Abstract
Alternative fuels have numerous advantages compared to fossil fuels as they are renewable, biodegradable; provide energy security and foreign exchange saving besides addressing environmental concerns and socio-economic issues as well. Renewable fuels can be used predominantly as fuel for both transportation and power generation applications. Improved engine performance with reduced engine exhaust emissions is a major research objective in engine development. Today, the use of biomass derived producer gas is more relevant for addressing rural power generation and is a promising technique for controlling both nitric oxide (NOx) and soot emission levels. In view of this, exhaustive experiments on the use of Honge oil methyl ester (HOME)–Producer gas in a dual fuel engine have been carried out with an intension of improving its fuel efficiency. This paper mainly presents results on a single cylinder four stroke direct injection diesel engine operated in dual fuel mode using HOME–Producer gas combination with and without bio-ethanol addition and thermal barrier coating (TBC). Further, the results were compared with diesel–producer gas mode of operation. Experimental investigation on dual fuel operation using HOME+5% bioethanol (BE5)–Producer gas operation with TBC showed 12.35% increased brake thermal efficiency with decreased hydrocarbon and carbon monoxide emissions and increased NOx emission levels compared to HOME–Producer gas mode of operation.
1. Introduction
Fossil fuels have long been used as the predominant fuel all over the world. However, in the present day, concerns for the greenhouse gas emissions along with energy security are spawning greater interest in renewable and alternative sources of energy for power generation applications. In 1998, the total world reserves were estimated to be about 2.1–2.8 trillion barrels. In 2004, it was 1.50 trillion barrels, and in 2010, it was decreased to 1–1.2 trillion barrels (http://www.saltmarch.com/pressRelease2.html). The increasing industrialization and motorization of the world has led to a steep rise in the pollutants. In view of this, the use of renewable and alternative fuels may reduce dependency on imported fuels and reduces addition of exhaust emissions to the environment. Biofuels derived from biomass such as biodiesel, bioethanol and producer gas are the most promising alternative fuels for engine applications as they are renewable, biodegradable and provide energy security and foreign exchange saving besides addressing environmental concerns and have emerged as a potential alternative substitute for diesel fuel. The substitution of even a small fraction of total consumption by alternative fuels will have a significant impact on the economy and the environment (Anand, Sharma, and Mehta Citation2011).
Biodiesel, bioethanol production, properties of both biodiesel and ethanol and their utilization in diesel engines have been reported (Vivek and Guptha Citation2004; Dien et al. Citation2006; Pérez et al. Citation2008; Park et al. Citation2008; Yaliwal et al. Citation2013). Utilization of vegetable oil and its related problems when used directly in diesel engines have been reported in the literature (Banapurmath, Tewari, and Vinod Kumar Citation2009; Liang et al. Citation2009; Yaliwal et al. Citation2010; Xue, Grift, and Hansen Citation2011). Biodiesel operated diesel engine results in a reduced engine performance with increased emission levels such as hydrocarbon (HC) and carbon monoxide (CO) has been reported. Improved performance with lower emission levels have been reported with diesel/biodiesel–bioethanol (DE/BE) blends compared to neat biodiesel operation. Additives improve ignition delay and mitigate cycle irregularity. Addition of ethanol in diesel/biodiesel greatly reduces the emission levels such as HC and CO emission levels with considerable improvement in brake thermal efficiency (BTE). Studies on ethanol–diesel blend confirm substantial reductions in particulate matter emission and higher oxides of nitrogen (NOx) emission. From the engine durability point of view, blends with up to 15% (by vol.) ethanol in diesel/biodiesel fuel can be considered relatively safe (Likos, Callahan, and Moses Citation1982; Banapurmath, Hosmath et al. Citation2011).
Decreased performance with biodiesel operation can also be improved by utilizing thermal barrier coating (TBC) on the combustion surfaces. In an LHR engine, some part of the heat transfer loss will be recovered, leading to increased performance and reduced emission levels. Many investigators have studied various ceramic materials as coating for diesel engine application (Miller Citation1987; Kamo et al. Citation1999; Jaichandar and Tamilporai Citation2003; Ekrem, Tahsin, and Muhammet Citation2006; Rakopoulos and Giakoumis Citation2006; Banapurmath and Tewari Citation2008). Based on the literatures, partially stabilized Zirconia (PSZ) has been found to be quite desirable for LHR engine application. Structure, durability with various TBCs, increased thermal efficiency and decreased specific fuel consumption and volumetric efficiency with reduced HC and CO and increased NOx emission levels have been reported. Further, shorter ignition delay and decreased premixed fraction with an increase in the amount of fuel burnt during the diffusion phase of combustion has been reported (Rakopoulos and Giakoumis Citation2006; Banapurmath and Tewari Citation2008).
Use of gaseous fuels such as producer gas, liquefied petroleum gas (LPG) and compressed natural gas (CNG) in diesel engines have been reported. Dual fuel engine operated on these gaseous fuels results in slightly lower power output with increased HC and CO and lower smoke and NOx emission levels (Karim, Jones, and Raine Citation1989; Parikh et al. Citation1989; Papagiannakis and Hountlas Citation2003; Ramadas, Jayaraj, and Muralidharan Citation2006; Roy et al. Citation2009; Sahoo, Sahoo, and Saha Citation2009; Palash et al. Citation2013; Dasappa and Sridhar Citation2011; Wategave et al. Citation2013). Incomplete combustion, irreversibility, lower calorific value, improper mixing of gas with air, lower flame velocity and adiabatic flame temperature could be the reasons for this behaviour. Several literatures on performance combustion and emission characteristics of diesel engine operating on dual fuel mode using diesel/biodiesel and producer gas induction have been reported in the literature. Based on the literatures, it is observed that, 60–90% liquid fuel saving. Heating value and stoichiometric air–producer gas ratio quantitatively affect the dual fuel engine performance. Various methods to improve the producer gas fuelled dual fuel engine such as advancing injection timing, increased compression ratio and enhanced hydrogen utilization has been reported (Parikh et al. Citation1989; Ramadas, Jayaraj, and Muralidharan Citation2006; Banapurmath, Tewari, and Vinod Kumar Citation2009; Banapurmath, Yaliwal et al. Citation2011; Sahoo and Saha Citation2011; Roy et al. Citation2009; Dasappa and Sridhar Citation2011).
The review of literature suggests that the effect of bioethanol and TBC on the performance of producer gas-diesel/biodiesel dual fuel engine has been less investigated. Hence, this area still needs detailed investigation. In this context, the present study was carried out with the main objective to study the effect of bioethanol and TBC properties on the performance, combustion and emission characteristics of single cylinder four stroke direct injection stationary diesel engine operating on dual fuel mode using Honge oil methyl ester (HOME) and producer gas induction. In the present work, an effort has been made to enhance the fuel efficiency of HOME–Producer gas operation using bio-ethanol blending and TBC.
2. Characterization of fuels used
The properties of fuels are among the key factors that influence the performance, combustion and emission levels of dual fuel engine. Therefore, characterization of fuels is the first step for efficient utilization of its energy potential. For the present work HOME and producer gas derived from biomass feed stock of Babul or ordinary (OW) were used. The different properties of the fuels used were determined as shown in Tables and . Table shows the properties of liquid fuels and proximate and ultimate analysis of biomass feed stock used in the present study. The composition of producer gas derived from babul wood is shown in Table .
Table 1 Properties of liquid fuels and proximate and ultimate analysis of Babul wood.
Table 2 Composition of producer gas derived from babul wood.
3. Experimental setup
Experimental investigations were conducted on four-stroke single cylinder direct injection water cooled compression ignition engine coupled with down draft gasifier is shown in Figure (a),(b). The specification of the engine and gasifier is given in Tables and . The engine was always operated at a rated speed of 1500 rev/min. The engine was having a conventional fuel injection system. The fuel consumption was measured with burette and stopwatch. A calibrated venturimeter for gas flow along with a digital gas flow meter was used to measure the producer gas flow rate. Air flow rate was measured using air-box method fitted with orifice plate. The injector opening pressure and static injection timing as specified by the engine manufacturer were 205 bar and 23° before top dead centre (bTDC), respectively. The injector is fitted to the nozzle tester and checked for the spray pattern at a given injector opening pressure before fitting it onto the engine. For the present study, the injection timing and injection opening pressures of 27° bTDC and 230 bar was used with dual fuel operation. However, for Diesel–Producer gas operation injection pressure was kept at 205 bar. The engine had been provided with a hemispherical combustion chamber with overhead valves operated through push rods. The cylinder pressure was measured with Piezo electric transducer fitted in the cylinder head as shown in Figure . Five gas analyser and Hart ridge smoke meter was used to measure HC, CO, CO2, NOx and smoke opacity. Tables and show the specification of the exhaust gas analyser and smoke meter. For air and gas mixing, a parallel flow gas entry carburetor was used in the experimentation and is shown in Figure . The amount of injected fuel i.e., biodiesel is varied using a suitable mechanism and accordingly the gas flow rate was varied. The air-to-producer gas mixture ratio was controlled using a carburettor suitably designed for the purpose. Figure shows photographic view of a Downdraft Gasifier. The biodiesel was blended with ethanol to get three different fuel blends each ranging from 0% to 15% with an increment of 5%. The homogeneity of HOME and bioethanol mixture was ensured by placing the test tube containing the mixture above the black paper and the solution was checked for transparency without any turbidity Indicating good miscibility of bioethanol in HOME (Anand, Sharma, and Mehta Citation2011). Also, a mechanical stirrer was used to mix HOME and bioethanol blends. Figure shows the mechanical stirrer for ensuring proper mixing to prevent phase separation. The fuel blends were prepared just before starting the experiment to ensure that the fuel mixture is homogeneous. Figure shows flaring for checking quality of producer gas.
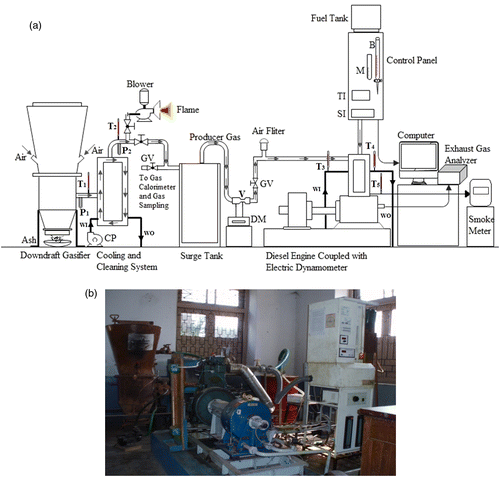
Table 3 Specification of experimental test rig.
Table 4 Specification of the downdraft gasifier.
Table 5 Specifications of exhaust gas analyser.
Table 6 Specifications of smoke meter.
Figure (a),(b) shows the ceramic coating of PSZ coating on cylinder head and piston respectively. Coating of 500 μm thickness was done on the combustion chamber and both inlet and outlet valves. The major problem associated with such coatings is that they have temporary life as they wear out very quickly. The particles removed can have a negative effect on the liner. All measurements were carried out when the engine was attained steady state. For each load, five readings were recorded and averaged out values were only considered while plotting the graphs to ensure accuracy of the data recorded and careful experimental arrangements were made to obtain consistent and repeatable measurements.
4. Results and discussions
Present experimental investigations were carried out in two phases. In the first phase of the work, HOME–bioethanol blend ratio was optimized. Further, in the next phase, engine testing with optimized blend ratio and the findings of Diesel–Producer gas and HOME–Producer gas operation were compared with BE5–Producer gas, BE10–Producer gas, BE15–Producer gas and HOME–Producer gas (PSZ) operation. Initially, experiments were conducted by using HOME and bioethanol blends in steps of 5% to optimize the blend ratio. The optimum parameters in terms of advanced injection timing, increased injection pressure, carburetor type and better biomass feed stock for gasification were kept constant and were reported in the earlier studies by the same authors (Banapurmath, Tewari, and Hosmath Citation2008; Banapurmath, Tewari, and Vinod Kumar Citation2009; Yaliwal et al. Citation2013). Babul wood was used as a fuel for the gasifier.
4.1 Optimization of HOME–bioethanol blend ratio for dual fuel operation
In this section, effect of HOME–bioethanol blend properties on the performance of producer gas fuelled dual fuel engine has been presented. BTE variation with 80% engine load for the studied fuels is shown in Figure . Results showed BE5–Producer gas operation resulted in higher BTE compared to BE10–Producer gas and BE15–Producer gas operation. Decreased BTE for BE10–Producer gas and BE15–Producer gas operation might be attributed to the combined effect of lower energy content of the blend with their higher fuel consumption for the same power output. During BE10–Producer gas and BE15–Producer gas operation, higher viscosity of the HOME and latent heat of vaporization of bioethanol leads poorer combustion than that of BE5–Producer gas combination. However, good volatility at lower bioethanol (BE5) content improves the atomization and spray characteristics. Based on the results, it is concluded that latent heat of vaporization of bioethanol at lower proportion does not have any negative impact on the performance; instead it helps to enhance the mixing of fuel combinations along with producer gas. The maximum BTE values for BE5–Producer gas and BE10–Producer gas and BE15–Producer gas operation at 80% load were found to be17.34%, 16.15% and 15.4%, respectively, compared to 20.95% for Diesel–Producer gas operation.
The obtained results in case of smoke opacity related to 80% engine load are presented in Figure . Formation of smoke is basically a process of conversion of molecules of HC fuels into particles of soot. The greater smoke opacity of BE10 –Producer gas and BE15–Producer gas operation is mainly attributed to emission of higher molecules of HC and particulate due to incomplete combustion. Presence of free fatty acids in an HOME (does not burn at higher percentage of bioethanol mixing), lower energy content and reduced cetane number of BE10 and BE15 and lower combustion temperature caused by the cooling effect of bioethanol are responsible for this trend. However, lower proportion of bioethanol helps to promote the blend to become volatile and improve the mixing tendency of fuel combination. The smoke opacity values for BE5–Producer gas, BE10–Producer gas and BE15–Producer gas operation at 80% load were found to be 48, 51 and 56 HSU compared to 32 HSU for Diesel–Producer gas operation, respectively.
Figures and shows the effect of blend properties on the HC and CO emission levels at 80% engine load. HC and CO emission is an indication of incomplete combustion of the premixed mixture. HC emissions in diesel engines are caused due to lean mixture during the delay period and over mixing of fuel leaving fuel injector nozzle at lower velocity. Experimental results showed that BE10–Producer gas and BE15–Producer gas operation resulted in increased HC and CO emissions compared to BE5–Producer gas operation. Decreased combustion efficiency, poor spray characteristics of injected fuel and improper mixing might be responsible for poor combustion with BE10–Producer gas and BE15–Producer gas operation. Lower energy density and negative effect of latent heat of vaporization with higher percentage of bioethanol in an HOME may also be responsible for this trend. The HC emission values for BE5–Producer gas, BE10–Producer gas and BE15–Producer gas operation at 80% load were found to be 44, 50 and 52 ppm compared to 38 ppm for Diesel–Producer gas operation respectively. Similarly, higher CO emission levels were observed for BE10–Producer gas and BE15–Producer gas operation compared to BE5–Producer gas operation. This could be attributed to incomplete combustion of the HOME+Producer gas combination with higher bioethanol proportion. Higher latent heat of bioethanol leads to cooling effect and hence, lower combustion temperature may be the reason for higher CO emission levels. Also, presence of already CO in producer gas may tend to increase the CO production in the exhaust. However, it is observed that, both HC and CO emission levels are lower at lower bioethanol content as compared to other BE10–Producer gas and BE15–Producer gas combinations tested. The CO emission values for BE5–Producer gas, BE10–Producer gas and BE15–Producer gas operation at 80% load were found to be 0.39%, 0.43% and 0.45%, respectively, compared to 0.31% for Diesel–Producer gas operation.
The engine's NOx emission levels for the analysed test fuels at 80% engine load are presented in Figure . Generally, higher NO emission levels were observed for the fuel combinations which have higher combustion efficiency. In general, when the injection timing is retarded, the combustion process gets retarded, resulting in lower NOx emission levels. For the same operating condition, NO concentration levels were found to be higher for BE5–Producer gas operation compared to BE10–Producer gas and BE15–Producer gas operation. It could be attributed to reduced cetane number, higher cooling effect caused by higher latent heat of vaporization and lower energy content of BE10–Producer gas and BE15–Producer gas combination compared to BE5–Producer gas operation. Higher combustion temperature and improved heat release rate during premixed combustion phase with BE5–Producer gas operation compared to BE10–Producer gas and BE15–Producer gas combination is responsible for this trend. Hence, BE5–Producer gas operation resulted in higher BTE with lower HC and CO emission levels. The NO emission values for BE5–Producer gas, BE10–Producer gas and BE15–Producer gas operation at 80% load were found to be 92, 85 and 75 ppm, respectively, compared to 110 ppm for Diesel–Producer gas operation.
4.2 Engine operation with optimized blend ratio and TBC
In the second phase of the work, effect of HOME–bioethanol blend and TBC on the performance of producer gas fuelled dual fuel engine has been explained in the subsequent paragraph.
4.2.1 Performance characteristics
4.2.1.1 Brake thermal efficiency
Figure shows variation in BTE with different modes of engine operation at 80% load. Highest BTE was observed with Diesel–Producer gas operation compared to HOME–producer gas operation. It could be due to lower calorific value of HOME and producer gas. Lower volatility character of HOME, improper mixing of air and fuel and lower flame temperature of producer gas are also responsible for this trend. The results are in close agreement with already published literatures (Parikh et al. Citation1989; Banapurmath, Tewari, and Hosmath Citation2008; Banapurmath, Tewari, and Vinod Kumar Citation2009; Banapurmath, Tewari, and Yaliwal Citation2010; Banapurmath, Yaliwal et al. Citation2011; Dasappa and Sridhar Citation2011; Sahoo, Sahoo, and Saha Citation2009; Ramadas, Jayaraj, and Muralidharan Citation2006; Singh, Singh, and Pathak Citation2007). However, bioethanol blending with HOME or adopting suitable TBC on combustion chamber surfaces improves BTE of engine. It could be attributed to improved spray characteristics of HOME due to increased volatility with bio-ethanol addition. Better mixture formation and enhanced flame velocity of air and gas mixture is also responsible for the observed trend. However, LHR engine operation with HOME–producer gas combination resulted in marginally improved performance. It may be due to enhanced flame velocity of air and gas mixture and reduced heat transfer rates. Further, increased BTE was observed with BE5–Producer gas operation with PSZ coating compared to other fuel combinations tested. This could be due to combined effect of increased volatility of liquid fuel and higher in-cylinder temperature, reduced irreversibility and heat transfer rates. In general, dual fuel operation with HOME–producer gas combination shows lower BTE compared to Diesel–Producer gas operation. The BTE obtained for Diesel–Producer gas, HOME–producer gas, BE5–Producer gas and HOME–producer gas (PSZ) and BE5–Producer gas (PSZ) operation were found to be 20.65%, 16.65%, 18.1%, 17.34% and 18.9%, respectively, at 80% load.
4.2.1.2 Exhaust gas temperature
The exhaust gas temperature (EGT) shown in Figure is relatively higher with HOME–producer gas operation compared to Diesel–producer gas operation for all modes of engine operation. It could be due to reduced premixed combustion phase with HOME–producer gas operation (Parikh et al. Citation1989; Banapurmath, Tewari, and Vinod Kumar Citation2009). However, the EGT for HOME–producer gas combination are slightly lower in bioethanol blended mode or insulated engine operation compared to HOME–producer gas operated normal engine operation. Bioethanol blending with HOME lowers the viscosity and increases the volatility as well. This may enhance the mixture formation tendency resulting in better mixing and burning of the fuel combination. Both bioethanol blending and TBC resulted in better combustion during premixed combustion phase caused by increased flame velocity of the air–fuel mixture. TBC lowers the heat transfer and reduces the irreversibility caused by the incomplete combustion due to improper fuel mixing and it facilitates the combustion favourably.
Further, decreased EGT was observed with the BE5–Producer gas (PSZ) operation compared to other fuel combinations tested. It could be attributed to better mixing of fuel combinations due to increased volatility of HOME and increased combustion temperature due to reduced heat transfer rates. Also, use of oxygenated fuel (BE5) in presence of high temperature leads to better combustion. Higher combustion temperature due to PSZ coating can burn the high viscous fuel and increased flame velocity of air and gas mixture may also responsible for this trend. In general, dual fuel operation with HOME–producer gas combination always resulting in higher EGT compared to Diesel–Producer gas operation. The EGT obtained for Diesel–Producer gas, HOME–producer gas, BE5–Producer gas and HOME–producer gas (PSZ) and BE5–Producer gas (PSZ) operation were found to be 385, 495, 432, 445 and 418°C, respectively, at 80% load.
4.2.1.3 Volumetric efficiency
Figure shows variation of volumetric efficiency for HOME–producer operation with dual fuel mode of operation. Results showed a drop in volumetric efficiency with power output for all fuel combinations. It may be due to the fact that part of air was replaced by producer gas. HOME–Producer gas operation was resulted in lower volumetric efficiency compared to Diesel–Producer gas combination. Improper utilization of air as more HOME is injected for the same power generation with producer gas during dual fuel operation is responsible. However, BE5–Producer gas operation resulted in slightly higher volumetric efficiency. It could be attributed to injection of more oxygen in to the engine cylinder and better utilization of air due to increased volatility of HOME. More complete burning of the fuel combination due to better mixing of fuel combination with air and increased flame velocity of the gas and air mixture is also responsible for this trend. But, the volumetric efficiency was found to be lower with PSZ coated operation due to higher combustion temperature caused by ceramic coating compared to BE5–Producer gas operation. However, addition of 5% bioethanol to HOME and operating the engine with PSZ coating slightly improves volumetric efficiency. Addition of lower percentage of bioethanol to HOME may compensate the effect of higher combustion temperature. It could also be attributed to more complete burning of the fuel combination due to better mixing of the fuel combination. In general, dual fuel operation with HOME–producer gas combination resulted in lower volumetric efficiency compared to Diesel–Producer gas operation. The volumetric efficiency obtained for Diesel–Producer gas, HOME–producer gas, BE5–Producer gas and HOME–Producer gas (PSZ) and BE5–Producer gas (PSZ) operation were found to be 76%, 72%, 74%, 71.45% and 73.12%, respectively, at 80% load.
4.2.2 Emission characteristics
Emission Characteristics of an engine are important as for as environmental aspects is concerned. Combustion quality can be represented on the basis of emission levels from the engine. The emission levels from diesel engine is due to the variation of producer gas composition, type of liquid fuel used, engine design and operating parameters (Parikh et al. Citation1989; Banapurmath, Tewari, and Hosmath Citation2008; Roy et al. Citation2009). The different emission parameters during the dual fuel mode of operation with different fuel combinations are discussed below.
4.2.2.1 Smoke opacity
Figure show the smoke levels with dual fuel mode of engine operation at 80% load. It is observed that smoke emissions were higher for HOME–Producer gas operation compared to Diesel–Producer gas operation. Higher smoke levels from HOME–producer gas operation is mainly caused by incomplete combustion due to poor atomization, irreversibility and combined effect of lower calorific value of both HOME and Producer gas. Incomplete combustion due to burning of higher viscosity fuel in presence of slow burning producer gas is also responsible for the observed trend. The results are in close agreement with published literatures (Parikh et al. Citation1989; Dasappa and Sridhar Citation2011; Banapurmath, Tewari, and Hosmath Citation2008; Banapurmath, Tewari, and Vinod Kumar Citation2009; Banapurmath, Yaliwal et al. Citation2011; Sahoo, Sahoo, and Saha Citation2009; Ramadas, Jayaraj, and Muralidharan Citation2006; Singh, Singh, and Pathak Citation2007; Roy et al. Citation2009). However, it is observed that, smoke emission levels were found to be lower for BE5–Producer gas and HOME–Producer gas (PSZ) operation than normal engine operated on HOME–Producer gas operation. It could be due to slightly better combustion caused by the enhanced volatility of HOME with bioethanol blending and enhanced soot oxidation. Also, in case of TBC or LHR facility, lower smoke emission levels were observed for the same fuel combination. It could be attributed to higher combustion temperature causing increased flame velocity resulting in better conversion of fuel energy in to work. However, BE5-bioethanol operation with PSZ coating further reduces smoke emission levels compared to other fuel combinations tested. It could be due to more complete burning of the fuel combination caused by better soot oxidation due to addition of more oxygen in presence of high combustion temperature. As an overall comparison it is observed that the dual fuel operation with HOME–producer gas combination resulted in lower smoke emission levels when engine was operated on ethanol blending and TBC. The smoke emission levels obtained for Diesel–producer gas, HOME–producer gas, BE5–Producer gas and HOME–Producer gas (PSZ) and BE5–Producer gas (PSZ) operation were found to be 32, 58, 48, 46 and 42 HSU, respectively, at 80% load.
4.2.2.2 HC and CO emissions
Figures and shows HC and CO emission levels at 80% power output. Results showed lower HC and CO emission levels for Diesel–Producer gas combination compared to HOME–Producer gas operation. It could be due to incomplete combustion of HOME–Producer gas combination and presence of CO already in a producer gas (Parikh et al. Citation1989; Dasappa and Sridhar Citation2011; Banapurmath, Tewari, and Hosmath Citation2008; Banapurmath, Tewari, and Vinod Kumar Citation2009; Ramadas, Jayaraj, and Muralidharan Citation2006; Singh, Singh, and Pathak Citation2007; Roy et al. Citation2009). It is observed that HC and CO emission levels are reduced slightly with bioethanol addition to HOME or TBC operation compared to normal engine operated on the same fuel combination. Improved combustion caused by the improved spray characteristics due to increased volatility of HOME by adding bioethanol is responsible for this trend. Also, reductions in emission levels were observed for HOME–Producer gas combination when operated on LHR or coated engine. It could be due to better burning of high viscous fuel caused by the increased combustion and wall temperature. However, BE5–Producer gas operation with PSZ coating resulted in further reduction of HC and CO emissions compared to other fuel combinations tested. Use of oxygenated fuels in presence of high combustion temperature promotes better combustion i.e. oxygen availability in BE5 blend is comparatively higher and with operation of PSZ coating; carbon easily combines with oxygen and lowers CO emission levels. This is the reason for HC and CO emission reduction with BE5–Producer gas (PSZ) operation. Increased volatility of HOME and combustion temperature and decrease in heat losses going to cooling and surrounding causes more unburned HC of high viscous HOME to be added to the combustion. This feature significantly lowers both HC and CO emissions. Hence, it could be concluded that engine operation with more oxygen addition and PSZ coating contributes positively to higher combustion efficiency. In general, dual fuel operation with Diesel–Producer gas combination resulted in lower HC and CO emission levels compared to HOME–Producer gas operation. The HC emission levels obtained for Diesel–Producer gas, HOME–Producer gas, BE5–Producer gas and HOME–Producer gas (PSZ) and BE5–Producer gas (PSZ) operation were found to be 38, 55, 44, 43 and 41 ppm, respectively at 80% load. Similarly, CO emission levels obtained for Diesel–Producer gas, HOME–Producer gas, BE5–Producer gas and HOME–Producer gas (PSZ) and BE5–Producer gas (PSZ) operation were found to be 0.31, 0.48, 0.39, 0.41 and 0.36% respectively at 80% load.
4.2.2.3 NOx emission
Figure show NOx variation with dual fuel mode of engine operation. NOx emission is lower with HOME–Producer gas combination compared to Diesel–Producer gas operation. It could be attributed to lower combustion rates due to increased ignition delay and EGT for HOME–Producer gas operation (Dasappa and Sridhar Citation2011; Banapurmath, Tewari, and Hosmath Citation2008; Banapurmath, Tewari, and Vinod Kumar Citation2009; Sahoo, Sahoo, and Saha Citation2009; Ramadas, Jayaraj, and Muralidharan Citation2006). However, bioethanol blending with HOME resulted in marginally similar NOx emissions. Whereas, LHR engine operation resulted in slightly higher NOx emissions than the normal engine operated on HOME–Producer gas combination. Better combustion of the fuel mixture during premixed combustion phase due to increased gas and cylinder wall temperature is responsible for this observed trend. However, BE5–Producer gas operation resulted in slightly higher NO emission levels compared to HOME–producer gas combination. It could be attributed to better burning of the fuel combination resulting higher cylinder pressure due to improved premixed combustion phase. In general, dual fuel operation with Diesel–Producer gas combination recorded higher NOx emission levels compared to HOME–Producer gas operation. The NOx emission levels obtained for Diesel–Producer gas, HOME–Producer gas, BE5–Producer gas and HOME–Producer gas (PSZ) and BE5–Producer gas (PSZ) operation were found to be 110, 78, 84, 98 and 92 ppm, respectively, at 80% load.
4.2.3 Combustion characteristics
4.2.3.1 Ignition delay
Figure show the variation of ignition delay with dual fuel mode of engine operation at 80% load. Ignition delay is longer for HOME–Producer gas operation compared to Diesel–Producer gas operation. Improper spray pattern and reduced mixing rates of air–producer gas combination with HOME and reduced heat release rate during premixed combustion phase is responsible for this trend (Banapurmath, Tewari, and Hosmath Citation2008; Banapurmath, Tewari, and Vinod Kumar Citation2009). However, marginal decrease in ignition delay was observed with bioethanol blending with HOME or LHR engine operation. It could be attributed to better spray pattern along with enhanced mixing rates due to reduced volatility of HOME when bioethanol was blended. By examining the Figure , it is observed that lower diffusion combustion phase with both 5% bioethanol blended fuel and TBC operation compared to the respective one under normal dual fuel operation. This could be due to the fact that total burning rate during premixed combustion phase was comparatively higher. Increased volatility of HOME due to bioethanol addition or increased combustion temperature and increased chemical kinetics during the delay period and flame velocity due to bioethanol addition to HOME and/or TBC is responsible for this observed trend. This feature may progress enough the combustion of the producer gas along with liquid fuel and contributes significantly to the fastest growth of the flame front surrounding the burning zone. This is the result of improvement of the producer gas combustion quality caused by the bioethanol addition and TBC. In general, ignition delay of the injected fuels was found to increase with HOME–Producer gas dual fuel mode of operation compared to Diesel–Producer gas operation. The ignition delay obtained for Diesel–Producer gas, HOME–Producer gas, BE5–Producer gas and HOME–Producer gas (PSZ) and BE5–Producer gas (PSZ) operation were found to be 11.2°, 14.1°, 12.28°, 12.38° and 12.012° CA, respectively, at 80% load.
4.2.3.2 Combustion duration
Figure shows the variation of combustion duration with different fuel combinations operated on dual fuel mode with and without bioethanol and TBC. Combustion duration values were found to be higher for normal dual fuel engine operated on HOME–Producer combination compared to Diesel–Producer gas combination. Reduced heat release rate during premixed combustion phase caused by the improper mixing of fuel combination is the reason for the observed trend with HOME–Producer combination. However, this operation with bioethanol blending to HOME or TBC leads to a shorter combustion duration compared to normal dual fuel engine. It may be attributed to the decreased ignition delay caused by better mixing rates and higher combustion temperature leading to improved combustion quality. Increased flame velocity due to enhanced volatility caused by addition of bioethanol to HOME and/or increased combustion temperature due to the use of TBC is also responsible for this trend. However, BE5–Producer gas (PSZ) operation resulted in lower combustion duration compared to other fuel combinations tested. It could be attributed to effect of improved combustion due to enhanced premixed combustion caused by burning of oxygenated fuel in presence of high temperature. In general, HOME–Producer gas dual fuel operation resulted in higher combustion duration compared to Diesel–Producer gas operation due to better burning characteristic of the diesel. The combustion duration obtained for Diesel–Producer gas, HOME–Producer gas, BE5–Producer gas and HOME–Producer gas (PSZ) and BE5–Producer gas (PSZ) operation were found to be 40.65°, 46.0°, 43.1°, 43.8° and 42.12° CA, respectively at 80% load.
4.2.3.3 Peak pressure
Variation of peak pressure for Diesel–Producer gas and HOME–Producer gas operation with or without bioethanol and TBC are presented in Figure . Higher peak pressure was observed with Diesel–Producer gas operation compared to HOME–Producer gas combination. Better burning at rapid combustion phase occurring with Diesel–Producer gas combination is responsible for this trend. However, peak pressure with bioethanol blended HOME–producer gas operation under dual fuel mode of operation was found to be higher compared to HOME–producer gas operation. This may be attributed to improved fuel mixing rate caused by the increased volatility of HOME in presence of lower content of bioethanol and increased flame velocity of air–gas mixture leading to better combustion. However, TBC might also increases the peak pressure of HOME–Producer gas operation. It could be due to better combustion of high viscous HOME caused by higher combustion and wall temperature. However, BE5–Producer gas (PSZ) operation resulted in further reduction in combustion duration. Better mixing rates of the fuel combination and increased combustion efficiency due to improved spray pattern of injected fuel (BE5) and presence of high combustion temperature are responsible for this trend.
4.2.3.4 Cylinder pressure
Figure provides the variation of cylinder pressure with crank angle at 80% load for dual fuel operation. Lower cylinder pressure was observed for HOME–Producer gas fuelled dual fuel operation compared to Diesel–Producer gas combination. This could be the result of lower combustion rate, combined effect of lower calorific value of HOME and producer gas and longer ignition delay. By examining the Figure , it is observed that bioethanol addition to HOME and/or TBC affects the cylinder pressure history. HOME–Producer gas dual fuel operation with bioethanol addition or adopting TBC increases the cylinder pressure compared to normal dual fuel operation with same fuel combination. This could be attributed to sudden rise in the rapid combustion phase caused by the increased mixing rates along with decreased diffusion combustion phase. This positive effect may be due to increased volatility of fuel combination or presence of liquid fuel with high cylinder and wall temperature. This effect becomes more evident for HOME–Producer gas operation when lower percent of bioethanol was blended to HOME and/or TBC was used for high viscous fuelled engine. However, if positive effect of both bioethanol blending and TBC simultaneously harnessed, further it is possible to attain higher BTE. This is the improvement in combustion quality of combination caused by the improvement in the cylinder charge conditions. In general, normal dual fuel engine operated with HOME–producer gas combination and with bioethanol blending and TBC resulted in lower cylinder pressure compared to the one with Diesel–Producer gas combination. The cylinder pressure obtained for Diesel–Producer gas, HOME–producer gas combination ethanol and TBC were found to be 66.1, 49.8, 58.2 and 57.12 bar, respectively.
4.2.3.5 Heat release rate
Figure presents comparative data pertaining to heat release rate for the normal dual fuel operation with HOME–Producer gas combination with and without ethanol addition and/or TBC at optimum parameters. As far as the heat release rate curve is concerned, it is revealed that Diesel–Producer gas operation always resulted in higher heat release rate compared to HOME–Producer gas operation. This is because the premixed burning phase associated with a higher heat release rate is significant with Diesel–Producer gas dual fuel operation due to higher energy content of diesel and comparatively better mixing of the fuel combination with air. This is the reason for the higher thermal efficiency for Diesel–Producer gas operation. However, it is observed that for the same fuel combination of HOME and producer gas, dual fuel operation with 5% bioethanol addition to HOME and/or TBC resulted in slightly higher heat release rate. It could be attributed to better combustion caused by the better mixing of air and producer gas combination along with HOME, increased flame velocity of the gas and air mixture, reduced volatility of HOME with bioethanol addition and/or increased combustion and wall temperature due to use of TBC. Furthermore, increased fuel burning rate (due to the bioethanol blending and TBC) during premixed combustion phase rather than diffusion combustion phase, reduced irreversibility affect HOME–producer gas combustion positively.
5. Estimation of uncertainty
All experimental data were associated with uncertainties that ultimately limit the conclusions of the present study. Random errors arise from the fluctuations that can result from multiple trials of any given measurement. Systematic errors can be corrected by the periodic calibration of the instruments. The uncertainties of different quantities were estimated and are found to be 1.98%, 15.5%, 3.56%, 6.56%, 4.35%, 12.4% and 3.6% for BTE, EGT, volumetric efficiency, smoke opacity, CO, NOx and fuel substitution, respectively.
6. Conclusions
The following conclusions were made for the present study:
Operating HOME and Producer gas in Gasifier–engine system with optimum engine parameters makes the system completely independent from fossil fuel with improved performance. HOME can be used in dual fuel mode with producer gas induction and this feature does not require any major engine modifications.
The result on the integrated gasifier–engine system resulted in better performance with BE5–Producer gas operation and PSZ coating compared to other operating modes.
Dual fuel operation with BE5–Producer gas combination resulted in higher BTE with lower emission levels compared BE10–Producer gas and BE15–Producer gas combination.
On an average, for BE5–Producer gas operation with PSZ coating, BTE was increased by 12.35%, 8.5% and 4.4% compared to HOME–Producer gas, BE5–Producer gas and HOME–Producer gas (PSZ) operation, respectively. But, it was decreased approximately by 7.0% compared to Diesel–Producer gas operation at 80% load. Similarly, for BE5–Producer gas operation with PSZ coating, EGT was decreased by 15.6%, 3.2% and 6.1% compared to HOME–Producer gas, BE5–Producer gas and HOME–Producer gas (PSZ) operation, respectively. But, it was increased approximately by 8.6% compared to Diesel–Producer gas operation at 80% load.
On an average, Smoke emission levels were decreased by 27.1%, 12.5% and 8.7% for HOME–Producer gas, BE5–Producer gas and HOME–Producer gas (PSZ) operation compared to BE5–Producer gas operation with PSZ coating, respectively. But, it was increased approximately by 31.3% compared to Diesel–Producer gas operation at 80% load.
On an average, for BE5–Producer gas operation with PSZ coating, HC emission levels were decreased by 25.5%, 6.8% and 4.65% compared to HOME–Producer gas, BE5–Producer gas and HOME–Producer gas (PSZ) operation at 80% load, respectively. Similarly, for BE5–Producer gas operation with PSZ coating, CO emission levels were decreased by 25.0%, 7.7% and 12.2% compared to HOME–Producer gas, BE5–Producer gas and HOME–Producer gas (PSZ) operation, respectively. But, HC and CO were increased approximately by 7.9% and 16.1% compared to Diesel–Producer gas operation at 80% load.
On an average, for BE5–Producer gas operation with PSZ coating, NOx emission levels were decreased by 23.1%, 17.9% and 10.3% compared to HOME–Producer gas, BE5–Producer gas and HOME–Producer gas (PSZ) operation, respectively. But, it was increased approximately by 41.0% compared to Diesel–Producer gas operation at 80% load.
On the whole it is seen that dual fuel mode of operation with the all selected alternative fuel combinations and different operating modes resulted in lower performance compared to diesel–producer gas operation and smooth engine operation was observed. Among the selected alternative fuel combinations and operating modes, BE5–producer gas operation with PSZ coating resulted in slightly improved performance and decreased smoke, HC and CO emission levels compared to HOME–producer gas operation.
References
- Anand, K., R. P.Sharma, and P. S.Mehta. 2011. “Experimental Investigations on Combustion, Performance and Emissions Characteristics of Neat Karanja biodiesel and Its Methanol Blend in a Diesel Engine.” Biomass and Bioenergy35: 533–541.
- Banapurmath, N. R., and P. G.Tewari. 2008. “Performance of a Low Heat Rejection Engine Fuelled with Low Volatile Honge Oil and Its Methyl Ester (HOME).” Proceedings of the Institution of Mechanical Engineers, Part A: Journal of Power and Energy222 (3): 323–330.
- Banapurmath, N. R., P. G.Tewari, and R. S.Hosmath. 2008. “Experimental Investigation of Four Stroke Direct Injection Diesel Engine Operated on Dual Fuel Mode with Producer Gas as a Inducted Fuel and Honge Oil and Honge Oil Methyl Ester as Injected Fuels.” Renewable Energy33 (9): 2007–2018.
- Banapurmath, N. R., P. G.Tewari, and V. VinodKumar. 2009. “Combustion and Emission Characteristics of a Direct Injection CI Engine When Operated on Marotti Oil Methyl Ester and Blends of Marotti Oil Methyl Ester and Diesel.” Sustainable Engineering2 (3): 192–200.
- Banapurmath, N. R., R. S.Hosmath, V. S.Yaliwal, and P. G.Tewari. 2011. “Improving Fuel Efficiency of Compression Ignition Engines Fueled with Vegetable Oil.” In Fuel Efficiency, edited by J. K.Bernard, 67–100. New York: NOVA Science Publishers Inc.
- Banapurmath, N. R., P. G.Tewari, V. S.Yaliwal, S.Kambalimath, and Y. H.Basavarajappa. 2009. “Combustion Characteristics of a 4-Stroke CI Engine Operated on Honge Oil, Neem and Rice Bran Oils When Directly Injected and Dual Fuelled with Producer Gas Induction.” Renewable Energy34: 1877–1884.
- Banapurmath, N. R., V. S.Yaliwal, S.Kambalimath, A. M.Hunashyal, and P. G.Tewari. 2011. “Effect of Wood Type and Carburetor on the Performance of Producer Gas-Biodiesel Operated Dual Fuel Engines.” Waste and Biomass Valorization2 (4): 403–413. doi:10.1007/s12649-011-9083-5.
- Dasappa, S., and H. V.Sridhar. 2011. “Performance of a Diesel Engine in a Dual Fuel Mode Using Producer Gas for Electricity Power Generation.” Sustainable Energy1: 1–10. 10.1080/14786451.2011.605945.
- Dien, B. S., X. L.Li, L. B.Iten, D. B.Jordan, N. N.Nichols, P. J.O'Bryan, and M. A.Cotta. 2006. “Enzymatic saccharification of Hot-Water Pretreated Corn Fiber for Production of Monosaccharides.” Enzyme and Microbial Technology39 (5): 1137–1144.
- Ekrem, B., E.Tahsin, and C.Muhammet. 2006. “Effects of Thermal Barrier Coating on Gas Emissions and Performance of a LHR Engine with Different Injection Timings and Valve Adjustments.” Energy Conversion Management47: 1298–1310. http://www.saltmarch.com/pressRelease2.html.
- Jaichandar, S., and P. Tamilporai. 2003. “Low Heat Rejection Engines – An Overview.” Society of Automotive Engineers, Paper No. 2003-01-0405.
- Kamo, R., N. S.Mavinahally, L.Kamo, W.Bryzik, and E. E.Schwartz. 1999. “Injection Characteristics that Improve Performance of Ceramic Coated Diesel Engines.” Society of Automotive Engineers, Paper 1999-01-0972. https://doi.org/doi:10.4271/1999-01-0972.
- Karim, G. A., W. Jones, and R. R. Raine. 1989. “An Examination of the Ignition Delay Period in Dual Fuel Engines.” Society of Automotive Engineers, Paper No. 892140.
- Liang, Xuezheng, ShanGao, JiangaoYang, and MingyuanHe. 2009. “Highly Efficient Procedure for the Transesterification of Vegetable Oil.” Renewable Energy34 (10): 2215–2217.
- Likos, B., T. J. Callahan, and C. A. Moses. 1982. “Performance and Emissions of Ethanol and Ethanol–Diesel Blends in Direct-Injected and Pre-Chamber Diesel Engines.” Society of Automotive Engineers, Paper No.: 821039.
- Miller, Robert A.1987. “Current Status of Thermal Barrier Coatings – An Overview.” Surface and Coatings Technology30: 1–11.
- Palash, S. M., H. H.Masjuki, M. A.Kalam, B. M.Masum, A.Sanjid, and M. J.Abedin. 2013. “State of the Art of NOx Mitigation Technologies and Their Effect on the Performance and Emission Characteristics of Biodiesel-Fueled Compression Ignition Engines.” Energy Conversion and Management76: 400–420.
- Papagiannakis, R. G., and D. T.Hountalas. 2003. “Experimental Investigation Concerning the Effect of Natural Gas Percentage on Performance and Emissions of a DI Dual Fuel Diesel Engine.” Applied Thermal Engineering23 (3): 353–365.
- Parikh, P. P., A. G.Bhave, D. V.Kapse, and Shashikantha. 1989. “Study of Thermal and Emission Performance of Small Gasifier-Dual-Fuel Engine Systems.” Biomass19: 75–97.
- Park, S. H., S. H.Yoon, H. K.Suh, and C. S.Lee. 2008. “Effect of the Temperature Variation on Properties of Biodiesel and Biodiesel–Ethanol Blends Fuels.” Oil and Gas Science and Technology – Review, IFP63 (6): 737–745.
- Pérez, J. A., I.Ballesteros, M.Ballesteros, F.Saez, M. J.Negro, and P.Manzanares. 2008. “Optimizing Liquid Hot Water Pretreatment Conditions to Enhance Sugar Recovery from Wheat Straw for Fuel–Ethanol Production.” Fuel87: 3640–3647.
- Rakopoulos, C. D., and E. G.Giakoumis. 2006. “Second-Law Analyses Applied to Internal Combustion Engines Operation.” Progress in Energy and Combustion Science32 (1): 2–47.
- Ramadas, A. S., S.Jayaraj, and C.Muralidharan. 2006. “Power Generation Using Coir Pith and Wood Derived Producer Gas in a Diesel Engine.” Fuel Processing Technology87: 849–853.
- Roy, Murari Mohon, EijiTomita, NobuyukiKawahara, YujiHarada, and AtsushiSakane. 2009. “Performance and Emission Comparison of a Supercharged Dual-Fuel Engine Fueled by Producer Gases with Varying Hydrogen Content.” Hydrogen Energy34: 7811–7822.
- Sahoo, B. B., and U. K.Saha. 2011. “Theoretical Performance Limits of a Syngase Diesel Fueled Compression Ignition Engine from Second Law Analysis.” Energy36 (2): 760–769.
- Sahoo, B. B., N.Sahoo, and U. K.Saha. 2009. “Effect of Engine Parameters and Type of Gaseous Fuel on the Performance of Dual-Fuel Gas Diesel Engines – A Critical Review.” Renewable and Sustainable Energy Reviews13: 1151–1184.
- Singh, R. N., S. P.Singh, and B. S.Pathak. 2007. “Investigations on Operation of CI Engine Using Producer Gas and Rice Bran Oil in Mixed Fuel Mode.” Renewable Energy32: 1565–1580.
- Vivek, and A. K.Guptha. 2004. “Biodiesel from Karanja Oil.” Journal of Scientific and Industrial Research63: 39–47.
- Wategave, S. P., M. S. Sawant, M. S. Tandale, G. Suresh, V. S. Yaliwal, N. R. Banapurmath, and P. G. Tewari. 2013. “Effect of Injection Timing, Injector Opening Pressure and Nozzle Geometry on the Performance of a Compression Ignition Engine Operated on Non-Edible Oil Methyl Esters from Different Sources.” Sustainable Engineering. 10.1080/19397038.2013.777134.
- Xue, Jinlin, GriftT.E., and HansenA.C.. 2011. “Effect of Biodiesel on Engine Performances and Emissions.” Renewable and Sustainable Energy Reviews15 (2): 1098–1116.
- Yaliwal, V. S., S. R.Daboji, N. R.Banapurmath, and P. G.Tewari. 2010. “Production and Utilization of Renewable Liquid Fuel in a Single Cylinder Four Stroke Direct Injection Compression Ignition Engine.” International Journal of Engineering Science and Technology2 (10): 5938–5948.
- Yaliwal, V. S., K. M. Nataraj, N. R. Banapurmath, and P. G. Tewari. 2013. “Honge Oil Methyl Ester and Producer Gas-Fuelled Dual-Fuel Engine Operated with Varying Compression Ratios.” Sustainable Engineering. 10.1080/19397038.2013.837108.