Abstract
The building sector has been regarded as a potential sector where there is large capacity to reduce the climate change effect. This study has proposed solutions to mitigate environmental impacts and achieve low CO2 emission from residential sector. Therefore, full life cycle assessment (LCA) has been run to assess the CO2 emission and its effect on the atmosphere and climate change. Based on the result, timber scheme is the best choice due to releasing less CO2 emissions to the atmosphere. However, house builders in Malaysia have almost completely neglected timber as a building material, with timber use as building components reduced to 5%. In this study, LCA Software was used to assess CO2 emissions from different wall construction. The alternative building scheme has been made by reinforce steel stud, wooden beam and timber wall (S8) to improve the scheme deficiency while releasing less CO2 emissions compared to other schemes. Therefore, S8 has a decreased CO2 effect by 85% less than precast concrete frame and 90% less than brick over their lifetime. (S8) increased the load bearing compared to conventional timber beam. Thus, new scheme S8 could be replaced by current scheme and promote more adjustable scheme for Malaysian housing.
1. Introduction
Residential buildings are responsible for more than 40% of global energy use and as much as one third of global greenhouse gas emissions. Malaysia intends to reduce its greenhouse gas (GHG) emissions intensity by 45% by 2030 (second national communication of the UNFCCC (NC2)). Given the above target, in 2009 Malaysian Prime Minister Datuk Seri Najib Razak announced that Malaysia would cut 40% of the CO2 emissions intensity by 2020 (Dryzek, Norgaard, and Schlosberg Citation2011). This article presents a life cycle assessment (LCA) for a Malaysia single-family house comparing seven alternative exterior wall (scheme) solutions aimed at identifying environmentally preferable solutions.
Climate change mitigation of building construction or improvement in the building construction process will lead to significant economic, financial, social and environmental benefits (Wang, Zmeureanua, and Rivard Citation2005). To realise such benefits it is necessary to not only select the appropriate technologies, but also the right materials (Moeck and Yoon Citation2004). The demands for residential building have been increasing over the past two decades. Based on the study of (Yoke, Hassim, and Kadir Citation2003), home construction from 1995 to 2020 would be around 8,850,554 units. This implies that emissions from Malaysian building sector have been increasing year by year.
Recent measurement shows there is no sign of reduction in Malaysia carbon dioxide emissions, which was approximately 257.69M mt (metric tons) in 2014 (Burck, Christoph, and Bohnenberge Citation2015) compared to 6.188 M in 1965 (World Development Indicators Report). Results show an increasing amount every year while Malaysia aims to decrease the CO2 emission. As the above figure shows, over the past 40 years the emission rate of carbon dioxide reached a maximum. Carbon dioxide emissions are normally the result of burning of fossil fuels, manufacture of cement, transportation and other activities related to emission. One of the main sectors related to CO2 emission is building sector that has a same dramatic increasing trend, and no prompt action has been taken as yet to control it.
The trend shows an increasing amount of CO2 emission has been released to the atmosphere every year by Malaysian building sector. Climate change recorded daily by Keeling_Curve from the Scripps Institution of Oceanography revealed that carbon dioxide in the air is 400.03 ppm (Keeling and Whorf Citation2004, October ). Malaysia was ranked 51st in CCPI (Climate Change Performance Index) (Burck, Christoph, and Bohnenberge Citation2015) in 2014 which is ‘very poor’ in comparison to Japan or Singapore and the ranking in 2015 (Burck, Christoph, and Bohnenberge Citation2015) shows an even worse scenario where Malaysia has fallen to 52nd. Therefore, an immediate response should be considered to control CO2 emission in all categories where emissions can be eliminated.
The remainder of this research is organised as follows: Section 1 of this manuscript has been related to the introduction. Section 2 expanded and described the Literature review; Section 3 explained the impact assessment methods and goals of this study. Section 4 assesses the environmental effect of alternative residential building choice in Malaysia. Section 5 assesses the status of best building scheme in order of popularity. Section 5 assesses the status of best building scheme in order of popularity and interprets results with specific focus on new CO2 emissions. The objective of this research is running the full LCA of current building structure available in Malaysia to introduce the best building scheme that has the least effect on climate change.
2. Literature review
Three billion tons of raw materials, accounting for almost 50% of the total flow of the global economy, has been used in the manufacturing of building products and components worldwide every year (Roodman and Lenssen Citation1995; Anink, Boonstra, and Mak Citation1996). Basically, raw materials are extracted, processed, transported, applied to the construction phase and finally used by the building sector. Generally, the building sector is responsible for consuming high amount of materials with high-embodied energy content, such as aluminium and cement and the production of these materials requires the application of fossil fuels, thus resulting in CO2 emissions.
The first life cycle study about residential building was accomplished about 19 years ago (Adalberth Citation1997b). For example, Adalberth (Citation1997a) proposed a methodology to calculate the full life cycle of building and applied it on three prefabricated Swedish single-family houses.
With recent interest in decreasing environment effect from building sector, a number of researches have highlighted the importance of the environmental impact caused by construction material production (Chen, Burnett, and Chau Citation2001; Mithraratne and Vale Citation2004; Citherlet and Defaux Citation2007; Ding Citation2007; Huberman and Pearlmutter Citation2008; Monteiro and Freire Citation2012). For example, Peuportier (Citation2011) studied three different building structures built by concrete, wood etc. and the result shows that wooden structure had the lowest impact compared to concrete. Monteiro and Freire (Citation2012) evaluated LCA study of seven alternative building materials to identify environmentally preferable solutions. These results indicate the wood-wall is the preferable solution. Frenette et al. (Citation2010) assessed five wood frame exterior walls with three life cycle impact assessment method LCIA methods namely: TRACI, Impact 2002 and EI’99. The result shows that wood has a small effect on environmental impact. Furthermore, the result of (Impact 2002; and EI′99) in this study represented that climate change category is acceptable to be used as a single environmental index. Tighnavard and Kadir (Citation2013) studied the LCA of comparing human health effect of timber and concrete and result indicated that timber had a lower damage impact in construction and use phase of building than concrete. Koch (Citation1992) contended that using wood instead of alternative material such as concrete, steel and brick can significantly reduce carbon dioxide to the atmosphere. This reduction amount is about 717 million gallons of oil annually, and about 7.5 million tons of carbon dioxide yearly. Gustavsson and Sathre (Citation2006) assessed wood building vs. concrete-framed building. The result shows that wood frame has lower CO2 emission than concrete frame and use of wood means reduction in fossil fuel consumption. Kuenniger and Richter (Citation1995) compared utility poles made of wood, concrete and steel. They found the wood poles to have lower environmental impact in most categories, including GHG emission, than the other materials. Scharai-Rad and Welling (Citation2002) analysed single-family houses made with either wood or brick. They considered the utilisation of processing and demolition residues to replace fossil fuels, and found that net GHG emission decreased as the volume of recovered wood increased.
In the previous study of Kadir and Tighnavard (Citation2015) the cradle to gate study had been done that included the extraction of raw material till erection of building, but the current study has surveyed the full life cycle of building including end of life and demolition. Therefore, the result of assessing the environmental impact of the current research is more comprehensive and reliable. In addition, the new approach for reducing the global warming has been suggested in this study, which has some advantages compared to previous schemes.
3. LCA methodology and LCIA method
This research represents a LCA analysis for a Malaysian single-family house and compared alternative building scheme material solutions aimed at identifying environmentally preferable solutions. LCA is a technique to assess the potential environmental impacts associated with a product or service throughout its life cycle. The case study implemented using the Simapro 8.0 Software has been based on a basic architecture model (Figure ) representing different schemes (precast concrete, block work, brick, timber etc.) of single-family house.
Prominent method to assess the global warming effect by Simapro Software 8.0 in this study was life cycle impact assessment (LCIA) IPCC GWP (The Intergovernmental Panel on Climate Change – global warming potential). The result represents the IPCC method (LCIA) for global warming in kg CO2 eq into the air and related damage of climate change. Global warming potential values were recorded on a 20, 100 and 500-year timescale in accordance with IPCC’s 4nd Assessment Report, because the effect on the atmosphere will not disappear in a short timeframe. The result of LCIA has presented mid-point indicators, which typically represent the environmental situation that requires solutions to current problems based on international conventions GWP.
Based on the international standard ISO14040 (Citation2006), any LCA study is initiated by a goal, and the goal of this assessment is to determine the most preferable building scheme for Malaysian residential house. Therefore, decision-making can be explicit and easy. According to ISO 14040 (Principles and Framework 2006), the scope has been accomplished with a functional unit, which is a reference unit used to quantify the system performance in LCA techniques. In the present study, the functional unit ‘M2 of wall area’ is chosen. Contribution to greenhouse gas from a residential one story building in Johor Bahru, Malaysia with an expected life span of 50 years and 75 M2 of living area was assigned for assessment of a life cycle scenario for an improved solution in this study. The second step of LCA study is Inventory analysis, which is related to data collection. Data have been collected from various sources such as journals and books, but mostly from visiting the manufacturing and building sites. The last stage is Interpretation (Table ) that contains the conclusion and recommendations obtained from impact assessment stage (global warming IPCC).
Designers are concentrating on minimising the impact their buildings make on the environment. Building construction possesses life cycle stages including product stage (raw materials supply, transport and manufacture), construction stage (transport and construction installation, use stage (maintenance, repair and replacement) and end-of-life stage (deconstruction, transport, recycling/re-use and disposal). All stages have been assessed in this research by Simapro software in order to determine the CO2 emission. Therefore, a decision has to be made to reduce and minimise their effect on climate change by human activity. Meanwhile, other activities such as the energy requirement of other operation-phase activities (lighting, electric appliances, cooking and domestic hot water) have not been included since they do not affect the exterior wall (Monteiro and Freire Citation2012).
Table shows the characterisation of each building component for different schemes. The approach of determining the environmental impact associated with any product from cradle to grave is called life-cycle assessment (LCA). Therefore, the boundary of this study has been expanded from cradle to grave. The phase is including the system boundary cover extraction of raw material (cradle) to end of life (grave) of product. All the material characterisation, material weight, size and thickness have been collected from site observation in the state of Johor Bahru.
Table 1. Characterisation of scheme components.
End of life includes incinerated, land filled and recycled, which depends on the types of material that have been used in building scheme. This study focused on extraction of raw material used in manufacturing including transportation, repairing of wall material and demolition. The primary data have been shown in Table .
Life cycle inventory measures all the raw materials and energy related to the particular product or process, inputs and outputs to manufacture on a per unit basis within carefully defined system boundaries of this research. Table described the quantity of materials in any scheme and mass of the various building components. The inventory data associated with this process were obtained from Kellenberger and Althaus (Citation2009).
The total resource input for building mass is the sum of all attributes expressed in kilogram. The weight of the functional unit for (timber post & beam -S7) hardwood of 1 m2 is 146 kg/m2 and the total weight of screws to attach the panels and beams is 40 kg for the whole scheme. Transportation includes hauling the wood frame and beam structure 40 km by truck from manufacturing site and a passenger car has been used to haul screws and other components over a distance of 4 km. The inventory data associated with this process were obtained from Ecoinvent database.
Block (S1) is the first material assessed in this study and lightweight block of 1 m2 is 117 kg/m2 has been applied for Malaysian building. Materials have been transported by lorry from 30 km away to site. The mortar for block used was 4 kg/m2. End of life includes disassembling scheme for disposal and transfer to landfill, a distance of 50 kms. The fuel consumed by lorry use has already been assigned. The second scheme widely used in conventional building in Malaysia consists of 1/3 brick plus 2/3 concrete (S2) and material is inclusive of bricks and concrete. After demolition, brick and concrete were transferred 50 km away from site.
The third scheme is brick (S3) which contains one layer of brick for interior walls and double layer for exterior walls. In end of life stage, all 46500 kg are transferred for disposal by lorry, a distance of 45 km. Precast concrete (S4) has recently become one of the most popular schemes in Malaysia. However, the entire environmental impact has not been assigned yet in any study for Malaysian sector. In the end of life stage, all material will be sent by lorry for disposal within a range of 35 km. The steel scheme (S5) contains of few subcomponents such as steel stud, wool for insulation, screws for attaching the components to each other and finally board to cover the external side of walls. This scheme is the only pattern that has a different end of life stage in which the material will be recycled for reuse in the demolition stage.
Brick & timber (S6) is one of the schemes that has been used in Malaysia in the past few decades. The brick in this scheme is used mostly to beautify the building aspect. These scheme components are comprised of wood, screw, brick and mortar between brick layers. Normally, half of the walls are applied by brick and the other half is built using wood. End of the life stage and demolition wood is disposed to landfill and brick disposed about 40 km away from site by lorry. The most conventional type of building material in Malaysia is timber post & beam (S7) in which hardwood is applied for wall panels. The end of the life is separated into two parts, landfill and waste incineration. In the end of life, most of the wood is usually disposed into the landfill.
4. Results and discussion
4.1. Wall scheme comparison
This research presented a comparison of the seven different combinations of real construction scenarios for external and internal walls that are presently applied in Malaysia while taking into account the environmental aspects. Industrial building system (IBS) (CIDB Citation2009) is one of the new building systems that have been introduced to Malaysia in order to achieve faster completion of construction. Hereby, in this study some types of IBS that are currently in use in Malaysia have been chosen for assessment. These types are interlocking block system (S1), precast concrete (S4), steel framework system (S5), prefabricated timber frame system (S7). Table shows the result of CO2 emission of seven different schemes over their full lifetime.
Table 2. Comparison of seven different wall scenarios.
Interlocking block system (S1) released 2.55E+04 kg CO2 eq, 2.35E+04 kg CO2 eq, 1.92E+04 kg CO2 eq within time frames of 20, 100 and 500 years and it has highest effect and burden to environment compared to other schemes. (S1) has been known as one of the off-site (IBS) constructions in Malaysia and it has been encouraged by government over the past few decades. However, in terms of environmental issues it is not good choice at all. The second scheme that has a high emission to atmosphere is Steel scheme system (S5). The global warming emission for steel structure are 1.97E+04 kg CO2 eq, 1.95E+04 kg CO2 eq and 1.91E+04 kg CO2 eq in time frames of 20, 100 and 500 years. This scheme released the second highest amount of emission in comparison with other buildings.
Figure represented a diagram of comparison between schemes. As shown, Brick system (S3) is ranked third in terms of release of CO2 emission. The emission related to scheme S3 is 1.44E+04 kg CO2 eq, 1.39E+04 kg CO2 eq and 1.32E+04 kg CO2 eq in time frames of 20, 100 and 500 years. Precast concrete (S4) is one of the most common components for building in Malaysia. Despite much interest demonstrated towards the use of precast, results show that this material is not the most environmentally friendly component for Malaysia. The emission from precast concrete S4 is 9.06E+03 kg CO2 eq, 8.92E+03 kg CO2 eq and 8.45E+03 kg CO2 eq in time frames of 20, 100 and 500 years.
Figure 2. LCIA IPCC GWP scenario analysis results.
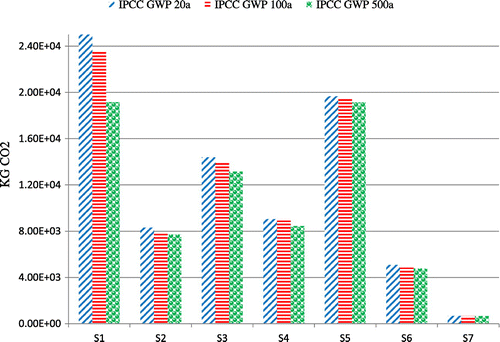
Brick & concrete scheme S2 and Timber & Brick scheme S6 are schemes that released less CO2 emission compared to other schemes. The effect of brick & concrete scheme S2 to atmosphere by releasing CO2 is 8.32E+03 kg CO2 eq, 7.90E+03 kg CO2 eq and 7.73E+03 kg CO2 eq in time frames of 20, 100 and 500 years. However, the amount for Timber & Brick scheme S6 is 5.08E+03 kg CO2 eq, 4.85E+03 kg CO2 eq and 4.79E+03 kg CO2 eq in time frames of 20, 100 and 500 years. This result shows the initial impact of life cycle thinking and highlights the consequences of different material choices. Prefabricated timber frame system (S7) represents the timber scheme for housing in Malaysia and the result in Figure shows it releases less CO2 emission to the environment over its life span. The emission related to S7 is 6.81E+02 kg CO2 eq, 6.72E+02 kg CO2 eq and 6.69E+02 kg CO2 eq in time frames of 20, 100 and 500 years. The result shows timber (S7) is the perfect choice in order to release less CO2 emission.
Result of assessing the whole life cycle of building scheme shows that timber scheme has profoundly less effect on climate change. Although timber has been nominated as the best choice of material in previous study by Kadir and Tighnavard (Citation2015), end of life which includes the demolition of building and transfer of the material for recycle and landfill has not been considered for assessing the life cycle calculation and therefore not assigned for environmental impact.
This research has covered the full LCA (extraction of raw material, use phase and demolition). The result of nominating timber as the best material for releasing less CO2 emission remains the same because the extraction and production of raw material has dominated due to effect on the environment compared to demolition phase. Thus, another result is that in Malaysia, the production phase for building material uses more energy leading to about 70% to 80% greater contribution to the environmental impact.
4.2. Lack of interest in wood components related to Malaysian construction
The use of wood in building has been substantially decreased in the last 40 years in Malaysia. Based on Housing and Population Census Malaysia (2010) use of wood in building elements has decreased from 65% (2/3 of buildings used timber before 1970) to 5% in the last 40 years. Thus, to encourage Malaysian building industry to use wood in their buildings, this study first reviewed the grounds for this lack of interest in wood in construction and then presented a new scheme for residential construction.
Not all wood products in the market have high density that can be trusted for load bearing and its load bearing has not been tested. This is the main reason that wood structure has been neglected during the past few decades in Malaysia. Thus, with the advantage of wood in decreasing the CO2 emission from housing structure, this research aims to add the contribution of wood into the building scheme by removing its negative side. Che-Ani et al. (Citation2009) evaluated and assessed traditional building methods in Malaysia and discovered that most problems in timber structure occurred on the beams of a building, in both staircase and structural beams. According to surveys, the element of the beam in staircase area is found to have serious defects caused by fungal infestation, with the type of defect being dry rot. The second serious defect is caused by the fungal infestation located behind the area of defective beams.
Based on Burden (Citation2004), most defects in timber structure are result of lack of routine maintenance, thus new proposed scheme has a stronger post and beam, which stay strong for longer time. According to Burden (Citation2004), defects refer to an improper condition that affects the structure, leading to failure or low performance and utilisation of a wooden building. By improving the structure with proper steel stud, not only does the scheme have a longer life span, it can be seen as attractive by homebuilders and residents.
The main weakness of timber structure as already mentioned is related to wood beam, hence the new beam design has to eliminate this problem and improve the stability and strength of this component. Therefore, new beam design has been composed of wooden beam covered by steel stud. In comparison with stone and concrete, wood is relatively light and usually less expensive. Steel is actually one of the most efficient structural materials on our planet (Abdul Quader et al. Citation2015). Based on Johar et al. (Citation2013), columns are the 2nd part of timber building that had been discovered by detection and detonation. Defects in column are mostly caused by mechanical factor and 52% are caused by aesthetical defects. This study shows that column and beam is the part of wooden building that are most affected by defect. Derinaldis and Tampone (Citation2007) studied wooden buildings and found that all defects of timber structure occurred on beams in buildings.
The second part of this research related to proposing a new composite beam; therefore, some background of composite will be mentioned here. Many studies are available focused on composite and infill components with different material. For example, Kavi, Toksoy, and Guden (Citation2006) studied foam filled aluminium tubes under Flexural strength loading and the result shows filled component has higher energy absorption. José and Antonio (Citation2015) studied timber–concrete composite beams with and without glass fibre reinforced polymer. The composite timber–concrete has reached an average efficiency of 80%. Other studies such as Ulrike et al. (Citation2016) and Gutkowski et al. (Citation2011) assessed wood – concrete beam structures. Over the past two decades, many research efforts have focused on different steel structures to create new reinforcing composite. For example, Varma et al. (Citation2002) studied the axial behaviour of square concrete-filled steel tubes. The study accomplished the inelastic response of steel infill with concrete and investigated the crushing of the concrete.
4.3. Designing new combination of timber with steel materials for buildings scheme
This study proposed a new scheme for Malaysian residential house with two main aims. First, to find structure that released less CO2 emission to atmosphere compared to other current schemes. Second, the material selected must be strong and have a long life span. The second aim can make the scheme an interesting choice for the house builder and residents because it does not require that extra budget be spent for repairing or replacing the old and rotten components of buildings. Unlike other building materials such as concrete or steel, wood requires less fossil energy in the manufacturing process and this leads to less energy use (Petersen and Solberg Citation2002). The reason of this usage is their availability, low thermal conductivity and aesthetic appeal compared to other materials.
Making a composite material is not a new science, as they have been applied since ancient times as humans made walls from a combination of straw and mud. Timber and steel structure is the second alternative method that introduced a steel cover over the wood frame structure. Timber framed buildings and internal beams are often admired for their pristine beauty and perfection but they are vulnerable to decay and damage. Unfortunately, poorly thought out alterations and the use of inappropriate materials have frequently wrought havoc with the timber, not only causing damage aesthetically but structurally, with the result that they are no longer adequate to bear the loads placed upon them. Figure shows a new combination of timber wall with steel covering beam. Steel stud has been used in the steel framing structure wall (H5), therefore it is strong enough to keep the beam in the proper condition for a long life span.
Furthermore, in the design of a new building scheme for Malaysia, earthquake is not issue because Malaysia has very low seismicity. Seismic provision in the structural design has never been calculated, even for multi-story building (Nabilah and Balendra Citation2012). The aim of this study is not to promote the exclusive use of wood but to use more timber, particularly from legal and sustainable sources. The main concern related to wood is prone to catching fire, which can be eliminated by applying plasterboards. Some attempts have been made to use timber for buildings, but despite some suggestions to use wood and wood composite in Malaysian buildings, timber use has increased only for tile flooring and not walls. Therefore, in this study, bigger steps are taken to control the emission. The component of new beam has been shown in Figures and , which are timber beams covered by steel studs. This new composite can be highly efficient for residential building in order to be more economical and cheaper compared to brick or concrete building.
This structure is comprised of a wood frame (timber wall panel) and wood post & beam (covered by steel stud). The steel sheet is attached to post and beam to increase the stability of the structure because if any damage occurs to the structure, a timber frame must undergo major repairs. The quantities of materials used in this structure are 23436 kg wood, 40 kg of screws for attaching the panels, 300 kg of steel studs to cover the beam and 7 kg of bolts to attach the steel stud to beam. Steels are bonded around timber beam with high strength adhesives to produce stronger composite material.
SIMAPRO software will be used to assess the proposed new scheme. Table shows the overall result of analysing the new scheme. The emission from bolt used to attach the wall panels also affects climate change by 107 kg CO2 eq, 98.7 kg CO2 eq and 94.6 kg CO2 eq for time frames of 20, 100 and 500 years. For steel studs that cover the beam, the emissions are 1.02E+03 kg CO2 eq, 1.02E+03 kg CO2 eq and 1.02E+03 kg CO2 eq for time frames of 20, 100 and 500 years. The emissions for timber material used in this scheme are 197 kg CO2 eq, 193 kg CO2 eq and 189 kg CO2 eq for time frames of 20, 100 and 500 years.
Table 3. IPCC GWP scenario analysis results for new proposed component of timber cover by steel sheet (S8).
Table shows the result of assessing full life cycle of new proposed structure, which is a combination of timber and steel stud. The carbon emission of new proposed scheme (S8) is 1.34E+03 kg CO2 eq, 1.32E+03 kg CO2 eq and 1.31E+03 kg CO2 eq for the timeframe of 20, 100 and 500 years.
5. Comparison of new scheme (S8) with others option available in Malaysia
Figure shows the comparison of the new nominated structure (S8) with the other schemes that have been assessed in the previous part. The result reveals that S8 is the best scheme and structure to decrease the carbon emission (CO2) of the Malaysian building industry. The new structure will have longer life span without needing major refurbishment. Basically, steel carries a higher load than wood, thus the combination of these two materials can provide stronger beam that can load greater and stronger components. In addition, the life expectance of this new beam is much longer than normal wood beam.
Figure 6. LCIA IPCC GWP scenario analysis results.
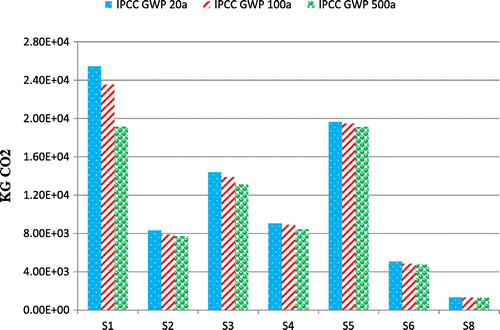
Therefore, (S8) can replace the current schemes for residential building in Malaysia. The results revealed that the new scheme Steel-Wood Frame and Timber wall (S8) represents the most beneficial environmental scheme for the building sector in Malaysia by addressing the defects of timber through the removal or replacement of structures with other materials or increasing the strength of the structure.
6. Conclusion
Full LCA assessment analysis has been accomplished for Malaysian residential building sector. Based on CCPI declaration, Malaysia is ranked in a ‘very poor’ situation, therefore this research is trying to control and decrease the CO2 emission from building sector due to construction and manufacturing, which is responsible for 14% of global warming.
The first aim is assessing the full LCA for seven building schemes. The result shows that timber houses release significantly less CO2 emission and thus have a minimal effect on global warming. However, timber houses are not currently being built by house makers in Malaysia. The second aim was to develop a new combination of building material for minimising the effect of climate change by encouraging the private and government sector to use timber material in building scheme, and the new suggested building should also improve the previous structure problem. This study presented the new prototype building with an improved structure that allows for a more environmentally safe house that released less emission.
The new scheme S8 has released 7.72E+03 kg CO2 eq less emission than precast concrete frame, 1.31E+04 kg CO2 eq less than brick and 1.83E+04 kg CO2 eq less than the steel frame system. Therefore, new combination of steel stud covering the wood beam (S8) can remove concerns about structure defects and can significantly reduce the CO2 emission from this sector. New composite beam has released less CO2 emission compared to current scheme from Malaysian sector.
Therefore, S8 has decreased the CC effect on atmosphere by 85% less than precast concrete frame and 90% less than brick over their lifetime. New beam is much stronger than normal wooden beam when strengthened with steel stud, and in terms of ductility and stiffness, the remarkable behaviour of composite timber-steel beam is demonstrated. Therefore, humidity and extra load bearing cannot change the stability of new beam, which can extend the lifetime of new proposed scheme and provide residents with assurance that it is a feasible and safe system to use.
Disclosure statement
No potential conflict of interest was reported by the authors.
Notes on contributors
Ali Tighnavard Balasbaneh, PhD, works in some university as a part-time university lecturer in Iran. His main research interests are an environmental impact, Climate change, Sustainable building construction, Green Building, Building Information Modelling (BIM), and energy efficiency in building. Previous publications have appeared in Electronic Markets, journal of Journal of Construction and Building Materials, Research Journal of Applied Sciences, Engineering and Technology, and others.
Abdul Kadir Bin Marsono, Assoc. Prof. PhD. works as a university lecturer at university technology (UTM) in Malaysia. His main research interests are in smart IBS assembly, Building Information Modelling (BIM), sustainability and Seismic issues. Previous publications have appeared in Electronic Markets, Journal of Construction and Building Materials, British Journal of Applied Science & Technology, and others.
References
- Abdul Quader, M., A. Shamsuddin, R. G. Ariffin, A. Shameem, and D. Mahidzal. 2015. “A Comprehensive Review on Energy Efficient CO2 Breakthrough Technologies for Sustainable Green Iron And Steel Manufacturing.” Renewable and Sustainable Energy 50 (10): 594–614. doi:10.1016/j.rser.2015.05.026.
- Adalberth, K. 1997b. “Energy Use during the Life Cycle of Single-unit Dwellings: Examples.” Building and Environment 32 (4): 321–329. doi:10.1016/S0360-1323(96)00069-8.
- Adalberth, K. 1997. “Energy Use during the Life Cycle of Buildings: A Method.” Journal of Building and Environment 32 (4): 317–320. doi:10.1016/S0360-1323(96)00068-6.
- Anink, D., C. Boonstra, and J. Mak. 1996. Handbook of Sustainable Building. London: James & James Science Publishers.
- Burck, J., B. Christoph, and K. Bohnenberge. 2015. The Climate Change Performance Index Results. http://www.germanwatch.org.
- Burden, E. 2004. Illustrated Dictionary of Architectural Preservation: Restoration, Renovation, Rehabilitation and Reuse. New York: McGraw Hill.
- Che-Ani, A. I., A. Zaharim, M. F. M. Zain, N. Mohd-Tawil, and M. Surat. 2009. “Timber Defects in Building: A Study of Telapak Naning, Malacca, Malaysia.” WSEAS Transactions on Environment and Development Malaysia 5 (1): 109–118.
- Chen, T. Y., J. Burnett, and C. K. Chau. 2001. “Analysis of Embodied Energy Use in the Residential Building of Hong Kong.” Energy 26 (4): 323–340. doi:10.1016/S0360-5442(01)00006-8.
- CIDB. 2009. Industrialised Building System (IBS): Implementation Strategy from R&D Perspective, Kuala Lumpur.
- Citherlet, S., and T. Defaux. 2007. “Energy and Environmental Comparison of Three Variants of a Family House during Its Whole Life Span.” Building and Environment 42 (2): 591–598. doi:10.1016/j.buildenv.2005.09.025.
- Derinaldis, P. P., and G. Tampone. 2007. “The Failure of the Timber Structures Caused by Incorrect Design-execution of the Joints.” International Symposium Mechanical Behaviour and Failures of the Timber Structures, Florence, Venice and Vicenza Italy.
- Ding, G. 2007. “Life Cycle Energy Assessment of Australian Secondary Schools.” Building Research & Information 35 (5): 487–500. doi:10.1080/09613210601116408.
- Dryzek, J. S., R. B. Norgaard, and B. Schlosberg. 2011. “The Oxford Handbook of Climate Change and Society, Oxford Handbooks” Politics & International Relations. Oxford University Press. https://www.amazon.com/Oxford-Handbook-Climate-Society-Handbooks/dp/0199566607
- Frenette, C. D., C. Bulle, R. Beauregard, A. Salenikovich, and D. Derome. 2010. “Using Life Cycle Assessment to Derive an Environmental Index for Light-frame Wood Wall Assemblies.” Building and Environment 45 (10): 2111–2122. doi:10.1016/j.buildenv.2010.03.009.
- Gustavsson, L., and R. Sathre. 2006. “Variability in Energy and Carbon Dioxide Balances of Wood and Concrete Building Materials.” Building and Environment 41 (7): 940–951. doi:10.1016/j.buildenv.2005.04.008.
- Gutkowski, R. M., N. Miller, M. Fragiacomo, and J. Balogh. 2011. “Composite Wood-concrete Beams Using Utility Poles: Time-dependent Behavior.” Journal of Structural Engineering 137 (6): 625–634. doi:10.1061/(ASCE)ST.1943-541X.0000293.
- Huberman, N., and D. Pearlmutter. 2008. “A Life-cycle Energy Analysis of Building Materials in the Negev Desert.” Energy and Buildings 40 (5): 837–848. doi:10.1016/j.enbuild.2007.06.002.
- ISO-14040, ISO 14040. 2006. Environmental Management – Life Cycle Assessment – Principles and Framework. [CHD 34 : Environmental Management].
- Johar, S., A. I. Che-Ani, N. M. Tawil, M. Surat, and S. N. Kamaruzzaman. 2013. “Preliminary Survey and Defects Analysis of Traditional Timber Mosques in Malaysia.” WSEAS Transactions on Environment and Development 9 (1): 13–23.
- José, L. M., and A. D. Antonio. 2015. “Structural Efficiency of Full-scale Timber–Concrete Composite Beams Strengthened with Fiberglass Reinforced Polymer.” Composite Structures 128: 145–154. doi:10.1016/j.compstruct.2015.03.054.
- Kadir, B. M., and A. B. Tighnavard. 2015. “Combinations of Building Construction Material for Residential Building for the Global Warming Mitigation for Malaysia.” Construction and Building Materials 85: 100–108. doi:10.1016/j.conbuildmat.2015.03.083.
- Kavi, H., A. K. Toksoy, and M. Guden. 2006. “Predicting Energy Absorption in a Foam-filled Thin-walled Aluminum Tube Based on Experimentally Determined Strengthening Coefficient.” Materials and Design 27 (4): 263–269. doi:10.1016/j.matdes.2004.10.024.
- Keeling, C. D., and T. P. Whorf. 2004. “Atmospheric CO2 from Continuous Air Samples at Mauna Loa Observatory, Hawaii, U.S.A.” In Carbon Dioxide Information Analysis Center, Oak Ridge National Laboratory.
- Kellenberger, D., and H. J. Althaus. 2009. “Relevance of Simplifications in LCA of Building Components.” Building and Environment 44 (4): 818–825. doi:10.1016/j.buildenv.2008.06.002.
- Koch, P. 1992. “Wood versus Nonwood Materials in U.S. Residential Construction: Some Energy-related Global Implications.” Forest Products Journal 42 (5): 31–42.
- Kuenniger, T., and K. Richter. 1995. “Life Cycle Analysis of Utility Poles: A Swiss Case Study.” In: Proceedings of the 3rd International Wood Preservation Symposium, Cannes-Mandelieu, France, February 6–7.
- Mithraratne, N., and B. Vale. 2004. “Life Cycle Analysis Model for New Zealand Houses.” Building and Environment 39 (4): 483–492. doi:10.1016/j.buildenv.2003.09.008.
- Moeck, M., and J. Y. Yoon. 2004. “Green Buildings and Potential Electric Light Energy Savings.” Journal of Architectural Engineering 10 (4): 143–159. doi:10.1061/(ASCE)1076-0431(2004)10:4(143).
- Monteiro, H., and F. Freire. 2012. “Life-cycle Assessment of a House with Alternative Exterior Walls: Comparison of Three Impact Assessment Methods.” Energy and Buildings 47: 572–583. doi:10.1016/j.enbuild.2011.12.032.10.1016/j.enbuild.2011.12.032
- Nabilah, A. B., and T. Balendra. 2012. “Seismic Hazard Analysis for Kuala Lumpur, Malaysia.” Journal of Earthquake Engineering 16 (7): 1076–1094. doi:10.1080/13632469.2012.685208.
- Petersen, A. K., and B. Solberg. 2002. “Greenhouse Gas Emissions, Life-cycle Inventory and Cost-efficiency of Using Laminated Wood Instead of Steel Construction. Case: Beams at Gardermoen Airport.” Journal of Environmental Science & Policy 5 (2): 169–182. doi:10.1016/S1462-9011(01)00044-2.
- Peuportier, B. 2011. “Life Cycle Assessment Applied to the Comparative Evaluation of Single Family Houses in the French Context.” Journal of Energy Building 33 (5): 443–450. doi:10.1016/S0378-7788(00)00101-8.
- Roodman, D. M., and N. Lenssen. 1995. “A Building Revolution: How Ecology and Health Concerns are Transforming Construction.” Worldwatch 124, Washington, DC.
- Scharai-Rad, M., and J. Welling. 2002. Environmental and Energy Balances of Wood Products and Substitutes. Rome: Food and Agriculture Organization of the United Nations (FAO).
- Tighnavard, A. B., and B. M. Kadir. 2013. “Life Cycle Assessment of IBS in Malaysia and Comparing Human Health on Timber and Concrete Pre-cast.” Research Journal of Applied Sciences, Engineering and Technology 6 (24): 4697–4702.
- Ulrike, D., L. Jianchun, R. Rajendra, and C. Keith. 2016. “A Dynamic-based Method for the Assessment of Connection Systems of Timber Composite Structures.” Construction and Building Materials 102 (2): 999–1008. doi:10.1016/j.conbuildmat.2015.10.009.
- Varma, A. H., J. M. Ricles, R. Sause, and L. W. Lu. 2002. “Experimental Behavior of High Strength Square Concrete-filled Steel Tube Beam-columns.” Journal of Structural Engineering 128 (3): 309–318. doi:10.1061/(ASCE)0733-9445(2003)129:9(1286).
- Wang, W., R. Zmeureanua, and H. Rivard. 2005. “Applying Multi-objective Genetic Algorithms in Green Building Design Optimization.” Building and Environment 40 (11): 1512–1525. doi:10.1016/j.buildenv.2004.11.017.
- Yoke, L.L., S. Hassim, and M. R. A. Kadir. 2003. “Computer-based Cost Control Model for Industrialised Building System Construction.” International Conference of Industrialised Building Systems, Kuala Lumpur, Malaysia, July 20–21.