Abstract
Thermal barrier coated diesel engine, also known as low heat rejection (LHR) engine have offered the promise of reducing heat rejection to the engine coolant and increase the combustion temperature which results in increase of thermal efficiency, decrease of fuel consumption and emission rate of the engine. Biodiesel derived from the vegetable oils are a promising alternative fuel for diesel fuel. The viscosity of vegetable oil after transestrification is still higher than that of diesel fuel. The various researchers have reported that the energy of the biodiesel could be released more efficiently with the concept of LHR engine. In the case of LHR engine running on different biodiesel blends, almost all experimental studies has predicted improved performance. This paper analyses and discussed the operating conditions under which the experimental studies are carried out and the factors which affect thermal efficiency and exhaust emissions in LHR engine.
Introduction
Energy conservation and efficiency have always been the quest of engineers concerned with internal combustion engines. The diesel engine generally offers better fuel economy than its counterpart petrol engine. Even the diesel engine rejects about two-thirds of the heat energy of the fuel, one-third to the coolant and one-third to the exhaust, leaving only about one-third as useful power output. Theoretically, if the heat rejected could be reduced, then the thermal efficiency would be improved, at least up to the limit set by the second law of thermodynamics. Low heat rejection engines aim to do this by reducing the heat lost to the coolant (Domakonda and Puli, Citation2012; Soltani et al. Citation2005; Jaichandar and Tamilporai Citation2003). Thermal barrier coatings (TBCs) in diesel engines lead to advantages including higher power density, fuel efficiency and multi-fuel capacity due to higher combustion chamber temperature (Zhu and Miller, Citation1999; Ramaswamy et al. Citation2000). Using TBC can increase engine power by 8%, decrease the specific fuel consumption by 15–20% and increase the exhaust gas temperature (EGT) 200 K (Ahmaniemi et al. Citation2003).
The different material deposition methods are available for coating the engine combustion chamber components. There are electron beam physical vapour deposition (EBPVD), air plasma spray (APS), high-velocity oxygen fuel (HVOF), electrostatic spray-assisted vapour deposition (ESAVD) and direct vapour deposition. Among the different methods, plasma spray is the most common method of depositing thermal barrier material for diesel engine applications. It creates a splat structure with 10–20% volume fraction of voids and cracks (Cao, Vassenb, and Stoeverb Citation2004). The generally known principle that increased operation temperatures in energy conversion systems lead to an increase in efficiency, fuel savings and reduced emissions as particles in carbon monoxides (CO), hydrocarbons (HC), but increase in NOx emissions (Qian, Nakamura, and Berndt Citation1998) . The limited reserve of fossil fuels, increasing the price of crude oils and environmental concerns, biodiesel from vegetable oil is a viable alternative fuel for diesel engine. Considerable research has been conducted to investigate the properties of biodiesel and its performance in engines because of biodiesel is renewable, non-toxic and biodegradable. Non-conventional feed stocks are the possible sources for production of biodiesel at low cost (Abedin et al. Citation2013). The combustion characteristics of LHR diesel engines are different from standard diesel engines in four ways (Sun et al. Citation1994): (a) Ignition delay period shortens; (b) Diffusion burning period increases while premixed burning period decreases; (c) Total combustion duration increases; (d) Heat release rate in diffusion burning period decreases. The selection of TBC materials is restricted by some basic requirements (Cernuschi et al. Citation1999; Ni and Zhou Citation2012; Vassen et al. Citation2000) such as (i) high melting point, (ii) no phase transformation between room temperature and operation temperature, (iii) low thermal conductivity, (iv) chemical inertness, (v) thermal expansion match with the metallic substrate, (vi) good adherence to the metallic substrate and (vii) low sintering rate of the porous microstructure. Different materials such as silicon carbide, silicon nitride, aluminium, magnesium silicate and ceramic materials like zirconia, NiCrB, MgO–ZrO2, yttria-stabilised zirconia (YSZ), partially stabilised zirconiza (PSZ) and sintered silicon nitrate (SSN) have been used as thermal insulators for a LHR engine. So far, only a few materials have been found to basically satisfy the requirements. The literature reported that almost all type of biodiesels have been used for experimental study in conventional diesel engine but only few biodiesels were tried for experimentally in LHR engine concept. This paper describes to review on different thermal barrier coating (TBC) materials that can be used for coating the engine components and its effect on engine performance and emissions running on various type of biodiesel and to identify the best thermal coating material and biodiesel in order to justify their feasibility of implementation in diesel engine application.
Conversion of standard engine in to LHR engine
Cylinder head, cylinder liner, piston crown, exhaust and inlet valves of the diesel engine were selected for the thermal barrier coating. The suitable thermal barrier material is to be deposited on combustion chamber components using thermal spraying processes. Thermal spraying is a group of coating processes wherein a feedstock material is heated and propelled as individual particles or droplets onto a surface (). The thermal spray gun generates the necessary heat using combustible gases or an electric arc. As the materials are heated, they are changed to a plastic or molten state which are confined and accelerated by a compressed gas stream to the substrate. The particles strike the substrate, flatten and form thin platelets (splats) that conform and adhere to the irregularities of the prepared substrate and to each other. As the sprayed particles impinge upon the surface, they cool and build up splat by splat into a laminar structure forming the thermal spray coating. The thermal spraying techniques can be divided into several processes that differ according to the thermal energy source and the respective kinetic energy of the sprayed particles (Hiemann Citation1996).
Plasma spraying method is the most popular technique to deposit thermal barrier coating in engine components due to its higher porosity (Karaoglanli et al. Citation2011; Salman et al. Citation2006). This method is suitable to apply in a surface which melts at a very high temperature. Prior to coating, the substrate surface was sand blasted to produce a surface roughness (Ra) of 4–5 μm. The sand blasted substrates were ultrasonically cleaned using anhydrous ethylene alcohol and dried in cold air. A plasma spray system consists of a power supply, gas source, gun and powder feeding mechanism. An arc is formed between an electrode and spray nozzle, which acts as a secondary electrode. A pressurised inert gas is passed between the electrodes where it is heated to a very high temperature (>16,000 °C) to form plasma jet (Bhatia Citation1999). The material to be deposited (feedstock) can be a powder, liquid, suspension or wire and is introduced into the plasma jet by emanating from a plasma torch. For a deposition rate of 1–5 kg/h, particle velocity reaches 200–300 m/s, porosity is reduced to 5–10% and oxide content to 1–3%. In the jet, the material is melted and propelled towards a substrate. Therefore, the molten droplets flatten, rapidly solidify and form a deposit. In this process, thickness of material is deposited on engine component in the range of 50–500 μm.
Comparison on performance and emission characteristics of different thermal barrier coated engine running on various type of biodiesel
The performance and emission characteristics of different thermal barrier coated engine running on different types biodiesel are compared to uncoated engine diesel operation have been discussed in this study. Only few of the thermal barrier coating materials has been used for biodiesel operation compared to diesel fuel (Abedin et al. Citation2014). This review manuscript has considered those papers for comparison. Thermal barrier coated materials, engine constraint and engine running on various type of biodiesel are listed in . Performance and emissions characteristics of those engines are discussed in the Table .
Table 1. Comparison on thermal barrier coated materials, engine specifications and coated engine running on various type of biodiesel.
Table 2. Performance characteristics of different thermal barrier coated engine running on various type of biodiesel compared with uncoated engine diesel operation.
Table 3. Emission characteristics of different thermal barrier coated engine running on various type of biodiesel compared with uncoated engine diesel operation.
Engine performance characteristics
An engine performance characteristic mainly consists of brake thermal efficiency (BTE) and brake-specific fuel consumption (BSFC). Both parameters could be improved in coated engine compared to uncoated engine for both diesel and biodiesel fuel. This was happened due to the reduction in heat transfer from combustion chamber wall to the cooling water. This leads to higher combustion temperature that means higher in-cylinder pressure which ensures higher mean effective pressure (Aydin Citation2013; Aydin, Sayin, and AydinCitation2015; Janardhan et al. Citation2013; Prabhahar and Rajan Citation2013; Srithar et al. Citation2013; Suresh, Kamath, and Banapurmath Citation2014). Higher in cylinder temperature also improves power and torque by 2–3% in making better vaporisation of fuel drops which results in shorter ignition delay, hence better combustion (Pradeep Kumar, Annamalai, and Premkartikkumar Citation2012) . Engine power increases with the increase of engine speed. The power produced by an engine is limited by the amount of air used in combustion. The faster an engine speed, the more air per unit time moves through the engine, the more energy is released for that unit of time. This was caused in coated engine for both diesel and biodiesel fuels (Mohamed Musthafa, Sivapirakasam, and Udayakumar Citation2011; Modi and Gosai Citation2010; Rajendra Prasath, Tamilporai, and Shabir Citation2010; Hazar Citation2009).The variations in SFC reflect the diversities in some of the fuel physical properties, especially calorific value and density. SFC in LHR engine was less than that of the standard engine for diesel fuel as well as for biodiesels due to higher gas and wall temperature. The complete combustion characteristics of biodiesels are weaker than diesel fuel due to its lower calorific value and higher viscosity. Therefore, the SFC values are higher for biodiesels compared to conventional diesel in both engines because more fuel is required for same output power. In the ignition delay (ID) period, the fuel atomises and mixes with air. This is a physical delay. Simultaneously, the chemical delay occurs due to slow chemical reaction. If the total ID period is longer, the more fuel will be injected into the combustion chamber. However, shorter ID period in LHR engine affects both the physical and chemical delay positively. The delay period is found to be decreased with the increase of cylinder pressure, temperature and equivalence ratio (Mohammed and Nemit-allah Citation2013). The increase in cylinder temperature was observed in coated engine helps to decrease in SFC compared to uncoated engine (Aydin Citation2013; Rajendra Prasath, Tamilporai, and Shabir Citation2010; Suresh, Kamath, and Banapurmath Citation2014). Hasimoglu (Citation2012) have conducted experiments on a turbocharged direct injection diesel engine coated with CaZrO3 using diesel and biodiesel(produced from sunflower oil) fuels and they reported that with the LHR diesel and STD diesel conditions the brake thermal efficiency was increased approximately 3, 4 and 6.5%, respectively, as shown , compared to STD diesel condition. This can be explained as follows: although there is a difference between fuel’s lower heating values of approximately 14%, the engine power and torque decrease to a maximum of 4.5%. It is estimated that these circumstances increased the brake thermal efficiency in STD biodiesel condition. In LHR biodiesel and LHR diesel conditions due to the reduction of specific fuel consumption, the brake thermal efficiency was increased. Hasimoglu (Citation2012) experimental result of the variation-specific fuel consumption against engine speed for the test a fuel is shown in . They found that specific fuel consumption decrease approximately 4% in coated engine for both fuels. The reason was the positive effect of increased in cylinder temperature due to heat insulation.
Figure 2. Variations of brake thermal efficiency vs. engine speed (Hasimoglu Citation2012).
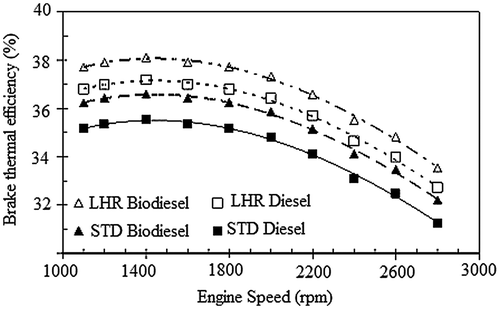
Figure 3. Variations of brake-specific fuel consumption vs. engine speed (Hasimoglu Citation2012).
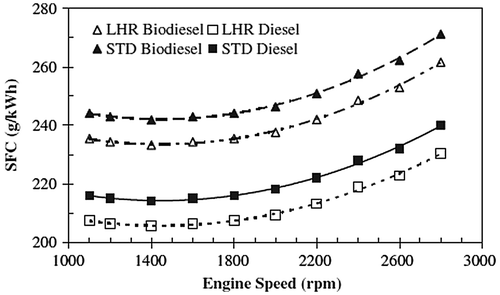
Emission characteristics
Exhaust Gas Temperature (EGT)
The EGT is an indicator of the amount of energy released during combustion. EGT increases with the increase of engine load for all fuels in both engines. This was due to the fact that fuel burning rate increases at higher loads as a result in heat release rate increases. The increase in trend is more pronounced in LHR engine as cooling heat losses decrease significantly due to thermal barrier coating. At variable speed engine, the oxygenated nature of biodiesel will lead more complete combustion, and so higher EGT at low speeds. At higher speeds the exhaust temperature of all blends decrease than diesel fuel. From Hasimoglu (Citation2012) graph result as shown in , EGT of the test engine was increased with the increment of the engine load. The temperature of exhaust gas reaches a maximum for LHR Diesel during all engines. EGT of STD biodiesel was decreased because of the reduced calorific value of biodiesel. The EGT of LHR diesel increased, due to thermal insulation. For LHR biodiesel, as if the temperature increases due to insulation, this increment in EGT is limited because of lower heating value of biodiesel.
Figure 4. Variation of an exhaust gas temperature vs. engine speed (Hasimoglu Citation2012).
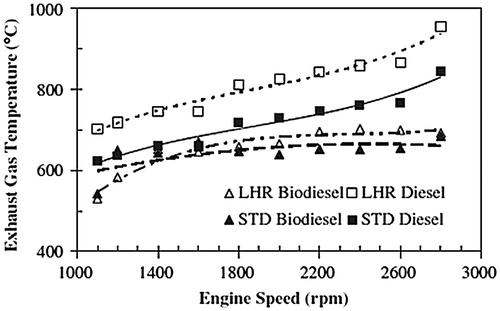
Nitrogen oxides (NOx) emission
NOx forms by chain reactions involving O2 and N2 with the presence of sufficient temperature. The oxygen concentration and surrounding temperature are the key influence factors for NOx emission. The kinetics of NOx formation is governed by Zeldovich kinetics and the availability of oxygen (Hazar Citation2011; Palash et al. Citation2013). The biodiesel and biodiesel/diesel blend fuels produced higher NOx for all engine speeds as expected. There were some reasons for this behaviour: (1) Regarding the adiabatic flame temperature, some authors state that it is slightly higher for biodiesel because of its oxygenated nature which help for more complete combustion and so higher temperature and NOx emission (Banapurmath and Tewari Citation2008; Hasimoglu Citation2012; Prabhahar and Rajan Citation2013). (2) Regarding the reduction in soot formation with biodiesel. Radiation from soot produced in the flame zone is a major source of heat transfer away from the flame, and can lower bulk flame temperatures by 25–125 K, depending on the amount of soot produced at the engine operating conditions (Hazar Citation2009; Mohamed Musthafa, Sivapirakasam, and Udayakumar Citation2011). (3) Biodiesel typically contains more double-bonded molecules than petroleum derived diesel. These double-bonded molecules have a slightly higher adiabatic flame temperature, which leads to the increase in NOx production for biodiesel(Lin, Wu, and Chang Citation2007; Sahoo and Das Citation2009). NOx emissions measured by Huseyin Aydin (Citation2013) from the gas analysing of the test engine for all test fuels are presented in . NOx emissions are mainly produced at considerably high-temperature combustion. The prolongation of the high-temperature regime results in NOx emissions accumulation. Thus, total NOx emissions increase. As can be seen in , the lowest NOx emissions were obtained for D2 usage in uncoated engine operation. When engine is insulated, the combustion temperature increases and results in increased NOx emissions. Therefore, D2 usage in the coated engine operation resulted in considerably higher NOx emissions than normal D2 operation. When vegetable based fuels are used in the test engine, the higher oxygen content is considered to improve combustion and results in higher combustion temperature and thus higher NOx emissions as well.
Figure 5. Variations of NOx emission at different engine speed (Aydin Citation2013).
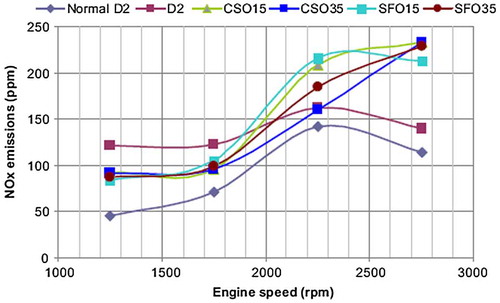
Hydrocarbon (HC) emission
Hydrocarbon emission from LHR engines is more likely to be decreased due to shorter quenching distance and leaner flammability limit associated with LHR engine combustion. High in-cylinder gas temperature and wall temperature in LHR engine assist the oxidation reactions to proceed close to completion. HC emission in LHR engine is very much low for pure diesel and biodiesels compared to uncoated engine. This happened due to the increase of after-combustion temperature as a consequence of cooling heat loss reduction in LHR engine. Neat biodiesel significantly reduces HC emission compared to blends and pure diesel fuel in both LHR and standard engine. The reasons are: biodiesels contain about 10% higher oxygen molecule than diesel; quenching distance decreases and lean flammability limit increases in LHR engine compared to uncoated engine (Prabhahar and Rajan Citation2013). Most of the investigations show reduction in HC level. From Aydin (Citation2013) results, HC emissions for all test fuels can be seen in . It can clearly be observed from that HC emissions were considerably decreased when using vegetable oil blends fuel in diesel engine. The higher oxygen contented in the vegetable oil-diesel blend fuels take part in combustion and make the combustion environment enriched with oxygen. Hence, the surplus oxygen content helps to achieve more complete combustion thus results in decreased in-complete combustion products such as HC and CO emissions. When both coated and uncoated diesel engine experiments were compared, average HC emissions were found slightly lower for coated diesel engine operation.
Figure 6. Variations of HC emission at different engine speed (Aydin Citation2013).
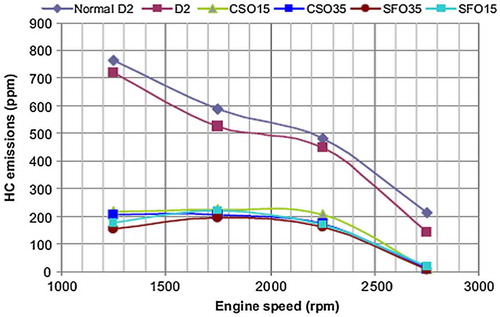
Carbon monoxide (CO) emission
CO emission occurs due to the incomplete combustion of fuel and depends on many engine parameters, mostly in-cylinder temperature and equivalence ratio (Hazar Citation2011; Karabektas Citation2009). It might be expected that LHR engines would produce less CO, for reasons similar to those for HC emission. The reduced level of pre-mixed combustion in LHR engine decreases initial production of CO and later (i.e. during diffusion combustion) high temperature accelerates CO oxidation. At lower engine speeds, due to poor combustion, CO emission is high at lower speed and decreases at medium speeds in both LHR engine and standard engine for all fuels. Due to ceramic coatings the post compression temperature increases which decreases CO emission in LHR engine. As higher oxygen content makes better combustion, the higher biodiesel blended fuel decreases CO emission than diesel fuel. When both coated and uncoated diesel engine experiments were compared with each other, average CO emissions were found slightly lower for coated diesel engine operation even though the main purpose of insulation of the engine was to improve the performance and usability of vegetable oils in diesel engines. (Banapurmath and Tewari Citation2008; Hasimoglu Citation2012; Hazar Citation2011; Modi and Gosai Citation2010). It can clearly be observed from of Aydin (Citation2013) results.
Figure 7. Variations of CO emission at different engine speed (Aydin Citation2013).
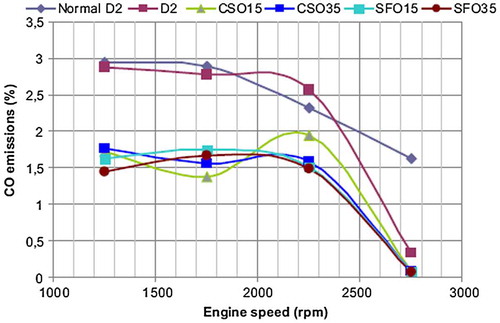
Smoke emission
LHR engines would produce less smoke and particulates than standard engines for reasons such as high-temperature gas and high-temperature combustion chamber wall. Factors such as short ignition delay, poor air–fuel mixing are also responsible for the formation of smoke and particulates. Biodiesels and their blends have favourable effect on smoke emissions due to enhanced soot oxidation, which was made possible by both the high combustion temperature and the intense turbulence created by the reversed squish (Hasimoglu et al. Citation2011). As the cylinder wall and gas temperature increase due to thermal insulation, the smoke density decreases by a large amount in LHR engine. The trend is more pronounced in case of biodiesel fuels and their blends. Smoke opacity levels of diesel fuel and vegetable fuels application in the coated diesel engine for different engine speeds are presented by Aydin (Citation2013) in .Smoke levels obtained in the exhaust were decreased with increase of engine speed for all test fuels. It can be attributed to the increased air jets, swirl and turbulence movements which increase more homogeneous charge and thus resulting in more complete combustion. The highest smoke opacity levels were ever obtained for diesel fuel in uncoated engine. The oxygen inherently contained in the biodiesel blends which remove any possible local oxygen deficiencies are considered to have helped in the concern of decreasing smoke level. At high engine speeds, the combustion must be completed in a shorter period than that of low speed. So, it can be said that the reduction in the ignition delay period assists to reduce the smoke further.
Figure 8. Variations of smoke opacity at different engine speed (Aydin Citation2013).
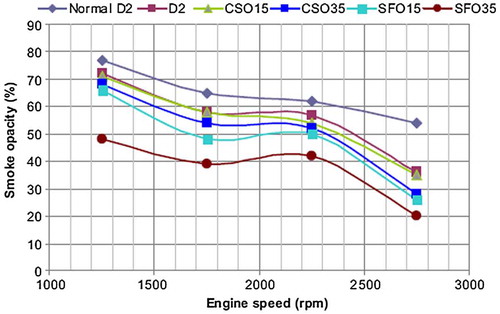
Conclusion
In this study, the usability of various biodiesel as fuel in a thermally insulated diesel engine coated with different material was reviewed. Comparisons were made on the different thermal barrier material-coated engine fuelled by various biodiesel with normal uncoated diesel engine operation using diesel fuel. Almost all authors have reported that an improved engine performance in terms of decreased specific fuel consumption and increased brake thermal efficiency by combustion chamber insulation hence, heat transfer through the walls is reduced which results in higher in cylinder temperature. CO and HC emissions were considerably reduced for all mentioned biodiesel fuels usage in the coated engine. They have mentioned common reasons as the surplus oxygen content of biodiesel blend fuels helps to achieve a more complete combustion thus results in decreased in-complete combustion products such as HC and CO emissions. However, NOx emissions were increased probably due to the increased combustion temperature. Smoke emissions were lower than those obtained from diesel fuel in both coated and uncoated diesel engine.
The critical and comprehensive studies have been carried out to justify the future possibilities of the LHR engine to be used in wider application from the view point of best thermal barrier materials, coating techniques and biodiesel. The following conclusions are drawn from the detailed literature review.
Partially stabilised zirconia (PSZ) is identified as the best thermal barrier coating material for LHR concept among the mentioned materials in the table1, because of PSZ coating exhibited low thermal diffusivity, thermal conductivity, chemical stability and strong adherence to the substrate than the other thermal barrier materials have mentioned in the studies.
Atmospheric plasma thermal spray method is extensively used to make thermal barrier coating and to achieve the required thickness of coating by the selection of the optimum combination of input variables (current, powder feed rate, stand-off distance, number of passes, etc).
Cotton seed oil, corn oil, jatropha and pongamia methyl ester are found as the best biodiesel fuel among the mentioned biodiesel in the table1, because of their superior physiochemical properties, viz. viscosity and calorific value are close to diesel fuel and they are in non-edible oil.
R&D activities required to promote the further development are
The higher combustion chamber temperature of coated engine deteriorates the properties of lubricating oil. Hence, the main direction of the research in adiabatic engines is in finding of alternate lubricating oils should be capable of retaining viscosity at the higher temperatures encountered in the engine.
The thicknesses of the coating must be optimised to minimise the stresses under service conditions.
The objectives of improved thermal efficiency, improved fuel economy and reduced emissions are attainable, but much more investigations under proper operating constraints with improved engine design are required to explore the full potential of low heat rejection engines
Disclosure statement
No potential conflict of interest was reported by the author.
Notes on contributor
M Mohamed Musthafa is a senior assistant professor in School of Mechanical Engineering, SASTRA University. His area of interests are Alternate fuels for Internal combustion engine, fuel combustion, Heat transfer and solar.
References
- Abedin, M., H. Masjuki, M. Kalam, A. Sanjid, S. Rahman, and B. Masum. 2013. “Energy Balance of Internal Combustion Engines Using Alternative Fuels.” Renewable and Sustainable Energy Reviews 26: 20–33. doi:10.1016/j.rser.2013.05.049.
- Abedin, M. J., H. H. Masjuki, M. A. Kalam, A. Sanjid, and A. M. Ashraful. 2014. “Combustion, Performance, and Emission Characteristics of Low Heat Rejection Engine Operating on Various Biodiesels and Vegetable Oils.” Energy Conversion and Management 85: 173–189. doi:10.1016/j.enconman.2014.05.065.
- Ahmaniemi, S., C. Gualco, A. Bonadei, and R. Di Maggio. 2003. Characterization of Modified Thick Thermal Barrier Coatings, 1477–1486. Ohio: Thermal Spray.
- Aydin, H. 2013. “Combined Effects of Thermal Barrier Coating and Blending with Diesel Fuel on Usability of Vegetable Oils in Diesel Engines.” Applied Thermal Engineering 51: 623–629. doi:10.1016/j.applthermaleng.2012.10.030.
- Aydin, S., C. Sayin, and H. Aydin. 2015. “Investigation of the Usability of Biodiesel Obtained from Residual Frying Oil in a Diesel Engine with Thermal Barrier Coating.” Applied Thermal Engineering 80: 212–219. doi:10.1016/j.applthermaleng.2015.01.061.
- Banapurmath, N., and P. Tewari. 2008. “Performance of a Low Heat Rejection Engine Fuelled with Low Volatile Honge Oil and Its Methyl Ester (HOME).” Proceedings of the Institution of Mechanical Engineers, Part a: Journal of Power and Energy 222: 323–330. doi:10.1243/09576509JPE514.
- Bhatia, A. 1999. Thermal Spraying Technology and Applications. Washington, DC: US Army Corps of Engineers.
- Cao, X. Q., R. Vassenb, and D. Stoeverb. 2004. “Ceramic Materials for Thermal Barrier Coatings.” Journal of the European Ceramic Society 24: 1–10.10.1016/S0955-2219(03)00129-8
- Cernuschi, F., P. Bianchi, M. Leoni, and P. Scardi. 1999. “Thermal diffusivity/Micro Structure Relationship in Y-PSZ Thermal Barrier Coatings.” Journal of Thermal Spray Technology 8: 102–109.10.1361/105996399770350629
- Domakonda, V. K., and R. K. Puli. 2012. “Application of Thermal Barrier Coatings in Diesel Engines: A Review.” Energy and Power 2 (1): 9–17. doi:10.5923/j.ep.20120201.02.
- Hasimoglu, C. 2012. “The Effects of Biodiesel on Performance and Exhaust Emissions of a Low Heat Rejection Diesel Engine.” Energy Sources, Part a: Recovery, Utilization, and Environmental Effects 34: 570–580. doi:10.1080/15567031003627955.
- Hasimoglu, C., M. Ciniviz, I. Ozsert, Y. Icingur, A. Parlak, and M. Sahir Salman. 2008. “Performance Characteristics of a Low Heat Rejection Diesel Engine Operating with Biodiesel.” Renewable Energy 33: 1709–1715. doi:10.1016/j.renene.2007.08.002.
- Hasimoglu, C., M. Ciniviz, A. Parlak, I. Ozsert, and Y. Icingur. 2011. “Part Load Performance Characteristics of a Low-heat Rejection Diesel Engine Fueled with Biodiesel.” Journal of Energy Engineering 137 (2): 70–75.10.1061/(ASCE)EY.1943-7897.0000037
- Hazar, H. 2009. “Effects of Biodiesel on a Low Heat Loss Diesel Engine.” Renewable Energy 34: 1533–1537. doi:10.1016/j.renene.2008.11.008.
- Hazar, H. 2011. “Characterization and Effect of Using Cotton Methyl Ester as Fuel in a LHR Diesel Engine.” Energy Conversion and Management 52: 258–263. doi:10.1016/j.enconman.2010.06.066.
- Hazar, H., and U. Ozturk. 2010. “The Effects of Al2O3–TiO2 Coating in a Diesel Engine on Performance and Emission of Corn Oil Methyl Ester.” Renewable Energy 35: 2211–2216. doi:10.1016/j.renene.2010.02.028.
- Hiemann, R. B. 1996. Plasma-spray Coating-principles and Applications. New York: Wiley.10.1002/9783527614851
- Jaichandar, S., and P. Tamilporai. 2003. “Low Heat Rejection Engines – An Overview.” SAE Technical Paper. 2003- 01-0405. doi:10.4271/2003-01-0405.
- Janardhan, N., M. V. S. M. Krishna, P. Ushasri, and P. V. K. Murthy. 2013. “Control of Exhaust Emissions of Jatropha Oil in Crude Form and Biodiesel from High Grade Low Heat Rejection Diesel Engine.” International Journal of Mechanical and Production Engineering Research and Development 3: 199–212.
- Karabektas, M. 2009. “The Effects of Turbocharger on the Performance and Exhaust Emissions of a Diesel Engine Fuelled with Biodiesel.” Renewable Energy 34: 989–993. doi:10.1016/j.renene.2008.08.010.
- Karaoglanli, A., E. Altuncu, I. Ozdemir, A. Turk, and F. Ustel. 2011. “Structure and Durability Valuation of YSZ + Al2O3 Composite TBCs with APS and HVOF Bond Coats under Thermal Cycling Conditions.” Surface Coating Technology 205: S369–S373. doi:10.1016/j.surfcoat.2011.04.081.
- Lin, Y-F., Y.-P. G. Wu, and C. T. Chang. 2007. “Combustion Characteristics of Waste Oil Produced Bio-diesel, Diesel Fuel Blends.” Fuel 86: 1772–1780. doi:10.1016/j.fuel.2007.01.012.
- Modi, A., and D. Gosai. 2010. “Experimental Study on Thermal Barrier Coated Diesel Engine performance with Blends of Diesel and Palm Biodiesel.” SAE International Journal of Fuel Lubricants 3: 246–259. doi:10.4271/2010-01-1519.
- Mohamed Musthafa, M., S. Sivapirakasam, and M. Udayakumar. 2011. “Comparative Studies on Fly Ash Coated Low Heat Rejection Diesel Engine on Performance and Emission Characteristics Fueled by Rice Bran and Pongamia Methyl Ester and Their Blend with Diesel.” Energy 36: 2343–2351. doi:10.1016/j.energy.2010.12.047.
- Mohamed Musthafa, M., S. P Sivapirakasam, and M. Udayakumar. 2012. “Comparative Study of Al2O3 Coated LHR Engine Characteristic Using Rice Bran and Mahua Methyl Ester as a Fuel.” International Journal of Vehicle Design 59 (2/3): 182–195.10.1504/IJVD.2012.048694
- Mohammed E.-K., M. A. Nemit-allah. 2013. “Experimental Investigations of Ignition Delay Period and Performance of a Diesel Engine Operated with Jatropha Oil Biodiesel.” Alexandria Engineering Journal 52(2): 141–149. doi:10.1016/j.aej.2012.12.006.
- Ni, L., and C. Zhou. 2012. “Effects of Surface Modification on Thermal Cycling Lifetime of Thermal Barrier Coatings with HVOF NiCrAlY Bond Coat.” The Journal of Progress in Natural Science: Materials International 22 (3): 237–243. doi:10.1016/j.psnc.2012.04.007.
- Palash, S. M., H. H. Masjuki, M. A. Kalam, B. M. Masum, A. Sanjid, and M. J. Abedin. 2013. “State of the Art of NOx Mitigation Technologies and Their Effect on the Performance and Emission Characteristics of Biodiesel-fueled Compression Ignition Engines.” Energy Conversion Management 76: 400–420. doi:10.1016/j.enconman.2013.07.059.
- Prabhahar, M., and K. Rajan. 2013. “Performance and Combustion Characteristics of a Diesel Engine with Titanium Oxide Coated Piston Using Pongamia Methyl Ester.” Journal of Mechanical Science and Technology 27: 1519–1526. doi:10.1007/s12206-013-0332-3.
- Pradeep Kumar, A., K. Annamalai, and S. Premkartikkumar. 2012. “Performance Comparison of DI Diesel Engine and LHR Engine with Bio Diesel as Fuel.” International Conference on Advances in Engineering, Science and Management (ICAESM). 312–316.
- Qian, G., T. Nakamura, and C. C. Berndt. 1998. “Effects of Thermal Gradient and Residual Stresses on Thermal Barrier Coating Fracture.” Mechanics of Material 27: 91–110.10.1016/S0167-6636(97)00042-2
- Rajendra Prasath, B., P. Tamilporai, and M. F. Shabir. 2010. “Analysis of Combustion, Performance and Emission Characteristics of Low Heat Rejection Engine Using Biodiesel.” International Journal of Thermal Sciences 49: 2483–2490. doi:10.1016/j.ijthermalsci.2010.07.010.
- Ramaswamy, P., S. Seetharamu, K. B. R. Varma, N. Raman, and K. J. Rao. 2000. “Thermomechanical Fatigue Characterization of Zirconia (8% Y2O3-ZrO2) and Mullite Thermal Barrier Coatings on Diesel Engine Components: Effect of Coatings on Engine Performance.” Proceedings of the Institution of Mechanical Engineers, Part C: Journal of Mechanical Engineering Science 214: 729–742.
- Reddy, T. R., M. M. Krishna, C. K. Reddy, and P. Murthy. 2012. “Performance Evaluation of a Low Heat Rejection Diesel Engine with Mohr Oil Based Biodiesel.” British Journal of Applied Science Technology 2 (2): 179–198.10.9734/BJAST
- Sahoo, P. K., and L. M. Das. 2009. “Combustion Analysis of Jatropha, Karanja and Polanga Based Bio-diesel as Fuel in a Diesel Engine.” Fuel 88: 994–999. doi:10.1016/j.fuel.2008.11.012.
- Salman, S., R. Kose, L. Urtekin, and F. Findik. 2006. “An Investigation of Different Ceramic Coating Thermal Properties.” Materials and Design 27: 585–590. doi:10.1016/j.matdes.2004.12.010.
- Soltani, R., H. Samadi, E. Garcia, and T. Coyle. 2005. “Development of Alternative Thermal Barrier Coatings for Diesel Engines.” SAE Paper No, 2005-01-0650. doi:10.4271/2005-01-0650.
- Srithar, K., K. ArunBalasubramanian, M. Vivar, and I. Skryabin. 2013. “An Experimental Investigation on Diesel and Low Heat Rejection Engines with Dual Biodiesel Blends.” International Journal of Green Energy 10: 1041–1055. doi:10.1080/15435075.2012.738448.
- Sun, X., W. Wang, R. Bata, and X. Gao. 1994. “Performance Evaluation of Low Heat Rejection Engines.” Journal of Engineering Gas Turbines Power 116 (4): 758–764. doi:10.1115/1.2906883.
- Suresh, G., H. C. Kamath, and N. R. Banapurmath. 2014. “Studies on the Use of Low-volatile Non-edible Oils in a Thermal Barrier-coated Diesel Engine.” International Journal of Sustainable Engineering 7: 341–351. doi:10.1080/19397038.2013.862581.
- Vassen, R., X. Cao, F. Tietz, D. Basu, and D. Stover. 2000. “Zirconates as New Materials for Thermal Barrier Coatings.” Journal of American Ceramic Society 83 (8): 2023–2028.
- Zhu, D., and R. A. Miller. 1999. Thermal Barrier Coatings for Advanced Gas Turbine and Diesel Engines. NASA/TM—1999-209453, Ohio: Glenn Research Center.