Abstract
The generation of power from the reduction of fossil fuels is the biggest challenge for the next half century. The idea of converting solar energy into electrical energy using photovoltaic panels holds its place in the front row compared to other renewable sources. But the continuous change in the relative angle of the sun with reference to the earth reduces the watts delivered by solar panel. In this context solar tracking system is the best alternative to increase the efficiency of the photovoltaic panel. Solar trackers move the payload towards the sun throughout the day. In this paper different types of tracking systems are reviewed and their pros and cons are discussed in detail. The results presented in this review confirm that the azimuth and altitude dual axis tracking system is more efficient compared to other tracking systems. However in cost and flexibility point of view single axis tracking system is more feasible than dual axis tracking system.
1. Introduction
Nowadays the energy deficiency problems faced by the world, more especially the third world countries, are urging researchers to find an alternative energy source that would complement the conventional fossil fuel. The alternative energy sources include solar, nuclear and wind. Solar energy is the energy generated by harnessing the power of the solar radiation. It is the cleanest source of energy which can pollute the climate the least. The power from the sun intercepted by the earth is approximately 1.8 × 1011 MW, which is many thousands of times larger than the present consumption rate on the earth from all other in-use commercial energy sources. The main problem with the solar energy is its dilute nature. Even in the hottest regions on the earth, the solar radiation flux available rarely exceeds 1 kW/m, which is insufficient for technological utilisation. This problem can be rectified by a device solar tracker which ensures maximum intensity of sun rays hitting the surface of the panel from sunrise to sunset.
1.1. Solar geometry and solar angles
The earth’s orbit about the sun is almost circular at an average distance of 149.6 million km. The earth’s axis of rotation is tilted by an angle ε = 23.441° with respect to the normal to the plane of the earth’s orbit (Figure ) (Mitton Citation1977). The plane of the earth’s orbit is named as the plane of the ecliptic. The plane passing through the earth’s equator is inclined perpendicularly to the plane of the ecliptic, at an angle ε (angle of obliquity). Based on conservation of angular momentum, the earth’s axis of rotation points as a fixed direction in space which means for the same location on earth, at a fixed time (for midday as determined by solar time), the altitude of the sun (the angular height above the horizon) will vary throughout the year.
Figure 1. Schematic diagram of earth orbit around sun. Source: Sproul (Citation2007).
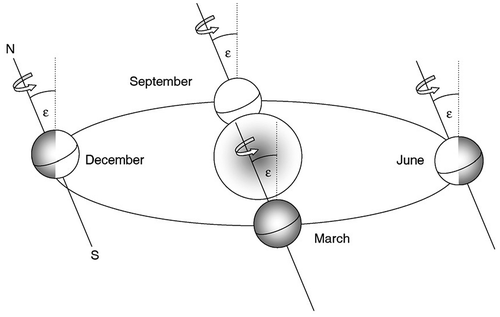
In order to derive the solar angles, need to define suitable reference frames. Three principal reference frames will be used, the ecliptic, the equatorial and the horizon reference frames. These reference frames are centred or referenced to the centre of the earth and the apparent motion of the sun is considered for calculations. The sun and other celestial bodies are assumed to lay on the celestial sphere as shown in Figure with a large radius. The daily rotation of the earth is described by the rotation of the celestial sphere about the polar axis, and the instantaneous position of the sun is given by the hour angle ω, the angle between the meridian passing through the sun and the meridian of the site. The celestial sphere is imagined to rotate about the fixed Earth to depict the daily, apparent motion of the sun and other celestial bodies (Figure ) (Sproul Citation2007).
Figure 2. Celestial sphere geometry of the Sun and Earth. Source: Sproul (Citation2007).
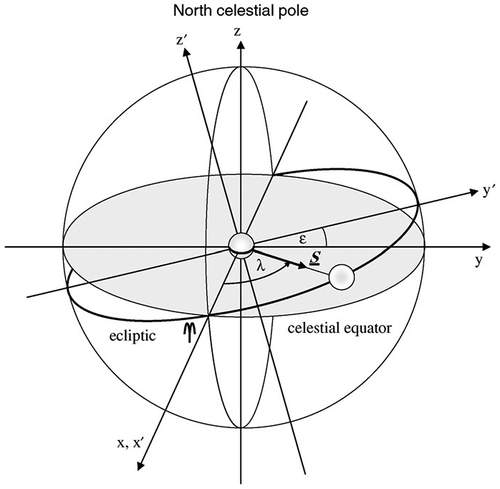
1.2. The nomenclature
1.2.1. Declination angle (δ)
It is the angular distance of the sun’s position in north or south of the earth’s equator (Figure ). The earth’s axis is tilted 23.34° from the plane of the earth’s orbit around the sun and the earth is in its annual path around the sun causes the declination angle to vary from 23.45° north on December 21 (Winter Solstice) to 23.45° south on June 21 (Summer Solstice) ( Li and Lam Citation2007; Reda and Andreas Citation2008; Rockwell Automation Citation2009).
1.2.2. The altitude angle or elevation angle (α)
It shows how high the sun appears in the sky. The angle is measured between an imaginary line between the observer and the sun and the horizontal plane the observer is standing on. The altitude angle is negative when the sun drops below the horizon (Figure ).
1.2.3. Solar azimuth angle (ϒs)
It is the angular distance between south and the projection of the line of sight to the sun on the ground. A positive solar azimuth angle indicates a position east of south, and a negative azimuth angle indicates west of south (Figure ).
1.2.4. The latitude (w)
It is a point or location is the angle made by the radial line joining the location to the centre of the earth with the projection of the line on the equatorial plane. The earth’s axis of rotation intersects the earth’s surface at 90° latitude (North Pole) and −90° latitude (South Pole). Any location on the surface of the earth then can be defined by the intersection of a longitude angle and a latitude angle.
2. Components of solar tracking system
The main mechanism of the solar tracking system consists of the tracking device, tracking algorithm, control unit, positioning system, driving mechanism and sensing devices. The tracking algorithm determines the angles which are used to determine the position of solar tracker. There are two types of algorithms-astronomical algorithms and real-time light intensity algorithms. The astronomical algorithm is a purely mathematical algorithm based on astronomical references. The real-time light intensity algorithm is based on real-time light intensity readings. The control unit performs the tracking algorithm and manages the positioning system and the driving mechanism. The positioning system operates the tracking device to face the sun at the calculated angles. The positioning system can be electrical or hydraulic. The driving mechanism is responsible for moving the tracking device to the position determined by the positioning system. The sensing devices are group of sensors and measurements that measure the ambient conditions, the light intensity in case of real-time light intensity algorithms, and the tilt angle of the tracker by means of an inclinometer or a combination of limit switches and motor encoder counts (Rockwell Automation Citation2009).
3. Solar tracking system vs. fixed panel
The amount of output mainly depends on the cosine angle of incidence which is known as the angle between the sun ray and horizontal surface. The minimum incidence angle gives the maximum power output. In case of fixed panel except noon time the angle is maximum for the movement of the sun. The efficient solar tracker is which can correct this problem. The first solar tracker introduced by Finster in 1962, was completely mechanical. One year later, Saavedra presented a mechanism with an automatic electronic control, which was used to orient an Eppley pyrheliometer (Roth, Georgiev, and Boudinov Citation2005). Anusha, Chandra, and Reddy (Citation2013) compared the fixed photovoltaic (PV) panel and single axis solar tracking based on real-time clock using ARM processor. The experiment was conducted using both fixed and tracking system for 6 days. The results showed that the solar tracking system increased the efficiency around 40% and energy received from the sun is improved from 9.00 am to 6.00 pm Dhanabal et al. (Citation2013) compared the efficiencies of static panels and tracking systems of single axis and dual axis fixed mount. The readings were taken from morning 8 am to evening 6 pm for fixed panel, single axis tracker and dual axis tracker for every one hour. The results showed the efficiency of the single axis tracking system over that of the static panel is calculated to be 32.17% and dual axis tracking system over that of the static panel is calculated to be 81.68%. Tudorache, Oancea, and Kreindler (Citation2012) compared the solar tracking PV panel with a fixed PV panel in terms of electric energy output and efficiency. The proposed device automatically searches the optimum PV panel position with respect to the sun by means of a DC motor controlled by an intelligent drive unit that receives input signals from dedicated light intensity sensors. The solar tracking PV panel produced more energy than fixed one with about 57.55%. Bione, Vilela, and Fraidenraich (Citation2004) compared the pumping systems driven by fixed, tracking and tracking with concentration PVs. The results showed that for a given irradiance, the pumped water flow rate was significantly different from one another. The fixed PV, the PV with tracker and the concentrating-tracking systems pumped 4.9, 7.4 and 12.6 m3/day, respectively. Hon and Kolte (Citation2013) proposed a field programmable gate array (FPGA) sensor based standalone solar tracking system which was composed of fuzzy logic controller implemented on FPGA sensors, PV panel, stepper motor, and input-output interface. Xilinx_ISE software is used for coding of the tracking. The results showed that tracking has maximum efficiency than fixed panels.
4. Different types of solar tracking techniques
4.1. Based on collectors
4.1.1. Flat plate photovoltaic panel (PV)
In flat-panel photovoltaic applications, trackers are used to minimise the angle of incidence between the incoming sunlight and a photovoltaic panel. Masakazu et al. (Citation2003) proposed a comparative study of fixed and tracking system of very large-scale PV systems in the world deserts. The work focused on the potential and simulation of the 100 MW. Life cycle analysis (LCA) was applied for the simulation. The potentials were evaluated from economic viewpoint by LCA method. The results showed that the cost was reduced by applying tracking system. Suri et al. (Citation2012) produced solar electricity from fixed-inclined and sun-tracking crystalline silicon (C-SI) photovoltaic modules in South Africa. The work presented a method for estimating the energy output from fixed-mounted and single-axis tracking flat-plate PV systems. The simulation used the solar radiation and temperature time series representing a historical record of 18 years (1994–2011).The results showed that one axis tracker with vertical axis inclined 30° north typically gains from 15% up to 35% more electricity, compared to fixed mounting at optimum tilt. Anyaka, Ahiabuike, and Mbunwe (Citation2013) studied the improvement of PV systems power output using sun-tracking techniques. The work presented the detailed view of sun tracking systems developed over the past years. The results prove that the applicability of sun tracking system gives a diverse range of high performance solar-based applications (Figure ).
4.1.2. Concentrated photovoltaic
The optics in concentrated photovoltaic (CPV) modules accept the direct component of the incoming light to maximise the energy collected. The tracking functionality in CPV modules is used to orient the optics such that the incoming light is focused to a photovoltaic collector. Kerzmann and Schaefer (Citation2013) studied the flow rate optimisation of a linear concentrating photovoltaic system. The work focused on a two-dimensional linear concentrated photovoltaic (LCPV) combined with an active cooling and waste heat recovery system. The results showed that an optimal cooling fluid flow at a rate of 4 gal/min (2.52 × 10−4 m3/s), would produce and average of 45.9 kWh of electricity and 15.9 kWh of heat energy. Tripanagnostopoulos et al. (Citation2005) proposed the design and performance aspects for low concentration photovoltaic system. The non-uniform distribution of solar radiation on the PV surface reduces the electrical efficiency and can be rectified by combining the PV with low concentration devices. The diffuse reflectors are used instead of specular reflectors because of their low cost. The PV temperature reduction is also a factor so several modes for heat extraction are applied; using water or air cooled hybrid photovoltaic/thermal (PV/T) solar systems. Benecke, Van Dyk, and Vorster (Citation2013a) developed the optical design of low concentrator photovoltaic modules. This work addressed the necessary procedures that need to be considered when designing an optical sub-system of LCPV module. Various design considerations were taken into account to construct a LCPV module that is characterised with respect to optical design and electrical performance. Benecke, Van Dyk, and Vorster (Citation2013b) implemented the design and analysis of a vertical receiver LCPV system. The work presented the design aspects of the optical and electrical subsystem of LCPV with a geometric concentration ratio of 4.6. An electrical estimation is conducted by the use of I–V (current–voltage) characteristics obtained under sun as well as under concentration.
4.1.3. Concentrated solar power
Concentrating solar power or concentrated solar thermal systems use mirrors or lenses to concentrate a large area of sunlight or solar thermal energy onto a small area. Electrical power is produced when the concentrated light is converted into heat, which drives a heat engine (usually a steam turbine) connected to an electrical power generator or powers a thermo chemical reaction. Tariq et al. (Citation2012) studied the effect of design variation on saved energy of concentrating solar power prototype. The work discussed about the methods to improve the efficiency of the concentrated solar thermal plant. Eljai and Chalqi (Citation2013) implemented a modified model for parabolic trough solar receiver using concentrated solar thermal energy. The work concentrated on the original mathematical model that describes the heat exchange between the main components of a thermal solar collector in an integrated solar combined cycle plant. The solar plant was an integrated combined cycle thermo-solar power plant consists of 256 parabolic trough solar collectors and classified in 64 parallel loops and each loop is 618 m long. The use of the solar tracking mechanism was to maintain the incident solar radiation perpendicular to the reflector and to the focal line of the parabola where a receiver tube contains the heat transfer fluid. The different simulation results showed that both the fluid temperature and the metal tube temperature grow until reaching a certain equilibrium value.
4.2. Based on the axis
4.2.1. Single axis tracker
Single axis trackers have one degree of freedom that acts as an axis of rotation. The axis of rotation of single axis trackers is typically aligned along a true north meridian. Rizk and Chaiko (Citation2008) developed solar tracking system with more efficient use of solar panels. This work included the potential system benefits of simple tracking solar system of single axis tracker using a stepper motor and light sensor. This method was increasing power collection efficiency by implementing a device that tracks the sun to keep the panel at a right angle to sun rays. The power gain was increased 30% over a fixed horizontal array.
4.2.1.1. Horizontal single axis tracker
The axis of rotation for horizontal single axis tracker (HSAT) is horizontal with respect to the ground. Backtracking is one of the drawback in computing the disposition of panels (Jacobson, Seaver, and Tang Citation2011). Li, Tang, and Zhang (Citation2012) calculated the optical performance of HSAT solar panels. From the results it was clear that the east-west placed HSAT was worst to boost the energy while the north-south placed HSAT increased the efficiency drastically around 36%.
4.2.1.2. Vertical single axis tracker
The axis of rotation for vertical single axis trackers (VSATs) is vertical with respect to the ground. These trackers rotate from east to west over the course of the day. Lorenzo et al. (Citation2002) designed the tracking of photovoltaic systems with a single vertical axis. The vertical single axis tracking also called as azimuth tracking is mainly used for the energy gain which can be 40% more compared to tilted static panels. This research work deals with the design of VSAT photovoltaic plant in Tudela. The problems of shadowing in E–W direction and also N–S direction and the methods to rectify were explained in detail. The results clearly specified that VSAT boosted the energy gain around 40% per annum.
4.2.1.3. Tilted single axis tracker
The tracker with axes of rotation between horizontal and vertical is named as tilted single axis tracker. Tracker tilt angles are often limited to reduce the wind profile and decrease the elevated end height. As a module tracks, it sweeps a cylinder that is rotationally symmetric around the axis of rotation (Rockwell Automation Citation2009).
4.2.1.4. Polar aligned single axis tracker
This method is scientifically well known as the standard method of mounting a telescope support structure. The tilted single axis is aligned to the polar star. It is therefore called a polar aligned single axis tracker (Li and Lam Citation2007).
4.2.2. Dual axis tracking
Dual axis trackers have two degrees of freedom that act as axes of rotation normal to one another. The axis that is fixed with respect to the ground is a primary axis. The axis that is referenced to the primary axis is secondary axis (Jacobson, Seaver, and Tang Citation2011).
4.2.2.1. Tip-tilt dual axis tracker
A tip-tilt dual axis tracker is so-named because the panel array is mounted on the top of a pole. Normally the east-west movement is driven by rotating the array around the top of the pole. The vertical azimuth axis is fixed so as to allow great flexibility of the payload connection to the ground mounted equipment because there is no twisting of the cabling around the pole. Tip-tilt trackers can make to minimise up-sun shading and therefore maximise the total power being collected (Al Mohamad Citation2004; Alata, Al-Nimr, and Qaroush Citation2005; Al-Naima and Yaghobian Citation1990; Batayneh, Owais, and Nairoukh Citation2013; Mehleri et al. Citation2010).
4.2.2.2. Azimuth–altitude dual axis tracker
An azimuth–altitude dual axis tracker has its primary axis (the azimuth axis) vertical to the ground and the secondary axis (elevation axis) is normal to the primary axis. The operation is similar to tip-tilt systems but differ in the way the array is rotated for daily tracking. Instead of rotating the array around the top of the pole, they use a large ring mounted on the ground with the array mounted on a series of rollers. The main advantage of this arrangement is the weight of the array is distributed over a portion of the ring (Reda and Andreas Citation2008). Liu et al. (Citation2013) discussed about the influence factors analysis of the best orientation relative to the sun for dual-axis sun tracking. In this research work different types of tracking systems were reviewed such as fixed panel, single axis tracking in east-west, single axis tracking in north-south, and dual axis tracking using both tip-tilt and altitude-azimuth tracking. The results showed that the influencing factors are time error, latitude, and azimuth and tilt angle of the photovoltaic, reflectivity and composite transparent coefficient. Arbab, Jazi, and Rezagholizadeh (Citation2009) implemented a computer tracking system of solar dish with two-axis degree freedoms based on picture processing of bar shadow. The design was based on computer image processing of a bar shadow to obtain the optimised picture of solar dish displacements. The system was independent to geographical location of the solar dish and periodical changes like daily or monthly regulations. Song et al. (Citation2013) implemented a high precision dual axis tracking system based on a hybrid strategy designed for concentrated sunlight transmission via fibres. This system was based on a two-stage tracking process, which consists of a coarse adjustment based on the coordinate calculation algorithm and a fine adjustment using a specially designed photosensitive sensor. In this design optical fibres were used for the precision tracking of concentrated sunlight. The advantage of this design is the higher resolution of the sun sensor because of the use of photosensitive arrays in closer arrangement. From the results it is clear that system tracked the sun’s focal spot with a position precision of less than 0.3 mm and the tracking angle precision is 0.1°. Robert et al. (Citation2014) proposed the optimisation of a small scale dual-axis solar tracking system using nanowatt technology. The solar module was placed first in any one direction and the PV array has to search and stop at the highest current gained by the solar cell. The process was continued for every 30 min from 0600 h up to 1800 h. The results were measured in these positions for current, voltage and power. An open loop control is used for controlling the motors. Reis et al. (Citation2010) proposed the modelling of the performance of low concentration photovoltaic systems. A theoretical model was implemented to study the response of voltage-through systems in terms of temperature, power output and energy yield using as inputs. The device was constructed by integrating dual axis system and conventional crystalline Si-module, named as double sun technology. The results concluded that the double sun technology increased the efficiency around 86% compared to fixed panels. Mohammed et al. (Citation2010) designed a parabolic solar cooker with automatic two axes sun tracking system. The solar cooker with automatic two axes eliminated the standing in the sun for hours to get frequent tracking and facing the concentrated solar cooker. The results of the continuous test performed for three days from 8:30 to 16:30 h in the year 2008 and showed that the water temperature inside the cooker’s tube reached 90 °C in typical summer days for the maximum registered ambient temperature was 36 °C. Rhif (Citation2013) implemented a position control review for a photovoltaic system dual axis sun tracker. This work presented a sun tracker without using sun sensors. The sun tracking was performed by changing the solar panel orientation in horizontal and vertical directions by two motors. The control of these motors was controlled by a microcontroller. Sliding mode control was used to solve the nonlinear equations. The simulation results concluded that tracking improves the efficiency around 40% than fixed panel. Okpeki and Otuagoma (Citation2013) designed and constructed a bi-directional solar tracking system. This research work included the design and fabrication of a bi-directional tracker which can rotate in both azimuth and altitude directions. The two influencing factors of power output are intensity and wave length of sun light were addressed in detail. The results concluded that the efficiency is drastically more compared to fixed panel and the total cost of the tracker is very low. Bakos (Citation2013) implemented a two-axis sun tracking system for parabolic trough collector efficiency improvement. Parabolic trough is used with two axis rotation to the sun. The results conclude that parabolic collector is increasing the energy than flat collector. Rodiek, julie, and steve (Citation2010) conducted solar photovoltaic array tilt angle and tracking performance experiment. Advanced software models were developed to calculate the change of path by the sun throughout the year and to predict the optimum angle for the single axis tracker to obtain maximum energy output for the entire year. Two modelling programs were discussed to predict the optimal angle. For the experiment five panels were used in a single axis to track azimuthally at the tilt angles of 20°, 25°, 32° (latitude), 40° and 50°. One more panel was a fixed control panel facing south at latitude tilt. The results were taken for one year and they conclude that a higher tilted panel (50°) will produce more power throughout the year compared to modelling from PV Watts that suggests a 32° tilt.
4.3. Based on driver
4.3.1. Active tracker
4.3.1.1. Microcontroller and electro-optical sensor based solar tracker
Ray et al. (Citation2012) presented two ways of rotating freedom solar tracker by using microcontroller. The work included the design of a two ways rotating freedom solar tracker based on microcontroller. The microcontroller PIC16F72 was used to activate the motors to get two ways rotation. Light detection sensors were used to get the information about sun radiation. The results were compared with conventional solar tracker without microcontroller and also with fixed panels. The difference was almost 37% between fixed panel and tracking system with microcontroller. Tous, Badran, and Al-Mofleh (Citation2012) studied the thermal evaluation of a sun tracking solar cooker using microcontroller. The work contained the implementation of tracking system developed for getting the solar heating using solar cooker. A microcontroller was used for rotating the solar heater with the movement of the sun. Programmable logic control system was used as control system. A comparison between fixed and sun tracked cooker showed that the use of sun tracking increased the heating temperature by 36%. Ghassoul (Citation2013) proposed design of an automatic solar tracking system to maximise energy extraction. This solar tracking system was controlled by a micro chip PIC 18F452 micro controller. The search mechanism PILOT located the position of the sun and the intelligent panel mechanism rotates itself with the PILOT to extract the maximum energy. The main defect in this was the rotation only takes place, if the energy obtained in the new position is higher than that consumed by the panels during the transition. One miniature motor was used to search the best position for maximum energy extraction. The panel’s mechanism rotated to the position automatically when energy extraction is optimal. The system was designed in such a way that panels only follow the sun if that contributes to extra energy extraction and at the same time, the energy consumed by the panel driving motor is less than that extracted. Wang and Lu (Citation2013) proposed the design and implementation of a sun tracker with a dual-axis single motor for an optical sensor-based photovoltaic system. This work proposed a novel design of a dual-axis solar tracking PV system which utilises the feedback control theory along with a four-quadrant light dependent resistor sensor and simple electronic circuits to provide robust system performance. The proposed system used a unique dual-axis AC motor and a stand-alone PV inverter to accomplish solar tracking. Experiment results indicated that the developed system increased the energy gain up to 28.31% for a partly cloudy day.
4.3.1.2. Auxiliary bifacial solar cell based solar tracker
Gupta et al. (Citation2013) explained the design, construction and effectiveness of a hybrid automatic solar tracking system for amorphous and crystalline solar cells. This work included the design of a hybrid solar tracking system implemented by integrating with amorphous and crystalline solar panel, and microcontroller. The experiment consisted of the analysis on the use of two different materials of solar panel like Amorphous and Crystalline in a solar tracking system at stationary, single axis, and dual axis and hybrid axis solar tracker. The comparison showed that the use of the dual-axis tracking system produced 17.87% gain of power output than a single-axis tracking system. The gain of output power with the hybrid tracking system is further more (52%) than a stationary system inclined at 23.5° to the horizontal.
4.3.1.3. Date, time and sensor based
In the date/time and sensor-based tracking systems, electronic devices like microprocessor calculate the sun’s position from basic formulae or algorithms from geographical information and send signals to the electro motor (Figure ).
Edwards (Citation1978) presented the operation of a computer based sun following system for parabolic collectors. The computer continuously varied the speed of each collector actuators at regular intervals throughout the day. The results concluded that for accurate sun following, the system requires a data output from the central controller of only 500 bit/s for 10,000 collectors per day.
4.3.2 Passive tracker
The passive trackers use a boiling point from a compressed fluid which moves from one side to other by the solar heat which creates a gas pressure results the tracker movement (Semma and Imamura Citation1980). Due to the bad quality of precision orientation, it is unsuitable for certain types of photovoltaic collectors. In the passive tracker the photovoltaic panels include a hologram behind stripes of photovoltaic cells so that sunlight reflects (Tous, Badran, and Al-Mofleh (Citation2012)) on the hologram which allows the cell heat from behind, thereby increasing the modules’ efficiency. Moreover, the plant need not require moving while the hologram still reflects sunlight from the needed angle towards the photovoltaic cells. Ganesh et al. (Citation2011) proposed the design and development of a sun tracking mechanism using the direct shape memory alloy (SMA) actuation. The SMA element acts as sensor and actuator position the solar receptor tilted appropriately to face the sun directly at all times during the day. The thermal stimulus required to activate the SMA element is provided by the concentration and direct focusing of the incident sun rays on to the SMA element. The results show the possibility of the design and development of a sun tracking mechanism using SMA that directly uses sunlight without the need for any additional external power source (Tables and ).
Table 1. Comparison table for different tracking systems.
Table 2. Comparison table on cost and payback for different tracking systems.
5. Conclusion
The innovative designs in sun tracking systems have enabled the development of many solar thermal and photovoltaic systems for a diverse variety of applications in recent years compared to the traditional fixed panels. Solar systems which track the changes in the sun’s trajectory over the course of the day collect a far greater amount of solar energy, and therefore generate a significantly higher output power. This paper has presented a review of the major types of sun tracking systems developed over the past 20 years. It has been shown that these sun tracking systems can be broadly classified as single axis and dual axis, depending on their mode of rotation. Further it can be classified as active and passive tracker depending on the actuator. The sub division and their basic principles of each method have been reviewed. Overall, the results presented in this review confirm that the azimuth and altitude dual axis tracking system is more efficient compared to other tracking systems. However in cost and flexibility point of view single axis tracking system is more feasible than dual axis. In future the present paper details will be useful in selecting an accurate and particular tracker with respect to region, available space and estimated cost. The present work may be useful to improve the design characteristics of different types of solar tracking systems to improve performance. The main contribution of solar tracking involves in resulting an efficient, profitable and clean power production in future.
Notes on contributors
Suneetha Racharla, is a PhD research scholar in Department of Mechanical Engineering, St. Peter’s University, Chennai, Tamilnadu, India. Her research focuses on renewable energy. She has published five papers in international/National Journals and conferences.
K. Rajan, ME, Ph.D, is a professor in the Department of Mechanical Engineering, Dr.M.G.R Educational & Research Institute, University, Chennai, Tamilnadu, India. His area of research includes thermal engineering, IC Engines and renewable energies. He has published more than 75 papers in International/National Journals and Conferences.
Disclosure statement
No potential conflict of interest was reported by the authors.
References
- Al Mohamad, Ali. 2004. “Efficiency Improvements of Photo-Voltaic Panels Using a Sun-Tracking System.” Applied Energy 79 (3): 345–354. doi:10.1016/j.apenergy.2003.12.004.
- Alata, Mohanad, M. A. Al-Nimr, and Y. Qaroush. 2005. “Developing a Multipurpose Sun Tracking System Using Fuzzy Control.” Energy Conversion & Management 46 (7): 1229–1245. doi:10.1016/j.enconman.2004.06.013.
- Al-Naima, F. M., and N. A. Yaghobian. 1990. “Design and Construction of a Solar Tracking System.” Solar Wind Technology 7 (5): 611–617. doi:10.1016/0741-983X(90)90072-A.
- Anusha, K., S. Chandra, and Mohan Reddy. 2013. “Design and Development of Real Time Clock Based Efficient Solar Tracking System.” International Journal of Engineering Research and Applications (IJERA) 3 (3): 1219–1223.
- Anyaka, B. O., D. C. Ahiabuike, and M. J. Mbunwe. 2013. “Improvement of PV Systems Power Output Using Sun-Tracking Techniques.” International Journal of Computational Engineering Research 3 (9): 80–98.
- Arbab, H., B. Jazi, and M. Rezagholizadeh. 2009. “A Computer Tracking System of Solar Dish with Two-axis Degree Freedoms Based on Picture Processing of Bar Shadow.” Renewable Energy 34 (2009): 1114–1118. doi:10.1016/j.renene.2008.06.017.
- Bakos, George C. 2013. “Design and Construction of a Two-Axis Sun Tracking System for Parabolic Trough Collector (PTC) Efficiency Improvement.” Solar Energy Materials and Solar Cells 31 (15): 2411–2421. doi:10.1016/j.renene.2005.11.008.
- Batayneh, W., A. Owais, and M. Nairoukh. 2013. “An Intelligent Fuzzy Based Tracking Controller for a Dual-axis Solar PV System.” Automatic in Construction 29 (2013): 100–106. doi:10.1016/j.autcon.2012.09.006.
- Benecke, M. A., E. E. Van Dyk, and F. J. Vorster. 2013a. “Optical Design of Low Concentrator Photovoltaic Modules.” Journal of energy in South Africa 24 (2): 4–9. doi:10.1016/j.renene.2011.06.043.
- Benecke, M. A., E. E. Van Dyk, and F. J. Vorster. 2013b. “The Design and Analysis of a Vertical Receiver LCPV System.” Journal of Energy in South Africa 20 (3): 16–19. doi:10.1177/1077546313488988.
- Bione, J., O. C. Vilela, and N. Fraidenraich. 2004. “Comparison of the Performance of PV Water Pumping Systems Driven by Fixed, Tracking and V-trough Generators.” Solar Energy 76 (2): 703–711. doi:10.1016/j.apenergy.2013.11.001.
- Dhanabal, R., V. Bharathi, R. Ranjitha, A. Ponni, S. Deepthi, and P. Mageshkannan. 2013. “Comparison of Efficiencies of Solar Tracker Systems with Static Panel Single Axis Tracking System and Dual Axis Tracking System with Fixed Mount.” International Journal of Engineering and Technology (IJET) 5 (2): 1925–1933. doi:10.7763/IJET. ISSN: .
- Edwards, B. P. 1978. “Computer Based Sun following System.” Solar Energy 21 (1): 491–496. doi:10.1016/0038-092X(78)90073-7.
- Eljai, M. C., and F. Z. Chalqi. 2013. “A Modified Model for Parabolic Trough Solar Receiver.” American Journal of Engineering Research (AJER) 2: 200–211.
- Ganesh, N. Jeya, S. Maniprakash, L. Chandrasekaran, S.M. Srinivasan, and A. R. Srinivasa. 2011. “Design and Development of a Sun Tracking Mechanism Using the Direct SMA Actuation.” Journal of Mechanical Design 133 (1): 1–14.
- Ghassoul, Mostefa. 2013. “Design of an Automatic Solar Tracking System to Maximize Energy Extraction.” International Journal of Emerging Technology and Advanced Engineering 3 (5): 453–460. .
- Gupta, Bhupendra, Neha Sonkar, Brahman Singh Bhalavi, and J. Pankaj Edla. 2013. “Design, Construction and Effectiveness Analysis of Hybrid Automatic Solar Tracking System for Amorphous and Crystalline Solar Cells.” American Journal of Engineering Research 2 (10): 221–228.
- Hon, P. Snehal, and M. T. Kolte. 2013. “FPGA Based Standalone Solar Tracking System.” International Journal of Scientific and Research Publications 3 (10): 1–5.
- Jacobson, Larry, Alan Seaver, and Jiashen Tang. 2011. “AstroCalc4R: Software to Calculate Solar Zenith Angle; Time at Sunrise, Local Noon, and Sunset; and Photosynthetically Available Radiation Based on Date, Time, and Location.” Northeast Fisheries Science Center Reference Document: 11–14. http://www.nefsc.noaa.gov/nefsc/publications/.
- Kerzmann, Tony, and Laura Schaefer. 2013. “Flow Rate Optimization of a Linear Concentrating Photovoltaic System.” Journal of Solar Energy Engineering 135 (2): 10–14. doi:10.1115/1.4023006.
- Li, H. W. Danny, and Tony N. T. Lam. 2007. “Determining the Optimum Tilt Angle and Orientation for Solar Energy Collection Based on Measured Solar Radiance Data.” International Journal of Photo Energy 2007: 1–10. doi:10.1155/2007/85402.
- Li, Guihua, Runsheng Tang, and Hao Zhang. 2012. “Optical Performance of Horizontal Single Axis Tracked Solar Panels: 2012.” International Conference on Future Energy, Environment and Materials 16 (2012): 1744–1752. doi:10.1016/j.egypro.2012.01.270.
- Liu, Liqun, Han Xiaoqing, Chunxia Liu, and Jing Wang. 2013. “The Influence Factors Analysis of the Best Orientation Relative to the Sun for Dual-Axis Sun Tracking System.” Journal of Vibration and Control 21 (2): 328–330. doi:10.1177/1077546313488988.
- Lorenzo, E., M. Perez, A. Ezpeleta, and J. Acedo. 2002. “Design of Tracking Photovoltaic Systems with a Single Vertical Axis.” Progress in Photovoltaic: Research and Applications 10: 533–543. doi:10.1002/pip.442.
- Masakazu, Ito, Kazuhiko Kato, Keiichi Komoto, Tetsuo Kichimi, Hiroyuki Sugihara, and Kosuke Kurokawa. 2003. “Comparative Study of Fixed and Tracking System of Very Large-Scale PV (VLS-PV) Systems in the World Deserts.” Proceedings of 3rd WCPEC 3O 19: 2113–2116.
- Mehleri, E., P. Zervas, H. Sarimveis, J. Palyvos, and N. Markatos. 2010. “Determining of the Optimal Tilt Angle and Orientation for Solar Photovoltaic Arrays.” Renewable Energy 35 (11): 2468–2475. doi:10.1016/j.renene.2010.03.006.
- Mitton, S. 1977. The Cambridge Encyclopedia of Astronomy. 1st ed. London: Prentice-Hall of Canada
- Mohammed, S. Al-Soud, Essam Abdallah, Ali Akayleh, Salah Abdallah, and Eyad S. Hrayshat. 2010. “A Parabolic Solar Cooker with Automatic Two Axes Sun Tracking System.” Applied Energy 87 (2010): 463–470. doi:10.1016/j.apenergy.2009.08.035.
- Okpeki, U. K., and S. O. Otuagoma. 2013. “Design and Construction of a Bi–Directional Solar Tracking System.” International Journal of Engineering and Science 2 (5): 32–38.
- Ray, Kumar Sobuj, and Md. Abul Bashar, Maruf Ahmad, and Fahad Bin. 2012. “Two Ways of Rotating Freedom Solar Tracker by Using ADC of Microcontroller.” Global Journal of Researches in Engineering 12 (4): 29–34.
- Reda, Ibrahim, and Afshin Andreas. 2008. “Solar Position Algorithm for Solar Radiation Applications.” National Renewable Energy Laboratory NREL/TP-560-34302 40 (3): 1–40.
- Reis, F., M. C. Brito, V. Corregidor, and J. Wemans, and G. Sorasio. 2010. “Modeling the Performance of Low Concentration Photovoltaic Systems.” Solar Energy Materials and Solar Cells 1 (2010): 1–5. doi:10.1016/j.solmat.2010.03.010.
- Rhif, A. Ahmed. 2013. “A Position Control Review for a Photovoltaic System-dual Axis Sun Tracker.” IETE Technical Review 28 (6): 478–485. doi:10.4103/0256-4602.90755.
- Rizk, J., and Y. Chaiko. 2008. “Solar Tracking System: More Efficient Use of Solar Panels.” World Academy of Science, Engineering and Technology 17: 313–315.
- Robert, A. Jay, Del Rosario, C. G. Reggie, and P. Elmer Dadios. 2014. “Optimization of a Small Scale Dual-axis Solar Tracking System Using Nanowatt Technology.” Journal of Automation and Control Engineering 2 (2): 134–137. doi:10.12720/joace.2.2.134-137.
- Rockwell Automation. 2009. “Solar Tracking Application.” A Rockwell Automation White Paper: 1–8.
- Rodiek, A. Julie, and Steve R. Best, and Casey Still. 2010. “Auburn University’s Solar Photovoltaic Array Tilt Angle and Tracking Performance Experiment.” American Institute of Aeronautics and Astronautics: 46th AIAA/ASME/SAE/ASEE Joint Propulsion Conference & Exhibit. 1–5. doi:10.2514/6.2010-7098.
- Roth, P., A. Georgiev, and H. Boudinov. 2005. “Cheap Two Axis Sun following Device.” Energy Conservation and Management 46 (7–8): 1179–1192. doi:10.1016/j.enconman.2004.06.015.
- Semma, R. P., and M. S. Imamura. 1980. “Sun Tracking Controller for Multi kW Photovoltaic Concentrator System.” In Proceedings of the 3rd International Photovoltaic Sol Energy Conf, Cannes, France 27 (31): 375–380. doi:10.1007/978-94-009-8423-3_54.
- Song, Jifeng, Yongping Yang, Yong Zhu, and Zhou Jin. 2013. “A High Precision Tracking System Based on a Hybrid Strategy Designed for Concentrated Sunlight Transmission via Fibers.” Renewable Energy 57 (2013): 12–19. doi:10.1016/j.renene.2013.01.022.
- Sproul, A. B. 2007. “Derivation of Solar Geometric Relationships Using Vector Analysis.” Renewable Energy 32 (2007): 1187–1205. doi:10.1016/j.renene.2006.05.001.
- Suri, Marcel, Tomas Cebecauer, Artur Skoczek, and Juraj Betak. 2012. “Solar Electricity Production from Fixed-inclined and Sun-Tracking c-Si Photovoltaic Modules in South Africa.” Southern African Solar Energy Conference (SASEC), slovakia, south africa, 1–8.
- Tariq, C. Miqdam, I. A. Khalil, A. K. Hussein, Feras Hasoon, S. Hakim Sultan Aljibori, A. K. Ali Alwael, S. Firas Raheem, and H. A. Ali Alwaeli. 2012. “Effect of Design Variation on Saved Energy of Concentrating Solar Power Prototype.” Proceedings of the World Congress on Engineering 3 (1): 1–6. ISBN: 978-988-19252-2-0.
- Tous, El Yousif, O. Omar Badran, and Anwar Al-Mofleh. 2012. “Thermal Evaluation of a Sun Tracking Solar Cooker.” International Journal of Energy and Environment 3 (1): 83–90.
- Tripanagnostopoulos, Y., M. Souliotis, S. Tselepis, V. Dimitriou, and Th. Makris. 2005. “Design and Performance Aspects for Low Concentration Photovoltaics.” European Photovoltaic Solar Energy Conference and Exhibition, Barcelona, Spain.
- Tudorache, Tiberiu, and Constantin Daniel Oancea, and Lliviu Kreindler. 2012. “Performance Evaluation of a Solar Tracking PV Panel.” Bucharest Scientific Bulletin, Series C: Electrical Engineering 74 (1): 3–10. ISSN: .
- Wang, Jing Min, and Chia Liang Lu. 2013. “Design and Implementation of a Sun Tracker with a Dual-Axis Single Motor for an Optical Sensor-Based Photovoltaic System.” Sensors 13 (2): 3175–3168. doi:10.3390/s130303157.