Abstract
Biosorption efficiency of coir pith, a waste product from coir industry, was investigated in this study for the removal of metallic pollutants such as Ni, Cu and Zn from aqueous solutions. The disposal of coir pith is a major problem associated with the coir industries, especially working in the small-scale sector. The present study explores the effectiveness of utilization of coir pith, an accumulating waste, as a biosorbent for heavy metal removal. Batch mode studies were done to evaluate the efficiency of removal of metals under varying adsorption conditions of pH, metal concentration and contact time. Characterization studies of the biosorbent and SEM analysis were done. Kinetic modelling studies were tried using Lagergren pseudo-first-order and second-order models. Equilibrium studies were done using well-known Freundlich, Langmuir and D–R isotherm models. It was found that all isotherms are fitting well indicating the efficiency of coir pith as an adsorbent of heavy metals. The applicability of all the three isotherms to the sorption processes shows that both monolayer adsorption and heterogeneous energetic distribution of active sites on the surface of the adsorbent are possible. Due to the abundance and low cost of these materials, adsorption technologies developed can act as good sustainable options for the future in heavy metal removal from industrial effluents.
1. Introduction
Environmental pollution by heavy metals is an issue of concern for humanity in this developing world. Nickel (Ni), Copper (Cu) and Zinc (Zn) are metals that are most commonly used in the electroplating industry and hence considerable amounts of these metals find their way into the effluent of these industries. The presence of metals in natural environment can deteriorate these systems due to their mobility, persistence, bioaccumulation and toxic properties (Abas et al. Citation2013; Karnib et al. Citation2014; Mishra Citation2014; Swarnalatha, Letha, and Ayoob Citation2014; Swarnalatha et al. Citation2015); higher concentration of Ni can cause dermatitis and cancer of lungs and bones. Cu can cause neurotoxicity and Zn forms complexes with inorganic and organic ligands and are strongly adsorbed onto soil surfaces (Abas et al. Citation2013). Several methods have already been developed for the removal of heavy metals from wastewater viz., chemical precipitation, reverse osmosis, electrolysis and ion exchange (Hasan, Talat, and Rai Citation2006; Jindal and Samorkhom Citation2005). The limitations of these methods are high costs, difficult process implementation and maintenance. Moreover, these methods are found to be inappropriate or ineffective for effluents containing low concentrations of metals (Bhatnagar and Minocha Citation2006; Mishra Citation2014). Hence, researchers are always interested in the look out of devising an inexpensive and simple system for heavy metal remediation in contaminated wastewaters.
Adsorption is one of the promising methods for heavy metal removal and has been proved to be an excellent method to treat industrial waste effluents offering significant advantages like their low cost, easy availability, profitability, ease of operation and efficiency. Adsorption is a mass transfer process by which a substance is transferred between any two phases, such as liquid–liquid, gas–liquid, gas–solid or liquid–solid interfaces (Alley Citation2000; Weber Citation1972). Several studies have been done by researchers in exploring the effectiveness of different materials as adsorbents. Commercial activated carbon (CAC) is widely used as an adsorbent for removing different types of pollutants from wastewater but is an expensive material and hence adsorption systems based on it are not economically viable (Johnson et al. Citation2009). Biosorption using low-cost adsorbents for removing metallic pollutants is a comparatively new technology (only in the 1990s) and has a high potential for being developed as a cost effective one (Bhatnagar and Minocha Citation2006). In the present study the effectiveness of coir pith, a by-product of coir industries, is explored as an adsorbent. Coir pith is a waste product generated out of coir production in the rural villages and as of now, faces lot of disposal problems. Hence, in this study the effectiveness of using coir pith as an adsorbent in removing heavy metals such as Ni, Cu and Zn from aqueous solutions is investigated.
2. Methodology
Studies for removal of metals such as Ni, Cu and Zn from synthetic metallic solutions are carried out using raw coir pith as adsorbent in batch reactors. Raw pith was collected from a nearby industry. It was cleaned, sundried, grinded and then used for the adsorption studies. Characterization of pith was done to study the parameters such as surface area, lignin content, etc. For studying the micrographic variations in the structure of the adsorbent, scanning electron microscopic (SEM) analysis of coir pith before and after heavy metal removal are taken. SEM analysis can produce very high-resolution images of a sample surface, revealing surface texture details less than 1 nm in size. The adsorption rate is strongly influenced by several parameters such as pH, contact time, initial concentration, adsorbent dosage, adsorbent size, etc. and hence their effects are also studied. These parameters are optimized for getting maximum removal efficiency for the three metals, Ni, Cu and Zn selected in this study. Stock solutions (100 mg/l) of copper, zinc and nickel were prepared separately by dissolving 0.4475 g of Ni SO4.6H2O, 0.3927 g of CuSO4.5H2O and 0.2467 g of ZnSO4.7H2O in 1000-ml distilled water, respectively. Solutions of the metal ions were prepared by suitable dilution of the stock solution with distilled water depending upon the experimental requirements.
Experiments are then conducted under optimized conditions for developing the kinetic and isotherm models for the adsorption study. The pH of solution is adjusted using 0.1 N solution of HCl or NaOH. Equal volumes of all these metal solutions are treated with equal quantities of adsorbent and mixed in a shaker at 100 rpm till equilibrium is reached. After equilibrium, the solution is filtered using a Whatman filter paper No. 40 and the filtrate is analysed for residual metal using an Atomic Absorption spectrophotometer (ICP-AES (Thermo electron IRIS INTREPID II SXP DUO). The detection limits were 0.01 mg/L for all the three metals studied (STIC Citation2014). The percentage removal of heavy metals was then determined as follows.
% removal of metal
where Co and Cf are the initial and final heavy metal concentrations, respectively.
Deionised water was used for the experiments. All adsorption studies were done in duplicate and the mean results are only presented in the results.
3. Characterization of coir pith
The physical and chemical properties of an adsorbent play an important role in determining its performance in terms of its sorption capacity. The results of characterization studies on coir pith are shown in Table .
Table 1. Characteristics of coir pith.
It can be seen that coir pith is having a high percentage composition of lignin (29.6%) and hollocellulose (42.3%) which are responsible for its physical stability causing poor biodegradability. These characteristics are typical for agricultural byproducts and are referred to as lignocellulosic materials (Israel and Eduok Citation2012). Lignin and cellulose are biopolymers bearing multiple phenolic, hydroxyl, carboxyl and amino groups which are reported as responsible for the removal of pollutants from waste water. The low values of bulk density and high values of pore space volume of coir pith are found to be favourable for the process of adsorption.
The SEM image of raw coir pith before the adsorption process is shown in Figure at a magnification of 50 μm.
Figure 1. Scanning electron microscopic (SEM) photograph of raw coir pith at 50 μm magnification before adsorption of heavy metals.
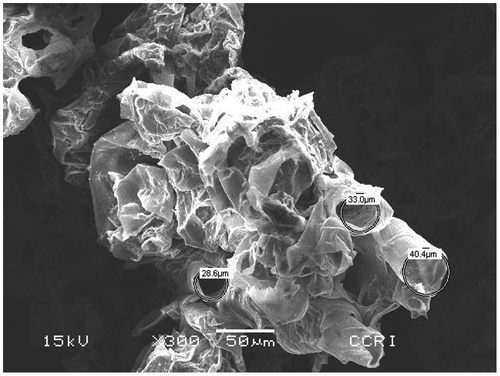
It can be seen that the raw coir pith surface consists of close thin-walled ribbon shape cells and porous pith tissue. The SEM image of cleaned coconut pith depicts a tubular honeycomb-like morphology of the grains. The microscopic size of adsorbent particles and porous structure of pith is evident from the SEM images. The anisotropic tubular grains are arranged in unidirectional mode. The particle surface showing the presence of nanopores and pits demonstrates a rough and a highly porous surface texture that may be effective for high sorptive removal. Figure shows the coir pith at a magnification of 10 μm. The tubular structure of a single strand can be seen clearly here. Figure shows the SEM photograph of coir pith after the adsorption process. After adsorption, the strands are visible in a more regular and straightened pattern than it was before the adsorption process.
4. Effect of pH
pH has a significant effect on the process of adsorption. The effect of pH on the adsorption of Ni, Cu and Zn by raw coir pith is studied by conducting experiments in batch reactors. Metal solutions of same concentrations with varying pH are prepared for each metal studied. The pH was varied from 2–8 and the removal efficiency of metals by coir pith was observed.
The removal of metal ions is found to be at a lesser rate at very low pH values (Figure ). The decrease in adsorption at very low pH (2–4) may be due to the weakly acidic nature of surface functional groups of coir pith. At low pH, acidic surface functional groups tend to be protonated, and hence, do not significantly participate in adsorption reactions. A strong competition effect between the metals and protons for the active sites of the adsorbent can lead to decrease in uptake (Amarasinghe Citation2011; Ramesh et al. Citation2012) and hence the adsorption decreases at very low pH values. It can be seen that the uptake of metal increased with an increase in pH. The maximum removal of metals was observed at a pH of 4 for Ni, 6 for Cu and 5 for Zn, respectively. These pH values are found to be comparable with the studies of previous researchers (Mishra Citation2014). For Ni, the maximum removal (75%) of it from solution is obtained at pH of 4. With increase in pH to 5, the removal is found to be decreasing and then remained a constant. For Cu, the optimum pH is found to be 6, at which a maximum removal of 75% is obtained. The suitable pH for the adsorption of Zn is found to be 5 when a removal efficiency of 76% is observed. With an increase in pH beyond the optimum, removal efficiency slightly decreased and then remains same. At high values of pH, complex formation of metal may occur thus reducing the adsorption efficiency (Amarasinghe Citation2011).
5. Effect of Initial metal ion concentration
The concentration of all metal solutions used for the study was varied from 10 to 50 mg/L for studying the effect of initial metal ion concentration on heavy metal removal. The mixture of adsorbent and solution are mixed well in temperature controlled shaker incubator at 100 rpm and samples are withdrawn at predetermined intervals (0–60 min) and analysed for residual metal concentrations.
It can be seen (Figure ) that the efficiency of removal of metals is maximum for low concentrations of metals and is decreasing with an increase in concentration. At low concentration of metals in solution, they are adsorbed by specific adsorption sites whereas exchange sites are getting filled at higher concentrations leading to a decrease in efficiency (Karnib et al. Citation2014). Thus for Ni, the efficiency decreased from 77 to 43% as the metal ion concentration increased from 10 to 50 mg/L. Similarly for Cu and Zn, the efficiency decreased from 77 to 50% and 80 to 55%, respectively. At low ion concentrations, the ratio of surface active sites to total metal ions in the solution is also high and hence, more metal ions might have interacted with the adsorbent and removed from the solution.
6. Effect of adsorbent dosage
For studying the effect of adsorbent dosage on adsorption of heavy metals, equal volumes of synthetic metallic solutions are treated with varying dosages of the adsorbent. Thus for each metal, adsorbent dosage is varied from 0.5 to 5 g for 150 ml of synthetic solutions. The pH, contact time and the initial metal ion concentration of the solution are kept constant at the optimum values for each metal as obtained from the previous experiments. After equilibrium, the solution is filtered and the filtrate is analysed for determining the residual heavy metals concentration.
It was observed that the efficiency of metal removal gradually increased with an increase in dosage of adsorbent (Table ). It can be seen that the maximum removal of metals is at a dosage of 1 g/150 ml for Ni and Zn whereas for Cu it is observed as 2 g/150 ml. The observed increase in removal efficiency with increase in adsorbent dosage implies that the process is dependent upon the availability of sorptive binding sites. This sorption pattern may be indicating the predominance of surface adsorption in metal removal mechanisms as the available active sites for adsorption are increasing with adsorbent dosage. But further increase in adsorbent dosage is found to be of no use as the availability of higher energy sites are decreasing. The constant values of removal efficiency observed after achieving the maximum may be indicating this effect.
Table 2. Effect of adsorbent dosage on the adsorption of heavy metals.
The competitive uptake of zinc, copper and nickel was also studied for studying the interference of metals ions. The pH was optimized as 5 for all the metals as there were different pH values for all the three metals. The contact time was optimized as 60 min as the adsorption of metals were found to remain almost a constant value after reaching the maximum adsorption. The binding sites on the surface of the coir pith are finite and when a mixture of cations (Cu, Ni and Zn) is present in the same solution they will compete for the binding sites. The amount sorbed would depend on the equilibrium between sorption competitions from all the cations. The results are shown in Table .
Table 3. Competitive adsorption of zinc, copper and nickel onto coir pith.
At low concentration, most of the metal ions were sorbed by the coir pith as there were sufficient sorption sites for all the ions. At higher concentration, the order of sorption was still the same, even though the percentage sorption was greatly reduced. Thus, for observing the competitive adsorption of metal ions, the initial metal ion concentration was optimized as 50 ppm as maximum metal uptake was obtained for all the metals at this concentration.
7. Adsorption kinetics
Kinetic studies on metals show that the uptake is in a biphasic mode with a rapid increase in the initial few minutes followed by a slow phase. The maximum removal occurred in 20 min for Ni, 40 min for Cu and 10 min for Zn. It is generally felt that the initial rapid intake indicates surface bound adsorption and the slow second phase indicates the long-range diffusion of metal ions into the interior pores of the adsorbent. The percentage removal decreased with increase in concentrations of metallic solutions. Thus, the efficiency of Ni decreased from 84.2 to 53.6%, efficiency of Cu decreased from 74 to 44.3% and efficiency of Zn decreased from 90.5 to 62.6% as the metal concentration was increased from 10 to 50 mg/L. The removal rate was found to be only 50–60% for the solutions at a concentration of 50 mg/L for all metals. After the respective optimum contact times, the percentage removal of metals is found to be more or less constant showing equilibrium conditions.
8. Kinetic modelling studies on removal of metals using coir pith
Predicting the rate at which adsorption takes place for a given system is probably the most important factor in adsorption design, as the adsorbate residence time and reactor dimensions are controlled by system’s kinetics. Kinetic models based on the capacity of adsorbents i.e. the Lagergren’s (pseudo-first-order equation) and Ho’s (pseudo-second-order equation) models (Ho Citation2006) were tried out in this study.
Lagergren’s equation is the first kinetic equation for the sorption of liquid/solid system based on solid capacity and is used extensively to describe the sorption kinetic. This model assumes that the rate of change of solute uptake with time is directly proportional to difference in saturation concentration and the amount of solid uptake with time. The Lagergren’s pseudo-first-order rate equation can be expressed as given in Equation (1):(1)
where, qt is the amount of adsorbed metal ion onto the adsorbent in mg/g at time t, qe is equilibrium sorption uptake (mg/g) and k1 is the pseudo-first-order rate constant (min −1).
If the rate of sorption is a second-order mechanism, the pseudo-second-order chemisorptions kinetic rate equation can be expressed as (Ho and McKay Citation1998) shown in Equation (2):(2)
where, k2 is pseudo-second-order rate constant (g/mg min) and h is the initial sorption rate (mg/g/min).
The amount of adsorbed metal ion on the adsorbent in mg/g at time t (q) and the equilibrium sorption uptake in mg/g (qe) for Ni under different concentrations of Ni are shown in Table .
Table 4. Pseudo-first-order kinetic study for the adsorption of nickel at different concentrations (mg/L).
The plots of log(qe − q) vs. time (t) for varying concentrations of Ni from 10 to 50 mg/L are drawn for studying the pseudo-first-order model. Near linear fits are observed in the plots. This reasonable fitting of kinetic data shows the possibilities of a diffusion controlled sorption mechanism (Crini et al. Citation2007). The pseudo-first-order constants k1, are calculated from the slopes of the plots for different concentrations of Ni. The average first-order constant (k1) is observed as 0.127 min−1. The qe estimated by this model differs substantially from those measured experimentally, suggesting that the adsorption is not a first-order reaction. The first-order model has a lower correlation (average correlation of 0.936) indicating that this model is less appropriate for the adsorption of Ni.
Plots based on the second-order model (Figure ) show that a straight line fit is obtained for all different concentrations of Ni. The data are almost perfectly fitting into the model which indicates that the reaction follows a second order. The pseudo-second-order rate constant (k2) and qe values are determined from the plots. The average second-order constant is observed as 0.4198 g mg−1 min−1. The highly significant regression values obtained (average R2 = 0.998) also supports the second-order model.
A comparison of the adsorption rate constants, correlation coefficients, adsorption capacities obtained using the pseudo-first-order model and pseudo-second-order models is shown in Table .
Table 5. Comparison between model parameters of Lagergren pseudo-first-order and pseudo-second-order kinetic models for nickel.
Similar results are observed for metals such as Cu and Zn also. The second-order models showed a highly significant straight line fit with correlation coefficients of 0.989 and 0.999 for Cu and Zn, respectively. This suggests that the adsorption of studied metals by coir pith followed the second-order kinetic model. It is also found from the kinetic studies that the average adsorption constant of Zn (3.269) is higher than that of Cu (1.388) and Ni (2.613). Generally, the lower values of adsorption capacities indicate faster adsorption process (Maliyekkal, Sharma, and Philip Citation2006). This indicates that the adsorption of Zn by coir pith is a rapid process when compared to that of Ni and Cu.
9. Study of equilibrium adsorption isotherms
To optimize the design of an adsorption system for the removal of adsorbate, it is important to establish the most appropriate correlation for the equilibrium data. Three adsorption isotherms of Langmuir, Freundlich and D–R models are employed to fit the equilibrium data. The Langmuir isotherm takes an assumption that the adsorption occurs at specific homogeneous sites within the adsorbent. Freundlich model can be applied to non-ideal sorption onto heterogeneous surfaces as well as multilayer sorption. The Dubinin–Radushkevich (D–R) isotherm is more general than the Langmuir isotherm, because it does not assume a homogeneous surface or constant sorption potential. The empirical Freundlich equation (Equation (3)) based on sorption on a heterogeneous surface is as follows:
(3)
where qe is the amount of metal adsorbed at equilibrium (mg/g), Ce is the equilibrium concentration of metal (mg/L), Kf is the equilibrium constant indicative of adsorption capacity and n is the equilibrium constant indicative of adsorption intensity. For n=1, adsorption is linear; n<1 implies that adsorption process is chemical and if n>1, adsorption is a favourable physical process.
The Langmuir equation has the form as given in Equation (4)(4)
where, qe is the amount of adsorbate adsorbed at equilibrium per unit weight of adsorbent (mg/g), qmax is the saturated monolayer adsorption capacity (mg/g), b is the Langmuir constant related to binding energy or affinity parameter (l/mg) of the sorption system and Ce is the equilibrium solute concentration (mg/L).
The Dubinin–Radushkevich (D–R) isotherm is more general than Langmuir isotherm since it does not assume a homogeneous surface or constant sorption potential. It can be used to distinguish between physical and chemical adsorption of metal ions and has limited utility (Ceyhan and Baybas Citation2001). The Dubinin–Radushkevich has the following form as shown in Equation (5).(5)
where, qm is the Dubinin–Radushkevich monolayer capacity or theoretical saturation capacity (mg/g), β is a constant related to adsorption energy, ε is Polanyi potential which is related to the equilibrium concentration by Equation (6)(6)
where R is the universal gas constant (8.314 J/mol K), T is the absolute temperature (K). The constant β gives the mean free energy, E (kJ/mol), of sorption per molecule of the sorbate when it is transferred to the surface of the solid from infinity in the solution and can be computed using the relationship (Qadeer et al. Citation1995) as given in the Equation (7) below:(7)
For a magnitude of E between 8 and 16 kJ/mol, the adsorption process follows chemical ion exchange, while the value of E below 8 kJ/mol indicates the physical adsorption (Ozcan, Oncu, and Ozcan Citation2006).
Though many other isotherm models have been developed (Kumar and Sivanesan Citation2006; Weber Citation1972), it can be seen that the Langmuir and Freundlich isotherms still remain as the two most commonly used equilibrium adsorption equations, due to their simplicity and the ease with which their adjustable parameters can be estimated.
From the isotherm studies (Table ), it is found that all isotherms are fitting well with the experimental data which indicates the efficiency of coir pith as an adsorbent of heavy metals. The applicability of all the three isotherms to the sorption processes shows that both monolayer adsorption and heterogeneous energetic distribution of active sites on the surface of the adsorbent are possible. Among the three isotherms studied, it is found that Langmuir isotherms with r2 value of 0.999 are best representing the adsorption data for all heavy metals using coir pith. Langmuir equations refer to a monolayer sorption onto a surface containing a finite number of accessible sites. The Freundlich constant n value for the metals Ni, Cu and Zn is observed as 2.67, 3.07 and 2.63, respectively. The n values lying in the range of 1–10 confirm a favourable condition of adsorption (Ramesh et al. Citation2012).
Table 6. Isotherm constants for the adsorption of nickel, copper and zinc onto coir pith.
The Langmuir constant Qo defines the total capacity of the adsorbent as mg/g for metals at 300 K and it is temperature dependent. The constant, b, represents the affinity between the adsorbent and adsorbate. A large value of b implied strong bonding of metals to the coir pith. The values of Kf from the Freundlich model are indicators of the adsorption capacity of a given adsorbent. The exponent n is greater than unity indicating favourable adsorption processes. From the D–R isotherm the magnitude of the mean free energy, E is estimated as < 8 kJ/mol for Ni, Cu and Zn, which shows that the adsorption is related to physical processes. Since physical adsorption is the prevailing phenomena with coir pith, it also indicates possibility of better regeneration of the used material.
10. Conclusion
The present study has demonstrated the adsorption capacity of coir pith in removal of Ni, Cu and Zn from aqueous solutions. Studies show the potential of coir pith as an adsorbent of metallic pollutants from aqueous solutions and are found as effective when heavy metals are in lesser concentrations as well as at higher concentrations. This property can be of high significance in industrial wastewater treatment. The adsorption capacity of coir pith is found to be much lesser when compared to other adsorbents and hence the consumption of adsorbent required may be high. The adsorbent being locally available in abundance, the costs incurred for the process can be considerably reduced. But as the material is cheap as well as available in abundance in the state, this may not be a problem for its selection as an adsorbent.
From the isotherm studies it is found that the adsorption is of physical in nature. This adsorption process is also found to be faster. Due to these properties regeneration of the adsorbent can also be attempted. From an overview of all these properties, it can be concluded that coir pith has the potential for commercialization as it is technically feasible, eco friendly, cheap and having good metal binding capacity. Biosorption by low-cost adsorbents is considered as a comparatively new technology, and is facing several limitations such as lower efficiency, high operational cost, disposal of spent adsorbents, etc. Hence, extensive research in this area is essential for developing this environmental friendly process as a sustainable technology for the future.
Disclosure statement
No potential conflict of interest was reported by the authors.
Funding
This work was supported by the Centre for Engineering Research and Development (CERD) Government of Kerala, India.
Acknowledgement
Authors thank the Sophisticated Test and Instrumentation Centre (STIC), Cochin University of Science and Technology, CUSAT, Kochi for extending laboratory facilities.
References
- Abas, S. N. A., M. S. Ismail, M. L. Kamal, and S. Izhar. 2013. “Adsorption Process of Heavy Metals by Low-cost Adsorbent: A Review.” World Applied Sciences Journal 28 (11): 1518–1530.
- Alley, E. R. 2000. Water Quality Control Handbook. Vol. 8, 125–141. New York: Mc Graw Hill.
- Amarasinghe, B. M. W. P. K. 2011. “Lead and Cadmium Removal from Aqueous Medium Using Coir Pith as Adsorbent: Batch and Fixed Bed Column Studies.” Journal of Tropical Forestry and Environment 1 (1): 36–47.
- Bhatnagar, A., and K. Minocha. 2006. “Conventional and Non-conventional Adsorbents for Removal of Pollutants from Water – A Review.” Indian Journal of Chemical Technology 13: 203–217.
- Ceyhan, O., and D. Baybas. 2001. “Adsorption of Some Textile Dyes by Hexadecyltrimethyl–Ammonium Bentonite.” Turkish Journal of Chemistry 25: 193–200.
- Crini, G., H. N. Peindy, F. Gimbert, and C. Robert. 2007. “Removal of C.I. Basic Green $ (Malachite Green) from Aqueous Solutions by Adsorption Using Cyclodextrin-based Adsorbent: Kinetic and Equilibrium Studies.” Separation and Purification Technology 53: 97–110.10.1016/j.seppur.2006.06.018
- Hasan, S. H., M. Talat, and S. Rai. 2006. “Sorption of Cadmium and Zinc from Aqueous Solutions by Water Hyacinth (Eichhornia crassipes).” Bioresource Technology 98 (4): 918–928.
- Ho, Y. S. 2006. “Review of Second Order Models for Adsorption Systems.” The Journal of Hazardous Materials B136: 681–689.10.1016/j.jhazmat.2005.12.043
- Ho, Y. S., and G. McKay. 1998. “A Comparison of Chemisorptions Kinetic Models Applied to Pollutant Removal on Various Adsorbents.” Transactions of the Institution of Chemical Engineers 76: 332–340.10.1205/095758298529696
- Israel, A. U., and U. M. Eduok. 2012. “Removal of Some Metal Ions from Aqueous Solution Using Coconut Coir Dust and Modified Coconut Coir Dust Extract Resins.” Research Journal of Applied Sciences 7: 481–488.
- Jindal, R., and Samorkhom, N. 2005. “Cadmium Removal from Wastewater in Constructed Wetlands”, Practice Periodical of Hazardous, Toxic, and Radioactive Waste Management 3 (173): 173–178. doi:10.1061/(ASCE)1090-025X(2005)9.
- Johnson, T. A., N. Jain, H. C. Joshi, and S. Prasad. 2009. “Agricultural and Agro-processing Wastes as Low Cost Adsorbents for Metal Removal from Waste Water: A Review.” Journal of Scientific and Industrial Research 67: 647–658.
- Karnib, M., A. Kabbani, H. Holail, and Z. Olama. 2014. “Heavy Metals Removal Using Activated Carbon, Silica and Silica Activated Carbon Composite.” Energy Procedia 50: 113–120.10.1016/j.egypro.2014.06.014
- Kumar, K. V., and S. Sivanesan. 2006. “Pseudo Second Order Kinetics and Pseudo Isotherms for Malachite Green onto Activated Carbon: Comparison of Linear and Non Linear Regression Methods.” Journal of Hazardous Materials 136: 721–726.10.1016/j.jhazmat.2006.01.003
- Maliyekkal, S. M., A. K. Sharma, and L. Philip. 2006. “Manganese Oxide Coated Alumina: A Promising Sorbent for Defluoridation of Water.” Water Research 40: 3497–3506.10.1016/j.watres.2006.08.007
- Mishra, S. P. 2014. “Adsorption–Desorption of Heavy Metal Ions.” Current Science 107 (4): 601–612.
- Ozcan, A., E. M. Oncu, and A. S. Ozcan. 2006. “Kinetics, Isotherm and Thermodynamic Syudies of Adsorption of Acid Blue 193 from Aqueous Solutions onto Natural Sepiolite, Colloids and Surfaces.” Colloids and Surfaces A: Physicochemical and Engineering Aspects 277: 90–97.10.1016/j.colsurfa.2005.11.017
- Qadeer, R., J. Hanif, M. Khan, and M. Saleem. 1995. “Uptake of Uranium Ions by Molecular Sieve.” Radiochemica Acta 68: 197–201.
- Ramesh, S. T., N. Rameshbabu, R. Gandhimathi, P. V. Nidheesh, and M. Srikanth Kumar. 2012. “Kinetics and Equilibrium Studies for the Removal of Heavy Metals in Both Single and Binary Systems Using Hydroxyapatite.” Applied Water Science 2: 187–197.10.1007/s13201-012-0036-3
- STIC. 2014. Sophisticated Test and Instrumentation Centre. www.sticindia.com
- Swarnalatha, K., J. Letha, and S. Ayoob. 2014. “Effect of Seasonal Variations on the Surface Sediment Heavy Metal Enrichment of a Lake System in South India.” Environmental Monitoring and Assessment 186: 4153–4168. doi:10.1007/s10661-014-3687-8.
- Swarnalatha, K., J. Letha, S. Ayoob, and G. N. Ajith. 2015. “Risk Assessment of Heavy Metal Contamination in Sediments of a Tropical Lake.” Environmental Monitoring and Assessment 187: 322.10.1007/s10661-015-4558-7
- Weber, W. J. 1972. Physicochemical Processes for Water Quality Control, A Wiley-Inter Science Publication. New York: Wiley.