Abstract
During recent years, the issue of gas flaring has become an increasingly relevant problem for the international community. The claim to more sustainable development offers a renewed prospective in the observation of gas flaring and emphasizes that recovering the associated gas could induce economic, environmental and social benefit. The true core of the issue, as is widely accepted, is not a lack of appropriate technologies, but in the number of criteria involved, which makes it difficult to identify a list of alternatives and connecting all the involved stakeholders in the negotiation process. The complexity of this problem can be mitigated using a decision-making method. The objective of the present work is to identify and formulate a supportive decision system based on the Analytic Hierarchy Process. Application of the model to a real case underlines the strengths and weaknesses of the model, leading to the conclusion that a selection of the most sustainable alternatives needs to be based on a number of criteria that need to be recognized and agreed amongst the stakeholders.
1. Introduction: the practice of gas flaring
According to a report by the National Oceanic and Atmospheric Administration (NOAA) (Elvidge et al. Citation2009), gas flaring remains widely used. Indeed, gas flaring represents a better alternative to gas venting. Methane has a global warming potential equal to 25 on a mass basis whereas the global warming potential of CO2 is equal to 1 (Eggleston et al. Citation2006; IPCC Citation2007); therefore, burning the gas decreases its environmental impact. In this framework, gas flaring is still considered due to many constraints that limit the possibility of using the gas differently. According to Petrosyan (Citation2005) and to the Global Gas Flaring Reduction initiative (GGFR Citation2004b), there are three main classes of constraints on associated gas utilization:
market constraints: the absence of a local market, uncompetitive downstream energy markets and prohibitive connection costs between production and consumption areas;
company constraints: lack of experience in dealing with associated gas, poor information about innovative technologies and the fact that flaring reduction projects are not always remunerative;
technical constraints: low and unstable or multiphase gas flows and excessive contents of sulphur and/or liquids.
It is evident that these constraints do not refer only to a purely economic dimension. This fact makes gas flaring a complex, multi-criteria problem. For this reason, economic considerations should be blended with environmental and social evaluations to meet the three core dimensions of sustainability.
Another relevant characteristic of the gas flaring issue is the existence of several stakeholders, each with their own perspective on the problem; this makes it very difficult to reach a shared final solution.
2. The relevance of gas flaring
Gas flaring practice is a massive waste of energy; NOAA has estimated that approximately 134 billion m3 of associated gas were burned in 2010. In term of energy equivalent, this represents approximately 1% of the total global primary energy supply (IEA Citation2009b). The practice occurs in many countries, but 20 are most relevant. Figure shows the value for each country.
In some countries, such as Angola and Nigeria, the lack of relevant legislation or the low institutional capacity does not sufficiently limit the diffusion of the practice (GGFR Citation2004a; PFC Energy Citation2007). In these countries, the amount of gas flared may be compared with the national natural gas production. If this gas were hypothetically recovered and converted into electricity, its share over the whole electric energy supply for the country could be evaluated (IEA Citation2009a). To make the evaluation even more appropriate, it is reasonable to adopt simple turbogas (250 MW, efficiency 0.35) or combined cycle turbogas (800 MW, efficiency 0.56) as state of the art conversion technology, depending on the technological level of the country. In Figure , the absolute and relative values of the potential electric production for each country are shown (heating value about 38 MJ/m3 and load factor 85%).
2.1 Environmental, economic and social considerations
The problem of gas flaring is analysed based on the three main dimensions of sustainability: environmental, economic and social (UNGA Citation1987, Citation2005).
Gas flaring emits CO2 (280 Mton in 2010) and pollutants, mainly SOx, NOx, CO and unburned hydrocarbons (Table ). These pollutants cause acid rain, which damages ecosystems and agriculture (Dung, Leonard, and Tano Citation2008; Efe Citation2010; Nwaugo, Onyeagba, and Nwahcukwu Citation2006).
Table 1 Emissions by flaring per year (reference year, 2010).
Moreover, from an economic point of view, gas flaring is a direct waste of valuable resources that could be used to improve the economy. In Table , this waste is expressed as a share of GDP in each country (International Monetary Fund Citation2011). As an indirect effect, each country could increase its income due to taxation and royalties associated with the commercial use of associated gas (GAO Citation2010).
Table 2 Economic data.
Finally, social implications also exist; pollutants affect the health of populations living near the stacks, causing cancer, respiratory illness and other diseases (Argo Citation2002; Marcus, Godwin, and Leonard Citation2010). Furthermore, a quantitative analysis of the social implication of flaring gas may be conducted by comparing the eventual income from reduced gas flaring with the size of Official Development Assistance (ODA) received by a country (OECD Citation2009). Table illustrates that this ratio is often greater than 1, meaning that if gas flaring were reduced, the income generated by selling recovered gas would be more significant than the international aid received by these countries.
2.2 Access to energy
Recovering associated gas represents a way to improve access to energy and therefore living conditions in oil-producing developing countries. This is particularly true in reference to the vision of the Advisory Group of the Secretary General of the United Nations on Energy and Climate Change (AGECC Citation2010): ‘access to clean, reliable and affordable energy services for cooking and heating, lighting, communications and productive uses’.
Improving access to energy is a preliminary step to promoting development because energy is necessary to provide healthcare, education, access to drinking water and IT. According to a World Bank study (World Bank Citation2009), underperforming energy systems decreases potential annual growth by up to 1–2% of GDP.
The distribution of gas or synthetic fuels obtained from associated petroleum gas in villages could also constitute a business opportunity in itself. LPG programmes in Brazil and Senegal demonstrate that rural distribution challenges can be overcome, while at the same time creating local jobs and providing livelihoods (Lucon, Coelho, and Goldemberg Citation2004; United Nations Foundation Citation2008).
2.3 The issue of multiple criteria and stakeholders
To consider all the elements when attempting to reduce the gas flaring practice, it is necessary to analyse multiple stakeholders and criteria.
As per stakeholders, gas producers are often interested in reducing gas flaring only when environmental constraints limit their action because otherwise, the economic return associated with these projects is low or even negative.
Local governments have a strong impact on the diffusion of gas flaring or venting and should define clear rules about the use of associated gas. In many cases, standards are not specified and therefore, producers are not encouraged to develop gas recovery projects. Furthermore, governments should coordinate the various stakeholders to expand the opportunities for gas utilization; frequently, gas producers, customers and other stakeholders do not operate together and therefore are unable to take advantage of some opportunities. Finally, governments can foster the development of dedicated markets for products derived from associated gas conversion.
Gas infrastructure owners should evaluate new investments to extend gas transfer capacity and maximize the use of existing infrastructure, prioritize the purchase of associated gas and transfer third-party gas while collecting reasonable tariffs that consider the final selling price.
International organizations can play a strong role in encouraging local governments: they can provide governments with common guidance to assist countries to meet their objectives regarding flaring reduction and to develop potential associated gas markets.
Regarding criteria, a complete evaluation of the problem and of possible solutions begins with the concept of sustainability, as mentioned in paragraph 1. It is clear that a number of specific criteria should be used within each dimension of sustainability (economic, environmental and social), when the goal is to analyse a problem in this terms.
For instance, it may be important to emphasize that energy access is improved by recovering and using associated gas because it combines positive features such as reducing local and global pollution and land degradation due to the use of non-commercial biomass, improving the health of the population and providing better living conditions for women and children, who usually collect the wood for households needs (Aureli and Brelet Citation2004; Ishisone Citation2004).
The most important international initiative that addresses this problem is closely linked to the concept of sustainability: the Global Gas Flaring Reduction initiative, which is promoted by the World Bank, is a public–private partnership based on a voluntary asset that was launched at the World Summit on Sustainable Development in Johannesburg in 2002, having as its final goal the reduction of gas flaring to preserve the environment and save energy resources (GGFR Citation2012).
3. Decision method
As previously underlined, the problem of reducing gas flaring involves multiple criteria and stakeholders, and alternatives must be chosen and designed to meet these requirements. The technologies that can be used to recover associated gas and increase its value are well known: on-site re-injection, pipeline transportation to industrial or domestic customers, conversion to Liquefied Petroleum Gas (LPG) and Liquefied Natural Gas (LNG), Power generation (gas to power), Gas To Liquid (GTL) processes and methanol production (Devold Citation2009; Global Methane Initiative Citation2010; Kidnay and Parrish Citation2006). However, the choice amongst these alternatives is often not simple and needs to include various players. Therefore, it is very important to involve all stakeholders in defining a common strategy for increasing the acceptability of the final solution. In fact, the objective of this work is to provide a tool that can be used when a decision is required among various alternatives that have been identified in a pre-feasibility project phase. Gas flaring has various consequences, and a multi-criteria approach is used to consider them. Several multi-criteria methods are available (Department for Communities and Local Government Citation2009; Figueira, Greco, and Ehrgott Citation2005), among which the analytic hierarchy process (AHP) (Harker and Vargas Citation1987; Saaty Citation1994) was chosen in this study.
3.1 Analytic hierarchy process
AHP is based on several axioms, which were first stated by Saaty (Citation2000). First, n alternatives Alt1, Alt2, …Altn must exist. The decision-makers must compare the alternatives on the basis of any criterion and state the strength of the performance of these alternatives based on pairwise comparison. As a consequence, absolute judgement regarding the performance of the alternatives is not required.
In addition, the decision problem is assumed to be formulated as a hierarchy (Figure ). Finally, all criteria and alternatives that affect the decision should be included. The AHP decision model comprises several phases, which are explained in the following paragraphs.
3.1.1 Hierarchical deconstruction of the problem
After identifying the goal of the problem, the selection criteria and the alternatives, it is necessary to combine these elements into a hierarchy. The goal, i.e. the objective of the decision-makers is located at the top level. Another feature of the hierarchy is the criteria level: each criterion is an element to be considered during the choice of the appropriate alternative. Each criterion can be deconstructed into sub-criteria (second level criteria) and so on. A set of indicators must be used to evaluate the alternatives within each criterion. Each criterion might need one or more indicators, and these must be carefully chosen.
3.1.2 Rating alternatives based on pairwise comparisons
Ratings of each element of the hierarchy (termed priority vectors) are obtained based on several comparisons using a pairwise comparison matrix A (n × n).
Each element aij represents the comparison of element i with element j obtained using the scale of values introduced by Saaty (Citation2008).
Matrix A should be positive, reciprocal and consistent; i.e. the following conditions must be satisfied:
Small errors in judgement can affect the consistency of the matrix. However, AHP deals formally with judgement error as defined by Saaty (Citation1977) and Harker (Citation1987).
Saaty proposed an eigenvector approach for estimating the weights when judgement error exists. His approach is described by the following matrix:
The eigenvector obtained by solving the previous equation represents the priorities assigned to each compared element. For this reason, this eigenvector is termed the priority vector.
3.1.3 Hierarchy construction
Finally, one normalized priority vector is obtained for each matrix of the hierarchy.
In particular, the following exist:
a vector that expresses the priority of the first-level criteria within the goal;
several vectors that express the priority of the any-level criteria within their upper-level criteria;
several vectors that express the priority of the alternatives within the bottom-level criteria.
The priorities of each alternative within multiple criteria are obtained by multiplying each priority of an alternative by the priority of its corresponding criterion and adding over all the criteria to obtain the overall priority of that alternative. Hence, a new priority vector is obtained at the end of this process. This vector can itself be combined with other vectors to obtain another priority vector referring to the next higher level of the hierarchy, and so on.
3.1.4 Consistency test
According to Saaty's method, the obtained results can be considered mathematically reliable only if they satisfy some constraints (Masuda Citation1990; Saaty Citation2003).
A consistency test ensures that all data used in the pairwise comparison matrices are coherent. The eigenvector method yields a natural measure for inconsistency: λmax is always greater than n for positive reciprocal matrices and equal to n for consistent matrices. To measure the deviation of consistency, a consistency index (CI) is defined:
The Consistency Ratio (CR) compares CI and the Random Index (RI), which is the average CI of a set of judgements for random reciprocal matrices:
In addition to the consistency test, it is also suggested to conduct a sensitivity analysis to test the effect of the uncertainty introduced during the process.
The strengths and weaknesses of AHP as applied to a gas flaring reduction problem
The AHP method has advantages regarding the gas flaring reduction problem.
First, AHP deconstructs the problem hierarchically, thereby allowing a complex issue to be analysed as a number of simpler and more specific sub-problems.
Second, the analysis considers all dimensions of sustainability, leading to the use of several different indicators. In addition, the decision can involve a number of alternatives.
Third, scarcity of quantitative data in pre-feasibility phases is a critical issue for complete sustainability analysis because the costs and efforts associated with a quantitative, more detailed study are usually prohibitive. A good compromise can be based on the use of full qualitative comparisons, which are allowed by a method based on the Saaty's scale.
When AHP is evaluated by a single stakeholder, the method allows evaluation of the effect of the multi-criteria hierarchy on the final choice compared to a traditional choice based on a single criterion (i.e. a cost evaluation).
Moreover, when AHP is evaluated by all of the main stakeholders, the method permits each evaluation to be combined to reach a compromise solution that has the strong potential to become, through a number of participative iterations, a shared solution (Simons and Wiegel Citation2009).
It is also possible to evaluate the impact of the main weaknesses of AHP in regard to the context of gas flaring reduction.
One problem is related to the number of comparisons to be made, which arises more than proportionally to the number of alternatives (Carmone, Kara, and Zanakis Citation1997). This appears not to be a limiting factor because the time required for the pairwise comparisons is far shorter the time consuming process of involving all of the many stakeholders in the evaluation process.
Lastly, we discuss the main limitation of the AHP method: some conditions can lead to an inversion in the ranking of the alternatives (rank reversal). This phenomenon mainly occurs when very similar alternatives are compared, and its frequency increases with the number of considered alternatives (Belton and Gear Citation1983; Triantaphyllou and Mann Citation1994). However, in the specific context of a pre-feasibility phase, very similar alternatives cannot be considered because the uncertainty linked to the necessary hypotheses and estimations leads us to consider very similar alternatives as the same alternative. In addition to this, market and technical constraints generally strongly limit the number of alternatives. Therefore, rank reversal is unlikely to occur in the application under study.
4. A sound hierarchy for gas flaring
The first step of the AHP procedure is to define the hierarchy of the problem (Figure ).
At the top-level of the hierarchy, we find the goal: a reduction in gas flaring. As previously explained, the problem is analysed considering the three dimensions of sustainability as fundamental criteria, which establish the second level of the hierarchy. Each of these criteria is then evaluated on the basis of several specific sub-criteria, which together form the third level of the hierarchy. Some sub-criteria are then further identified (such as air pollution). Indicators are finally used to measure sub-criteria and always represent the last level of the hierarchy.
It is assumed that eligible alternatives need to be proposed by the involved company on the basis of a techno-economical pre-feasibility study. This leads us to consider the value of the investment as a general sub-criterion for the economic dimension and, thus, to consider some standard indicators such as the internal rate of return (IRR), PayBack Time (PBT) or net present value (NPV) as measures of this criterion.
More specific and selected sub-criteria are indeed given for the other two main dimensions. The choice made by the authors is based on guidance provided by a similar exercise conducted by the IAEA and United Nations regarding indicators for sustainable development (IAEA Citation2005; United Nations Citation2007).
Therefore, the Environmental sub-criteria attempt to consider the effects of the alternatives on the soil, water, air and ecosystems. Social sub-criteria attempt to link the alternatives mainly with health, local employment and access to different services (electricity, heat and fuels).
The identified indicators for the Environmental and Social dimensions are similar to or coincide with those indicated by IAEA and in projects related to the Clean Development Mechanism (Brent, Heuberger, and Manzini Citation2005).
Consulting international standards (ISO 17776:Citation2000; ISO 14004:Citation2004) helped to identify the main sources of environmental impact to be taken into account on a case-by-case basis.
The hierarchy has been structured to describe the more general issue of reducing gas flaring. When applied to a specific case, some indicators may be neglected because they might not be relevant. The full set of indicators is listed in Figure , and a detailed explanation is provided in the following paragraphs.
4.1 Identification of indicators for criterion 1: economic criterion
4.1.1 Eco1 and Eco2: IRR and NPV (quantitative)
All other parameters being equal, using IRR and NPV often results in the same findings. However, using IRR as an economic indicator is not as effective as using NPV in several situations. The major limitation of IRR is also its greatest strength: it uses a single discount rate to evaluate each investment. In most cases, IRR and NPV lead to the same results, IRR is preferred to NPV because it simplifies projects to a single number that the decision-maker can use to determine the best project in terms of profitability. In contrast, the evaluation of NPV is inherently complex and requires assumptions to be made at each stage (e.g. the discount rate, etc.) but yields better results with long-term projects that have multiple cash flows at different discount rates and when cash flows are very uncertain. Moreover, IRR does not provide explicit information regarding the size of the investment (Keheller and Mac Cormack Citation2005; Turvey Citation1963). Therefore, the decision-makers can choose one or more of the three indicators, depending on the context.
4.1.2 Eco 3: PBT (quantitative)
PBT refers to the length of time within which the benefits received from an investment can repay the costs incurred. PBT ignores any benefits that occur after the payback period and, therefore, does not measure profitability; however, it provides useful information in geographical areas that are characterized by high risks due to social or political instability.
4.2 Indicators for criterion 2: environmental criterion
4.2.1 Env1: soil consumption (quantitative)
Soil consumption provides a quantitative evaluation for the sub-criterion ‘soil’, which represents the physical area occupied by plants, i.e. the area occupied by pipeline networks, process units, utilities, buildings and new roads or railways.
4.2.2 Env2: water consumption (quantitative)
This is the indicator for the sub-criterion ‘water’ and is defined as the total quantity of water that is not returned in the same, or quite the same, condition as that in which it was taken. For example, this indicator includes the quantity of water consumed by evaporating towers or converted in chemical processes.
4.2.3 Env3: air pollution (quantitative)
These indicators need to be detailed using a further level of sub-criteria. The model proposes three indicators referring to CO2 equivalent, SOx and NOx.
These indicators are evaluated as follows:
CO2equivalent emissions are evaluated as follows:
where p(i) is the i-th considered pollutant emission and k(i) is the corresponding coefficient of conversion.Footnote3(4.1)
Sulphur-related emissions and NOxemissions are the quantities of pollutants emitted by the processes and plants encompassed by the alternative under evaluation.
When combined in Env3, their relative importance is evaluated by pairwise comparisons.
4.2.4 Env4: noise (qualitative)
This indicator refers to the noise sub-criterion and a qualitative estimation of the noise level that causes deterioration in the environmental conditions, with particular reference to the living conditions of the local population. The indicator values should be estimated considering the increase in noise levels due to new plant operations and the distance between the plant and residential areas.
4.2.5 Env5: impact during construction (qualitative)
This is a qualitative estimate of the temporary impact due to the construction phase. This indicator evaluates the sub-criterion of the short-term impact on the concerned territory. The estimate should consider the amount of soil that is temporarily occupied by the construction site and is then reinstated, the temporary local use of resources and the inconvenience caused to the population. The estimate should consider especially the temporary aspect of the construction, meaning that it has a low weight in the hierarchy. In fact, it is evaluated as a short-term effect, unlike the other indicators.
4.2.6 Env6: impact on the ecosystem (qualitative)
The indicator is a qualitative estimate of the impact of the project on the local ecosystem. This impact increases with the importance of the ecosystems affected by the project and assumes very high values when sensitive areas are affected. Everything else being equal, the impact should generally be proportional to the size of the affected area.
4.3 Indicators for criterion 3: social criterion
4.3.1 Soc1: number of local employees (quantitative)
This quantitative indicator is related to employment and is evaluated as the number of people recruited from local communities who are directly employed in the plant. The number can be estimated by comparison with similar existing facilities.
4.3.2 Soc2: electrical connections increase (quantitative)
The indicator electrical connections increase (ECI) is defined as the estimated number of new electrical connections to houses (i.e. the number of families that get new access to electricity or that record a significant improvement in the quality of the electric service). A simple estimate of the indicator is provided by the following equation:
4.3.3 Soc3: heat connections increase (quantitative)
The indicator heat connections increase (HCI) is defined as the estimated number of living units that receive a new connection to a district heating network. A simple estimate of the indicator is provided by Equation (4.3):
The same considerations as those made for electrical connections apply if none of the alternatives considers the presence of a district heating plant.
4.3.4 Soc4: local distribution of fuel (quantitative)
This indicators needs to be detailed using a further level of sub-criteria; the local distribution of fuel may refer to connection to Natural gas, local GTL/LPG usage or local LNG usage.
Therefore, the model proposes three indicators that can give a measure of the above sub-criteria:
Natural Gas connections increase (quantitative): the number of new local users connected to a natural gas distribution network.
Local GTL/LPG users increase (quantitative): the number of new local users of synthetic fuels derived from associated gas conversion and/or the number of new local users of LPG derived from associated gas fractionation.
Local LNG users increase (quantitative): the number of new local users of LNG obtained from the recovery and treatment of associated gas.
When combined together in Env3, their relative importance is again evaluated by pairwise comparisons.
4.3.5 Soc5: impact on health (qualitative)
This qualitative indicator permits the evaluation of the impact on the health of local populations in accordance with the requirements of the sub-criterion health. Decision-makers should consider the following main factors: the distance between settlements and plants, the type and quantity of emissions and the eventual increase in traffic.
4.3.6 Soc6: other local impacts (qualitative)
This indicator is used in the case of an evident improvement in indirect employment induced by the presence of the new plants (e.g. the creation of cooperatives that distribute liquid fuels produced in the plants).
5. Application: a case study in Russia
The project analysed in the case study aimed at the recovery of associated gas from Western Siberia and was carried out by an Italian Engineering, Procurement and Construction (EPC) Company. The project involved more than 30 fields, with a total flow rate of raw associated gas of more than 2500 million standard cubic metres per year.
NOAA estimated that approximately 35 billion cubic meters were flared in 2010 in Russia. Most of the gas flaring operations are located in the Khanty Mansiysk, Yamalo-Nenetsk and northern Tomskaya regions of Western Siberia. The area is characterized by extreme conditions: this causes serious consequences such as an increase in difficulties associated with cost estimation.
5.1 Definition of alternatives
The identification of the alternatives is the result of a complex screening process based on several considerations and steps.
A preliminary study based on the criterion of technical feasibility led to the consideration of only those alternatives that included the separate sale of natural gas and heavier components.
Therefore, four alternatives are considered for the case study (Table ):
Two possible locations of the gas processing plant (sites A and B);
Table 3 Description of the alternatives.
Two different plant configurations for each location (1 and 2).
In all of the alternatives, the raw gas must be processed. In this case this is achieved using the following facilities:
A collection pipeline network to bring crude oil and associated gas from the wells to a treatment plant and a Gas–Oil Separation Plant that yields gas, oil and water as separate flows. The water is re-injected into the wells, the oil is pumped into the oil pipeline and the gas is compressed into a pipeline and transported to the gas treatment facility.
A gas treatment facility that includes dehydration and mercury removal. Neither nitrogen rejection nor acid gas removal was necessary. Separation of C3+ (LPG) was achieved by compression and expansion, followed by fractionation of C3, C4s and C5+.
Process flow diagrams for each alternative are given in Appendix A.
5.2 Selection and definition of indicators
Considering the specific alternatives and context, some indicators are irrelevant and therefore neglected in the model:
in the Economic dimension, IRR is the only economic indicator used in the pre-feasibility study;
in the Environmental dimension, the Sulphur-related emissions indicator is disabled because of the irrelevant presence of sulphur compounds in the raw gas;
in the Social dimension, ECI and Local distribution of fuels are disabled because there is no production of electricity or fuels for local purposes. Other local impacts is also disabled.
Quantitative data were available to compute some indicators.
Figure shows the priority vectors obtained after the pairwise comparisons that are used to evaluate the alternatives in relation to the last level of the hierarchy.
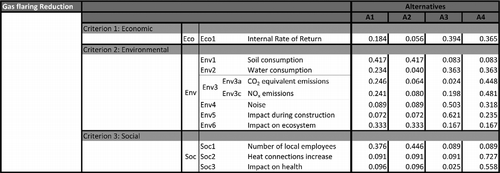
For example, the priority vector for indicator Env2 (water consumption) was computed by numerical estimation of the water plant needs in each scenario. In Table , the results obtained are presented.
Table 4 An example of Priority vector calculation.
5.3 Evaluation of weights
Before combining priority vectors, the weights of all indicators and criteria must be evaluated using pairwise comparisons: for example, in the context of this case study, Air is much more important than Soil.
In this first application of the method, the focus was on the effect obtained by shifting from a traditional cost-benefits evaluation to a new multi-criteria evaluation. Hence, we consider only weights established by a single stakeholder, i.e. engineering company. The obtained weights are shown in Figure .
Combining each indicator with its own weight, priority vectors are obtained for the main criteria of the hierarchy (Figure ).
Finally, all the criteria are considered of equal relevance at this stage, and therefore, the weight is 0.333 for each of them (Economic, Environmental, Social). A global priority vector is obtained by combining the vectors for the criteria with their own weights.
6. Results
The ranking shown in Figure shows that alternative B2 performs the best within the considered criteria: only alternative B1 appears to be slightly better from the economic perspective.
In Figure , the global priority vector is given.
The shares of the overall score among all the criteria and indicators for each alternative are displayed in Figures and .
Based on Figure , it is clear that alternative B2 differs considerably from the others mainly based on its performance within the social dimension. In addition, the economic and environmental performances are very good; however, in these categories, the difference between B2 and B1 is less large. It is also evident that alternatives A1 and A2 are both evaluated as less in each criterion.
Figure allows closer analysis and shows that the very good performance achieved by alternative B2 in the social criterion is mainly due to the impact on health (Soc5) and, to a lesser degree, to the increase in the number of heat connections, (Soc3); however, the effect on the number of employees (Soc1) is negligible. In contrast, the social performance obtained by A1 and A2 is equally due to both Soc1 and Soc5, although obviously the influence of Soc3 is very small.
The differences among the scores of the alternatives regarding the environmental criterion are less pronounced. The most influential indicators are those related to air quality (Env3a, Env3c), the impact of construction (Env5) and on ecosystems (Env6). For alternative B2, the effects of Env3a and Env3c are maximal, whereas for B1, Env5 dominates. Finally, Env6 has the highest weight in alternatives A1 and A2.
The results of the case study clearly show how the ranking obtained by using the proposed methodology is different from one based on a conventional cost-benefits analysis. Indeed this conventional methodology would have lead to select B1 as the best choice.
6.1 Consistency test and sensitivity analysis
First, the consistency of each pairwise comparison matrix has been checked using a consistency test.
Second, sensitivity analysis must be performed to check the stability of the solution. The analysis should test all qualitative elements that depend on the decision-makers' judgements. In this case, a simulation was performed by changing the weights assigned in each qualitative pairwise comparison matrix. An example of weight variation is shown in Figure : the vertical line represents the weight that alternative A2 reaches in relation to the indicator Impact on health, whereas the other straight lines represent the weight of each alternative on the global priority vector; it is possible to see how the global priority vector changes when the effect of Impact on health changes (i.e. when the vertical line is shifted left or right). In this example, no rank reversal occurs while A2 weight is less than 0.4.
The sensitivity analysis led to the conclusion that the stability of the solution is confirmed when a change occurs in the assigned weights in a range up to about ± 15%.
7. Conclusions
The analysis of the data on gas flaring highlights a serious waste of energy and the consequences that this practice might have in terms of lack of sustainability. The causes that led to the spread of this practice are complex; thus, developing a potential solution for proposing alternatives to gas flaring is very complex.
The presence of different players with different interest is a crucial aspect; therefore, it is very important to involve all of the stakeholders during the identification of possible alternatives. This element is discussed and faced also by the international organizations.
In this paper, a decision-making model based on AHP is selected to support the process of selection of the best alternatives. Application of the model to a case study permitted testing the response of the proposed model. It was not possible to involve all stakeholders; therefore, only a single stakeholder evaluation was carried out. Nevertheless, application of the method to the case study gave evidence to the major relevance of a multicriteria hierarchy in selecting alternatives, compared to a choice based on a conventional cost-benefits analysis. As a matter of facts, the choice was changed from a simpler solution (B1, selected with conventional procedure) to a more complex solution that yielded greater efficiency due to the presence of a district heating network (B2). The change in the ranking of the alternatives occurred because considerations based on the three dimensions of sustainability were included. This confirms that the evaluation of alternatives should include several criteria expressed with different weights by different stakeholders.
It is clear that the subjectivity of decision-makers can affect the final result. However, this is not a specific weakness of AHP but is an intrinsic feature of every decision method.
Disclosure statement
No potential conflict of interest was reported by the authors.
Notes
1. Email: [email protected]
2. Email: [email protected]
3. Only methane was a relevant element in the case study in Russia. The coefficient of conversion k(i) is 25 for CH4, according to IPCC (2007).
References
- AGECC (Advisory Group on Energy and Climate Change). 2010. Energy for a Sustainable Future. New York: Advisory Group on Energy and Climate Change.
- Argo, J. 2002. Unhealthy Effects of Upstream Oil and Gas Flaring. Nova Scotia: Save Our Seas and Shores (SOSS).
- Aureli, A., and C. Brelet. 2004. “Women and Water: An Ethical Issue.” Paris: UNESCO International Hydrological Program. Series on Water and Ethics, Essay 4.
- Belton, V., and T. Gear. 1983. “On a Short-Coming of Saaty's Method of Analytic Hierarchies.” Omega 11 (3): 228–230. doi:10.1016/0305-0483(83)90047-6.
- Brent, A. C., R. Heuberger, and D. Manzini. 2005. “Evaluating Projects that Are Potentially Eligible for Clean Development Mechanism (CDM) Funding in the South African Context: A Case Study to Establish Weighting Values for Sustainable Development Criteria.” Environment and Development Economic 10 (5): 631–649. doi:10.1017/S1355770X05002366.
- Carmone, F. J. Jr, A. Kara, and S. H. Zanakis. 1997. “A Monte Carlo Investigation of Incomplete Pairwise Comparison Matrices in AHP.” European Journal of Operational Research 102 (3): 538–553. doi:10.1016/S0377-2217(96)00250-0.
- Department for Communities and Local Government. 2009. Multi-Criteria Analysis: A Manual. London: Communities and Local Government Publications.
- Devold, H. 2009. Oil and Gas Production Handbook. Oslo: ABB Oil and Gas.
- Dung, J. E., S. B. Leonard, and D. A. Tano. 2008. “The Effects of Gas Flaring on Crops in the Niger Delta, Nigeria.” Geo Journal 73: 297–305. doi:10.1007/s10708-008-9207-z.
- Efe, S. I. 2010. “Spatial Variation in Acid and Some Heavy Metal Composition of Rainwater Harvesting in the Oil-Producing Region of Nigeria.” Nature Hazards 55: 307–319. https://doi.org/10.1007/s11069-010-9529-2.
- Eggleston, S., L. Buendia, K. Miwa, T. Ngara, and K. Tanara. 2006. Guidelines for National Greenhouse Gas Inventories. Geneva: International Panel on Climate Change.
- Elvidge, C. D., D. Ziskin, K. E. Baugh, B. T. Tuttle, T. Ghosh, D. W. Pack, E. H. Erwin, and M. Zhizhin. 2009. “A Fifteen Year Record of Global Natural Gas Flaring Derived from Satellite Data.” Energies 2 (3): 595–622. doi:10.3390/en20300595.
- Figueira, J., S. Greco, and M. Ehrgott. 2005. Multiple Criteria Decision Analysis: State of the Art Surveys. Boston, MA: Springer Science & Business Media.
- GAO (Government Accountability Office). 2010. “Opportunities Exist to Capture Vented and Flared Natural Gas, Which Would Increase Royalty Payments and Reduce Greenhouse Gases.” United States Government Accountability Office Reports.
- GGFR (Global Gas Faring Reduction). 2004a. Regulation of Associated Gas Flaring and Venting: A Global Overview and Lessons. Washington, DC: World Bank.
- GGFR (Global Gas Faring Reduction). 2004b. A Voluntary Standard for Global Gas Flaring and Venting Reduction. Washington, DC: World Bank.
- GGFR (Global Gas Faring Reduction). 2012. Global Gas Flaring Reduction Initiative, a Public–Private Partnership. Global Gas Faring Reduction. Accessed 6 December 2012. http://go.worldbank.org/Q7E8SP9J90.
- Global Methane Initiative. 2010. Control Technologies Fact Sheets. Global Methane Initiative. Accessed 13 January 2012. http://www.ontime.methanetomarkets.org/m2mtool/control.aspx.
- Harker, P. T. 1987. “Derivation of Perron Root of a Positive Reciprocal Matrix: With Application to Analytic Hierarchy Process.” Applied Mathematics and Computation 22: 217–232. doi:10.1016/0096-3003(87)90043-9.
- Harker, P. T., and L. G. Vargas. 1987. “The Theory of Ratio Scale Estimation: Saaty's Analytic Hierarchy Process.” Management Science 33 (11): 1383–1403. doi:10.1287/mnsc.33.11.1383.
- IAEA (International Atomic Energy Agency). 2005. Energy Indicators for Sustainable Development: Guidelines and Methodologies. Wien: STI/PUB/1222.
- IEA (International Energy Agency). 2009a. Key World Energy Statistics. Paris: OECD/IEA.
- IEA (International Energy Agency). 2009b. World Energy Outlook 2009. Paris: OECD/IEA.
- International Monetary Fund. 2011. World Economic Outlook Database. New York: International Monetary Fund.
- IPCC (Intergovernmental Panel on Climate Change). 2007. Climate Change 2007: Working Group I: The Physical Science Basis. Intergovernmental Panel on Climate Change. Accessed November 20, 2012. http://www.ipcc.ch/publications_and_data/ar4/wg1/en/ch2s2-10.html.
- Ishisone, M. 2004. Gas Flaring in the Niger Delta: The Potential Benefits of Its Reduction on the Local Economy and Environment. Berkeley, CA: Berkeley University.
- ISO 14004:2004. Environmental Management Systems – General Guidelines on Principles, Systems and Support Techniques.
- ISO 17776:2000. Petroleum and Natural Gas Industries – Offshore Production Installations – Guidelines on Tools and Techniques for Hazard Identification and Risk Assessment.
- Keheller, J. C., and J. J. Mac Cormack. 2005. Internal Rate of Return: A Cautionary Tale Quarterly. Toronto: McKinsey.
- Kidnay, A. J., and W. R. Parrish. 2006. Fundamentals of Natural Gas Processing. New York: CRC.
- Lucon, O., S. T. Coelho, and J. Goldemberg. 2004. “LPG in Brazil: Lessons and Challenges.” Energy for Sustainable Development 8 (3): 82–90. doi:10.1016/S0973-0826(08)60470-6.
- Marcus, O. E., N. N. Godwin, and S. B. Leonard. 2010. “Perceptions and Attitudes Towards Gas Flaring in the Niger Delta Nigeria.” Environmentalist 30: 67–75. doi:10.1007/s10669-009-9244-2.
- Masuda, T. 1990. “Hierarchical Sensitivity Analysis of Priority Used in Analytic Hierarchy Process.” International Journal of Systems Science 21 (2): 415–427. doi:10.1080/00207729008910371.
- Nwaugo, V. O., R. A. Onyeagba, and N. C. Nwahcukwu. 2006. “Effect of Gas Flaring on Soil Microbial Spectrum in Parts of Niger Delta Area of Southern Nigeria.” African Journal of Biotechnology 5 (19): 1824–1826.
- OECD (Organisation for Economic Co-operation and Development). 2009. OECD Statistics.
- Petrosyan, K. 2005. “What Are the Constraints on Associated Gas Utilizations?” Petroleum Economics 8 (19): 1–24.
- PFC Energy. 2007. Using Russia's Associated Gas. Washington, DC: PFC Energy.
- Saaty, T. L. 1977. “A Scaling Method for Priorities in Hierarchical Structures.” Journal of Mathematical Psychology 15 (3): 234–281. doi:10.1016/0022-2496(77)90033-5.
- Saaty, T. L. 1994. “How to Make a Decision: The Analytic Hierarchy Process.” Interfaces 24 (6): 19–43. doi:10.1287/inte.24.6.19.
- Saaty, T. L. 2000. Fundamentals of Decision Making with the Analytic Hierarchy Process. Pittsburgh, PA: RWS.
- Saaty, T. L. 2003. “Decision-Making with the AHP: Why Is the Principal Eigenvector Necessary.” European Journal of Operational Research 145 (1): 85–91. doi:10.1016/S0377-2217(02)00227-8.
- Saaty, T. L. 2008. “Decision Making with the Analytic Hierarchy Process.” International Journal of Services Sciences 1 (1): 83–98.
- Simons, L. P. A., and V. Wiegel. 2009. “Evaluating AHP as Multi-Stakeholder Decision Tool.” Paper presented at the ICE 2009 Conference Proceedings Illinois, February. Leiden, The Netherlands: Centre for Concurrent Enterprise, University of Nottingham.
- Triantaphyllou, E., and S. H. Mann. 1994. “A Computational Evaluation of the Original and Revised Analytic Hierarchy Process.” Computers and Industrial Engineering 26 (3): 609–618. doi:10.1016/0360-8352(94)90054-x.
- Turvey, R. 1963. “Present Value Versus Internal Rate of Return – An Essay in the Theory of the Third Best.” The Economic Journal 73 (289): 93–98.
- UNGA (United Nations General Assembly). 1987. Resolution 42/187: Report of the World Commission on Environment and Development.
- UNGA (United Nations General Assembly), “World Summit Outcome.” Resolution A/60/1, Adopted by the General Assembly on 15 September 2005.
- United Nations. 2007. Indicators of Sustainable Development: Guidelines and Methodologies. New York: UNDESA.
- United Nations Foundation. 2008. Sustainable Bio-Energy Development in UEMOA Member Countries. Ouagadougou, Burkina Faso: The West African Economic and Monetary Union and The Hub for Rural Development in West and Central Africa.
- World Bank. 2009. Making Development Climate Resilient: A World Bank Strategy for Sub-Saharan Africa. Washington, DC: Strategic Framework on Climate Change and Development.
Appendix A
Process flow diagrams for the considered alternatives (A1, A2, B1, B2) are given respectively in Figures .