroke, CI engine operated under a dual-fuel mode at three CRs of 15, 16 and 17.5 and at an advanced IT of 27° bTDC, an injection pressure of 205 bar for the diesel–producer gas and 230 bar for the HOME–producer gas operation. The optimum parameters such as advanced IT and increased injection pressure have been reported in earlier studies by the same authors (Banapurmath Citation2008; Banapurmath et al. Citation2009, Citation2011a).
2. Characterization of HOME and producer gas
In the present study, diesel and HOME were used as injected fuels and the producer gas derived from the babul wood as an inducted fuel. Honge tree is also called Karanja oil. This tree is chiefly found along the banks of streams and rivers or near the sea coast. It can grow in dry places and in any soil and climatic conditions and can attain heights of 15–18 m. It is often grown as a roadside avenue tree all over India. The expected life of this tree is 40–50 years and its potential yield on maturation is about 5000 kg/ha.
Acacia nilotica Linn. is a tree, belonging to the Leguminosae family and the subfamily of Mimosavea. It is a moderate-sized tree with a spreading crown, spiny, with dark bark brown to almost black, and an evergreen tree with a short, thick and cylindrical trunk found throughout India. It is also called babul, Kikar and Babur (Hindi). The babul tree grows in a scattered fashion and occurs in groups naturally. In favourable localities, it attains a height of 15–20 m and a girth of 2.4–3.0 m and is a stunted, shrubby or straggling tree. This species occurs abundantly, particularly in Karnataka. A. nilotica can regenerate naturally. It occurs in patches, usually in wastelands and common grazing lands. The wood contains 5–15% of moisture and traces of sugar, and yields 2.0–3.5% of ash, comprising 29% oleic and 44.5% linoleic acid and other traces of gallic acid, digallic acid, chlorogenic acid, etc.
The composition of HOME, including contribution of fatty acids and their chemical formula, structure and molecular weight, has been reported in the literature. Oils contain a small amount of two saturated fatty acids, namely palmitic (16:0) and steric (18:0) acids (Yaliwal et al. Citation2010). The properties of HOME and gasifier feedstock were determined experimentally and are summarized in Table . Table presents the typical composition of the producer gas obtained from gasification on a volumetric basis.
Table 1 Properties of the fuels tested.
Table 2 Composition of the producer gas.
3. Experimental set-up
The experiments were conducted on a Kirloskar TV1 type, single-cylinder, water-cooled, four-stroke diesel engine test rig. Figure shows the experimental set-up. An eddy current dynamometer was used for loading the engine. The fuel flow rate was measured on a volumetric basis using a burette and stopwatch. The engine was operated at a rated constant speed of 1500 rpm. The downdraft gasifier was suitably connected to the engine with a filter and cooling and cleaning system. The producer gas was generated using a downdraft gasifier. The gas is taken inside the combustion chamber by the suction of the engine. Gas flow was measured using a calibrated venturimeter provided with a digital gas flow meter. The CR can be increased up to a maximum of 17.5, according to the manufacturer's recommendations. The CR of the engine was changed using a movable cylinder head attached to the engine cylinder block. The head movement changes the clearance volume while keeping the swept volume constant. The cooling of the engine was accomplished by the water circulating through the jackets of the engine block and cylinder head. The cylinder pressure was measured using a piezoelectric transducer fitted to the cylinder head, as shown in Figure . Figure shows the parallel flow gas entry carburettor for producer gas induction fitted to the inlet manifold of the engine. In the present study, the amount/quantity of both the injected fuels (diesel and HOME) was measured on a volumetric basis. At a fixed brake power, a large amount of HOME was injected as its calorific value was comparatively lower and kinematic viscosity was higher compared to that of diesel. This was done by adjusting the governor speed so that a constant speed is maintained in both the injected fuels. At the 80% load, the specific fuel consumption for the diesel operation was 260 g/kW h, while for the HOME operation, it was 280 g/kW h. The emission characteristics were measured by using a Hartridge smoke meter and five-gas analyser during the steady-state operation. Throughout the experiments, the gas flow rate and the engine speed were kept constant. For the present study, the IT was kept constant at 27° bTDC and injection pressures were 205 and 230 bar for the diesel–producer gas and HOME–producer gas operations, respectively. A conventional mechanical injection system was used for the liquid fuel injection. The experiments were conducted with HOME–producer gas at three different CRs (15, 16 and 17.5). Finally, the results obtained with the HOME–producer gas operation were compared with those of the diesel–producer gas operation. The specifications of the CI engine, downdraft gasifier and piezoelectric transducer are given in Tables , and , respectively.
Table 3 Specifications of the engine.
Table 4 Specifications of the downdraft gasifier.
Table 5 Specifications of the piezoelectric transducer.
4. Results and discussion
This section presents the results and discussion on the performance of the HOME–producer gas dual-fuelled engine.
4.1 Performance characteristics
The BTE of the dual-fuel engine refers to the ratio of the brake power of the engine to the total energy supplied by both the liquid fuel and producer gas. The variations in BTE with brake power are shown in Figure . It can be observed that BTE was higher for a higher CR. This is mainly due to the decreased auto-ignition temperature of the mixed fuels (HOME–producer gas). The BTE was comparatively lower for the dual-fuel operation with the HOME–producer gas combination than for the diesel–producer gas operation. This could be attributed to the combined effect of higher viscosity, lower volatility and calorific value of HOME and slow-burning producer gas itself. However, the BTE of the HOME–producer gas-fuelled dual-fuel engine increased with an increase in the CR. This is because the combustion pressure and temperature of the working mixture increase with an increase in the CR. This leads to a higher flame temperature (Sridhar et al. Citation2005) and better combustion with a reduction in the concentration of residual gases. The results obtained are in close agreement with similarly published work (Sahoo, Sahoo, and Saha Citation2009). The CR of 17.5 resulted in a higher BTE than the CRs of 15 and 16. Improved performance with an increased CR for the HOME–producer gas operation is well supported by a higher octane number of the producer gas. The BTE obtained at the CRs of 15, 16 and 17.5 were 12.89, 16.2 and 17.25%, respectively, for the HOME–producer gas operation when compared with the value of 23.51% at the CR of 17.5 for the diesel–producer gas operation. On an average, for the HOME–producer gas operation at different CRs, the BTE was increased by 39.1% when the CR was increased from 15 to 17.5, respectively. It was increased by 24.4% when the CR was raised from 16 to 17.5, respectively.
The variations in EGT for the diesel–producer gas and HOME–producer gas operations with respect to various CRs are shown in Figure . The increase in the CR lowers the EGT. At a lower CR, the combustion temperature is lower, hence the unburnt hydrocarbon burns during the diffusion combustion phase rather than at the premixed combustion phase. Therefore, this results in increased EGT. However, at a higher CR, due to a higher combustion temperature, the fuel burns completely during the premixed combustion phase, and this favourable condition results in higher power output and efficiency. This may be due to the slightly reduced burning of the fuel combinations during the diffusion combustion phase at a higher CR. The EGT reduced at a higher CR, due to better combustion observed during the rapid combustion period. This may be due to the improved burning of the HOME–producer gas combination during the diffusion phase. The results are in line with those reported in the literature when using biodiesels (Raheman and Ghadge Citation2008; Muralidharan and Vasudevan Citation2011). The EGT obtained at the CRs of 15, 16 and 17.5 were found to be 560, 525 and 508°C, respectively, for the HOME–producer gas operation compared with the value of 410°C at the CR of 17.5 for the diesel–producer gas operation. On an average, for the HOME–producer gas operation at different CRs, the EGT was reduced by 26% when the CR was increased from 15 to 17.5, respectively. It was reduced by 18.9% when the CR was raised from 16 to 17.5, respectively.
Figure shows the variations in volumetric efficiency at different CRs for the HOME–producer gas operation. The volumetric efficiency indicates the breathing ability of an engine. A drop in volumetric efficiency with power output was observed for all the fuel combinations. It may be due to a higher gas temperature and an increase in the temperature of the inlet valve and combustion chamber walls. This feature decreases the density of the induced air, and hence there is a drop in volumetric efficiency. However, the part of the air replaced by the producer gas further adds to this observed trend. The diesel–producer gas operation resulted in better volumetric efficiency compared with the HOME–producer gas operation. This could be due to the improper utilization of the air as a large amount of HOME was injected for the same power generation with the producer gas in dual-fuel combinations. Also, a higher second peak was observed during the diffusion combustion phase at a lower CR for the HOME–producer gas operation. The volumetric efficiency values were found to be 74, 73 and 72.5% at the CR of 15, 16 and 17.5, respectively, for the HOME–producer gas operation compared with the value of 76% for the diesel–producer gas operation at the 80% load.
4.2 Emission characteristics
The emission characteristics of the engine are important from environmental perspectives. The emission from the engine reflects the quality of combustion taking place inside the engine. The emission levels for the producer gas operation under a dual-fuel mode were measured under steady-state conditions using calibrated instruments. The different emission parameter measurements during the dual-fuel mode of operation are discussed below.
Figure shows an increase in the CR with decreased smoke emission levels. When the CR is increased, the combustion temperature and pressure are increased, resulting in improved combustion, as the flame produced by the burning of the fuels reaches the entire area of the cylinder. Hence, the lower smoke emission levels were obtained at a higher CR for the dual-fuel operation. The smoke emission values obtained at the CRs of 15, 16 and 17.5 were 68, 61 and 54 HSU, respectively, for the HOME–producer gas operation compared with the value of 32 HSU at the CR of 17.5 for the diesel–producer gas operation. The smoke emission levels were found to be lower at the same CR for the diesel–producer gas operation than for the HOME–producer gas operation. The higher smoke emission levels resulting from the HOME–producer gas operation are mainly due to the heavier molecular structure, higher viscosity and density and poor atomization of HOME, leading to incomplete combustion. This leads to slightly higher smoke emission levels. On an average, for the HOME–producer gas operation at different CRs, smoke opacity was reduced by 20.5% when the CR was increased from 15 to 17.5, respectively. It was reduced by 11.5% when the CR was raised from 16 to 17.5, respectively.
The variations in hydrocarbon (HC) and carbon monoxide (CO) emission levels with respect to the varying CRs for the diesel–producer gas and HOME–producer gas operations are shown in Figures and , respectively. It can be observed that the increase in the CR decreases both the HC and CO emission levels. This could be due to the fact that when the CR is increased, the combustion temperature and pressure are increased, resulting in improved combustion. The increased flame propagation due to the increased CR helps in burning the entire fuel mixture, hence lower HC and CO emission levels were obtained at a higher CR of 17.5 compared with the CRs of 15 and 16. However, at a lower CR, the combustion temperature is lower, leading to the freezing of the oxidation process.
The higher BTE at a higher CR is also responsible for this observed trend. In general, the dual-fuel operation with HOME–producer gas leads to higher HC and CO emission levels than that with diesel–producer gas. This is because the producer gas contains CO as the main constituent, and it also has a lower flame velocity. This may require more time to ensure the complete combustion. Hence, the mixture of the producer gas and HOME biodiesel results in incomplete combustion. This in turn results in higher CO and HC emission levels in the exhaust. The higher HC and CO emission levels resulting from the HOME–producer gas-fuelled dual-fuel operation are also due to the fact that HOME has a higher viscosity and density, leading to relatively poor atomization. The CO emission values obtained at the CRs of 15, 16 and 17.5 were found to be 0.825, 0.75 and 0.46%, respectively, for the HOME–producer gas operation compared with the value of 0.32% for the diesel–producer gas operation. Similarly, HC emission values were found to be 75, 66 and 52 ppm at the CRs of 15, 16 and 17.5, respectively, for the HOME–producer gas operation compared with 41 ppm for the diesel–producer gas operation. On an average, for the HOME–producer gas operation at different CRs, the CO emission levels were reduced by 44.5% when the CR was increased from 15 to 17.5, respectively. The HC emission levels were reduced by 30.6% when the CR was raised from 16 to 17.5, respectively. Similarly, the HC emission levels were reduced by 30.6% when the CR was increased from 15 to 17.5, respectively. The levels were reduced by 21.2% when the CR was raised from 16 to 17.5, respectively.
The variations in NO x emission levels with brake power are shown in Figure . The NO x emission levels of the HOME–producer gas-fuelled dual-fuel operation were lower than those of the diesel–producer gas operation. This is because the combustion temperature inside the engine cylinder is lower for the HOME–producer gas operation, and also it has a lower calorific value. It can be observed that the HOME–producer gas operation exhibits increased NO x emission levels at a higher CR of 17.5 compared with the CRs of 15 and 16. This could be attributed to the increased combustion temperature due to the higher CR. For the HOME–producer gas operation, the NO x emission levels at CR of 15, 16 and 17.5 were found to 33, 36 and 44.5 ppm, respectively. In general, these values were lower than 55 ppm obtained for diesel–producer gas operation. On an average, for the HOME–producer gas operation at different CRs, the NO x emission levels were increased by 24.6% when the CR was increased from 15 to 17.5, respectively. The levels were increased by 19.1% when the CR was raised from 16 to 17.5, respectively.
4.3 Fuel substitution
Figure shows the fuel substitution at different CR for the dual-fuel operation. The maximum fuel substitution is given the prime importance in a dual-fuel mode of operation, which depends on the physico-chemical properties of the injected fuel such as cetane number, viscosity and calorific value and basic engine design. The fuel substitution values were found to be higher at higher CRs. The higher CR improves the BTE and lowers the specific fuel consumption. This means that a small amount of fuel is consumed at a higher CR and hence allows a large amount of producer gas burning for the same power output. The percentage values of the fuel substituted at a higher CR of 17.5 were found to be 65.18 and 54.56% for the diesel–producer gas and HOME–producer gas operations at the 80% load.
4.4 Combustion characteristics
The combustion in a diesel engine differs when gaseous fuels are used, and it depends on the air–fuel mixture quality. Different combustion characteristics are discussed below.
The variations in peak pressure with respect to the various CRs for the diesel–producer gas and HOME–producer gas operations are shown in Figure . The increase in the CR increases the peak pressure. This may be due to the better burning of the fuel combination at a rapid combustion phase and a shorter diffusion combustion phase occurring at a higher CR. However, at a higher CR of 17.5, the peak pressure was found to be lower for the HOME–producer gas operation under a dual-fuel mode than for the diesel–producer gas operation. This may be due to the combined effect of the lower calorific value of HOME and producer gas and the lower flame velocity, higher viscosity and density of HOME, leading to poor combustion at the rapid combustion phase. The peak pressure obtained at the CRs of 15, 16 and 17.5 was found to be 65, 69.89 and 71.8 bar, respectively, for the HOME–producer gas operation compared with the value of 77 bar for the diesel–producer gas operation. On an average, for the HOME–producer gas operation at different CRs, the peak pressure was increased by 9.87% when the CR was increased from 15 to 17.5, respectively. It was increased by 9.65 and 2.5% when the CR was raised from 15 to 17.5 and from 16 to 17.5, respectively. It is observed that peak pressure increased by 6.65% for diesel–producer gas operation at the same CR of 17.5 compared to HOME–producer gas operation.
The effect of brake power on the ignition delay is shown in Figure . The ignition delay is calculated based on the static IT. The dual-fuel operation with HOME–producer gas at different CRs shows the variations in ignition delay. It could be due to the large amount of producer gas taking part at the rapid combustion phase, the variations in the air–producer gas mixture and the combustion temperature at different CRs. Compared with the diesel–producer gas operation at the same CR, the HOME–producer gas operation shows a longer ignition delay. This may be due to the variations in the air–producer gas mixture, the lower calorific value of both HOME and producer gas, the lower flame temperature of the producer gas and the higher viscosity of HOME. Hence, it requires more time for burning. However, it is observed that lower ignition delay at a higher CR of 17.5 was obtained and could be due to increased combustion temperature. The ignition delay values obtained at the CRs of 15, 16 and 17.5 CR were found to be 21.5, 19.78 and 15.48° CA, respectively, for the HOME–producer gas operation compared with the value of 15° CA for the diesel–producer gas operation. On an average, for the HOME–producer gas operation at different CRs, the ignition delay was decreased by 27.8% when the CR was increased from 15 to 17.5, respectively. It was decreased by 21.5% when the CR was raised from 16 to 17.5, respectively. A decrease in ignition delay by about 4.32% was observed at the same CR of 17.5 for diesel–producer gas operation compared to HOME–producer gas operation.
Combustion duration, shown in Figure , was calculated based on the duration between the start of combustion and 90% cumulative heat release. The combustion duration increases with an increase in the power output for all the dual-fuel combinations. This is due to the increase in the quantity of the fuel injected. A higher combustion duration was observed for the HOME–producer gas operation, whereas a lower combustion duration was found for the diesel–producer gas operation. This could be due to the improper mixing of air–fuel, leading to a longer mixing time and hence incomplete combustion with a longer diffusion combustion phase. However, from Figure , it can be observed that the combustion duration was reduced and improved at a higher CR of 17.5 for the HOME–producer gas operation. This could be due to the improper mixing of air–producer gas, the lower adiabatic flame temperature of the producer gas, the high viscosity of the liquid fuel used and the reduced heat release rate obtained at a lower CR for the HOME–producer gas operation. The second peak was observed at the diffusion-burning phase and was greater for the HOME–producer gas operation than for the diesel–producer gas operation. This may also be due to the higher viscosity of HOME and the reduction in air–fuel mixing rates along with the slow-burning producer gas. This leads to small amount of fuel being used during the rapid combustion after ignition delay for HOME–producer gas operation. Therefore, a large amount of burning occurs at the diffusion phase rather than at the premixed phase for the HOME–producer gas operation. Significantly higher combustion rates during the later stages lead to higher exhaust temperatures and lower thermal efficiency for the HOME–producer gas operation. However, HOME–producer gas operation at a higher CR of 17.5 shows improvement in heat release rate compared with the HOME–producer gas operation at the lower CRs of 15 and 16. At the 80% load, the combustion duration values were found to be 54.2, 48.2 and 39.8° CA at the CRs of 15, 16 and 17.5, respectively, for the HOME–producer gas operation and the value of 34.8° CA at the CR of 17.5 for the diesel–producer gas operation. On an average, for the HOME–producer gas operation at different CRs, the combustion duration was decreased by 26.5% when the CR was increased from 15 to 17.5, respectively. It was decreased by 17.4% when the CR was raised from 16 to 17.5, respectively. However, a decrease in combustion duration of about 12.4% was observed at the same CR of 17.5 for the diesel–producer gas operation compared with that for the HOME–producer gas operation.
Figure shows in-cylinder pressure versus crank angle for the different HOME–producer gas combinations at different CRs. The peak pressure depends on how much fuel was consumed and how combustion was taking place during the rapid combustion period. The uncontrolled combustion phase is governed by the ignition delay period and the mixture preparation during the delay period. Therefore, mixture preparation and the slow-burning nature of the producer gas during the ignition delay period are responsible for this observed trend of peak pressure and the maximum rate of pressure rise. From Figure , it can be observed that, at the same brake power, the IMEP was comparatively lower for HOME than for diesel, as its peak pressure and the rate of pressure rise are lower.
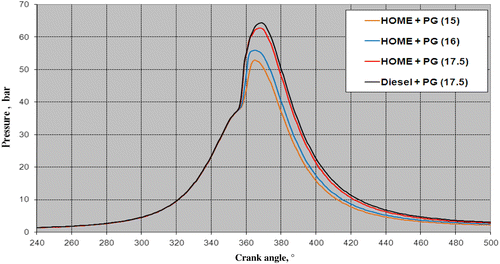
The results showed that the HOME–producer gas operation at the CR of 17.5 exhibits a higher peak pressure, as shown in Figure . The pressure is higher for the HOME–producer gas operation at the CR of 17.5 than for the HOME–producer gas operation at the CRs of 15 and 16. This could be due to the combined effect of the longer ignition delay, the lower adiabatic flame temperature and the slow-burning nature of the producer gas. Based on these results, the second peak was observed during the diffusion combustion phase for the HOME–producer gas operation at varying CRs compared with that for the diesel–producer gas operation at the same CR of 17.5. This could be attributed to the incomplete combustion due to the higher viscosity of HOME, the poor quality of the producer gas and the reduction in air entrainment and air–fuel mixing rates together with the slow-burning nature of the producer gas.
Figure shows heat release rate versus crank angle for the different HOME–producer gas combinations at different CRs. The HOME–producer gas operation at the CRs of 15 and 16 exhibited a lower heat release rate than the operation at the CR of 17.5. This is due to the result of a higher second peak obtained at the CRs of 15 and 16 at the diffusion combustion phase compared with that obtained for the dual-fuel operation at the CR of 17.5.
5. Conclusions
Some important findings on the engine performance and environmental aspects under the dual-fuel mode of operation while using the producer gas derived from the babul wood and HOME as the injected fuel with varying CRs were made from the present study:
Operation of the HOME and Gasifier – engine system with optimum engine parameters makes the system completely independent from fossil fuels. HOME can be used with producer gas induction under a dual-fuel mode, and this feature does not require any major engine modifications.
Results on the integrated gasifier–engine system favoured a higher CR.
On an average, for the HOME–producer gas operation at different CRs, the BTE was increased by 39.1% when the CR was increased from 15 to 17.5, respectively. It was increased by 24.4% when the CR was raised from 16 to 17.5, respectively. The EGT was reduced by 26% when the CR was increased from 15 to 17.5, respectively. It was reduced by 18.9% when the CR was raised from 16 to 17.5, respectively.
Smoke opacity was reduced by 20.5% when the CR was increased from 15 to 17.5, respectively. It was reduced by 11.9% when the CR was raised from 16 to 17.5, respectively. The CO emission levels were reduced by 44.5% when the CR was increased from 15 to 17.5, respectively. It was reduced by 36.8% when the CR was raised from 16 to 17.5, respectively. Similarly, the HC emission levels were reduced by 30.2% when the CR was increased from 15 to 17.5. It was reduced by 21.23% when the CR was raised from 16 to 17.5, respectively. The NO x emission levels were increased by 24.4% when the CR was increased from 15 to 17.5. It was reduced by 19.1% when the CR was raised from 16 to 17.5, respectively.
For the HOME–producer gas operation at different CRs, the peak pressure was increased by 9.65% when the CR was increased from 16 to 17.5, respectively. It was increased by 2.5% when the CR was raised from 16 to 17.5, respectively. An increase in peak pressure of about 6.5% was observed at the same CR of 17.5 for the diesel–producer gas operation compared with that for the HOME–producer gas operation.
Percentage values of the fuel substituted at a higher CR of 17.5 were found to be 65.18 and 56.66%, respectively, for the diesel–producer gas and HOME–producer gas operations at the 80% load.
Appropriate use of turbocharging in a producer gas-fuelled dual-fuel engine could further improve the power output.
Notes
1. Email: [email protected]
References
- Banapurmath, N. R. 2008. “Performance, Combustion and Emission Characteristics of a Single Cylinder Direct Injection CI Engine Operated on Dual Fuel Mode Using Honge Oil and Producer Gas.” PhD thesis. 1–195.
- Banapurmath, N. R. , P. G. Tewari , and R. S. Hosmath . 2008. “Experimental Investigations of a Four-Stroke Single Cylinder Direct Injection Diesel Engine Operated on Dual Fuel Mode with Producer Gas as Inducted Fuel and Honge Oil and Its Methyl Ester (HOME) as Injected Fuels.” Renewable Energy 33 (9): 2007–2018.
- Banapurmath, N. R. , P. G. Tewari , V. S. Yaliwal , Satish Kambalimath , and Y. H. Basavarajappa . 2009. “Combustion Characteristics of a 4-Stroke CI Engine Operated on Honge Oil, Neem and Rice Bran Oils When Directly Injected and Dual Fuelled with Producer Gas Induction.” Renewable Energy 34 (7): 1877–1884.
- Banapurmath, N. R. , V. S. Yaliwal , Satish Kambalimath , A. M. Hunashyal , and P. G. Tewari . 2011a. “Effect of Wood Type and Carburetor on the Performance of Producer Gas–Biodiesel Operated Dual Fuel Engines.” Waste and Biomass Valorization 2 (4): 1–11.
- Banapurmath, N. R. , V. S. Yaliwal , Satish Kambalimath , A. M. Hunashyal , and P. G. Tewari . 2011b. “Effect of Biomass feed stock, carburetor, Compression Ratio and Biodiesel- Ethanol Blends on the Performance of Dual Fuel Engine.” Waste and Biomass Valorization 2 (4): 403–413. doi: 10.1007/s12649-011-9083 .
- Churchill, D. B. , S. L. Hedden , J. D. Whitney , and L. N. Shaw . 1986. “Chipping Citrus Wood for Gasification.” Applied Engineering in Agriculture 2 (2): 238–240.
- Deepak, A. , and A. A. Kumar . 2007. “Performance and Emission Characteristics of a Jatropha Oil (Preheated and Blends) in a Direct Injection Compression Ignition Engine.” International Journal of Applied Thermal Engineering 27: 2314–2323.
- Karim, G. A., W. Jones, and R. R. Raine . 1989. “An Examination of the Ignition Delay Period in Dual Fuel Engines.” Society of Automotive Engineers, Paper No. 892140.
- Karim, G. A., R. R. Raine, and W. Jones . 1988. “An Examination of Cyclic Variations in a Dual Fuel Engine.” Society of Automotive Engineers, Paper No. 881661.
- Muralidharan, K. , and D. Vasudevan . 2011. “Performance, Emission and Combustion Characteristics of a Variable Compression Ratio Engine Using Methyl Esters of Waste Cooking Oil and Diesel Blends.” Applied Energy 88 (11): 3959–3968.
- Nwafor, O. M. I. 2000. “Effect of Advanced Injection Timing on the Performance of Rapeseed Oil in Diesel Engines.” Renewable Energy 21: 433–444.
- Nwafor, O. M. I. 2003. “The Effect of Elevated Fuel Inlet Temperature on Performance of Diesel Engine Running on Neat Vegetable Oil at Constant Speed Conditions.” Renewable Energy 28: 171–181.
- Nouni, M. R. , S. C. Mullick , and T. C. Kandpal . 2007. “Biomass Gasifier Projects for Decentralized Power Supply in India: A Financial Evaluation.” Energy Policy 35: 1373–1385.
- Parikh, P. P. , A. G. Bhave , D. V. Kapse , and S. Shashikantha . 1989. “Study of Thermal and Emission Performance of Small Gasifier–Dual-Fuel Engine Systems.” Biomass 19: 75–97.
- Raheman, H. , and S. V. Ghadge . 2008. “Performance of Diesel Engine with Biodiesel at Varying Compression Ratio and Ignition Timing.” Fuel 87: 2659–2666.
- Ramadas, A. S. , S. Jayaraj , and C. Muralidharan . 2006. “Power Generation Using Coir Pith and Wood Derived Producer Gas in a Diesel Engine.” Fuel Processing Technology 87: 849–853.
- Ravindranath, N. H. , and P. Balachandra . 2009. “Sustainable Bioenergy for India: Technical, Economic and Policy Analysis.” Energy 34: 1003–1013.
- Roy, Murari Mohon, Eiji Tomita, Nobuyuki Kawahara, Yuji Harada, and Atsushi Sakane . 2009. “Effect of Fuel Injection Parameters on Engine Performance and Emissions of a Supercharged Producer Gas–Diesel Dual Fuel Engine.” Society of Automotive Engineers. Paper No. 2009-01-1848.
- Sahoo, B. B. , N. Sahoo , and U. K. Saha . 2009. “Effect of Engine Parameters and Type of Gaseous Fuel on the Performance of Dual-Fuel Gas Diesel Engines – A Critical Review.” Renewable and Sustainable Energy Reviews 13: 1151–1184.
- Samir, J. D. , B. B. Lalit , and B. T. Shashikant . 2008. “Investigation on Performance and Emission Characteristics of CI Engine Fuelled with Producer Gas and Esters of Hingan (Balanites) Oil in Dual Fuel Mode.” International Journal of Mechanical Systems Science and Engineering 2 (3): 148–153.
- Sayin, Cenk , and Metin Gumus . 2011. “Impact of Compression Ratio and Injection Parameters on the Performance and Emissions of a DI Diesel Engine Fueled with Biodiesel Blended Diesel Fuel.” Applied Thermal Engineering 31: 3182–3188.
- Scholl, K. W., and S. C. Sorenson . 1993. “Combustion Analysis of Soyabean Oil Methyl Ester in a Direct Injection Diesel Engine.” Society of Automotive Engineers. Paper No. 930-934.
- Selim, Mohamed Y. E. 2004. “Sensitivity of Dual Fuel Engine Combustion and Knocking Limits to Gaseous Fuel Composition.” Fuel 45 (3): 411–425.
- Singh, R. N. , S. P. Singh , and B. S. Pathak . 2007. “Investigations on Operation of CI Engine Using Producer Gas and Rice Bran Oil in Mixed Fuel Mode.” Renewable Energy 32: 1565–1580.
- Sridhar, G. , H. V. Sridhar , S. Dasappa , P. J. Paul , N. K. S. Rajan , and H. S. Mukunda . 2005. “Development of producer gas engines.” Proceedings of the Institution Mechanical Engineers, Part D: Journal of Automobile Engineering 219: 423–438.
- Skoulou, V. , G. Koufodimos , Z. Samaras , and A. Zabaniotou . 2008. “Low Temperature Gasification of Olive Kernels in a 5-kW Fluidized Bed Reactor for H2-Rich Producer Gas.” International Journal of Hydrogen Energy 33: 6515–6524.
- Yaliwal, V. S. , N. R. Banapurmath , S. R. Daboji , and P. G. Tewari . 2010. “Production and Utilization of Renewable Liquid Fuel in a Single Cylinder Four Stroke Direct Injection Compression Ignition Engine.” International Journal of Engineering Science and Technology 2 (10): 5938–5948.